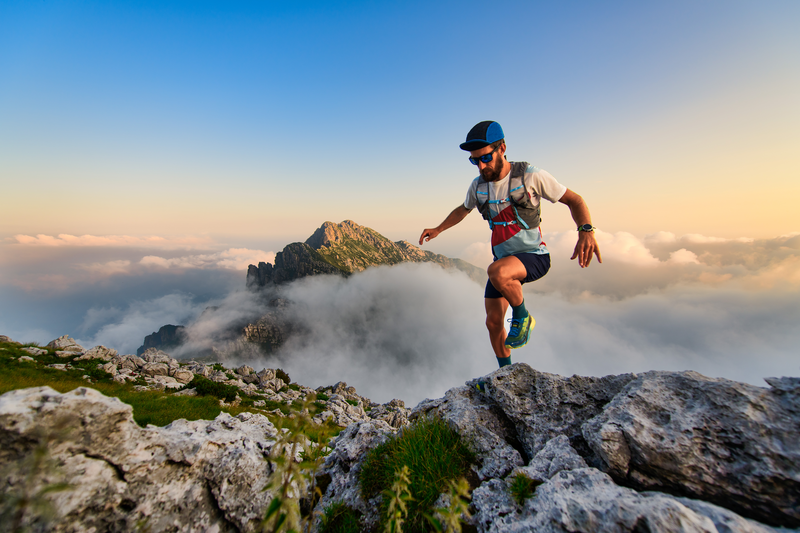
95% of researchers rate our articles as excellent or good
Learn more about the work of our research integrity team to safeguard the quality of each article we publish.
Find out more
REVIEW article
Front. Cardiovasc. Med. , 25 November 2022
Sec. Atherosclerosis and Vascular Medicine
Volume 9 - 2022 | https://doi.org/10.3389/fcvm.2022.1040971
This article is part of the Research Topic Emerging Roles of miRNAs in Cardiovascular Disease View all 6 articles
Atherosclerotic disease is a major cause of morbidity and mortality worldwide. Atherosclerosis may be present in different arterial territories and as a single- or multi-territorial disease. The different phenotypes of atherosclerosis are attributable only in part to acquired cardiovascular risk factors and genetic Mendelian inheritance. miRNAs, which regulate the gene expression at the post-transcriptional level, may also contribute to such heterogeneity. Numerous miRNAs participate in the pathophysiology of atherosclerosis by modulating endothelial function, smooth vascular cell function, vascular inflammation, and cholesterol homeostasis in the vessel, among other biological processes. Moreover, miRNAs are present in peripheral blood with high stability and have the potential to be used as non-invasive biomarkers for the diagnosis of atherosclerosis. However, the circulating miRNA profile may vary according to the involved arterial territory, considering that atherosclerosis expression, including the associated molecular phenotype, varies according to the affected arterial territory. In this review, we discuss the specific circulating miRNA profiles associated with atherosclerosis of different arterial territories, the common circulating miRNA profile of stable atherosclerosis irrespective of the involved arterial territory, and the circulating miRNA signature of multi-territorial atherosclerosis. miRNAs may consist of a simple non-invasive method for discriminating atherosclerosis of different arterial sites. The limitations of miRNA profiling for such clinical application are also discussed.
Atherosclerotic disease is highly prevalent and, despite the recent advances in the therapeutic field, it is still accountable for considerable cardiovascular morbidity and mortality worldwide (1). According to atherosclerosis location, it may present as a localized, single-territorial disease of different arterial territories or a systemic, multi-territorial disease (2). Little is known on the mechanisms that regulate the development of atherosclerosis in different arterial territories and the atherosclerosis extent to single or multiple arterial beds (3, 4). The different phenotypes of atherosclerosis are not only attributable to acquired cardiovascular risk factors and genetic Mendelian inheritance (5, 6). Post-transcriptional regulators, including miRNAs, also contribute to such heterogeneity (4, 7).
miRNAs, non-coding molecules of ribonucleic acid (RNA) of about 20 nucleotides, have received most of the attention during the last decades for their role as dynamic regulators of gene expression in different biological processes (8). They regulate the gene expression at the post-transcriptional level by binding to the target messenger RNA (mRNA), preventing its expression or inducing its degradation (9, 10). Most miRNAs are transcribed from DNA sequences into primary miRNAs (pri-miRNAs) and processed into precursor miRNAs (pre-miRNAs) and mature miRNAs (9). Generally, miRNAs interact with the 3′ UTR of target mRNAs to suppress expression, even though interaction with other regions, including the 5′ UTR, coding sequence, and gene promoters, have also been described (10). miRNAs regulate many biological processes associated with atherogenesis, including cell growth, proliferation, differentiation, migration, senescence, apoptosis, and angiogenesis (11–14). Besides their biological role, miRNAs can be used as diagnostic biomarkers and several studies reported dysregulated levels of circulating miRNAs in patients with atherosclerosis.
Atherosclerosis expression, including the associated molecular phenotype, varies according to the specific arterial territory. In fact, the composition of the atherosclerotic plaque and the levels of different circulating biomarkers vary according to the involved arterial territory (15, 16). Therefore, the circulating miRNA expression profile may vary also according to the involved arterial territory. In this review, after discussing the role of miRNAs in atherosclerosis regulation, we present a critical overview of the specific circulating miRNA profiles associated with atherosclerosis of different arterial territories, the common circulating miRNA profile of stable atherosclerosis irrespective of the involved arterial territory, and the circulating miRNA signature of multi-territorial atherosclerosis.
This review follows the work previously carried out by our research group (15), which assessed the circulating miRNA profiles in atherosclerosis of the carotid, lower extremity, and renal arteries, but not the coronary arteries. This is a more comprehensive review, considering that: atherosclerosis of the coronary territory is covered in this review; recently published articles of circulating miRNAs in coronary and non-coronary territories, which have not been included in prior reviews, are addressed in this review; and the miRNAs expression profile of multi-territory atherosclerosis is included. Of note, a critical analysis of the miRNAs' potential as diagnostic biomarkers of atherosclerosis of different territories, based on pre-established criteria, is presented.
These circulating miRNA profiles may provide clues to the underlying pathophysiology of atherosclerosis of different arterial territories. Importantly, such dysregulated miRNAs are potential non-invasive diagnostic biomarkers of stable atherosclerosis of different vascular beds. To our knowledge, this is the first overview of circulating miRNA profiles according to atherosclerosis of different territories, including the coronary, carotid, lower limbs, renal, and multi-territorial atherosclerosis. A critical analysis regarding robustness of results, based on predefined quality criteria, is presented.
There is growing evidence that different miRNAs are associated with the mediation of key cellular and molecular processes involved in the pathophysiology of atherosclerosis, including atherogenesis and atherosclerotic plaque progression and rupture (11–14, 17–20). An important concept is that some miRNAs participate in multiple pathways and in different steps of a specific pathway, acting as post-transcriptional hubs (11–14, 17, 18). Furthermore, each miRNA may exert atheroprotective effects, proatherogenic effects, or both, which highlights the complexity of atherosclerosis regulation by miRNAs (11–14, 17, 18). The role of miRNAs in the regulation of endothelial cell, monocyte–macrophage, and vascular smooth muscle functions, cholesterol homeostasis, and extracellular matrix composition are discussed below.
The first steps in atherosclerosis development include endothelial dysfunction, followed by inflammatory response and foam cell formation (14). Endothelial cells play an essential role in the occurrence of vascular diseases, particularly atherosclerosis, as they regulate the synthesis and secretion of vasoactive substances, platelet aggregation, leukocyte adhesion, and thrombosis, among other functions (14). In fact, endothelial dysfunction is considered an early marker of atherosclerosis development (14). Different miRNAs, such as miR-21 (21), miR-126 (22–24), miR-146a (25) and miR-221 (26), regulate endothelial cell senescence, proliferation, and migration and influence inflammatory pathways initiated in endothelial cells. Members of the miR-181 family have been shown to suppress the inflammatory response of endothelial cells by targeting mediators of NF-kB pathways (27–29). Also, miR-31 and miR-17-3p are involved in a negative feedback loop that directly inhibits the expression of the adhesion molecules (30). Some miRNAs, including miR-34a (31), miR-217 (32) and miR-146a (33) have important roles in the endothelial aging, which is intimately involved in alterations in the biomechanical and structural properties of the vascular endothelial cells. Interestingly, several miRNAs, including miR-10a (34), miR-19a (35), miR-23b (36), miR-101 (37), and miR-143/145 (38), are inducible by high shear stress and exert atheroprotective effects. Some miRNAs, including miR-21 (13, 21), miR-27b (39), and miR-218 (40), specifically modulate angiogenesis by targeting endothelial cells. Other miRNAs, such as miR-126 (41), miR-221 and miR-222 (42, 43), can also regulate angiogenesis development. Of note, the overexpression of miR-92a (44), miR-129-1 and miR-133 can block this process (45). Angiogenesis facilitates the growth of atherosclerotic lesions and, within the plaques themselves, plays a crucial role in plaque destabilization and rupture. Monocyte–macrophage activity is also regulated by miRNAs (14). In the early stages of atherogenesis, monocytes infiltrate into the sub-endothelium and differentiate into macrophages, uptake low-density lipoprotein (LDL) cholesterol and transform into foam cells, which promote the inflammatory immune responses and plaque formation (4). They also release chemokines and cytokines, that induce acute inflammatory reaction and hereby promote atherosclerosis progression (4, 14). Among other miRNAs, miR-21 (46) and miR-146a (47) modulate monocyte–macrophage activity, including monocyte differentiation, macrophage phenotype, and macrophage-mediated inflammation. MiR-21 is the most abundant miRNA in macrophages, and its overexpression has an anti-inflammatory role (18). MiR-146a does not only decrease the lipid uptake in macrophages, but also inhibits endothelial activation by promoting endothelial nitric oxide synthase expression (8). MiR-125a-5p can also play a protective role in atherosclerosis by regulating the pro-inflammatory response and the uptake of lipids by macrophages (48). On the other hand, miR-155 is crucial for the classical activation of macrophages and expression of inflammatory mediators (49). Vascular smooth muscle cell proliferation, migration, and phenotype influence the composition of the atherosclerotic plaque and are also highly regulated by miRNAs, including miR-21 (13, 14). In experimental models of neointima, there is overexpression of miR-21, miR-146, miR-214, miR-221 and miR-352, whereas miR-125a, miR-125b, miR-133, miR-143, miR-145, and miR-365 are downregulated (13, 17). Systemic cholesterol homeostasis and the LDL inflow into the arterial wall affect the atherosclerotic plaque development and are regulated by different miRNAs, particularly miR-27b (11). Beyond this, miR-33a and miR-33b were also described as key regulators of cholesterol and fatty acid homeostasis, being overexpressed in atherosclerosis (50). Finally, the extracellular matrix composition of the atherosclerotic plaque, including the fibrotic tissue, is associated with the atherosclerotic plaque size and its stability (20); miR-29 is one of the miRNAs that modulate the fibrotic content of the atherosclerotic plaque (51). Therefore, the pathological basis of atherosclerotic plaque formation and development is highly regulated by miRNAs (Figure 1). There are gaps in evidence regarding the regulation of atherosclerosis by miRNAs. In fact, some miRNAs were reported to be dysregulated in atherosclerotic disease, such as miR-363, although their role is not well established in vitro yet. Furthermore, some miRNAs, such as miR-132, may present both proatherogenic and atheroprotective effects and the underlying mechanisms that explain such heterogeneity of effects of each miRNA are not entirely known.
