- 1Innovation Research Institute of Traditional Chinese Medicine, Shandong University of Traditional Chinese Medicine, Jinan, China
- 2Cardiovascular Surgery Department, The First Affiliated Hospital of Xi'an Jiaotong University, Xian, China
- 3Department of Vascular Surgery, Shandong Provincial Hospital Affiliated to Shandong First Medical University, Jinan, China
Vascular remodeling (VR) is a structural and functional change of blood vessels to adapt to the changes of internal and external environment. It is one of the common pathological features of many vascular proliferative diseases. The process of VR is mainly manifested in the changes of vascular wall structure and function, including intimal hyperplasia, thickening or thinning of media, fibrosis of adventitia, etc. These changes are also the pathological basis of aging and various cardiovascular diseases. Mechanical force is the basis of cardiovascular biomechanics, and the newly discovered mechanical sensitive ion channel Piezo1 is widely distributed in the whole cardiovascular system. Studies have confirmed that Piezo1, a mechanically sensitive ion channel, plays an important role in cardiovascular remodeling diseases. This article reviews the molecular mechanism of Piezo1 in atherosclerosis, hypertension and pulmonary hypertension, in order to provide a theoretical basis for the further study of vascular remodeling.
Introduction
Vascular remodeling (VR) is a frontier field in the research of various cardiovascular diseases in recent years. As a dynamic pathological process, it is the change of the structure and function of blood vessels to adapt to the changes in the internal and external environment (1–3). The functional changes are manifested as alterations in vascular compliance and derangements in vascular regulation, while the structural changes are reflected in the synthesis, degradation and reorganization of the extracellular matrix (ECM) and the excessive proliferation, migration and apoptosis of vascular endothelial cells and smooth muscle cells (2, 4–8). VR is a key pathological characteristics of the development of a variety of cardiovascular diseases, and many biological processes can lead to pathological VR, such as endothelial cell dysfunction and damage, vascular smooth muscle cell migration, proliferation, apoptosis, oxidative stress, lipid accumulation, inflammatory response and imbalance of ECM synthesis and degradation (9–13). In addition, VR is also regulated by vascular growth factors, the vasoactive substances and hemodynamics (1, 14, 15). The molecular mechanisms of action of pathological VR are not well understood, which makes the treatment of VR disorders difficult and the prognosis poor (12). Therefore, exploring the pathogenesis and treatment strategies of VR disorders has become a hot topic in basic research, and it is also an urgent medical problem to be solved in clinical work.
The mechanosensitive ion channel Piezo1 is a mechanically activated, non-selective cation channel that efficiently converts mechanical forces into electrochemical signals (16). Piezo1 has been studied in a variety of disciplines, including biology, pathology and pathophysiology, and is manifested in a variety of biological functions such as touch, proprioception, pain, vascular development and blood pressure regulation (17–20). It has been established that Piezo1 is expressed on vascular endothelial cells, blood cells, epithelial cells and cardiomyocytes, etc and plays an important regulatory role in the physiopathological processes of the body (21, 22). Piezo1 acts in the cardiovascular system mainly by sensing the fluid shear stress caused by blood flow and non-selectively mediating the entry of cations such as Na+, Ca2+ into the cells (23, 24). Piezo1-mediated Ca2+ inward flow in vascular endothelial cells can affect vascular remodeling by regulating downstream signaling pathways related to vascular tension, vascular development, lymphatic valve formation and epithelial cell homeostasis, among other effects (25–30). Therefore, the present paper investigates the effects of Piezo1 on vascular remodeling. Thus, the present paper reviews the mechanosensitive ion channel Piezo1 in cardiovascular remodeling diseases, with the aim of providing a theoretical basis for the diagnosis and treatment of cardiovascular remodeling diseases.
Piezo1 channels structure-function
Piezo1 protein, first reported in 2006, is a Ca2+ ion channel membrane protein (31). At the beginning of 2010, Patapoutian's team first screened Neuron2A cell lines to determine that they could sense mechanical stress, and then used modern molecular biology techniques such as gene silencing and membrane clamp to silence 72 candidate genes with RNA, followed by stress testing and recording of currents in the treated cells (32). Coste et al. (32) eventually identified Fam38A, the gene that mediates mechanosensitive ionic currents, and found that knocking out the Fam38A gene eliminated currents activated by mechanical forces, naming it Piezo1, a discovery that was also awarded the Nobel Prize in Physiology or Medicine in 2021.
The Piezo1 protein contains 2,547 amino acids. It is a trimeric propeller-shaped channel protein (~900 kDa), consisting of a central anchor, three long beams and three blade-like structures (33). Observed under high-resolution cryo-electron microscopy (cryo-EM), it shows a three-leafed helical structural state (33–35). It is the unique structure of Piezo1 that allows it to respond with very specific mechanosensitivity to external stimuli and to internal signals generated by the cell (36).
It was demonstrated that Piezo1 can sense and transmit a variety of mechanical forces, including cell membrane tension (37), shear stress (17, 38), cellular stretch (32), and cyclic pressure (39). Increasingly, Piezo1 has been confirmed to play an important role in the maintenance of vascular development and vascular function, particularly in the regulation of vascular endothelial cell function (40). It was found that Piezo1 is highly expressed on vascular endothelial cells, and when researchers knocked out Piezo1 on mouse endothelial cells, it was observed that blood vessels failed to form in mouse embryos, resulting in embryonic death; experiments confirmed that Piezo1 has an important role in vascular development and molding (17, 41, 42). In addition, Retailleau et al. (43) found that in arterial smooth muscle cells, Piezo1 induced small arterial vascular neovascularization by activating TG and promoting thickening of small arterial blood vessel walls.
