- 1Division of Cardiology, Department of Internal Medicine, Severance Cardiovascular Hospital, Yonsei University College of Medicine, Seoul, Republic of Korea
- 2Department of Radiology, Severance Hospital, Yonsei University College of Medicine, Seoul, Republic of Korea
Background: In this study, we investigate the utility of geometric orifice area (GOA) on cardiac computed tomography (CT) and differences from effective orifice area (EOA) on Doppler echocardiography in patients with bicuspid aortic stenosis (AS).
Methods: A total of 163 patients (age 64 ± 10 years, 56.4% men) with symptomatic bicuspid AS who were referred for surgery and underwent both cardiac CT and echocardiography within 3 months were studied. To calculate the aortic valve area, GOACT was measured by multiplanar CT planimetry, and EOAEcho was calculated by the continuity equation with Doppler echocardiography. The relationships between GOACT and EOAEcho and patient symptom scale, biomarkers, and left ventricular (LV) functional variables were analyzed.
Results: There was a significant but modest correlation between EOAEcho and GOACT (r = 0.604, p < 0.001). Both EOAEcho and GOACT revealed significant correlations with mean pressure gradient and peak transaortic velocity, and the coefficients were higher in EOAEcho than in GOACT. EOAEcho of 1.05 cm2 and GOACT of 1.25 cm2 corresponds to hemodynamic cutoff values for diagnosing severe AS. EOAEcho was well correlated with the patient symptom scale and log NT-pro BNP, but GOACT was not. In addition, EOAEcho had a higher correlation coefficient with estimated LV filling pressure and LV global longitudinal strain than GOACT.
Conclusion: GOACT can be used to evaluate the severity of bicuspid AS. The threshold for GOACT for diagnosing severe AS should be higher than that for EOAEcho. However, EOAEcho is still the method of choice because EOAEcho showed better correlations with clinical and functional variables than GOACT.
Introduction
Aortic stenosis (AS) is a common heart valve disease in an aging society, and its incidence is continuously increasing (1, 2). For several decades, aortic valve (AV) area estimations by Doppler echocardiography have been the gold standard for the assessment of AS severity (3, 4). However, since assumptions and measurement errors affect each component used for calculating the effective orifice area (EOA) with the continuity equation, EOA on echocardiography (EOAEcho) has been criticized for primarily underestimating and sometimes overestimating the actual stenotic area (5).
In the era of transcatheter intervention, structural evaluation through cardiac computed tomography (CT) is actively performed when planning surgery or intervention in patients with symptomatic AS and has been established as a principal examination (6). By direct measurement of CT scans, it is possible to obtain the geometric orifice area (GOACT) of stenotic aortic valves (7). Therefore, in real clinical practice, there is often a difference between EOAEcho and GOACT in the same patient. In particular, the discrepancy between EOAEcho and GOACT is greater in patients with bicuspid AS compared with those with tricuspid AS (3, 8). Previous studies have shown different flow gradient patterns, greater jet eccentricity, and less pressure recovery in patients with bicuspid AS (3, 8). However, data to determine the utility of GOACT on assessment for AS severity are scarce, and there are no studies focusing on bicuspid AS. Therefore, in this study, we investigate the utility of GOACT and differences from EOAEcho in bicuspid aortic stenosis (AS).
Methods
Study population
Using a retrospective and prospective registry that consisted of 1,497 patients with BAV of over 19 years, (9). we identified 163 cases of symptomatic bicuspid AS who underwent transthoracic echocardiography and cardiac CT concomitantly (<3 months between examinations). The registry contains echocardiographic data, laboratory findings, and clinical information from medical records of patients in Severance Cardiovascular Hospital, Seoul, Korea. We excluded patients without symptoms related to AS (dyspnea on exertion, syncope, or chest pain), with endocarditis, with at least moderate dysfunction in mitral, tricuspid, or pulmonic valves, and with previous valve repair or replacement. Combined aortic regurgitation (AR) was identified when the patients had at least moderate AR. For determining clinical and laboratory data, including New York Heart Association (NYHA) class and N-terminal pro-b-type natriuretic peptide (NT-proBNP), information at the time closest to the time of echocardiography and CT was used. Natural log-transformed NT-proBNP values were used due to skewed distributions. Each patient was treated according to the clinical judgment of the physician based on the AS treatment guidelines at the time of diagnosis (10, 11). The Institutional Review Board of Severance Hospital approved this study, which was conducted in compliance with the Declaration of Helsinki. The requirement for informed consent was waived for patients included in the data collection of the retrospective registry and was obtained for the prospective data.
Transthoracic echocardiography
All echocardiographic studies were performed using commercially available equipment and were reviewed by experienced sonographers and imaging cardiologists without knowledge of the clinical data. Standard measurements were performed according to current guidelines (12). Bicuspid AV was diagnosed when only two cusps were unequivocally identified in systole and diastole in the short-axis view, as previously described (8, 9, 13). In all patients, bicuspid AV was confirmed on cardiac CT and echocardiography. Bicuspid AV morphology was classified into the following four types according to the position and pattern of the raphe and cusps, as previously described: type 1: one raphe with a fusion of the left coronary and right coronary cusps; type 2: one raphe with a fusion of the right coronary and non-coronary cusps; type 3, one raphe with a fusion of the left coronary and non-coronary cusps; and type 0: no raphe with two developed cusps (14).