Figure 1. Biological roles of miRNAs in atherosclerosis's pathophysiology. miRNAs with proatherogenic and atheroprotective effects are highlighted in red and green boxes, respectively. VSMC, vascular smooth muscle cell. The figure was partly generated using Servier Medical Art, provided by Servier, licensed under a Creative Commons Attribution 3.0 unported license (https://creativecommons.org/licenses/by/3.0/).
Intracellular miRNAs are released into body fluids, including peripheral blood, and circulating miRNAs remain stable in serum and other body fluids because they are protected from degradation by endogenous RNases (52). Some researchers hypothesized that the stability of circulating miRNAs may be achieved by the high stability of the Ago2 protein and Ago2-miRNA complexes (binding of miRNA to carrier molecules) (53) or due to the loading into lipoprotein complexes (such as HDL) or extracellular vesicles (such as exosomes and microvesicles), and the quantity of miRNA is crucial for its related functional impact (54). Importantly, the high stability of circulating miRNAs may facilitate their use as biomarkers of different diseases, including atherosclerosis. Of note, caution should be taken when assessing the EV-associated nucleic acids function since isolation methods influence the yield of recovery of EV-derived RNA (54).
As aforementioned, the molecular phenotypes of atherosclerosis vary according to the specific arterial territory involved, including the circulating miRNA profiles. Major territories of atherosclerosis include the coronary, carotid, lower limbs, and renal arteries. Moreover, atherosclerosis may be expressed as a single- or multi-territorial disease. The circulating miRNAs profiles for each scenario are herein discussed and a critical overview of robustness of results is presented, based on the reproducibility between studies, validation methods, adjustment in multivariate analysis, and diagnostic accuracy. The selection of studies was based on the knowledge of experts in the field. Of note, data of major systematic reviews were analyzed, including those focused on miRNA profiles in coronary and non-coronary atherosclerosis. Importantly, we focused on stable atherosclerosis but not acute ischemic events considering that the pathophysiology of stable atherosclerosis is associated with the initiation and expression of stable lesions rather than plaque instability and activation of the thrombotic cascade (2–5). We selected studies that assessed miRNAs isolated from serum and plasma, but not from blood cells, since the former are more likely to be used as biomarkers in the near future. The change in expression of miRNAs refers to altered circulating levels of miRNAs as assessed by RT-PCR assays. To evaluate the diagnostic accuracy of miRNAs and their ability to discriminate the presence of atherosclerosis we assessed the area under the ROC curve.
For coronary artery disease (CAD) two systematic reviews reported a miRNA signature, for diagnostic purposes (55, 56). However, the results were based on both acute and stable atherosclerosis, which limits the interpretation of results considering the heterogeneity of included samples. Cardiac-enriched miRNAs, which are expressed abundantly in the heart and play crucial roles in cardiac physiology, were consistently dysregulated in CAD (miR-1, miR-133a, miR-208a/b, and miR-499) compared to controls (55, 56). In addition, the let-7 family, miR-92a, miR-145, miR-142, miR-17 and miR-126, which have a known role in cardiovascular pathophysiology, were also dysregulated in patients with CAD, with high reproducibility of the results (over 10 studies for each miRNA) (25). The identified miRNAs were found to regulate endothelial function and angiogenesis (miR-1 and miR-133), vascular smooth muscle cell differentiation (miR-133 and miR-145), communication between vascular smooth muscle cells and endothelial cells to stabilize plaques (miR-145), apoptosis (miR-1, miR-133, and miR-499), cardiac myocyte differentiation (miR-1, miR-133, miR-145, miR-208, and miR-499), and cardiac hypertrophy (miR-133) (18).
In studies focused on stable CAD, but not acute coronary syndrome, miR-145 (57–59), miR-29b (60), let-7 family (57–59), miR-92a (57, 61) and miR-126 (57, 58, 61, 62) were the most frequently downregulated miRNAs (57, 61, 63, 64), while miR-208, miR-215, miR-487a and miR-502 were up-regulated (60). There was higher reproducibility of results, with replication in different studies for miR-126 (57, 61, 63, 64), miR-145 (57–59), miR-92a (57, 61) and let-7 (58, 63). miR-155 (57, 58, 62) and miR-17 (57, 60, 65) were up-regulated in some studies and down-regulated in others. Importantly, levels of miR-215, miR-502, miR-487, and miR-29b were exclusively dysregulated in stable CAD (and not dysregulated in acute coronary syndrome), and therefore could be regarded as more specific biomarkers of stable CAD. Of note, the levels of some miRNAs are associated with the severity of CAD. In fact, the Gensini score was positively correlated with miR-155 (62) and miR-17-5p (65) expression levels and negatively correlated with miR-145 (58) and miR-126, miR-210 and miR-378 expression levels (61).
Regarding the aforementioned studies that report miRNAs' dysregulation in stable CAD, most were case-control studies (57–61, 63–65). The majority of studies defined CAD as obstructive atherosclerosis (stenosis of at least 50% of one major epicardial artery) on invasive coronary angiography, while controls presented no atherosclerosis (58–60, 62–64). One study defined obstructive CAD as an angiographical stenosis of at least 75% and compared with controls presenting non-significant CAD (65). In four studies, the results were obtained in a derivation cohort and prospectively tested in a validation cohort (57, 59–61). Those studies reported the dysregulation of miR-126 and miR-92a (57, 61), miR-17, miR-155, miR-145 (57), miR196a-5p, miR-3613-3p, miR-145-3p, miR-190a-5p (59), miR-17-5p, miR-210, miR-378 (61) miR-487a, miR-29b, miR-502, miR-208 and miR-215 (60). Of note, most studies of stable CAD (57, 61–65) had a targeted selection of miRNAs while the others had unbiased selection (58–60). Therefore, there may be a bias toward reporting popular miRNAs, considering the candidate-driven approaches.
Regarding adjustments for covariates, after adjustment for LDL-cholesterol, HDL-cholesterol, smoking, statins, angiotensin converter enzyme inhibitors, beta blocker, and calcium channel blockers, logistic regression indicated that lower levels of miR-155, miR-145 and let-7 were independently associated with stable CAD (58).
Some studies reported the area under the curve (AUC) of specific miRNAs to detect stable CAD. The AUC was 0.654 (95% confidence interval [CI]: 0.536–0.773) for let-7, 0.620 (95% CI: 0.491–0.747) for miR-155 and 0.670 (95% CI: 0.556–0.785) for miR-145 to detect CAD, whereas miR-92a showed a very low diagnostic accuracy, with an AUC of 0.520 (95% CI: 0.393–0.646). Interestingly, the combination of let-7, miR-155 and miR-145 resulted in a higher AUC value (0.708, 95% CI: 0.600–0.811), increasing the diagnostic accuracy (58). The AUC of miR-126, miR-17-5p, miR-92a, miR210 and miR-378 for predicting CAD was 0.641 (95% CI: 0.564–0.719), 0.609 (95% CI: 0.526–0.692), 0.622 (95% CI: 0.541–0.702), 0.617 (95% CI: 0.537–0.698) and 0.574 (95% CI: 0.492–0.656), respectively. When combining these five miRNAs, the AUC was 0.756 (95% CI: 0.687–0.725), which indicated a reasonable diagnostic accuracy (61). Of note, miR-17-5p presented an AUC of 0.894 (95% CI: 0.780–0.968) in one study (65). The AUC for combination of miR-487a, miR-502, miR-208 and miR-215, miR-29b was 0.850 (95% CI: 0.734–0.966, p < 0.001) and 0.909 (95% CI: 0.858–0.960; p < 0.001) in training and validation set, respectively (65). Individually, miR-29b (AUC of 0.867; 95% CI: 0.795–0.939) and miR-208 (AUC of 0.886; 95% CI: 0.811–0.941) were better than other miRNAs in discriminating atypical coronary artery disease from controls (60). MiR-196a-5p, miR-3613-3p, miR-145-3p, and miR-190a-5p had had good sensitivity and specificity for distinguishing patients with very early-onset CAD, with an AUC of 0.824 (95% CI: 0.731–0.917), 0.758 (95% CI: 0.651–0.864), 0.753 (95% CI: 0.643–0.863) and 0.782 (95% CI: 0.680–0.884), respectively (59).