It has also been reported that vascular endothelial cells constantly interact with the intra- and extra-luminal extracellular environment and that endothelial cells are endowed with physical stresses, such as shear stresses and tensile forces, which are sensed and transmitted by Piezo1, leading to altered cell behavior with excessive proliferation, migration and aggregation, forming primitive vascular vasculature and further leading to pathological vascular remodeling (28, 44–46). In summary, Piezo1 has a major function in vascular remodeling and may be a potential therapeutic target for vascular remodeling diseases.
Pharmacological modulators of Piezo1 channels
Piezo1 channel activator
In addition to physical-mechanical forces, Piezo1 also allows gating by chemical means. Syeda et al. (47) recently used high-throughput screening techniques to screen 3.25 million low molecular compounds one by one, eventually identifying the first chemical activator of the Piezo1 channel and naming it Yoda1, which activates Piezo1 without mechanical stimulation by acting on the intracellular region at the C-terminus of the Piezo1 channel protein (48–51). The results of the experimental research showed that the activation current of Piezo1 channels was found to be significantly reduced after depletion of extracellular Ca2+ with Ca2+ chelators, but was not altered when intracellular Ca2+ was depleted by the application of toxic carotene (Thapsigargin, A potent inhibitor of intracellular calcium transport enzymes), indicating that the Yoda1-induced Ca2+ response is likely to be dependent on the inward flow of extracellular Ca2+. In addition, it was found that the administration of certain mechanical stimuli enhanced the kinetic process of Yoda1-induced Piezo1 channels and prolonged the inactivation of transient currents (36, 49, 52, 53).
Jedi1/2 is a new recently discovered hydrophilic Piezo1 channel chemical activator that acts by acting on a distal paddle at the cell periphery, using a peripheral lever-like device consisting of a blade and a light beam to control the central Piezo1 ion conductance pore (54, 55). However, the specific mechanisms by which these chemicals activate Piezo1 channels remain elusive, and their role in cardiovascular remodeling needs to be further investigated.
Piezo1 channel non-specific inhibitor
Only a few drugs have been used in pharmacological studies of Piezo1 channel Non-specific inhibitors, broadly classified as Gd3+, Dooku1, and La3+ in the lanthanide family, gentamicin and streptomycin in the aminoglycoside group, ruthenium red (RR) and the spider venom peptide GsMTx-4 isolated from tarantula toxin (16, 32, 56–60). GsMTx-4 is a cation channel-specific blocker that not only blocks Piezo1 ion channels but also has the effect of inhibiting Piezo1-induced mechanically activated (MA) current generation (61–64). Mechanistically, GsMTx4 binds to the cell membrane and, by inserting its hydrophobic domain into the lipid bilayer, acts directly on the backbone proteins of the membrane, blocking mechanical force transmission by altering the tension in the membrane and thus achieving a blockade of the Piezo1 ion channel (62, 65, 66). It has been confirmed that two drugs, such as Phosphatidic acid (PA) and lysophosphatidylcholine (LPC), also exert a blocking effect on the mechanical ion channel Piezo1 via the above pathway (20, 66–69).
Previous research in the author's laboratory has revealed that the effective extract of the Chinese medicine tubeimoside I (TBMS1) antagonizes the activation of Piezo1 channels by Yoda1 through competing with Yoda1 for the target of action, thereby inhibiting the Yoda1-induced aortic diastole. Meanwhile, it was also found that although TBMS1 did not significantly inhibit phenylephrine-induced aortic ring contraction, it significantly reduced Yoda1-induced vasodilation, suggesting that TBMS1 directly inhibits Piezo1 channel activity or functions through other unknown mechanisms, thus speculating that TBMS1 may act on Piezo1 in vascular smooth muscle cells to partially inhibit vasoconstriction and thus slow down the onset of vascular remodeling (50). The above findings also confirm that herbal compounds can act through Piezo1 ion channels for the treatment of vascular remodeling diseases, which may also provide new targets for the development of disease-targeting drugs (Table 1).
The role of Piezo1 in atherosclerotic vascular remodeling
Atherosclerosis (AS) is the most common type of atherosclerosis, which is characterized by the accumulation of lipids and necrotic tissue in the intima of arteries, forming yellow atheromatous plaques (61, 70). AS is a chronic inflammatory disease and the pathological basis of many cardiovascular diseases. As the disease progresses, the lumen gradually becomes thickened, stiffened, and less elastic until it becomes occluded, eventually leading to myocardial infarction, stroke and other diseases (71–73).
It is well known that inflammation is a key factor driving the development and progression of AS, and that disturbed blood flow shear stress can exacerbate the inflammatory response by damaging vascular endothelial cells (28, 74–76). Albarran-Juarez et al. (77) demonstrated that Piezo1 activates P2Y2 receptors and Gq/G11 protein-mediated integrins after sensing vascular perturbations, which in turn activates adhesion kinase-dependent activation of the pro-inflammatory star factor NF-κB, thereby exacerbating the development of atherosclerosis. It was also found that when endothelial-specific Piezo1 or Gq/G11-deficient mice were induced, activation of integrins, activation of inflammatory signaling pathways was found to be reduced, and the area of atherosclerosis and the extent of atherosclerosis were both reduced to some extent. Further studies revealed that under high shear stress laminar flow, Piezo1-mediated vascular remodeling-related signaling pathways P2Y2 and Gq/G11 were inhibited, but activated downstream eNOS signaling pathways, which were instead protective against atherosclerotic disease (77–80). Experiments have shown that Piezo1 plays a regulatory role in the development of atherosclerotic pathology by mediating different downstream signaling pathways through sensing different blood flow patterns such as laminar and perturbed flow.