EOAEcho was derived from the continuity equation. The left ventricular outflow tract (LVOT) flow was assessed on the apical three-chamber view. The LVOT diameter was measured during mid-systole 0.5–1 cm below the aortic annulus on the parasternal long-axis view. The velocity–time integral of the LVOT (VTILVOT) was assessed using pulse-wave Doppler. Assuming the circular geometry of the LVOT, the stroke volume was calculated using the following formula: LVOT diameter2 × 0.785 × VTILVOT. The highest peak velocity across the AV, mean pressure gradient (MPG), and velocity-time integral of the AV (TVIAV) were measured from multiple windows using continuous-wave Doppler (Figure 1). All measurements represent an average of three cardiac cycles for patients with sinus rhythm and an average of five cardiac cycles for patients with atrial fibrillation. The severity of AS and combined aortic regurgitation (AR) was assessed based on the current guidelines (15, 16).
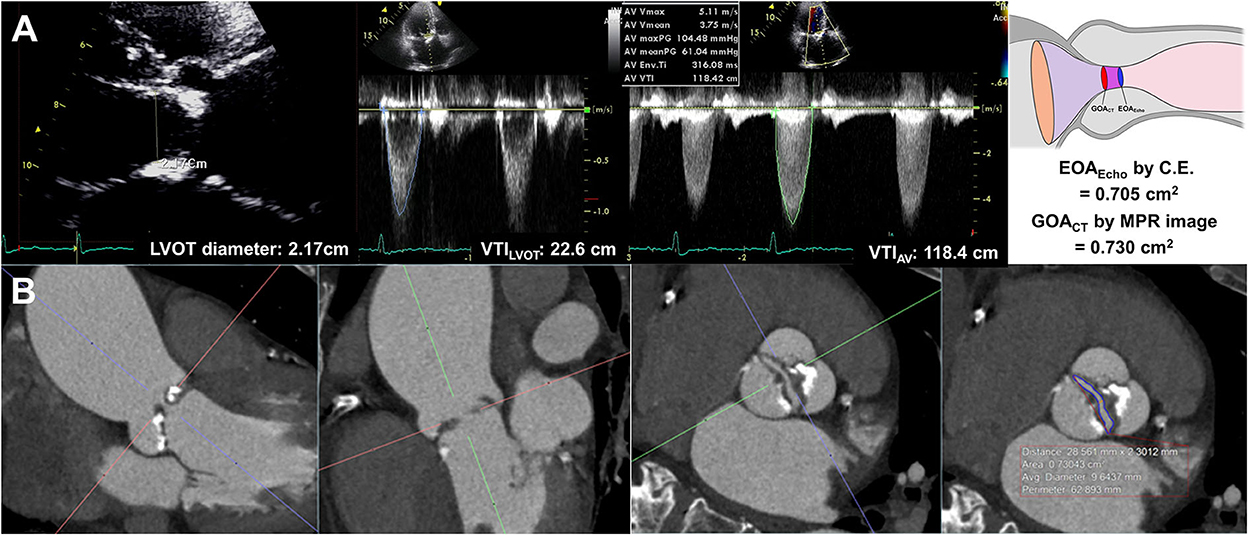
Figure 1. Effective orifice area by continuity equation (A) and geometric orifice area by multiplanar computed tomography image (B).
We calculated LV mass using the corrected American Society of Echocardiography method (17). Relative wall thickness was calculated as follows: (2 × posterior wall thickness/LV end-diastolic dimension). LV ejection fraction was measured using Simpson's biplane method (12). The mitral inflow velocities were recorded with pulsed wave Doppler with the sample volume placed at the tip of the mitral valve from the apical four-chamber views. Tissue Doppler imaging was used to measure mitral annular velocities at the septal corner of the mitral annulus. LV filling pressure was estimated by the early diastolic mitral inflow velocity (E)/early diastolic mitral annular velocity (e') ratio (18). LV global longitudinal strain (GLS) was measured with a two-dimensional speckle tracking analysis on apical two-, three-, and four-chamber views using a vendor-independent software package (TomTec Imaging System; 2D Cardiac Performance Analysis), as described earlier (19). |LV GLS| was defined as the absolute value of LV GLS (removing the conventional negative value of GLS data).