In carotid atherosclerosis, miR-21 (66, 67), miR-21-5p (68), miR-29c (69), miR-29a (70), miR-92a (71), miR-211 (67), miR-218 (67) and let-7 (72) were upregulated and miR-30 (73), miR-31 (67), miR-125a-5p (68), miR-126-3p (68), miR-143 (74), miR-145 (74), miR-221 (66), miR-221-3p (68), miR-222-3p (68) and miR-320b (75) downregulated. More recent studies added some data regarding miRNA profiles in carotid atherosclerosis, including the upregulation of miR-342-5p (76), miR-27a (77), and miR-146a (78) and downregulation of miR-181b (77) and miR-503-5p (79).
In most of the studies, the presence of atherosclerosis was assessed by carotid intima-media thickness (CIMT) measured by ultrasonography. Subclinical atherosclerosis was defined as CIMT 0.9–1.2 mm, whereas atherosclerosis was defined as CIMT ≥1.2 mm (68–73). In other studies, carotid atherosclerosis was defined as a carotid stenosis of at least 50% or carotid occlusion, either by ultrasound or angiography (66, 76).
The use of a derivation cohort followed by a validation cohort was reported in a study that assessed the expression levels of miR-21, miR-211, miR-218 and miR-31 (67).
Regarding adjustments for covariates, CIMT was associated with miR-29c levels (β = 0.529, 95% CI: 0.354–0.812; p < 0.001) adjusting for age, body mass index, systolic blood pressure, total cholesterol, and fasting blood-glucose (69). Adjusting for the traditional risk factors (including age, sex, hypertension, diabetes, hyperlipidemia, and smoking history), miR-21 upregulation and miR-221 downregulation were significantly associated with carotid artery disease severity, which was divided into three categories: absence of atherosclerosis, presence of atherosclerosis and clinical history of stroke (miR-21: adjusted p = 0.0047 and miR-221: adjusted p < 0.0001) (66). There was also an independent association between elevated miR-30 expression levels and CIMT in multivariate logistic regression analysis, adjusting for age, sex, body mass index, blood lipid, and blood pressure (β = −0.748, 95% CI: 0.278–0.806; p = 0.006) (73). Multiple linear regression analysis demonstrated that the expression of let-7 was independently associated with CIMT (β = 0.031, 95% CI: 0.015–0.047, p < 0.001) (72).
Regarding the diagnostic accuracy of miRNAs, miR-342-5p and miR-126-3p presented good discriminatory accuracy for detecting carotid atherosclerosis, with an AUC of 0.905 (95% CI not reported) and 0.888 (95% CI: 0.819–0.958), respectively (68, 76). MiR-181b and miR-27a presented reasonable accuracy, with an AUC of 0.791 (95% CI: 0.692–0.904) and 0.750 (95% CI: 0.651–0.885), respectively (77). Combined with traditional risk factors, miR-320b showed an AUC of 0.834 (95% CI: 0.765–0.903) (75), miR-21 an AUC of 0.90 (95% CI: 0.85–0.93) and miR-221 showed an AUC of 0.91 (95% CI: 0.87–0.94) (66). The combination of miR-21 and miR-21 presented a reasonable accuracy, with an AUC of 0.77 (95% CI: 0.72–0.82) (66). Combined with C-reactive protein, miR-29c presented an AUC of 0.900 (95% CI: 0.857–0.944) (69), in the diagnosis of carotid atherosclerosis. Individually, miR-29c also showed a good accuracy with an AUC of 0.870 (95% CI: 0.818–0.922) (69). The receiver operating characteristic curve showed that miR-146a may have some value for predicting plaque vulnerability in patients with carotid atherosclerosis (AUC of 0.64; 95% CI: 0.613–0.769) (78).
Regarding lower limbs atherosclerosis, miR-21 (80), miR-130a (81), miR-210 (80, 81), miR-124, miR-221-5p, miR-4284 (82) miR-411 (81), miR-4739 (83), miR-1827 (84), and miR-124-3p (85) were upregulated, while let-7 (86, 87), miR-15a (87), miR-15b, miR-16, miR-20b, miR-25, miR-26b, miR-28-5p, miR-126, miR-195, miR-335, miR-363 (86), miR-27b (81), miR-196b (87), miR-18a-5p, miR-30e-5p, miR-106a-5p, miR-223-3p, miR-652-3p (88), miR-221-3p, miR-432, miR-4306, and miR-4463 (82) were downregulated. One study showed a downregulation of miR-27b (76), while in other studies it was upregulated (80, 81).
Lower limbs atherosclerosis was defined as the presence of two major symptoms, intermittent claudication, and ischemic rest pain as the main criteria, complemented in some studies with arterial stenosis/occlusion identified on ultrasound imaging or angiography.
A derivation cohort followed by a validation cohort were used in studies that reported the dysregulation of let7e, miR-15b, miR-16, miR-20b, miR-25, miR-26b, miR-27b, miR-28-5p, miR-126, miR-195, miR-335, miR-363 (86) miR-124, miR-221-5p, miR-4284, miR-221-3p, miR-432, miR-4463, miR-4306 (82) and miR-4739 (83). MiR-1827 was evaluated in 3 phases: discovery, confirmation, and validation (84).
Levels of miR-4739 were assessed in patients with type 2 diabetes mellitus, adjusting for diabetes duration, hypertension, smoking, HbA1c, creatinine and total cholesterol (83). In the multivariate analysis, miR-4739 levels were independently associated with critical limb ischemia in patients with diabetes (OR = 12.818, 95% CI 1.148–143.143, p = 0.038) (83).
Regarding the diagnostic accuracy, miR-16 (AUC 0.93; 95% CI: 0.86–1.00; p < 0.001), miR-363 (AUC 0.93; 95% CI: 0.85–1.00; p < 0.001), and miR-15b (AUC 0.92; 95% CI: 0.82–1.00; p < 0.001) presented the highest AUC values. miR-195 (AUC 0.89; 95% CI: 0.79–1.00, p < 0.001), miR-126 (AUC 0.88; 95% CI: 0.77–0.98, p < 0.001), miR-27b (AUC 0.87; 95% CI: 0.75–0.99; p < 0.001), miR-28-5p (AUC 0.86; 95% CI: 0.75–0.97, p < 0.001), miR-26b (AUC 0.77; 95% CI: 0.62–0.93; p < 0.001), miR-335 (AUC of 0.76; 95% CI: 0.61–0.91, p < 0.001), miR-25 (AUC 0.75; 95% CI: 0.59–0.91; p = 0.01), let-7e (AUC 0.71; 95% CI: 0.55–0.88; p = 0.02), and miR-20b (AUC 0.69; 95% CI: 0.52–0.86; p = 0.04) presented good or reasonable diagnostic accuracy (86).
Regarding renal artery atherosclerosis, one study reported an upregulation of miR-126 (89). Data for this territory are less robust considering that it refers to a single study with a relatively small sample.
Considering the miRNAs with the most robust dysregulation in each territory, as discussed before, there are similar trends of dysregulation for the same miRNA in atherosclerosis of different arterial territories, particularly the coronary, carotid and lower limbs atherosclerosis (Figure 2). For instance, miR-126 was downregulated in coronary, carotid and lower limbs atherosclerosis, consisting thus in a common pattern of miRNA dysregulation in atherosclerosis of different territories. miR-126 was reported to be upregulated in renal atherosclerosis, although data are less robust for this territory as aforementioned. In addition, there was a common profile for atherosclerosis of the carotid and lower limbs arteries, consisting of an upregulation of miR-21 and downregulation of miR-30 and miR-221-3p. miR-145 was downregulated in both coronary and carotid territories.
Figure 2. Venn diagram showing the miRNAs shared between carotid, coronary, lower limbs, and renal territories.
Multi-territorial is frequently encountered in clinical practice and is associated with higher morbidity and risk of mortality compared with single-territorial atherosclerosis (16, 90). Multi-territorial atherosclerosis may have a different pathophysiology than that of single-territorial atherosclerosis and circulating miRNA profiles may also differ (16, 89). One study described the circulating miRNA expression profile in single- and multi-territorial atherosclerosis (90). Specifically, lower expression levels of miR-27b and miR-146 were independently associated with the presence of multi-territorial atherosclerosis involving the coronary, lower limbs, and carotid territories, after adjusting for other clinical and laboratory parameters (91). Moreover, a downregulation of miR-27b or miR-146 was associated with higher severity of atherosclerosis in different arterial territories (91). Both miRNAs showed reasonable accuracy for predicting severe systemic atherosclerosis with involvement of the three territories (AUC 0.76, 95% CI 0.60–0.91, p = 0.004 for miR-27b; and AUC 0.75, 95% CI 0.59–0.91, p = 0.009 for miR-126) (91). The expression levels of miR-21, miR-29a, miR-126, and miR-218 did not differ according to the systemic extent of atherosclerosis to multiple territories (91).
According to the four criteria in the “Mirnas As potential biomarkers of stable atherosclerosis” section, we selected a set of the most clinically relevant miRNAs in atherosclerosis of different territories and in multi-territorial atherosclerosis (Tables 1, 2).
In coronary atherosclerosis, the most relevant miRNAs include let-7, miR-29b and miR-208. Let-7 seems to be appropriate for the diagnosis considering that it was downregulated in different studies, the expression levels were adjusted in multivariate analysis and this miRNA showed reasonable diagnostic accuracy. Of note, a multiparameter approach, combining miR-487a, miR-502, miR-208, miR-215, and miR-29b presented a better diagnostic accuracy. On the other hand, miR-29b and miR-208 are also good candidates for diagnostic purposes since these miRNAs were validated in more than one cohort and, individually, presented the highest AUC.
In carotid atherosclerosis, the most relevant miRNAs include miR-21, miR-29c and miR-221. Both miR-21 and miR-221 had good reproducibility between different studies, were adjusted in the multivariate analysis, and had positive data regarding diagnostic accuracy. MiR-29c had a high AUC and its levels were adjusted in multivariate analysis.
In lower limb atherosclerosis the most relevant miRNAs are miR-27b, miR-15b, miR-16 and miR-363. While miR-27b had reproducibility in different studies, was validated in more than one cohort and presented reasonable diagnostic accuracy, miR-15b, miR-16, miR-363 were also validated in different cohorts and presented a higher diagnostic accuracy.