Proliferation, apoptosis and migration of vascular smooth muscle cells are important pathological changes in diseases such as atherosclerosis and restenosis and are major pathological features of arterial reconstruction due to mechanical stress (81, 82). Piezo1, as a mechanosensitive ion channel protein, is essential for the sensing and transduction of mechanical forces such as shear stress and tensile force (83, 84). Randolph et al. (41, 85, 86) showed that Piezo1 mediates cell proliferation-related factors such as matrix metalloproteinase-2 (MMP-2), matrix metalloproteinase-9 (MMP-9), and Platelet derived growth factor (PDGF) expression directly mediates the proliferation of vascular smooth muscle cells. In addition, the application of a 15% mechanical stretching force to mouse aortic smooth muscle cells in cell culture using the Flexcell cellular fluid shear stress system revealed that the expression of proteins and genes related to the Akt signaling pathway, which regulates cell proliferation, migration and anti-apoptosis, was upregulated, leading to sustained smooth muscle cell proliferation and exacerbating the progression of atherosclerotic disease (87–91). Meanwhile Jufri et al. (92) suggested that physiological mechanical stretch helps maintain vascular health and that pathological stretch leads to further disease development. When endothelial cells were stretched by pathological mechanical forces, elevated expression of specific vascular smooth muscle cell marker genes (SM22, SMA, Caldesmon-1, SM MHC and Calponin) and decreased expression of endothelial markers were observed, suggesting that endothelial cells are gradually damaged after a certain mechanical stretch force and smooth muscle cells proliferate abnormally, which is also driving plaque This is one of the important reasons for the progression of plaque (93–95).
This suggests that inhibition of Piezo1 overexpression may have a therapeutic effect on atherosclerotic disease. Recent studies in the author's laboratory have found that silencing the expression of Piezo1 in human umbilical vein endothelial cells (HUVEC) and murine liver endothelial cells (MLEC) has a protective effect against atherosclerosis. It was also demonstrated that the Chinese herbal medicine monomer salvianolic acid B could inhibit Ca2+ influx following Piezo1 activation by Yoda1, which inhibited pathological aortic luminal hypoelasticity and thus slowed down the formation of atherosclerotic plaques (96).
The role of Piezo1 in vascular remodeling in pulmonary hypertension
Pulmonary arterial hypertension (PAH) is a complex, progressive cardiopulmonary disease characterized by non-specific symptoms such as dyspnoea, fatigue, weakness, angina, and syncope. The main features of PAH are increased pulmonary vascular resistance and elevated pulmonary arterial pressure, ultimately leading to right heart failure and death (97–99). It has been shown that the main factors contributing to increased pulmonary vascular resistance during the development of PAH are abnormal systolic function of the small pulmonary arteries and remodeling of the pulmonary arteries (100–102). An increase in cytoplasmic free Ca2+ concentration ([Ca2+] cyt) in pulmonary arterial smooth muscle cells (PASMCs) is the main cause of pulmonary vasoconstriction, while an imbalance in intracellular calcium (Ca2+) homeostasis stimulates PASMCs to proliferate and inhibits their apoptosis, leading to pulmonary vascular remodeling. The imbalance in intracellular calcium (Ca2+) homeostasis stimulates PASMCs to proliferate and inhibits their apoptosis, leading to pulmonary vascular remodeling and driving PAH disease progression (53, 101–105). Under physiological conditions, pulmonary endothelial cells Piezo1 regulate endothelium-dependent pulmonary vasodilation by mediating Ca2+ inward flow (83, 105–107). It has been hypothesized that upregulation of Piezo1 expression in pulmonary arterial endothelial cells (PAECs) and pulmonary artery smooth muscle cells may play a key role in vascular remodeling in PAH disease. Piezo1 high expression induced Ca2+ influx in PAECs, and upregulated Notch ligands (JAG-1, and DLL4) in PAECs. The increased Notch ligands in PAECs, as signal-sending cells, then activate Notch receptors in PASMCs, as signal-receiving cells, and result in pulmonary arteriole muscularization and concentric pulmonary vascular remodeling (105). This suggests that Piezo1 plays a key role in regulating endothelial cell Ca2+ homeostasis and is important for pulmonary artery vascular remodeling.
Pulmonary vasodilation is closely related to the regulatory role of PAECs, but the biological mechanism of Piezo1 in PASMCs is unclear. It has been proposed that alterations in hemodynamics cause endothelial damage in the pulmonary vasculature to some extent and also play a regulatory role in the phenotypic transformation and morphological structure of PASMCs, and that hemodynamics are also necessary for the development and maintenance of vascular remodeling in pulmonary hypertension (105, 108, 109). It has been hypothesized that one of the important factors contributing to pulmonary artery remodeling is the increase in intracellular free calcium concentration ([Ca2+] i) caused by mechanical stimulation of PASMCs (93, 94). Experimental studies have shown that under pulmonary hypertension, elevated [Ca2+] i in PASMCs occurs, which also upregulates the expression of genes activating Ca2+-sensitive transcription factors such as STIM2, TRPC6 and Orai2, thereby differentiating the phenotype of PASMCs from a contractile/resting phenotype to a proliferative/synthetic phenotype, leading to vascular remodeling (94, 110, 111). In addition, it has been proposed that Piezo1 activates Ca2+-dependent signal transduction pathways such as YAP and other related transcription factors following an increase in intracellular cytoplasmic free calcium concentration, resulting in increased mechanosensitivity of the pulmonary vasculature to regulate cell proliferation and pulmonary vascular remodeling, and that aberrant mechanical stimulation of Piezo1 in PASMCs exacerbates blood flow disturbances, a process that circumferentially also promotes progression of pulmonary hypertension (53).