Cardiac CT measurements
All CT scans were performed with a dual-source CT scanner (SOMATOM Definition Flash; Siemens Healthcare, Forchheim, Germany). Before CT angiography, a non-enhanced prospective ECG-gated scan was performed to measure aortic valve calcium score with standardized calcium scan parameters (slice collimation of 0.6 mm, slice width of 3.0 mm, tube voltage of 120 kV, and a tube current of 50 mAs). CT angiography was performed in retrospective ECG-gated data acquisition mode using the triple-phase injection method (70 ml of iopamidol followed by 30 ml of 30% blended iopamidol with saline and 20 ml of saline at 5 ml/s). Images were generated using iterative reconstruction (sinogram-affirmed iterative reconstruction). Image reconstruction was performed with a medium kernel (I36f), and the reconstruction slice thickness was 0.75 mm with 0.5–mm increments. For all patients, 10 transverse data sets were reconstructed every 10% of the cardiac cycle. Image analysis was performed using 3D software (Aquarius iNtuition, Ver. 4.4.11, TeraRecon, San Mateo, CA, USA). For planimetry, the image volume was rotated into a plane perpendicular to the LVOT and aortic root (20). LVOT imaging involved the orientation of a cross-sectional plane of the LVOT using three orthogonal planes from multiplanar reconstruction at or immediately under the lowest implantation base of the aortic cusp, and two orthogonal diameters (minimal and maximal) were measured (4). GOACT was defined as the CT planimetry-derived AV area. Planimetry of the AV area was performed at the level of the aortic leaflet tips in the mid-systolic phase, which provided the best visualization of the open aortic valve, usually at 20–30% of the R-R interval, regardless of BAV types (Figure 1, Supplementary Figure 1). Planimetry of the LVOT was performed immediately below the AV in the same phase used for the measurement of the AV area. The angle between the LVOT-AV and aorta (°) was measured during the mid-systolic phase. The AV calcium score was measured using commercial software (Aquarius iNtuition, Ver. 4.4.11, TeraRecon, San Mateo, CA, USA). Observers segmented the AV calcium score by carefully including the region of interest in the AV leaflet and annulus and excluding calcium in the adjacent sinus of Valsalva, left ventricular outflow tract, or mitral annulus, and image noise or beam hardening artifact was excluded. From the segmented ROI, the AVC volume was measured, and an AVC score was calculated using the Agatston method (21, 22). All CT analyses were independently performed by two radiologists blinded to clinical information, echocardiographic results, and CT analysis results of the other reader. Intraclass correlation coefficients (ICCs) were calculated to assess the inter- and intra-observer variability of the GOACT. A total of 20 samples were assessed by the same observer on different occasions in random order and were also assessed by another observer.
Statistical analysis
The results are expressed as mean ± standard deviation or number (percentage). Differences between EOAEcho and GOACT were analyzed by paired t-test. Correlations and agreements between EOAEcho and GOACT were assessed by Pearson's correlation and Bland–Altman's methods. Correlations between echocardiographic and clinical parameters and EOAEcho or GOACT were determined by Pearson's correlation. Correlations between NYHA functional class and EOAEcho or GOACT were assessed using Kendall's coefficient of rank correlation. Linear regression was used to generate the fitted line for data pairs of EOAEcho and GOACT. Non-linear regression was used to generate the fitted curve for data pairs of conventional Doppler and clinical parameters (MPG, peak flow velocity, NYHA class, log-transformed NT-proBNP, E/e', and LV GLS) and EOAEcho or GOACT. Correlation coefficients between EOAEcho and GOACT were compared using Fisher's Z transformation. Subgroup analysis was performed according to the presence of combined significant AR and LV systolic dysfunction (LVEF <50%). A p-value of < 0.05 was considered statistically significant. Statistical analyses were conducted using R version 4.1.0 (The R Foundation for Statistical Computing; www.R-project.org).
Results
Baseline characteristics
Table 1 shows the baseline characteristics of the study population. The mean age was 64.3 ± 9.6 years, and 92 (56.4%) were male patients. In terms of comorbidities, 88 (53.9%) had hypertension, 36 (22.1%) had diabetes mellitus, and 21 (12.9%) had atrial fibrillation. Type 0 (no-raphe) was the most common BAV phenotype in our study population, followed by Type 1 (RCC+LCC fusion). The average log-transformed NT-proBNP was 6.14 ± 1.50 pg/ml. All patients were symptomatic, and NYHA class II dyspnea was the most common symptom. All patients without dyspnea (NYHA class I) had other AS cardinal symptoms such as chest pain or syncope. The baseline characteristics were not significantly different between patients with concordant and discordant AS degrees (Supplementary Table 1).
Table 2 presents measurement data from echocardiography and cardiac CT. The mean LVEF was 64 ± 12%, and the mean |LV GLS| was 16.3 ± 4.0%. The average LA volume index was 40.5 ± 15.0 ml/m2 and that of E/e' was 15.7 ± 6.1. The mean EOAEcho was 0.76 ± 0.20 cm2. According to EOA criteria, in 147 (90.2%) patients, the AV area was < 1.0 cm2, indicating severe AS. In the values measured through cardiac CT, the average GOACT was 0.83 ± 0.22 cm2, which was significantly higher than EOAEcho (mean difference: 0.07 ± 0.19 cm2, p < 0.001). There was a significant but modest correlation between EOAEcho and GOACT (r = 0.604, p < 0.001; Figure 2). The correlations were significant in both sinus rhythm (r = 0.555, p < 0.001) and atrial fibrillation (r = 0.783, p < 0.001, p for interaction=0.088). The correlations were also significant in BAV type 0 (r = 0.494, p < 0.001) and other types (r = 0.647, p < 0.001, p for interaction = 0.152). When the diagnostic criteria for severe AS through GOA were applied to < 1.0 cm2, the number of patients corresponding to severe AS was 136 (83.4%), which was reduced compared to the number of patients identified by EOAEcho as having severe AS. That is, there were 19 (11.7%) patients with discrepant AS severity evaluated by EOAEcho and GOACT. The inter- (ICC = 0.984; P < 0.001) and intra-observer variabilities (ICC = 0.976, P < 0.001) for the GOACT showed excellent agreement.