Regarding renal atherosclerosis, no major conclusions can be drawn considering the paucity of data.
The observed dysregulation of miR-27b and miR-146a according to the systemic severity of atherosclerosis and the accuracy of both miRNAs to predict multi-territorial atherosclerosis suggest that these miRNAs are potential diagnostic biomarkers for such a clinical scenario.
Understanding the molecular phenotypes of atherosclerosis may provide insights into its pathophysiology and contribute to identify biomarkers for use in clinical practice. Precise risk stratification tools, which can include circulating biomarkers, are important for cardiovascular risk prediction and the tailoring of treatment strategies, particularly for individuals in higher risk groups. In this review, we assessed the expression of circulating miRNAs in atherosclerosis of major arterial territories, in single- and multi-territorial disease. To the best of our knowledge, we provide the first critical review of circulating miRNA expression profiles in such clinical contexts.
Based on different criteria, we identified the most relevant miRNAs for atherosclerosis diagnosis. These results provide new insights into the role of miRNAs expression in atherosclerosis presentation in clinical practice and may consist of a non-invasive method. The potential diagnostic value of circulating miRNAs has been shown, which allows stratification of systemic atherosclerotic burden. Of note, screening of multi-territorial atherosclerosis in clinical practice by assessing simultaneously different arterial territories using currently available methods is potentially laborious. Therefore, a specific circulation miRNA signature could facilitate the early diagnosis of multi-territorial atherosclerosis.
Since premature atherosclerosis precedes the development of cardiovascular disease, identification of the associated biomarkers is of great importance. The identified miRNA profiles show a promising applicability as biomarkers in primary and secondary prevention of cardiovascular disease. They could be potentially useful for an early intensification of the atheroprotective regimens, such as antithrombotic and lipid-lowering therapies in patients with a higher atherosclerotic burden. Of note, the development of novel non-invasive biomarkers can improve the predictive capacity of current algorithms, beyond the traditional risk factors, as it was shown for some miRNAs.
The different circulating miRNA expression profiles described may also provide clues to pathophysiology of atherosclerosis. Although the pathophysiology of stable atherosclerosis of different territories is out of the scope of this review, the differential miRNA profiles suggest that there is a different pathophysiology of atherosclerosis in different territories. On the other hand, the common miRNA dysregulation in atherosclerosis of different territories suggests that there is a common pathophysiology in atherosclerosis irrespective of its location.
It was not appropriate to pool miRNA expression data since the methodologies of miRNAs analysis and presentation of results of miRNAs expression were heterogeneous and impaired the conversion to a common effect size. We present an objective assessment of their usefulness as diagnostic biomarkers based on four predefined criteria, including the diagnostic accuracy (area under the ROC curve), which are potentially meaningful for the clinical setting.
The selected key miRNAs are known to have a role in the atherosclerotic cascade. Theirs main functional relevance is here summarized.
Let-7 expression is critical for maintaining the integrity of endothelial cells (ECs). Let-7s are abundantly expressed in both ECs and VSMCs. In normal functioning, let-7 is responsible for transducing fibroblast growth factor (FGF) signaling into changes to TGF- β within endothelial cells, thereby limiting proliferation (92, 93). Let-7g has been shown to reduce endothelial cells inflammation and monocyte adhesion (94–97), diminish EC senescence, and play a role in controlling arterial stiffness and aging (98, 99). Moreover, has shown to be reduced in oxLDL treated VSMCs, and this reduction may partially be responsible to the oxLDL-induced cell proliferation and migration.
miR-208 acts on proteins associated with inflammation, endothelial apoptosis, VSMC proliferation and migration, including the PPAR, ACTA2, ROR2 and PI3K/AKT pathways (100). miR-208b overexpression inhibits the expression of both COL1 and ACTA2 (the gene encoding smooth muscle α-actin) by directly targeting and inhibiting GATA4 (101). Overexpression of miR-208b repress (102) and has a protective effect on blood vessels via ACTA2 inhibition (103).
miR-21 inhibits both endothelial cell apoptosis, through PTEN (104), and endothelial cell proliferation, through RhoB (105). It also promotes inflammatory activation of endothelial cells by targeting PPARα, which induces the expression of adhesion molecules and cytokines (106). Furthermore, enhances vascular smooth muscle cell migration and proliferation by targeting TSP-1 and c-Sk (107, 108), and reduces vascular inflammation by suppressing macrophage activity (109).
miR-29 family is highly expressed in fibroblast and vascular smooth cells (VSMC). Besides playing a role in vascular homeostasis, it also regulates genes encoding collagen (COL1A1, COL1A2, COL3A1) and extracellular matrix proteins, including fibrillin (FBN1) and elastin (ELN1) (110).
miR-221 promotes VSMC proliferation by repressing the cyclin-dependent kinase inhibitor p27Kip1 and reduces expression of contractile genes (111). The miR-221 also controls differentiation of ECs but inhibits their proangiogenic activation, proliferation, and migration, so it is implicated in maintaining endothelial integrity and supporting quiescent EC phenotype (102). miR-221 stimulates neointima formation leading to atherogenic calcification of vascular smooth muscle cells (112).
miR-27 was found to be concentrated in the cells associated with atherosclerotic lesions, such as human umbilical vein endothelial cells, smooth muscle cells and macrophage cells (113). In addition, miR-27 plays a key role in the cholesterol biosynthesis pathway, controlling the cholesterol efflux by reducing the expression of ABCA1 and apoA-1, and influx through targeting CD36 expression (114). In mice models, miR-27 regulated the expression of important metabolic genes, including angiopoietin-like 3 (ANGPTL3) and glycerol-3-phosphate acyltransferase 1 (GPAM) (114). miR-27b downregulates lipoprotein lipase gene expression and thereby reduces vascular inflammatory response (115). Moreover, miR-27b restrains the activity of NF-κB and the production of several proinflammatory factors (116). This contributes to a decreased monocyte–macrophage activation (39), which is atheroprotective as it results in decreased vascular inflammation (117). In addition, miR-27b also regulates angiogenesis through the angiogenic inhibitor semaphorin 6A and Notch ligand Dll4 (39, 118).
miR-15b regulates cell proliferation, migration, and apoptosis by regulating the PI3K/AKT signaling pathway. It also significantly inhibited the expression of IGF1R in hVSMCs (119).
miR-16 has potent angiostatic effects by preventing VEGF signaling, EPC senescence, and endothelial proliferation. miR-16 targets VEGFR2 and FGR1 transcripts in endothelial cells (120) and directly silences de VEGF transcript (121, 122). Also, it was found that miR16 was highly expressed in contractile VSMCs by targeting the oncogene yes-associated protein (YAP) (123).
miR-363 is purported to be involved in the angiogenesis homeostasis, including regulation of endothelial cells, expression of angiogenesis factors and interactions between hematopoetic and endothelial cells (124). One study suggested that miR-363-3p, by targeting NOX4 via inactivation of the p38 MAPK signaling pathway, protects against endothelial cell injury caused by inflammation (125).
miR-146a is induced in endothelial cells in response to proinflammatory cytokines and acts as a negative feedback regulator of inflammatory signaling in endothelial cells (126). It inhibits endothelial activation by promoting eNOS expression. On the other hand, the enhancement of miR-146a levels in monocytes and macrophages by cellular apoE was shown to suppress the NF-κB pathway and thus reduce the macrophage activity (127). Moreover, miR-146a targets the Toll-like receptor 4, reducing the formation of foam cells (46).
In addition to diagnosis role, in terms of therapeutics in the atherosclerosis field, the most successful RNA drugs in atherosclerosis are proprotein convertase subtilsin/kexin type 9 (PCSK9) inhibitors, a siRNA drug that targets PCSK9 mRNA (128). Inclisiran was approved in Europe in 2020 for use in adults with primary hypercholesterolaemia or mixed dyslipidaemia (129). Several pharmaceutical and biotech companies are working in the development of miRNA-based therapeutics, mainly miRNA mimics and antogomiRs. There are clinical trials focused on cancer, for example, targeting miR-155 in T-cell lymphoma (130); liver disease, as targeting miR-122 (Miravisen) in HCV infected hepatocytes (131, 132); or structural gastrointestinal diseases, for instance miRNA-124 as a target in inflammatory bowel disease (133). As the application of this biomarkers or intervention targets is increasing and miRNAs have proven to play an important role in vascular dysfunction, atherosclerotic disease should be a contemplated in future clinical trials given the high associated morbidity and mortality. In fact, there are currently some studies in the cardiovascular field. Still in preclinical stage are MGN-1374 (targets: miRNA-15 and miR-195) for the treatment of post-myocardial infarction, MGN-2677 (targets: miR-143/145) for the treatment of vascular disease, MGN-4220 (target: miR-29) for the treatment of cardiac fibrose, MGN-5804 (target: miR-378) for the treatment of cardiometabolic disease, MGN-6114 (target: miR-92) for the treatment of peripheral arterial disease and MGN-9103 (target: miR-208) for the treatment of chronic heart failure. MRG-110 (target: miR-92a) to target blood vessel growth and to control ischemia is in phase-I clinical trial (134, 135). Besides this last one and PCSK9 inhibitors, also IONIS-ANGPTL3-LRX (target: hepatic ANGPTL3 mRNA; NCT02709850) and AKCEA-APOCIII-LRX (target: hepatic APOC3 mRNA; NCT02709850) are in development in the atherosclerosis field. Furthermore, the emerging field on nanomedicine shows promising possibilities for using miRNA which can enhance treatment options.
Given this, panels of the miRNAs identified previously for each atherosclerosis location could be used for diagnosis and the integration of the expression levels could be used for preventive therapy and follow-up.