Fernandez et al. (94) found that Piezo1-mediated increases in [Ca2+] i were associated with contraction and abnormal proliferation of PASMCs. A dose-dependent relationship with vasoconstriction was found after stimulation of endothelium-denuded rat intrapulmonary arteries by applying Yoda1, and Piezo1 activity was significantly upregulated and increased in idiopathic PAH-PASMCs compared with donor PASMCs, which also explains the effect of proliferation caused by elevated Piezo1-mediated [Ca2+] i in PASMCs under PAH on pulmonary artery of the molecular mechanisms of vascular remodeling. In conclusion, these studies further elucidate the role of Piezo1 in vascular remodeling in pulmonary arterial hypertension, which has the potential to be an effective intervention molecule and potential target of action in the treatment of pulmonary hypertension in the future (Figure 1).
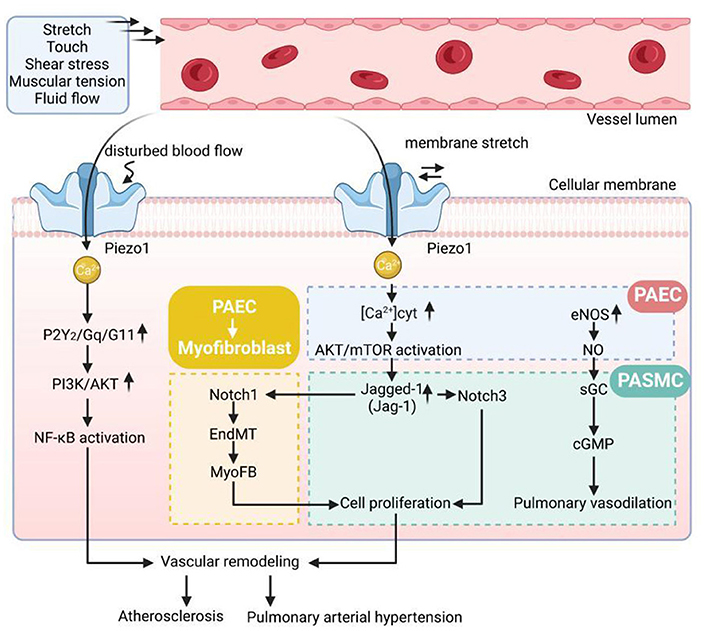
Figure 1. The pathological mechanisms of vascular remodeling disease. Gq/G11, Gprotein alpha-subunits Galphaq/Galpha11; AKT, protein kinase B; PI3K, phosphatidylinositol 3 kinase; NF-κB, nuclear factor kappa B; EndMT, endothelial-to-mesenchymal transition; [Ca2+]cyt, cytoplasmic free Ca2+ concentration; eNOS, endothelial nitric oxide synthase; NO, endothelial nitric oxide; sGC, soluble guanylate cyclase; cGMP, cyclic guanosine monophosphate.
Conclusions
Cardiopulmonary diseases, e.g., hypertension, atherosclerosis, pulmonary hypertension, asthma and chronic obstructive pulmonary disease, are among the most common causes of death worldwide. A common pathophysiological theme to these diseases is vascular remodeling, which is contributed by changes in expression and activation of piezo1 ion channels critical for either excitability or growth. In recent years, many scholars have made remarkable achievements in this field, and we have initially understood that it plays an important role in coupling various signaling pathways to promote vascular development and maintain vascular homeostasis by sensing blood flow shear stress and non-selectively mediating the flow of cations such as Na+ and Ca2+.
However, our investigation of the role of Piezo1 in vascular remodeling is still in its infancy, and further studies are needed to clarify whether Piezo1 and other mechanosensitive ion channels and membrane proteins act together, and the regulation of upstream and downstream specific signaling pathways when Piezo1 channels act. We believe that with further research on the mechanosensitive ion channel Piezo1, Piezo1 is likely to become a new target for the diagnosis and treatment of vascular remodeling diseases such as hypertension, atherosclerosis and pulmonary hypertension, opening up new ideas for the clinical treatment of vascular remodeling diseases.
Author contributions
HX, YH, TH, CB, MX, and JL contributed to conception and design of the study. HX and YH wrote the first draft of the manuscript. HX, TH, CB, MX, and JL wrote sections of the manuscript. All authors contributed to manuscript revision, read, and approved the submitted version.
Funding
This research was supported by the grant from National Natural Science Foundation of China (82174196 and 81770453), Major basic research projects of Natural Science Foundation of Shandong Province (ZR2020ZD16), Youth Natural Science Foundation of Shandong Province (ZR2020QH340), and Traditional Chinese Medicine Development Science and Technology Foundation of Shandong Province (2019-0051).
Conflict of interest
The authors declare that the research was conducted in the absence of any commercial or financial relationships that could be construed as a potential conflict of interest.
Publisher's note
All claims expressed in this article are solely those of the authors and do not necessarily represent those of their affiliated organizations, or those of the publisher, the editors and the reviewers. Any product that may be evaluated in this article, or claim that may be made by its manufacturer, is not guaranteed or endorsed by the publisher.