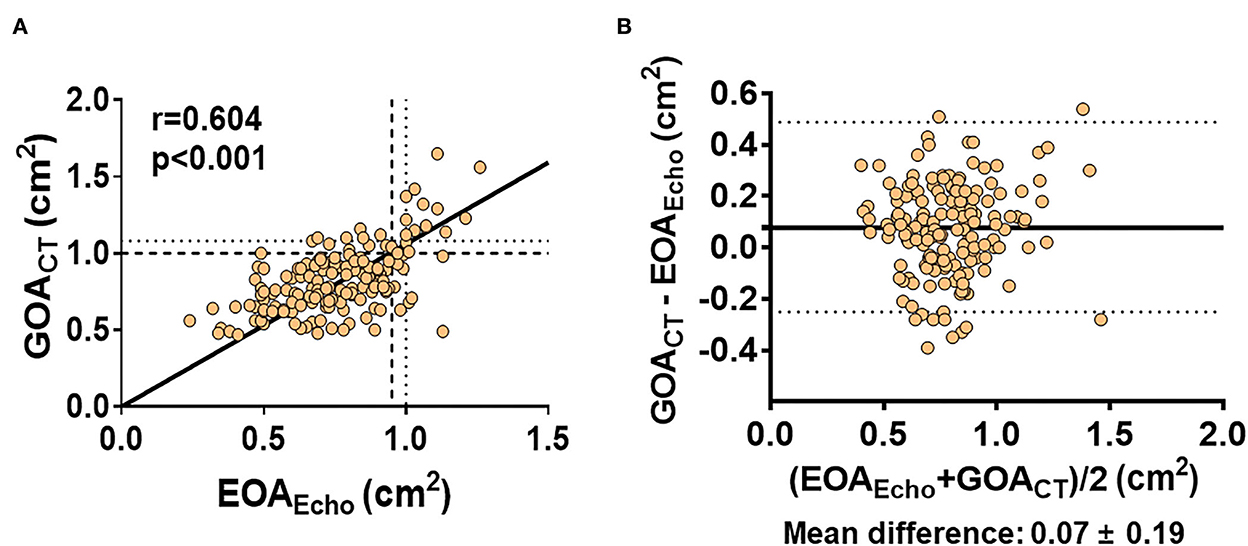
Figure 2. (A,B) Correlation between EOAEcho and GOACT. EOAEcho 1.0 cm2 correspond to GOACT of 1.08 cm2 (dotted line). GOACT 1.0 cm2 corresponded to EOAEcho of 0.95 cm2 (dashed line).
EOA vs. GOA: Correlations with transaortic velocity and MPG
Both EOAEcho and GOACT showed modest correlations with transaortic MPG and peak velocity (all p < 0.001; Figure 3). The correlation coefficients between the two methods were not significantly different. The correlations were consistent according to combined AR or EF (Supplementary Figure 2). MPG 40 mmHg corresponded to EOAEcho of 1.05 cm2 and GOACT of 1.25 cm2. The transaortic peak flow velocity of 4 m/s corresponded to EOAEcho of 1.12 cm2 and GOACT of 1.28 cm2, respectively (Figure 3).
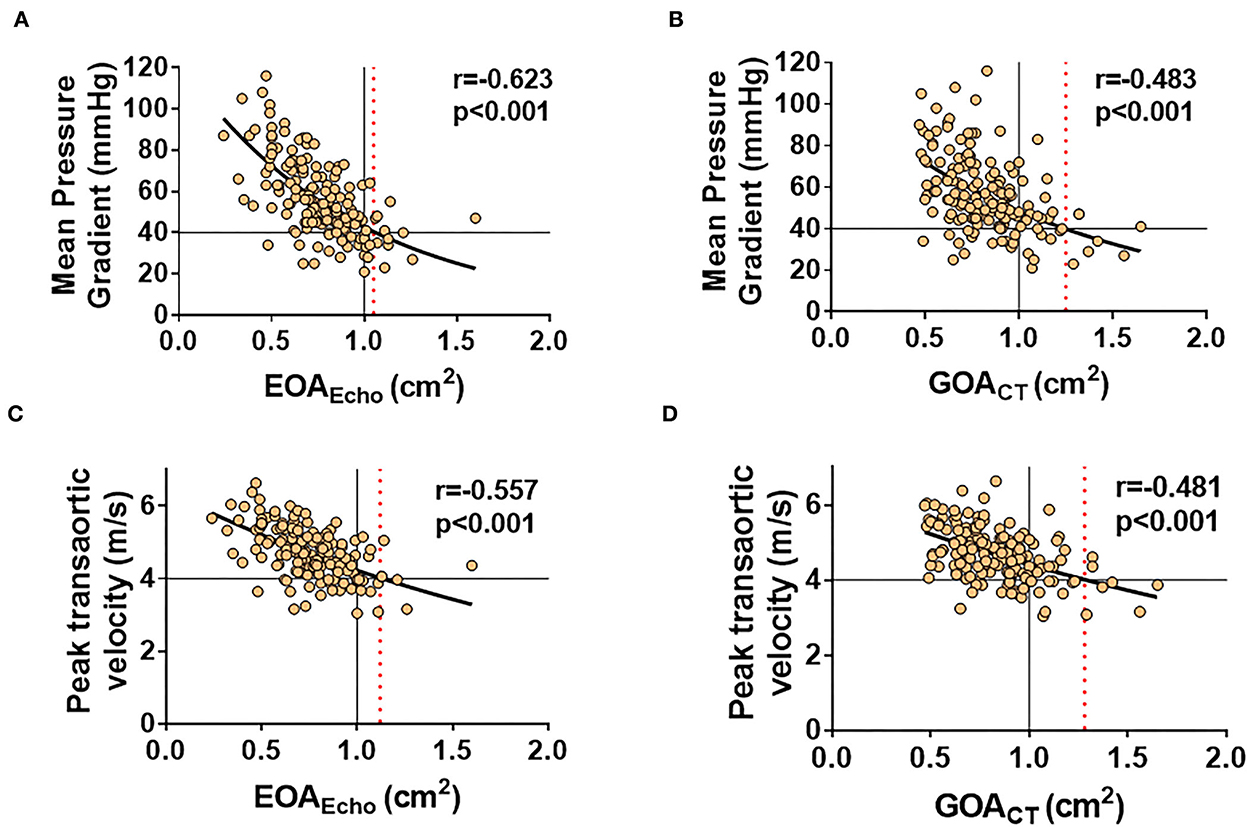
Figure 3. Correlations of EOAEcho and GOACT to hemodynamic variables across the stenotic valve. MPG 40 mmHg corresponded to EOAEcho of 1.05 cm2 (A) and GOACT of 1.25 cm2 (B). The transaortic peak flow velocity of 4 m/s corresponded to EOAEcho of 1.12 cm2 (C) and GOACT of 1.28 cm2 (D), respectively.