The identification of dysregulated miRNAs according to different arterial territories, based on available data, has some limitations. Most studies did not effectively exclude atherosclerosis in other territories other than the index territory. Moreover, a direct comparison of atherosclerosis of different territories has not been carried out in the same study and inferences were made using studies focused on different territories. In fact, the samples may have differed across studies and the differences in miRNAs expression may be attributable to unmeasured confounders, including clinical, demographic, and methodological parameters. Of note, there was a heterogeneity of technical approaches for miRNA assessment. Further prospective studies are needed to directly compare the miRNA profiles in atherosclerosis of different arterial territories.
miRNAs regulate different pathways associated with stable atherosclerosis initiation, progression, and expression, and circulating miRNAs are potential biomarkers of stable atherosclerosis. Atherosclerosis of different territories is associated with specific circulating miRNA profiles, including the coronary, carotid, and lower limbs single-territorial atherosclerosis. There is also a common circulating miRNA profile in atherosclerosis irrespective of its location and a miRNA signature in multi-territorial atherosclerosis. These miRNAs may consist of non-invasive biomarkers of atherosclerosis of different territories in clinical practice.
AT: conceptualization and writing. VF: conceptualization. TP-d-S: conceptualization and reviewing. RF: supervision. All authors contributed to the article and approved the submitted version.
The authors declare that the research was conducted in the absence of any commercial or financial relationships that could be construed as a potential conflict of interest.
All claims expressed in this article are solely those of the authors and do not necessarily represent those of their affiliated organizations, or those of the publisher, the editors and the reviewers. Any product that may be evaluated in this article, or claim that may be made by its manufacturer, is not guaranteed or endorsed by the publisher.
1. Virani SS, Alonso A, Aparicio HJ, Benjamin EJ, Bittencourt MS, Callaway CW, et al. Heart disease and stroke statistics−2021 update: a report from the American heart association. Circulation. (2021) 143:e254–743. doi: 10.1161/CIR.0000000000000950
2. Kraler S, Libby P, Evans PC, Akhmedov A, Schmiady MO, Reinehr M, et al. Resilience of the internal mammary artery to atherogenesis: shifting from risk to resistance to address unmet needs. Arterioscler Thromb Vasc Biol. (2021) 41:2237–51. doi: 10.1161/ATVBAHA.121.316256
3. Rothwell P. The Interrelation between carotid, femoral and coronary artery disease. Eur Heart J. (2001) 22:11–4. doi: 10.1053/euhj.2000.2226
4. Fruchart J-C. New risk factors for atherosclerosis and patient risk assessment. Circulation. (2004) 109:III15-9. doi: 10.1161/01.CIR.0000131513.33892.5b
5. Jebari-Benslaiman S, Galicia-García U, Larrea-Sebal A, Olaetxea JR, Alloza I, Vandenbroeck K, et al. Pathophysiology of atherosclerosis. Int J Mol Sci. (2022) 23:3346. doi: 10.3390/ijms23063346
6. Ross R. Atherosclerosis–an inflammatory disease. New Eng J Med. (1999) 340:115–26. doi: 10.1056/NEJM199901143400207
7. Feinberg MW, Moore KJ. MicroRNA regulation of atherosclerosis. Circ Res. (2016) 118:703–20. doi: 10.1161/CIRCRESAHA.115.306300
8. van Rooij E. The art of MicroRNA research. Circ Res. (2011) 108:219–34. doi: 10.1161/CIRCRESAHA.110.227496
9. O'Brien J, Hayder H, Zayed Y, Peng C. Overview of MicroRNA biogenesis, mechanisms of actions, and circulation. Front Endocrinol. (2018) 9:402. doi: 10.3389/fendo.2018.00402
10. Bartel DP. MicroRNAs: target recognition and regulatory functions. Cell. (2009) 136:215–33. doi: 10.1016/j.cell.2009.01.002
11. Donaldson CJ, Lao KH, Zeng L. The salient role of microRNAs in atherogenesis. J Mol Cell Cardiol. (2018) 122:98–113. doi: 10.1016/j.yjmcc.2018.08.004
12. Hosen MR, Goody PR, Zietzer A, Nickenig G, Jansen F. MicroRNAs as master regulators of atherosclerosis: from pathogenesis to novel therapeutic options. Antioxid Redox Signal. (2020) 33:621–644. doi: 10.1089/ars.2020.8107
13. Andreou I, Sun X, Stone PH, Edelman ER, Feinberg MW. miRNAs in atherosclerotic plaque initiation, progression, and rupture. Trends Mol Med. (2015) 21:307–18. doi: 10.1016/j.molmed.2015.02.003
14. Gao Y, Peng J, Ren Z, He N, Li Q, Zhao X, et al. Functional regulatory roles of microRNAs in atherosclerosis. Clinica Chimica Acta. (2016) 460:164–71. doi: 10.1016/j.cca.2016.06.044
15. Pereira-da-Silva T, Coutinho Cruz M, Carrusca C, Cruz Ferreira R, Napoleão P, Mota Carmo M. Circulating microRNA profiles in different arterial territories of stable atherosclerotic disease: a systematic review. Am J Cardiovasc Dis. (2018) 8:1–13.
16. Dalager S, Paaske WP, Bayer Kristensen I, Marsvin Laurberg J, Falk E. Artery-related differences in atherosclerosis expression. Stroke. (2007) 38:2698–705. doi: 10.1161/STROKEAHA.107.486480
17. Churov A, Summerhill V, Grechko A, Orekhova V, Orekhov A. MicroRNAs as potential biomarkers in atherosclerosis. Int J Mol Sci. (2019) 20:5547. doi: 10.3390/ijms20225547
18. Lu Y, Thavarajah T, Gu W, Cai J, Xu Q. Impact of miRNA in atherosclerosis. Arterioscler Thromb Vasc Biol. (2018) 38:e159–70. doi: 10.1161/ATVBAHA.118.310227
19. Madrigal-Matute J, Rotllan N, Aranda JF, Fernández-Hernando C. MicroRNAs and atherosclerosis. Curr Atheroscler Rep. (2013) 15:1–8. doi: 10.1007/s11883-013-0322-z
20. Solly EL, Dimasi CG, Bursill CA, Psaltis PJ, Tan JTM. MicroRNAs as Therapeutic Targets and Clinical Biomarkers in Atherosclerosis. J Clin Med. (2019) 8:2199. doi: 10.3390/jcm8122199
21. Chipont A, Esposito B, Challier I, Montabord M, Tedgui A, Mallat Z, et al. MicroRNA-21 Deficiency Alters the Survival of Ly-6Clo Monocytes in ApoE-/- Mice and Reduces Early-Stage Atherosclerosis-Brief Report. Arterioscler Thromb Vasc Biol. (2019) 39:170–7. doi: 10.1161/ATVBAHA.118.311942
22. Poissonnier L, Villain G, Soncin F, Mattot V. miR126-5p repression of ALCAM and SetD5 in endothelial cells regulates leucocyte adhesion and transmigration. Cardiovasc Res. (2014) 102:436–47. doi: 10.1093/cvr/cvu040
23. Schober A, Nazari-Jahantigh M, Wei Y, Bidzhekov K, Gremse F, Grommes J, et al. MicroRNA-126-5p promotes endothelial proliferation and limits atherosclerosis by suppressing Dlk1. Nat Med. (2014) 20:368–76. doi: 10.1038/nm.3487
24. Cheng X-W, Wan Y-F, Zhou Q, Wang Y, Zhu H-Q. MicroRNA-126 inhibits endothelial permeability and apoptosis in apolipoprotein E-knockout mice fed a high-fat diet. Mol Med Rep. (2017) 16:3061–8. doi: 10.3892/mmr.2017.6952
25. Nguyen M-A, Karunakaran D, Geoffrion M, Cheng HS, Tandoc K, Perisic Matic L, et al. Extracellular Vesicles Secreted by Atherogenic Macrophages Transfer MicroRNA to Inhibit Cell Migration. Arterioscler Thromb Vasc Biol. (2018) 38:49–63. doi: 10.1161/ATVBAHA.117.309795
26. Xue Y, Wei Z, Ding H, Wang Q, Zhou Z, Zheng S, et al. MicroRNA-19b/221/222 induces endothelial cell dysfunction via suppression of PGC-1α in the progression of atherosclerosis. Atherosclerosis. (2015) 241:671–81. doi: 10.1016/j.atherosclerosis.2015.06.031
27. Sun X, Icli B, Wara AK, Belkin N, He S, Kobzik L, et al. MicroRNA-181b regulates NF-κB–mediated vascular inflammation. J Clin Invest. (2012) 122:1973–90. doi: 10.1172/JCI61495
28. Sun X, He S, Wara AKM, Icli B, Shvartz E, Tesmenitsky Y, et al. Systemic delivery of microRNA-181b inhibits nuclear factor-κB activation, vascular inflammation, and atherosclerosis in apolipoprotein E-deficient mice. Circ Res. (2014) 114:32–40. doi: 10.1161/CIRCRESAHA.113.302089
29. Su Y, Yuan J, Zhang F, Lei Q, Zhang T, Li K, et al. MicroRNA-181a-5p and microRNA-181a-3p cooperatively restrict vascular inflammation and atherosclerosis. Cell Death Dis. (2019) 10:365. doi: 10.1038/s41419-019-1599-9
30. Suárez Y, Wang C, Manes TD, Pober JS. Cutting Edge: TNF-Induced MicroRNAs Regulate TNF-Induced Expression of E-Selectin and Intercellular Adhesion Molecule-1 on Human Endothelial Cells: Feedback Control of Inflammation. J Immunol. (2009) 184:21–5. doi: 10.4049/jimmunol.0902369