References
1. Ouarne M, Pena A, Franco AC. From remodeling to quiescence: the transformation of the vascular network. Cells Dev. (2021) 168:203735. doi: 10.1016/j.cdev.2021.203735
2. Qi YX, Han Y, Jiang LZ. Mechanobiology and vascular remodeling: from membrane to nucleus. Adv Exp Med Biol. (2018) 1097:69–82. doi: 10.1007/978-3-319-96445-4_4
3. Mendez-Barbero N, Gutierrez-Munoz C, Blanco-Colio ML. Cellular crosstalk between endothelial and smooth muscle cells in vascular wall remodeling. Int J Mol Sci. (2021) 22:7284. doi: 10.3390/ijms22147284
4. Shi N, Mei XH, Chen YS. Smooth muscle cells in vascular remodeling. Arterioscler Thromb Vasc Biol. (2019) 39:E247–52. doi: 10.1161/ATVBAHA.119.312581
5. Qi Y, Dai F, Gu J, Yao W. Biomarkers in VSMC phenotypic modulation and vascular remodeling. Pharmazie. (2019) 74:711–4. doi: 10.1691/ph.2019.9743
6. Liu S, Lin Z. Vascular smooth muscle cells mechanosensitive regulators and vascular remodeling. J Vasc Res. (2022) 59:90–113. doi: 10.1159/000519845
7. Lin TY, Yeh JL H, Hsu J. Role of extracellular matrix in pathophysiology of patent ductus arteriosus: emphasis on vascular remodeling. Int J Mol Sci. (2020) 21:4761. doi: 10.3390/ijms21134761
8. Ma Z, Mao C, Jia Y, Fu Y, Kong W. Extracellular matrix dynamics in vascular remodeling. Am J Physiol Cell Physiol. (2020) 319:C481–99. doi: 10.1152/ajpcell.00147.2020
9. Cai Z, Gong Z, Li Z, Li L, Kong W. Vascular extracellular matrix remodeling and hypertension. Antioxid Redox Signal. (2021) 34:765–83. doi: 10.1089/ars.2020.8110
10. Sorokin V, Vickneson K, Kofidis T, Woo CC, Lin XY, Foo R, et al. Role of vascular smooth muscle cell plasticity and interactions in vessel wall inflammation. Front Immunol. (2020) 11:599415. doi: 10.3389/fimmu.2020.599415
11. Wang DD, Uhrin P, Mocan A, Waltenberger B, Breuss JM, Tewari D, et al. Vascular smooth muscle cell proliferation as a therapeutic target. Part 1: molecular targets and pathways. Biotechnol Adv. (2018) 36:1586–607. doi: 10.1016/j.biotechadv.2018.04.006
12. Zhu GX, Zuo JL, Xu L, Li QS. Ginsenosides in vascular remodeling: cellular and molecular mechanisms of their therapeutic action. Pharmacol Res. (2021) 169:105647. doi: 10.1016/j.phrs.2021.105647
13. Zhuang T, Liu J, Chen X, Pi J, Kuang Y, Wang Y, et al. Cell-specific effects of GATA (GATA zinc finger transcription factor family)-6 in vascular smooth muscle and endothelial cells on vascular injury neointimal formation. Arterioscler Thromb Vasc Biol. (2019) 39:888–901. doi: 10.1161/ATVBAHA.118.312263
14. Yamamura A, Nayeem MJ, Sato M. [Roles of growth factors on vascular remodeling in pulmonary hypertension]. Nihon Yakurigaku Zasshi. (2021) 156:161–5. doi: 10.1254/fpj.21006
15. Liu J, Kang H, Ma X, Sun A, Luan H, Deng X, et al. Vascular cell glycocalyx-mediated vascular remodeling induced by hemodynamic environmental alteration. Hypertension. (2018) 71:1201–9. doi: 10.1161/HYPERTENSIONAHA.117.10678
16. Douguet D, Patel A, Xu A, Vanhoutte PM, Honore E. Piezo ion channels in cardiovascular mechanobiology. Trends Pharmacol Sci. (2019) 40:956–70. doi: 10.1016/j.tips.2019.10.002
17. Li J, Hou B, Tumova S, Muraki K, Bruns A, Ludlow MJ, et al. Piezo1 integration of vascular architecture with physiological force. Nature. (2014) 515:279–82. doi: 10.1038/nature13701
18. Marinelli S, Basilico B, Marrone MC, Ragozzino D. Microglia-neuron crosstalk: Signaling mechanism and control of synaptic transmission. Semin Cell Dev Biol. (2019) 94:138–51. doi: 10.1016/j.semcdb.2019.05.017
19. Qin L, He T, Chen S, Yang D, Yi W, Cao H, et al. Roles of mechanosensitive channel Piezo1/2 proteins in skeleton and other tissues. Bone Res. (2021) 9:44. doi: 10.1038/s41413-021-00168-8
20. Ridone P, Vassalli M, Martinac B. Piezo1 mechanosensitive channels: what are they and why are they important. Biophys Rev. (2019) 11:795–805. doi: 10.1007/s12551-019-00584-5
21. Emig R, Knodt W, Krussig MJ, Zgierski-Johnston CM, Gorka O, Gross O, et al. Piezo1 channels contribute to the regulation of human atrial fibroblast mechanical properties and matrix stiffness sensing. Cells. (2021) 10:663. doi: 10.3390/cells10030663
22. Jiang F, Yin K, Wu K, Zhang M, Wang S, Cheng H, et al. The mechanosensitive Piezo1 channel mediates heart mechano-chemo transduction. Nat Commun. (2021) 12:869. doi: 10.1038/s41467-021-21178-4