EOA vs. GOA: Correlations with patient symptoms, biomarkers, and LV function
EOAEcho showed weak correlations with NYHA class (r = −0.287, p < 0.001) and modest correlations with log-transformed NT-proBNP (r = −0.381, p = 0.001). GOACT showed weak correlations with NYHA class (r = −0.139, p = 0.023), but not with log-transformed NT-proBNP (r = −0.141, p = 0.193) (Figure 4). Table 3 shows correlations of AV area to functional echocardiographic parameters and biomarkers. EOAEcho had better correlations with LA volume index, E/e', LVEF, and LV GLS than GOACT. These correlations were similar according to the LVEF subgroup (Supplementary Figure 3).
Discussion
The main findings of the present study are as follows: (1) In bicuspid AS, there was a significant correlation between EOAEcho and GOACT, but the degree of correlation was modest. (2) Both EOAEcho and GOACT had modest correlations with conventional hemodynamic parameters determining AS severity. (3) Transaortic MPG of 40 mmHg corresponds to EOAEcho of 1.05 cm2 and GOACT of 1.25 cm2. (4) EOAEcho had better correlations with echocardiographic parameters indicating LV systolic and diastolic function, regardless of EF status, than GOACT. These findings suggest that GOACT is an attractive alternative to EOAEcho due to the direct measurement of stenotic valve area, but diagnostic criteria for severe AS should be applied as a higher cutoff compared to EOAEcho, and there are limitations in that the correlation with LV functional alteration is less than that of EOAEcho. To the best of our knowledge, this is the first study to investigate the utility of GOACT on bicuspid AS assessment and comprehensive comparison between EOAEcho and GOACT in bicuspid AS.
Assessment of AS severity
According to current guidelines, severe AS is defined as EOAEcho of <1.0 cm2, transaortic peak velocity of > 4.0 m/s, or MPG of > 40 mmHg (11, 23). Doppler echocardiography remains the standard method for assessing AS severity. Cardiac CT is particularly useful in patients with poor acoustic windows and provides additional anatomical information about both adjacent structures and the aortic valve itself for planning surgical or transcatheter aortic valve replacement (7, 24, 25). In tricuspid AS, EOAEcho showed a significant correlation with GOACT (4). However, there were some discrepancies in AS severity between echocardiographic parameters and GOA measurement by cardiac CT (5, 24).
In bicuspid AS, the functional severity measured by Doppler echocardiography tends to be more severe than that of tricuspid AS because of greater jet eccentricity and less pressure recovery, resulting in higher energy loss (3, 26). As a result, it has been reported that there was a greater discrepancy between EOAEcho and GOACT in bicuspid AS compared with tricuspid AS (3). However, no study had confirmed the associations with hemodynamic variables of the AV and LV or evaluated clinical significance in patients with bicuspid AS. Therefore, we believe that the modest correlation observed between EOAEcho and GOACT, the fact that valve area measured by both methods has good correlations with AV hemodynamic variables, and the stronger association of EOAEcho with LV functional variables observed will help clinicians better understand the AV area evaluated by multimodal imaging.
A previous study that reported inconsistencies of echocardiographic criteria for the grading of AS showed that MPG 40 mmHg corresponds to EOAEcho of 0.75 cm2 and peak velocity of 4.0 m/s to EOAEcho of 0.82 cm2 (5). In the present study, we observed higher corresponding EOAEcho and GOACT values with conventional echocardiographic parameter thresholds than previously reported values. Since the previous study was not limited to bicuspid AS, the majority of patients must have had tricuspid AS (5). Therefore, the larger EOAEcho corresponding to MPG 40 mmHg or peak velocity of 4.0 m/s is consistent with the results of previous studies showing higher gradients in bicuspid AS than in tricuspid AS (8, 27, 28). To the best of our knowledge, the present study is the first to provide a threshold for severe bicuspid AS assessed by cardiac CT. Further studies are needed to elucidate the prognosis of bicuspid severe AS determined by cardiac CT.