31. Ito T, Yagi S, Yamakuchi M. MicroRNA-34a regulation of endothelial senescence. Biochem Biophys Res Commun. (2010) 398:735–40. doi: 10.1016/j.bbrc.2010.07.012
32. Menghini R, Casagrande V, Cardellini M, Martelli E, Terrinoni A, Amati F, et al. MicroRNA 217 Modulates Endothelial Cell Senescence via Silent Information Regulator 1. Circulation. (2009) 120:1524–32. doi: 10.1161/CIRCULATIONAHA.109.864629
33. Vasa-Nicotera M, Chen H, Tucci P, Yang AL, Saintigny G, Menghini R, et al. miR-146a is modulated in human endothelial cell with aging. Atherosclerosis. (2011) 217:326–30. doi: 10.1016/j.atherosclerosis.2011.03.034
34. Fang Y, Shi C, Manduchi E, Civelek M, Davies PF. MicroRNA-10a regulation of proinflammatory phenotype in athero-susceptible endothelium in vivo and in vitro. Proc Natl Acad Sci U S A. (2010) 107:13450–5. doi: 10.1073/pnas.1002120107
35. Boon RA, Hergenreider E, Dimmeler S. Atheroprotective mechanisms of shear stress-regulated microRNAs. Thromb Haemost. (2012) 108:616–20. doi: 10.1160/TH12-07-0491
36. Wang K-C, Garmire LX, Young A, Nguyen P, Trinh A, Subramaniam S, et al. Role of microRNA-23b in flow-regulation of Rb phosphorylation and endothelial cell growth. Proc Natl Acad Sci U S A. (2010) 107:3234–9. doi: 10.1073/pnas.0914825107
37. Chen K, Fan W, Wang X, Ke X, Wu G, Hu C. MicroRNA-101 mediates the suppressive effect of laminar shear stress on mTOR expression in vascular endothelial cells. Biochem Biophys Res Commun. (2012) 427:138–42. doi: 10.1016/j.bbrc.2012.09.026
38. Hergenreider E, Heydt S, Tréguer K, Boettger T, Horrevoets AJG, Zeiher AM, et al. Atheroprotective communication between endothelial cells and smooth muscle cells through miRNAs. Nat Cell Biol. (2012) 14:249–56. doi: 10.1038/ncb2441
39. Veliceasa D, Biyashev D, Qin G, Misener S, Mackie AR, Kishore R, et al. Therapeutic manipulation of angiogenesis with miR-27b. Vasc Cell. (2015) 7. doi: 10.1186/s13221-015-0031-1
40. Small EM, Sutherland LB, Rajagopalan KN, Wang S, Olson EN. MicroRNA-218 regulates vascular patterning by modulation of slit-robo signaling. Circ Res. (2010) 107:1336–44. doi: 10.1161/CIRCRESAHA.110.227926
41. Zernecke A, Bidzhekov K, Noels H, Shagdarsuren E, Gan L, Denecke B, et al. Delivery of microRNA-126 by apoptotic bodies induces CXCL12-dependent vascular protection. Sci Signal. (2009) 2:ra81. doi: 10.1126/scisignal.2000610
42. Davis BN, Hilyard AC, Nguyen PH, Lagna G, Hata A. Induction of microRNA-221 by platelet-derived growth factor signaling is critical for modulation of vascular smooth muscle phenotype. J Biol Chem. (2009) 284:3728–38. doi: 10.1074/jbc.M808788200
43. Liu X, Cheng Y, Zhang S, Lin Y, Yang J, Zhang C, et al. necessary role of miR-221 and miR-222 in vascular smooth muscle cell proliferation and neointimal hyperplasia. Circ Res. (2009) 104:476–87. doi: 10.1161/CIRCRESAHA.108.185363
44. Bonauer A, Carmona G, Iwasaki M, Mione M, Koyanagi M, Fischer A, et al. MicroRNA-92a Controls Angiogenesis and Functional Recovery of Ischemic Tissues in Mice. Science. (2009) 324:1710–3. doi: 10.1126/science.1174381
45. Soufi-zomorrod M, Hajifathali A, Kouhkan F, Mehdizadeh M, Rad SMAH, Soleimani M. MicroRNAs modulating angiogenesis: miR-129-1 and miR-133 act as angio-miR in HUVECs. Tumor Biol. (2016) 37:9527–34. doi: 10.1007/s13277-016-4845-0
46. Feng J, Li A, Deng J, Yang Y, Dang L, Ye Y, et al. miR-21 attenuates lipopolysaccharide-induced lipid accumulation and inflammatory response: potential role in cerebrovascular disease. Lipids Health Dis. (2014) 13. doi: 10.1186/1476-511X-13-27
47. Yang K, He YS, Wang XQ, Lu L, Chen QJ, Liu J, et al. MiR-146a inhibits oxidized low-density lipoprotein-induced lipid accumulation and inflammatory response via targeting toll-like receptor 4. FEBS Lett. (2011) 585:854–60. doi: 10.1016/j.febslet.2011.02.009
48. Chen T, Huang Z, Wang L, Wang Y, Wu F, Meng S, et al. MicroRNA-125a-5p partly regulates the inflammatory response, lipid uptake, and ORP9 expression in oxLDL-stimulated monocyte/macrophages. Cardiovasc Res. (2009) 83:131–9. doi: 10.1093/cvr/cvp121
49. Li X, Kong D, Chen H, Liu S, Hu H, Wu T, et al. miR-155 acts as an anti-inflammatory factor in atherosclerosis-associated foam cell formation by repressing calcium-regulated heat stable protein 1. Sci Rep. (2016) 6:21789. doi: 10.1038/srep21789
50. Rayner KJ, Suarez Y, Davalos A, Parathath S, Fitzgerald ML, Tamehiro N, et al. MiR-33 Contributes to the Regulation of Cholesterol Homeostasis. Science. (2010) 328:1570–3. doi: 10.1126/science.1189862
51. Ulrich V, Rotllan N, Araldi E, Luciano A, Skroblin P, Abonnenc M, et al. Chronic miR-29 antagonism promotes favorable plaque remodeling in atherosclerotic mice. EMBO Mol Med. (2016) 8:643–53. doi: 10.15252/emmm.201506031
52. Li Z, Chen D, Wang Q, Tian H, Tan M, Peng D, et al. mRNA and microRNA stability validation of blood samples under different environmental conditions. Forensic Sci Int : Genet. (2021) 55:102567. doi: 10.1016/j.fsigen.2021.102567
53. Turchinovich A, Weiz L, Langheinz A, Burwinkel B. Characterization of extracellular circulating microRNA. Nucleic Acids Res. (2011) 39:7223–33. doi: 10.1093/nar/gkr254
54. Suades R, Greco MF, Padró T, Badimon L. Extracellular vesicles as drivers of immunoinflammation in atherothrombosis. Cells. (2022) 11:1845. doi: 10.3390/cells11111845
55. Navickas R, Gal D, Laucevičius A, Taparauskaite A, Zdanyte M, Holvoet P. Identifying circulating microRNAs as biomarkers of cardiovascular disease: a systematic review. Cardiovasc Res. (2016) 111:322–37. doi: 10.1093/cvr/cvw174
56. Kaur A, Mackin ST, Schlosser K, Wong FL, Elharram M, Delles C, et al. Systematic review of microRNA biomarkers in acute coronary syndrome and stable coronary artery disease. Cardiovasc Res. (2019) 116:1113–24. doi: 10.1093/cvr/cvz302
57. Fichtlscherer S, De Rosa S, Fox H, Schwietz T, Fischer A, Liebetrau C, et al. Circulating MicroRNAs in patients with coronary artery disease. Circ Res. (2010) 107:677–84. doi: 10.1161/CIRCRESAHA.109.215566
58. Faccini J, Ruidavets J-B, Cordelier P, Martins F, Maoret J-J, Bongard V, et al. Circulating miR-155, miR-145 and let-7c as diagnostic biomarkers of the coronary artery disease. Sci Rep. (2017) 7:42916. doi: 10.1038/srep42916
59. Xu J, Lo S. Fundamentals and role of intravascular ultrasound in percutaneous coronary intervention. Cardiovasc Diagn Ther. (2020) 10:1358370–1351370. doi: 10.21037/cdt.2020.01.15
60. Wang J, Pei Y, Zhong Y, Jiang S, Shao J, Gong J. Altered serum MicroRNAs as novel diagnostic biomarkers for atypical coronary artery disease. PLoS ONE. (2014) 9:e107012. doi: 10.1371/journal.pone.0107012
61. Zhang H, Hao J, Sun X, Zhang Y, Wei Q. Circulating pro-angiogenic micro-ribonucleic acid in patients with coronary heart disease. Interact Cardiovasc Thor Surgery. (2018) 27:336–42. doi: 10.1093/icvts/ivy058
62. Qiu X-K, Ma J. Alteration in microRNA-155 level correspond to severity of coronary heart disease. Scand J Clin Lab Invest. (2018) 78:219–23. doi: 10.1080/00365513.2018.1435904
63. Brittan M, Hunter A, Boulberdaa M, Fujisawa T, Skinner EM, Shah AS, et al. Impaired vascular function and repair in patients with premature coronary artery disease. Eur J Prev Cardiol. (2015) 22:1557–66. doi: 10.1177/2047487315600169
64. Jansen F, Yang X, Proebsting S, Hoelscher M, Przybilla D, Baumann K, et al. MicroRNA expression in circulating microvesicles predicts cardiovascular events in patients with coronary artery disease. J Am Heart Assoc. (2014) 3:e001249. doi: 10.1161/JAHA.114.001249
65. Chen J, Xu L, Hu Q, Yang S, Zhang B, Jiang H. MiR-17-5p as circulating biomarkers for the severity of coronary atherosclerosis in coronary artery disease. Int J Cardiol. (2015) 197:123–4. doi: 10.1016/j.ijcard.2015.06.037
66. Tsai P-C, Liao Y-C, Wang Y-S, Lin H-F, Lin R-T, Juo S-HH. Serum microRNA-21 and microRNA-221 as potential biomarkers for cerebrovascular disease. J Vasc Res. (2013) 50:346–54. doi: 10.1159/000351767
67. Zhang J-Y, Gong Y-L, Li C-J, Qi Q, Zhang Q-M, Yu D-M. Circulating MiRNA biomarkers serve as a fingerprint for diabetic atherosclerosis. Am J Transl Res. (2016) 8:2650–8.