23. Fang XZ, Zhou T, Xu JQ, Wang YX, Sun MM, He YJ, et al. Structure, kinetic properties and biological function of mechanosensitive Piezo channels. Cell Biosci. (2021) 11:13. doi: 10.1186/s13578-020-00522-z
24. Swain SM, Liddle RA. Piezo1 acts upstream of TRPV4 to induce pathological changes in endothelial cells due to shear stress. J Biol Chem. (2021) 296:100171. doi: 10.1074/jbc.RA120.015059
25. Beech DJ. Endothelial Piezo1 channels as sensors of exercise. J Physiol-London. (2018) 596:979–84. doi: 10.1113/JP274396
26. Faucherre Maati HMO, Nasr N, Pinard A, Theron A, Odelin G, Desvignes JP, et al. Piezo1 is required for outflow tract and aortic valve development. J Mol Cell Cardiol. (2020) 143:51–62. doi: 10.1016/j.yjmcc.2020.03.013
27. Friedrich EE, Hong Z, Xiong S, Zhong M, Di A, Rehman J, et al. Endothelial cell Piezo1 mediates pressure-induced lung vascular hyperpermeability via disruption of adherens junctions. Proc Natl Acad Sci U S A. (2019) 116:12980–5. doi: 10.1073/pnas.1902165116
28. Lai Cox CD, Sekar NC, Thurgood P, Jaworowski A, Peter K, Baratchi S. Mechanosensing by Piezo1 and its implications for physiology and various pathologies. Biological Rev. (2022) 97:604–14. doi: 10.1111/brv.12814
29. Nonomura K, Lukacs V, Sweet DT, Goddard LM, Kanie A, Whitwam T, et al. Mechanically activated ion channel PIEZO1 is required for lymphatic valve formation. Proc Natl Acad Sci U S. (2018) 115:12817–22. doi: 10.1073/pnas.1817070115
30. Zhang Y, Su SA Li W, Ma Y, Shen J, Wang Y, et al. Piezo1-mediated mechanotransduction promotes cardiac hypertrophy by impairing calcium homeostasis to activate calpain/calcineurin signaling. Hypertension. (2021) 78:647–60. doi: 10.1161/HYPERTENSIONAHA.121.17177
31. Dhaka A, Viswanath V, Patapoutian A. Trp ion channels and temperature sensation. Annu Rev Neurosci. (2006) 29:135–61. doi: 10.1146/annurev.neuro.29.051605.112958
32. Coste Mathur J, Schmidt M, Earley TJ, Ranade S, Petrus MJ, Dubin AE, et al. Piezo1 and Piezo2 are essential components of distinct mechanically activated cation channels. Science. (2010) 330:55–60. doi: 10.1126/science.1193270
33. Zhao Q, Zhou H, Li X, Xiao B. The mechanosensitive Piezo1 channel: a three-bladed propeller-like structure and a lever-like mechanogating mechanism. FEBS J. (2019) 286:2461–70. doi: 10.1111/febs.14711
34. Zhao Q, Zhou H, Chi S, Wang Y, Wang J, Geng J, et al. Structure and mechanogating mechanism of the Piezo1 channel. Nature. (2018) 554:487–92. doi: 10.1038/nature25743
35. Zhao X, Kong Y, Liang B, Xu J, Lin Y, Zhou N, et al. Mechanosensitive Piezo1 channels mediate renal fibrosis. JCI Insight. (2022) 7:e152330. doi: 10.1172/jci.insight.152330
36. Lin YC, Guo YR, Miyagi A, Levring J, MacKinnon R, Scheuring S. Force-induced conformational changes in PIEZO1. Nature. (2019) 573:230–4. doi: 10.1038/s41586-019-1499-2
37. De Vecchis D, Beech DJ, Kalli AC. Molecular dynamics simulations of Piezo1 channel opening by increases in membrane tension. Biophys J. (2021) 120:1510–1521. doi: 10.1016/j.bpj.2021.02.006
38. Jiang Y, Yang X, Jiang J, Xiao B. Structural designs and mechanogating mechanisms of the mechanosensitive piezo channels. Trends Biochem Sci. (2021) 46:472–88. doi: 10.1016/j.tibs.2021.01.008
39. Solis G, Bielecki P, Steach HR, Sharma L, Harman CCD, Yun S, et al. Mechanosensation of cyclical force by PIEZO1 is essential for innate immunity. Nature. (2019) 573:69–74. doi: 10.1038/s41586-019-1485-8
40. Siragusa M, Oliveira Justo AF, Malacarne PF, Strano A, Buch A, Withers B, et al. VE-PTP inhibition elicits eNOS phosphorylation to blunt endothelial dysfunction and hypertension in diabetes. Cardiovasc Res. (2021) 117:1546–56. doi: 10.1093/cvr/cvaa213
41. Kang H, Hong Z, Zhong M, Klomp J, Bayless KJ, Mehta D, et al. Piezo1 mediates angiogenesis through activation of MT1-MMP signaling. Am J Physiol Cell Physiol. (2019) 316:C92–C103. doi: 10.1152/ajpcell.00346.2018
42. Liu TT, Du XF, Zhang BB, Zi HX, Yan Y, Yin JA, et al. Piezo1-Mediated Ca(2+) activities regulate brain vascular pathfinding during development. Neuron. (2020) 108:180–92.e5. doi: 10.1016/j.neuron.2020.07.025