Differences in valve area in multimodal imaging
A previous study conducted head-to-head comparisons of valve area calculation by Doppler echocardiography and CT in 269 patients with calcified AS (4). They found that AV area measured by CT resulted in higher values than EOAEcho and had higher cutoff values (1.2 cm2) to predict mortality under medical treatment. However, AVACT did not improve concordance for AS grading or outcome prediction. Although in the present study, we did not examine clinical outcomes according to AV area, we evaluated clinical significance on LV alteration as we observed better correlations of EOAEcho with estimated LV filling pressure, LA volume index, and LV global longitudinal strain than GOACT. As LV GLS and the extent of cardiac damage associated with AS have important prognostic implications, our results indicate that EOAEcho is meaningful as a gold standard for AV area calculation, similar to the results of a previous study of tricuspid AS (4, 29, 30). In addition, we observed higher corresponding values of GOACT to MSPG > 40 mmHg, which indicates severe AS, than EOAEcho. This suggests that it is desirable to use a larger cutoff value for GOACT when using GOACT in cases where EOAEcho assessment is unreliable or impossible.
Study limitations
First, this study was a retrospective cross-sectional study from a single tertiary center and thus has intrinsic limitations. Clinical events and follow-up data of patients were not analyzed in this study. However, we provide clinically important information regarding AV area calculation from two different imaging modalities in patients with symptomatic bicuspid AS. Second, data to determine invasive hemodynamic data were not available in the present study. However, invasive hemodynamic data are rarely used to assess AS in clinical practice and current guidelines recommend the use of cardiac catheterization in poor echocardiographic windows and low-flow status. Our study subjects had a mean stroke volume index of 48.4 ± 10.2 ml/m2, which means most of our study population had normal-flow AS. Third, because this study was conducted among patients with severe bicuspid AS who were referred to surgery, the results of this study cannot be equally generalized to a wider patient range, that is, mild-to-moderate patients with AS or asymptomatic patients. Fourth, the present study was cross-sectional in design, and extensive cardiac damage might have been present before AS. However, bicuspid AS occurs in relatively younger and healthier patients than tricuspid AS (31). We believe that most of our study subjects had AS-associated structural abnormalities. Fifth, the study population underwent Doppler echocardiography and CT within 3 months, not simultaneously. The time difference between echocardiography and CT might result in discordance between hemodynamic parameters and GOACT. In the future, well-designed comparisons are warranted to clarify our findings and determine the clinical impact of GOACT in bicuspid AS. Sixth, not all patients underwent NT-proBNP tests, resulting in potential selection bias. Further prospective studies of the prognostic impact of EOAEcho and GOACT are needed.
Conclusion
GOACT can be used to evaluate the severity of bicuspid AS. The threshold for GOACT for diagnosing severe AS should be higher than that for EOAEcho. However, EOAEcho is still the method of choice because EOAEcho showed better correlations with clinical and functional variables than GOACT. These findings suggest that EOAEcho should be the method of choice to assess bicuspid AS severity, but GOACT is an effective alternative in patients with poor echocardiographic image quality.
Data availability statement
The raw data supporting the conclusions of this article will be made available by the authors, without undue reservation.
Ethics statement
The studies involving human participants were reviewed and approved by the Institutional Review Board of Severance Hospital. Written informed consent for participation was not required for this study in accordance with the national legislation and the institutional requirements.
Author contributions
KK, YK, and CS contributed to conception and design of the study. KK, SL, JS, IC, G-RH, and J-WH organized the database. KK performed the statistical analysis and wrote the first draft of the manuscript. KK, YS, YK, and CS wrote sections of the manuscript. All authors contributed to manuscript revision, read, and approved the submitted version.
Funding
This research was supported by the Korean Cardiac Research Foundation (202103-02). The funders had no role in study design, data collection and analysis, the decision to publish, and the preparation of the manuscript.
Acknowledgments
The authors would like to thank Medical Illustration and Design, part of the Medical Research Support Services of Yonsei University College of Medicine, for all the artistic support related to this work.
Conflict of interest
The authors declare that the research was conducted in the absence of any commercial or financial relationships that could be construed as a potential conflict of interest.
Publisher's note
All claims expressed in this article are solely those of the authors and do not necessarily represent those of their affiliated organizations, or those of the publisher, the editors and the reviewers. Any product that may be evaluated in this article, or claim that may be made by its manufacturer, is not guaranteed or endorsed by the publisher.
Supplementary material
The Supplementary Material for this article can be found online at: https://www.frontiersin.org/articles/10.3389/fcvm.2022.1035244/full#supplementary-material
Abbreviations
AR, aortic regurgitation; AS, aortic stenosis; AV, aortic valve; AVA, aortic valve area; CT, computed tomography; EOA, effective orifice area; EF, ejection fraction; GOA, geometric orifice area; GLS, global longitudinal strain; LBBB, left bundle branch block; LV, left ventricle; LVOT, left ventricular outflow track; MPG, mean pressure gradient; NYHA, New York Heart Association; NT-proBNP, N terminal pro-brain natriuretic peptide; SAVR, surgical aortic valve replacement; TAVR, transcatheter aortic valve replacement; VTI, velocity-time integral.