68. Zhang X, Shao S, Geng H, Yu Y, Wang C, Liu Z, et al. Expression profiles of six circulating MicroRNAs critical to atherosclerosis in patients with subclinical hypothyroidism: a clinical study. J Clin Endocrinol Metab. (2014) 99:E766–74. doi: 10.1210/jc.2013-1629
69. Huang Y-Q, Li J, Huang C, Feng Y-Q. Plasma MicroRNA-29c levels are associated with carotid intima-media thickness and is a potential biomarker for the early detection of atherosclerosis. Cell Physiol Biochem. (2018) 50:452–9. doi: 10.1159/000494158
70. Liu C, Zhong Q, Huang Y. Elevated plasma miR-29a levels are associated with increased carotid intima-media thickness in atherosclerosis patients. Tohoku J Exp Med. (2017) 241:183–8. doi: 10.1620/tjem.241.183
71. Huang Y, Tang S, Ji-yan C, Huang C, Li J, Cai A, et al. Circulating miR-92a expression level in patients with essential hypertension: a potential marker of atherosclerosis. J Hum Hyp. (2017) 31:200–5. doi: 10.1038/jhh.2016.66
72. Huang Y-Q, Huang C, Chen J-Y, Li J, Feng Y-Q. Plasma expression level of miRNA let-7 is positively correlated with carotid intima-media thickness in patients with essential hypertension. J Hum Hyptens. (2017) 31:843–7. doi: 10.1038/jhh.2017.52
73. Huang Y, Chen J, Zhou Y, Yu X, Huang C, Li J, et al. Circulating miR-30 is related to carotid artery atherosclerosis. Clin Exp Hypertens. (2016) 38:489–94. doi: 10.3109/10641963.2016.1163370
74. Liu K, Xuekelati S, Zhang Y, Yin Y, Li Y, Chai R, et al. Expression levels of atherosclerosis-associated miR-143 and miR-145 in the plasma of patients with hyperhomocysteinaemia. BMC Cardiovas Disord. (2017) 17:163. doi: 10.1186/s12872-017-0596-0
75. Zhang R, Qin Y, Zhu G, Li Y, Xue J. Low serum miR-320b expression as a novel indicator of carotid atherosclerosis. J Clin Neurosci. (2016) 33:252–8. doi: 10.1016/j.jocn.2016.03.034
76. Zhou A, Li Y, Wang P, Yu P, Lang L. Circulating miR-342-5p serves as a diagnostic biomarker in patients with carotid artery stenosis and predicts the occurrence of the cerebral ischemic event. Ir J Med Sci. (2021) 191:713–8. doi: 10.1007/s11845-021-02623-1
77. Jing GUO, Yi-qiang Y, Ming-jie W. Correlation of serum miR-181b and miR-27a expression with the severity of carotid atherosclerosis. Basic Clin Med. (2021) 41:1133.
78. Huang P, He X, Xu M. The role of miRNA-146a and proinflammatory cytokines in carotid atherosclerosis. BioMed Res Int. (2020) 2020:1–7. doi: 10.1155/2020/6657734
79. Yan Z, Wang H, Liang J, Li Y, Li X. MicroRNA-503-5p improves carotid artery stenosis by inhibiting the proliferation of vascular smooth muscle cells. Exp Ther Med. (2020) 20:1–1. doi: 10.3892/etm.2020.9213
80. Li T, Cao H, Zhuang J, Wan J, Guan M, Yu B, et al. Identification of miR-130a, miR-27b and miR-210 as serum biomarkers for atherosclerosis obliterans. Clin Chim Acta. (2011) 412:66–70. doi: 10.1016/j.cca.2010.09.029
81. Signorelli SS, Volsi GL, Pitruzzella A, Fiore V, Mangiafico M, Vanella L, et al. Circulating miR-130a, miR-27b, and miR-210 in patients with peripheral artery disease and their potential relationship with oxidative stress. Angiology. (2016) 67:945–50. doi: 10.1177/0003319716638242
82. He X, Zheng Y, Liu S, Liu Y, He Y, Zhou X. Altered plasma MicroRNAs as novel biomarkers for arteriosclerosis obliterans. J Atheroscler Thromb. (2016) 23:196–206. doi: 10.5551/jat.30775
83. Li J, Cheng B, Wang X, Wang Z, Zhang H, Liu S, et al. Circulating MicroRNA-4739 may be a potential biomarker of critical Limb Ischemia in patients with diabetes. Biomed Res Int. (2018) 2018:1–8. doi: 10.1155/2018/4232794
84. Syed MH, Zamzam A, Valencia J, Khan H, Jain S, Singh KK, et al. MicroRNA profile of patients with chronic limb-threatening Ischemia. Diagnostics. (2020) 10:230. doi: 10.3390/diagnostics10040230
85. Shi Y, Xu X, Luan P, Kou W, Li M, Yu Q, et al. miR-124-3p regulates angiogenesis in peripheral arterial disease by targeting STAT3. Mol Med Rep. (2020) 22:4890–8. doi: 10.3892/mmr.2020.11538
86. Stather PW, Sylvius N, Wild JB, Choke E, Sayers RD, Bown MJ. Differential MicroRNA expression profiles in peripheral arterial disease. Circ Cardiovas Genet. (2013) 6:490–7. doi: 10.1161/CIRCGENETICS.113.000053
87. Stather PW, Sylvius N, Sidloff DA, Dattani N, Verissimo A, Wild JB, et al. Identification of microRNAs associated with abdominal aortic aneurysms and peripheral arterial disease. Br J Surgery. (2015) 102:755–66. doi: 10.1002/bjs.9802
88. Vegter EL, Ovchinnikova ES, van Veldhuisen DJ, Jaarsma T, Berezikov E, van der Meer P, et al. Low circulating microRNA levels in heart failure patients are associated with atherosclerotic disease and cardiovascular-related rehospitalizations. Clin Res Cardiol. (2017) 106:598–609. doi: 10.1007/s00392-017-1096-z
89. Park MY, Herrmann SM, Saad A, Widmer RJ, Tang H, Zhu X-Y, et al. Circulating and renal vein levels of microRNAs in patients with renal artery stenosis. Nephrol Dial Transp. (2014) 30:480–90. doi: 10.1093/ndt/gfu341
90. Hirsch AT. Peripheral arterial disease detection, awareness, and treatment in primary care. JAMA. (2001) 286:1317. doi: 10.1001/jama.286.11.1317
91. Costa MC, Gabriel AF, Selas M, Silva F, et al. Circulating miRNAs are associated with the systemic extent of atherosclerosis: novel observations for miR-27b and miR-146. Diagnostics. (2021) 11:318. doi: 10.3390/diagnostics11020318
92. Deng Z, Wei Y, Yao Y, Gao S, Wang X. Let-7f promotes the differentiation of neural stem cells in rats. Am J Transl Res. (2020) 12:5752–61.
93. Chen P-Y, Qin L, Barnes C, Charisse K, Yi T, Zhang X, et al. FGF regulates TGF-β signaling and endothelial-to-mesenchymal transition via control of let-7 miRNA expression. Cell Rep. (2012) 2:1684–96. doi: 10.1016/j.celrep.2012.10.021
94. Bernstein DL, Zuluaga-Ramirez V, Gajghate S, Reichenbach NL, Polyak B, Persidsky Y, et al. miR-98 reduces endothelial dysfunction by protecting blood–brain barrier (BBB) and improves neurological outcomes in mouse ischemia/reperfusion stroke model. J Cereb Blood Flow Metab. (2019) 40:1953–65. doi: 10.1177/0271678X19882264
95. Rom S, Dykstra H, Zuluaga-Ramirez V, Reichenbach NL, Persidsky Y. miR-98 and let-7g* protect the blood-brain barrier under neuroinflammatory conditions. J Cereb Blood Flow Metab. (2015) 35:1957–65. doi: 10.1038/jcbfm.2015.154
96. Bernstein DL, Rom S. Let-7g* and miR-98 reduce stroke-induced production of proinflammatory cytokines in mouse brain. Front Cell Dev Biol. (2020) 8:632. doi: 10.3389/fcell.2020.00632
97. Kalomoiris S, Cicchetto AC, Lakatos K, Nolta JA, Fierro FA. Fibroblast growth factor 2 regulates high mobility group A2 expression in human bone marrow-derived mesenchymal stem cells. J Cell Biochem. (2016) 117:2128–37. doi: 10.1002/jcb.25519
98. Liao Y-C, Wang Y-S, Guo Y-C, Lin W-L, Chang M-H, Juo S-HH. Let-7g Improves multiple endothelial functions through targeting transforming growth factor-beta and SIRT-1 signaling. J Am Coll Cardiol. (2014) 63:1685–94. doi: 10.1016/j.jacc.2013.09.069
99. Mozos I, Malainer C, Horbańczuk J, Gug C, Stoian D, Luca CT, et al. Inflammatory markers for arterial stiffness in cardiovascular diseases. Front Immunol. (2017) 8:1058. doi: 10.3389/fimmu.2017.01058
100. Zhang X-T, Xu M-G. Potential link between microRNA-208 and cardiovascular diseases. J Xiangya Med. (2021) 6:12. doi: 10.21037/jxym-21-8
101. Zhou C, Cui Q, Su G, Guo X, Liu X, Zhang J. MicroRNA-208b alleviates post-infarction myocardial fibrosis in a rat model by inhibiting GATA4. Med Sci Monit Int Med J Exp Clin Res. (2016) 22:1808–16. doi: 10.12659/MSM.896428
102. Li B, Wang JH-C. Fibroblasts and myofibroblasts in wound healing: force generation and measurement. J Tissue Viabil. (2011) 20:108–20. doi: 10.1016/j.jtv.2009.11.004
103. Cheng J, Zhou X, Jiang X, Sun T. Deletion of ACTA2 in mice promotes angiotensin II induced pathogenesis of thoracic aortic aneurysms and dissections. J Thorac Dis. (2018) 10:4733–40. doi: 10.21037/jtd.2018.07.75
104. Weber M, Baker MB, Moore JP, Searles CD. MiR-21 is induced in endothelial cells by shear stress and modulates apoptosis and eNOS activity. Biochem Biophys Res Commun. (2010) 393:643–8. doi: 10.1016/j.bbrc.2010.02.045