43. Retailleau K, Duprat F, Arhatte M, Ranade SS, Peyronnet R, Martins JR, et al. Piezo1 in Smooth Muscle Cells Is Involved in Hypertension-Dependent Arterial Remodeling. Cell Rep. (2015) 13:1161–71. doi: 10.1016/j.celrep.2015.09.072
44. Chen P, Zhang G, Jiang S, Ning Y, Deng B, Pan X, et al. Mechanosensitive piezo1 in endothelial cells promotes angiogenesis to support bone fracture repair. Cell Calcium. (2021) 97:102431. doi: 10.1016/j.ceca.2021.102431
45. Gordon E, Schimmel L, Frye M. The importance of mechanical forces for in vitro endothelial cell biology. Front Physiol. (2020) 11:684. doi: 10.3389/fphys.2020.00684
46. Liu HL, Hu JL, Zheng QC, Feng XJ, Zhan FF, Wang XF, et al. Piezo1 channels as force sensors in mechanical force-related chronic inflammation. Front Immunol. (2022) 13:816149. doi: 10.3389/fimmu.2022.816149
47. Syeda R, Xu J, Dubin AE, Coste B, Mathur J, Huynh T, et al. Chemical activation of the mechanotransduction channel Piezo1. Elife. (2015) 4:e07369. doi: 10.7554/eLife.07369
48. Botello-Smith WM, Jiang W, Zhang H, Ozkan AD, Lin YC, Pham CN, et al. A mechanism for the activation of the mechanosensitive piezo1 channel by the small molecule yoda1. Nat Commun. (2019) 10:4503. doi: 10.1038/s41467-019-12501-1
49. Lacroix JJ, Botello-Smith WM, Luo Y. Probing the gating mechanism of the mechanosensitive channel piezo1 with the small molecule yoda1. Nat Commun. (2018) 9:2029. doi: 10.1038/s41467-018-04405-3
50. Liu S, Pan X, Cheng W, Deng B, He Y, Zhang L, et al. Tubeimoside I antagonizes yoda1-evoked piezo1 channel activation. Front Pharmacol. (2020) 11:768. doi: 10.3389/fphar.2020.00768
51. Bosutti A, Giniatullin A, Odnoshivkina Y, Giudice L, Malm T, Sciancalepore M, et al. Bernareggi: “time window” effect of yoda1-evoked piezo1 channel activity during mouse skeletal muscle differentiation. Acta Physiologica. (2021) 233:e13702. doi: 10.1111/apha.13702
52. Chong J, De Vecchis D, Hyman AJ, Povstyan OV, Ludlow MJ, Shi J, et al. Modeling of full-length piezo1 suggests importance of the proximal N-terminus for dome structure. Biophys J. (2021) 120:1343–56. doi: 10.1016/j.bpj.2021.02.003
53. Liao J, Lu W, Chen Y, Duan X, Zhang C, Luo X, et al. Upregulation of piezo1 (piezo type mechanosensitive ion channel component 1) enhances the intracellular free Calcium in pulmonary arterial smooth muscle cells from idiopathic pulmonary arterial hypertension patients. Hypertension. (2021) 77:1974–89. doi: 10.1161/HYPERTENSIONAHA.120.16629
54. Wang Y, Chi S, Guo H, Li G, Wang L, Zhao Q, et al. A lever-like transduction pathway for long-distance chemical- and mechano-gating of the mechanosensitive piezo1 channel. Nat Commun. (2018) 9:1300. doi: 10.1038/s41467-018-03570-9
55. Xiao B. Levering mechanically activated piezo channels for potential pharmacological intervention. Annu Rev Pharmacol Toxicol. (2020) 60:195–218. doi: 10.1146/annurev-pharmtox-010919-023703
56. Neuberger Nadezhdin KD, Sobolevsky AI. Structural mechanisms of TRPV6 inhibition by ruthenium red and econazole. Nat Commun. (2021) 12:6284. doi: 10.1038/s41467-021-26608-x
57. Coste Xiao B, Santos JS, Syeda R, Grandl J, Spencer KS, Kim SE, et al. Piezo proteins are pore-forming subunits of mechanically activated channels. Nature. (2012) 483:176–81. doi: 10.1038/nature10812
58. De Felice D, Alaimo A. Mechanosensitive piezo channels in cancer: focus on altered calcium signaling in cancer cells and in tumor progression. Cancers. (2020) 12:1780. doi: 10.3390/cancers12071780
59. Blythe NM, Muraki K, Ludlow MJ, Stylianidis V, Gilbert HTJ, Evans EL, et al. Mechanically activated Piezo1 channels of cardiac fibroblasts stimulate p38 mitogen-activated protein kinase activity and interleukin-6 secretion. J Biol Chem. (2019) 294:17395–408. doi: 10.1074/jbc.RA119.009167
60. Evans EL, Cuthbertson K, Endesh N, Rode B, Blythe NM, Hyman AJ, et al. Yoda1 analogue (dooku1) which antagonizes yoda1-evoked activation of piezo1 and aortic relaxation. Br J Pharmacol. (2018) 175:1744–59. doi: 10.1111/bph.14188
61. Hao L, Li L, Wang P, Wang Z, Shi X, Guo M, et al. Synergistic osteogenesis promoted by magnetically actuated nano-mechanical stimuli. Nanoscale. (2019) 11:23423–37. doi: 10.1039/C9NR07170A