References
1. Coffey S, Roberts-Thomson R, Brown A, Carapetis J, Chen M, Enriquez-Sarano M, et al. Global epidemiology of valvular heart disease. Nat Rev Cardiol. (2021) 18:853–64. doi: 10.1038/s41569-021-00570-z
2. Yadgir S, Johnson CO, Aboyans V, Adebayo OM, Adedoyin RA, Afarideh M, et al. Global, regional, and national burden of calcific aortic valve and degenerative mitral valve diseases, 1990-2017. Circulation. (2020) 141:1670–80. doi: 10.1161/CIR.0000000000000848
3. Donal E, Novaro GM, Deserrano D, Popovic ZB, Greenberg NL, Richards KE, et al. Planimetric assessment of anatomic valve area overestimates effective orifice area in bicuspid aortic stenosis. J Am Soc Echocardiogr. (2005) 18:1392–8. doi: 10.1016/j.echo.2005.04.005
4. Clavel MA, Malouf J, Messika-Zeitoun D, Araoz PA, Michelena HI, Enriquez-Sarano M. Aortic valve area calculation in aortic stenosis by CT and Doppler echocardiography. JACC Cardiovasc Imaging. (2015) 8:248–57. doi: 10.1016/j.jcmg.2015.01.009
5. Minners J, Allgeier M, Gohlke-Baerwolf C, Kienzle RP, Neumann FJ, Jander N. Inconsistencies of echocardiographic criteria for the grading of aortic valve stenosis. Eur Heart J. (2008) 29:1043–8. doi: 10.1093/eurheartj/ehm543
6. Shim CY, Hong GR. Timing and indications for aortic valve surgery in Korean bicuspid aortic valve patients. Korean Circ J. (2018) 48:82–3. doi: 10.4070/kcj.2017.0380
7. Kim YJ, Yong HS, Kim SM, Kim JA, Yang DH, Hong YJ, et al. Korean guidelines for the appropriate use of cardiac CT. Korean J Radiol. (2015) 16:251–85. doi: 10.3348/kjr.2015.16.2.251
8. Kim D, Shim CY, Kim YJ, Nam K, Hong GR, Lee SH, et al. Differences in flow-gradient patterns between severe bicuspid aortic stenosis and severe tricuspid aortic stenosis- mechanistic insight from multimodal imaging. Circ J. (2019) 84:119–26. doi: 10.1253/circj.CJ-19-0702
9. Kim K, Kim DY, Seo J, Cho I, Hong GR, Ha JW, et al. Temporal trends in diagnosis, treatments, and outcomes in patients with bicuspid aortic valve. Front Cardiovasc Med. (2021) 8:766430. doi: 10.3389/fcvm.2021.766430
10. Otto CM, Nishimura RA, Bonow RO, Carabello BA, Erwin JP, Gentile F, et al. 2020 ACC/AHA guideline for the management of patients with valvular heart disease: executive summary: a report of the American college of cardiology/American heart association joint committee on clinical practice guidelines. Circulation. (2021) 143:e35–71. doi: 10.1161/CIR.0000000000000932
11. Vahanian A, Beyersdorf F, Praz F, Milojevic M, Baldus S, Bauersachs J, et al. 2021 ESC/EACTS guidelines for the management of valvular heart disease. Eur Heart J. (2021) 41:561–632. doi: 10.1093/ejcts/ezac209
12. Lang RM, Badano LP, Mor-Avi V, Afilalo J, Armstrong A, Ernande L, et al. Recommendations for cardiac chamber quantification by echocardiography in adults: an update from the American society of echocardiography and the European association of cardiovascular imaging. J Am Soc Echocardiogr. (2015) 28:1–39. doi: 10.1016/j.echo.2014.10.003
13. Lee SY, Shim CY, Hong GR, Cho IJ, Chang HJ, Ha JW, et al. Determinants and prognostic significance of symptomatic status in patients with moderately dysfunctional bicuspid aortic valves. PLoS ONE. (2017) 12:e0169285. doi: 10.1371/journal.pone.0169285
14. Sievers HH, Schmidtke C. A classification system for the bicuspid aortic valve from 304 surgical specimens. J Thorac Cardiovasc Surg. (2007) 133:1226–33. doi: 10.1016/j.jtcvs.2007.01.039
15. Baumgartner H, Hung J, Bermejo J, Chambers JB, Edvardsen T, Goldstein S, et al. Recommendations on the echocardiographic assessment of aortic valve stenosis: a focused update from the European association of cardiovascular imaging and the American society of echocardiography. J Am Soc Echocardiogr. (2017) 30:372–92. doi: 10.1016/j.echo.2017.02.009
16. Zoghbi WA, Adams D, Bonow RO, Enriquez-Sarano M, Foster E, Grayburn PA, et al. Recommendations for noninvasive evaluation of native valvular regurgitation: a report from the American society of echocardiography developed in collaboration with the society for cardiovascular magnetic resonance. J Am Soc Echocardiogr. (2017) 30:303–71. doi: 10.1016/j.echo.2017.01.007
17. Devereux RB, Alonso DR, Lutas EM, Gottlieb GJ, Campo E, Sachs I, et al. Echocardiographic assessment of left ventricular hypertrophy: comparison to necropsy findings. Am J Cardiol. (1986) 57:450–8. doi: 10.1016/0002-9149(86)90771-X
18. Nagueh SF, Smiseth OA, Appleton CP, Byrd BF, Dokainish H, Edvardsen T, et al. Recommendations for the evaluation of left ventricular diastolic function by echocardiography: an update from the American society of echocardiography and the European association of cardiovascular imaging. J Am Soc Echocardiogr. (2016) 29:277–314. doi: 10.1016/j.echo.2016.01.011