105. Jin C, Zhao Y, Yu L, Xu S, Fu G. MicroRNA-21 mediates the rapamycin-induced suppression of endothelial proliferation and migration. FEBS Lett. (2013) 587:378–85. doi: 10.1016/j.febslet.2012.12.021
106. Zhou J, Wang K-C, Wu W, Subramaniam S, Shyy JY-J, Chiu J-J, et al. MicroRNA-21 targets peroxisome proliferators-activated receptor-alpha in an autoregulatory loop to modulate flow-induced endothelial inflammation. Proc Natl Acad Sci USA. (2011) 108:10355–60. doi: 10.1073/pnas.1107052108
107. Lin X, Zhan J-K, Wang Y-J, Tan P, Chen Y-Y, Deng H-Q, et al. Function, role, and clinical application of MicroRNAs in vascular aging. Biomed Res Int. (2016) 2016:6021394. doi: 10.1155/2016/6021394
108. Li J, Zhao L, He X, Yang T, Yang K. MiR-21 inhibits c-Ski signaling to promote the proliferation of rat vascular smooth muscle cells. Cell Signal. (2014) 26:724–9. doi: 10.1016/j.cellsig.2013.12.013
109. Canfrán-Duque A, Rotllan N, Zhang X, Fernández-Fuertes M, Ramírez-Hidalgo C, Araldi E, et al. Macrophage deficiency of miR-21 promotes apoptosis, plaque necrosis, and vascular inflammation during atherogenesis. Embo Mol Med. (2017) 9:1244–62. doi: 10.15252/emmm.201607492
110. van Rooij E, Sutherland LB, Thatcher JE, DiMaio JM, Naseem RH, Marshall WS, et al. Dysregulation of microRNAs after myocardial infarction reveals a role of miR-29 in cardiac fibrosis. Proc Nat Acad Sci. (2008) 105:13027–32. doi: 10.1073/pnas.0805038105
111. Minami Y, Satoh M, Maesawa C, Takahashi Y, Tabuchi T, Itoh T, et al. Effect of atorvastatin on microRNA 221 / 222 expression in endothelial progenitor cells obtained from patients with coronary artery disease. Eur J Clin Invest. (2009) 39:359–67. doi: 10.1111/j.1365-2362.2009.02110.x
112. Chistiakov DA, Sobenin IA, Orekhov AN, Bobryshev YV. Human miR-221/222 in Physiological and Atherosclerotic Vascular Remodeling. Biomed Res Int. (2015) 2015:354517. doi: 10.1155/2015/354517
113. Zhang M, Wu J-F, Chen W-J, Tang S-L, Mo Z-C, Tang Y-Y, et al. MicroRNA-27a/b regulates cellular cholesterol efflux, influx and esterification/hydrolysis in THP-1 macrophages. Atherosclerosis. (2014) 234:54–64. doi: 10.1016/j.atherosclerosis.2014.02.008
114. Khan AA, Agarwal H, Reddy SS, Arige V, Natarajan B, Gupta V, et al. MicroRNA 27a Is a key modulator of cholesterol biosynthesis. Mol Cell Biol. (2020) 40:e00470-19. doi: 10.1128/MCB.00470-19
115. Xie W, Li L, Zhang M, Cheng H-P, Gong D, Lv Y-C, et al. MicroRNA-27 Prevents atherosclerosis by suppressing lipoprotein lipase-induced lipid accumulation and inflammatory response in apolipoprotein E knockout mice. PLoS ONE. (2016) 11:e0157085. doi: 10.1371/journal.pone.0157085
116. Liang S, Song Z, Wu Y, Gao Y, Gao M, Liu F, et al. MicroRNA-27b Modulates inflammatory response and apoptosis during Mycobacterium tuberculosis infection. J Immunol. (2018) 200:3506–18. doi: 10.4049/jimmunol.1701448
117. Libby P, Ridker PM, Hansson GK. Inflammation in atherosclerosis. J Am Coll Cardiol. (2009) 54:2129–38. doi: 10.1016/j.jacc.2009.09.009
118. Urbich C, Kaluza D, Frömel T, Knau A, Bennewitz K, Boon RA, et al. MicroRNA-27a/b controls endothelial cell repulsion and angiogenesis by targeting semaphorin 6A. Blood. (2012) 119:1607–16. doi: 10.1182/blood-2011-08-373886
119. Sun Y, Gao Y, Song T, Yu C, Nie Z, Wang X. MicroRNA-15b participates in the development of peripheral arterial disease by modulating the growth of vascular smooth muscle cells. Exper Therap Med. (2019) 18:77–84. doi: 10.3892/etm.2019.7552
120. Caporali A, Emanueli C. MicroRNA-503 and the extended microRNA-16 family in angiogenesis. Trends Cardiovasc Med. (2011) 21:162–6. doi: 10.1016/j.tcm.2012.05.003
121. Hua Z, Lv Q, Ye W, Wong C-KA, Cai G, Gu D, et al. MiRNA-directed regulation of VEGF and other angiogenic factors under hypoxia. Valcarcel J PLoS ONE. (2006) 1:e116. doi: 10.1371/journal.pone.0000116
122. Sun Q, Zhou H, Binmadi NO, Basile JR. Hypoxia-inducible factor-1-mediated regulation of semaphorin 4D affects tumor growth and vascularity. J Biol Chem. (2009) 284:32066–74. doi: 10.1074/jbc.M109.057166
123. Xu F, Ahmed ASI, Kang X, Hu G, Liu F, Zhang W, et al. MicroRNA-15b/16 Attenuates vascular neointima formation by promoting the contractile phenotype of vascular smooth muscle through targeting YAP. Arterioscler Thromb Vasc Biol. (2015) 35:2145–52. doi: 10.1161/ATVBAHA.115.305748
124. Costa A, Afonso J, Osório C, Gomes AL, Caiado F, Valente J, et al. miR-363-5p regulates endothelial cell properties and their communication with hematopoietic precursor cells. J Hematol Oncol. (2013) 6:1–12. doi: 10.1186/1756-8722-6-87
125. Zhou T, Li S, Yang L, Xiang D. microRNA-363-3p reduces endothelial cell inflammatory responses in coronary heart disease via inactivation of the NOX4-dependent p38 MAPK axis. Aging. (2021) 13:11061–82. doi: 10.18632/aging.202721
126. Cheng HS, Sivachandran N, Lau A, Boudreau E, Zhao JL, Baltimore D, et al. MicroRNA-146 represses endothelial activation by inhibiting pro-inflammatory pathways. Embo Mol Med. (2013) 5:1017–34. doi: 10.1002/emmm.201202318
127. Li K, Ching D, Luk FS, Raffai RL. Apolipoprotein E enhances MicroRNA-146a in monocytes and macrophages to suppress nuclear factor-κB–driven inflammation and atherosclerosis. Circ Res. (2015) 117:e1–11. doi: 10.1161/CIRCRESAHA.117.305844
130. Anastasiadou E, Seto AG, Beatty X, Hermreck M, Gilles M-E, Stroopinsky D, et al. Cobomarsen, an oligonucleotide inhibitor of miR-155, Slows DLBCL tumor cell growth in vitro and in vivo. Clin Cancer Res. (2021) 27:1139–49. doi: 10.1158/1078-0432.CCR-20-3139
131. Janssen HLA, Reesink HW, Lawitz EJ, Zeuzem S, Rodriguez-Torres M, Patel K, et al. Treatment of HCV infection by targeting MicroRNA. New Eng J Med. (2013) 368:1685–94. doi: 10.1056/NEJMoa1209026
132. Gebert LFR, Rebhan MAE, Crivelli SEM, Denzler R, Stoffel M, Hall J. Miravirsen (SPC3649) can inhibit the biogenesis of miR-122. Nucleic Acids Res. (2013) 42:609–21. doi: 10.1093/nar/gkt852
133. Vermeire S, Sands BE, Tilg H, Tulassay Z, Kempinski R, Danese S, et al. ABX464 (obefazimod) for moderate-to-severe, active ulcerative colitis: a phase 2b, double-blind, randomised, placebo-controlled induction trial and 48 week, open-label extension. Lancet Gastroenterol Hepatol. (2022) 7:1024–35. doi: 10.1016/S2468-1253(22)00233-3
134. Chakraborty C, Sharma AR, Sharma G, Lee S-S. Therapeutic advances of miRNAs: a preclinical and clinical update. J. Adv. Res. (2021) 28:127–38. doi: 10.1016/j.jare.2020.08.012
Keywords: carotid, coronary, diagnostic biomarker, lower limbs, miRNAs, multi-territorial, stable atherosclerosis
Citation: Teixeira AR, Ferreira VV, Pereira-da-Silva T and Ferreira RC (2022) The role of miRNAs in the diagnosis of stable atherosclerosis of different arterial territories: A critical review. Front. Cardiovasc. Med. 9:1040971. doi: 10.3389/fcvm.2022.1040971
Received: 09 September 2022; Accepted: 20 October 2022;
Published: 25 November 2022.
Edited by:
Emiel Van Der Vorst, University Hospital RWTH Aachen, GermanyReviewed by:
Danish Sayed, The State University of New Jersey, United StatesCopyright © 2022 Teixeira, Ferreira, Pereira-da-Silva and Ferreira. This is an open-access article distributed under the terms of the Creative Commons Attribution License (CC BY). The use, distribution or reproduction in other forums is permitted, provided the original author(s) and the copyright owner(s) are credited and that the original publication in this journal is cited, in accordance with accepted academic practice. No use, distribution or reproduction is permitted which does not comply with these terms.
*Correspondence: Ana Rita Teixeira, cml0YS5yaWJlaXJvLnRlaXhlaXJhQGdtYWlsLmNvbQ==
Disclaimer: All claims expressed in this article are solely those of the authors and do not necessarily represent those of their affiliated organizations, or those of the publisher, the editors and the reviewers. Any product that may be evaluated in this article or claim that may be made by its manufacturer is not guaranteed or endorsed by the publisher.
Research integrity at Frontiers
Learn more about the work of our research integrity team to safeguard the quality of each article we publish.