19. Voigt JU, Pedrizzetti G, Lysyansky P, Marwick TH, Houle H, Baumann R, et al. Definitions for a common standard for 2D speckle tracking echocardiography: consensus document of the EACVI/ASE/industry task force to standardize deformation imaging. J Am Soc Echocardiogr. (2015) 28:183–93. doi: 10.1016/j.echo.2014.11.003
20. Halpern EJ, Mallya R, Sewell M, Shulman M, Zwas DR. Differences in aortic valve area measured with CT planimetry and echocardiography (continuity equation) are related to divergent estimates of left ventricular outflow tract area. AJR Am J Roentgenol. (2009) 192:1668–73. doi: 10.2214/AJR.08.1986
21. Agatston AS, Janowitz WR, Hildner FJ, Zusmer NR, Viamonte M, Detrano R. Quantification of coronary artery calcium using ultrafast computed tomography. J Am Coll Cardiol. (1990) 15:827–32. doi: 10.1016/0735-1097(90)90282-T
22. Kang NG, Suh YJ, Han K, Kim YJ, Choi BW. Performance of prediction models for diagnosing severe aortic stenosis based on aortic valve calcium on cardiac computed tomography: incorporation of radiomics and machine learning. Korean J Radiol. (2021) 22:334–43. doi: 10.3348/kjr.2020.0099
23. Writing Committee M, Otto CM, Nishimura RA, Bonow RO, Carabello BA, Erwin JP, et al. 2020 ACC/AHA guideline for the management of patients with valvular heart disease: a report of the American college of cardiology/American heart association joint committee on clinical practice guidelines. J Am Coll Cardiol. (2021) 77:e25–197. doi: 10.1016/j.jacc.2020.11.018
24. Park SJ, Dweck MR. Multimodality imaging for the assessment of severe aortic stenosis. J Cardiovasc Imaging. (2019) 27:235–46. doi: 10.4250/jcvi.2019.27.e48
25. Jilaihawi H, Chen M, Webb J, Himbert D, Ruiz CE, Rodes-Cabau J, et al. A Bicuspid aortic valve imaging classification for the TAVR Era. JACC Cardiovasc Imaging. (2016) 9:1145–58. doi: 10.1016/j.jcmg.2015.12.022
26. Richards KE, Deserranno D, Donal E, Greenberg NL, Thomas JD, Garcia MJ. Influence of structural geometry on the severity of bicuspid aortic stenosis. Am J Physiol Heart Circ Physiol. (2004) 287:H1410–6. doi: 10.1152/ajpheart.00264.2003
27. Eleid MF, Sorajja P, Michelena HI, Malouf JF, Scott CG, Pellikka PA. Flow-gradient patterns in severe aortic stenosis with preserved ejection fraction: clinical characteristics and predictors of survival. Circulation. (2013) 128:1781–9. doi: 10.1161/CIRCULATIONAHA.113.003695
28. Shen M, Tastet L, Capoulade R, Larose E, Bedard E, Arsenault M, et al. Effect of age and aortic valve anatomy on calcification and haemodynamic severity of aortic stenosis. Heart. (2017) 103:32–9. doi: 10.1136/heartjnl-2016-309665
29. Vollema EM, Sugimoto T, Shen M, Tastet L, Ng ACT, Abou R, et al. Association of left ventricular global longitudinal strain with asymptomatic severe aortic stenosis: natural course and prognostic value. JAMA Cardiol. (2018) 3:839–47. doi: 10.1001/jamacardio.2018.2288
30. Genereux P, Pibarot P, Redfors B, Mack MJ, Makkar RR, Jaber WA, et al. Staging classification of aortic stenosis based on the extent of cardiac damage. Eur Heart J. (2017) 38:3351–8. doi: 10.1093/eurheartj/ehx381
Keywords: bicuspid aortic stenosis, aortic valve area, cardiac computed tomography, echocardiography, multimodal imaging
Citation: Kim K, Lee SJ, Seo J, Suh YJ, Cho I, Hong G-R, Ha J-W, Kim YJ and Shim CY (2022) Assessment of aortic valve area on cardiac computed tomography in symptomatic bicuspid aortic stenosis: Utility and differences from Doppler echocardiography. Front. Cardiovasc. Med. 9:1035244. doi: 10.3389/fcvm.2022.1035244
Received: 02 September 2022; Accepted: 18 November 2022;
Published: 19 December 2022.
Edited by:
Ana Teresa Timoteo, Hospital de Santa Marta, PortugalReviewed by:
Yohann Bohbot, University Hospital Center (CHU) of Amiens, FranceAlberto Guido Pozzoli, Ospedale Regionale di Lugano, Switzerland
Copyright © 2022 Kim, Lee, Seo, Suh, Cho, Hong, Ha, Kim and Shim. This is an open-access article distributed under the terms of the Creative Commons Attribution License (CC BY). The use, distribution or reproduction in other forums is permitted, provided the original author(s) and the copyright owner(s) are credited and that the original publication in this journal is cited, in accordance with accepted academic practice. No use, distribution or reproduction is permitted which does not comply with these terms.
*Correspondence: Chi Young Shim, Y3lzcHJzJiN4MDAwNDA7eXVocy5hYw==; Young Jin Kim, ZHJ5aiYjeDAwMDQwO3l1aHMuYWM=