62. Gnanasambandam R, Ghatak C, Yasmann A, Nishizawa K, Sachs F, Ladokhin AS, et al. GsMTx4: mechanism of inhibiting mechanosensitive ion channels. Biophys J. (2017) 112:31–45. doi: 10.1016/j.bpj.2016.11.013
63. Grotle K, Huo Y, Harrison ML, Ybarbo KM, Stone AJ. GsMTx-4 normalizes the exercise pressor reflex evoked by intermittent muscle contraction in early stage type 1 diabetic rats. Am J Physiol Heart Circ. (2021) 320:H1738–48. doi: 10.1152/ajpheart.00794.2020
64. Jung HJ, Kim PI, Lee SK, Lee CW, Eu YJ, Lee DG, et al. Lipid membrane interaction and antimicrobial activity of GsMTx-4, an inhibitor of mechanosensitive channel. Biochem Biophys Res Commun. (2006) 340:633–8. doi: 10.1016/j.bbrc.2005.12.046
65. Cox CD, Gottlieb PA. Amphipathic molecules modulate PIEZO1 activity. Biochem Soc Trans. (2019) 47:1833–42. doi: 10.1042/BST20190372
66. Yang X, Lin C, Chen X, Li S, Li X, Xiao B. Structure deformation and curvature sensing of PIEZO1 in lipid membranes. Nature. (2022) 604:377–83. doi: 10.1038/s41586-022-04574-8
67. Buyan Cox CD, Barnoud J, Li J, Chan HSM, Martinac B, Marrink SJ, et al. piezo1 forms specific, functionally important interactions with phosphoinositides and cholesterol. Biophys J. (2020) 119:1683–97. doi: 10.1016/j.bpj.2020.07.043
68. Romero LO, Massey AE, Mata-Daboin AD, Sierra-Valdez FJ, Chauhan SC, Cordero-Morales JF, et al. Dietary fatty acids fine-tune Piezo1 mechanical response. Nat Commun. (2019) 10:1200. doi: 10.1038/s41467-019-09055-7
69. Ridone P, Pandzic E, Vassalli M, Cox CD, Macmillan A, Gottlieb PA, et al. Disruption of membrane cholesterol organization impairs the activity of PIEZO1 channel clusters. J Gen Physiol. (2020) 152:e201912515. doi: 10.1085/jgp.201912515
70. Pedro-Botet J, Climent E, Benaiges D. Atherosclerosis and inflammation. New therapeutic approaches. Med Clin. (2020) 155:256–62. doi: 10.1016/j.medcle.2020.04.020
71. Tabares-Guevara JH, Villa-Pulgarin JA, Hernandez JC. Atherosclerosis: immunopathogenesis and strategies for immunotherapy. Immunotherapy. (2021) 13:1231–44. doi: 10.2217/imt-2021-0009
72. Fan J, Watanabe T. Atherosclerosis: known and unknown. Pathol Int. (2022) 72:151–60. doi: 10.1111/pin.13202
73. Poznyak V, Bharadwaj D, Prasad G, Grechko AV, Sazonova MA, Orekhov AN. Renin-Angiotensin system in pathogenesis of atherosclerosis and treatment of CVD. Int J Mol Sci. (2021) 22:6702. doi: 10.3390/ijms22136702
74. Santovito D, Weber C. Atherosclerosis revisited from a clinical perspective: still an inflammatory disease? Thromb Haemost. (2017) 117:231–7. doi: 10.1160/TH16-10-0770
75. von Vietinghoff S, Koltsova EK. Inflammation in atherosclerosis: a key role for cytokines. Cytokine. (2019) 122:154819. doi: 10.1016/j.cyto.2019.154819
76. Yang Y, Wang D, Zhang C, Yang W, Li C, Gao Z, et al. Piezo1 mediates endothelial atherogenic inflammatory responses via regulation of YAP/TAZ activation. Hum Cell. (2022) 35:51–62. doi: 10.1007/s13577-021-00600-5
77. Albarran-Juarez J, Iring A, Wang S, Joseph S, Grimm M, Strilic B, et al. Piezo1 and Gq/G11 promote endothelial inflammation depending on flow pattern and integrin activation. J Exp Med. (2018) 215:2655–72. doi: 10.1084/jem.20180483
78. Wang S, Chennupati R, Kaur H, Iring A, Wettschureck N, Offermanns S. Endothelial cation channel piezo1 controls blood pressure by mediating flow-induced ATP release. J Clin Invest. (2016) 126:4527–36. doi: 10.1172/JCI87343
79. Demos C, Williams D, Jo H. Disturbed flow induces atherosclerosis by annexin a2-mediated integrin activation. Circ Res. (2020) 127:1091–3. doi: 10.1161/CIRCRESAHA.120.317909
80. Wang S, Iring A, Strilic B, Albarran Juarez J, Kaur H, Troidl K, et al. P2Y(2) and Gq/G(1)(1) control blood pressure by mediating endothelial mechanotransduction. J Clin Invest. (2015) 125:3077–86. doi: 10.1172/JCI81067
81. Weber DS. A DEAD-Box stop of vascular remodeling. Circ Res. (2019) 124:1405–7. doi: 10.1161/CIRCRESAHA.119.315097
82. Liu M, Gomez D. Smooth muscle cell phenotypic diversity. Arterioscler Thromb Vasc Biol. (2019) 39:1715–23. doi: 10.1161/ATVBAHA.119.312131
83. Lhomme Gilbert G, Pele T, Deweirdt J, Henrion D, Baudrimont I, Campagnac M, et al. Stretch-activated piezo1 channel in endothelial cells relaxes mouse intrapulmonary arteries. Am J Respir Cell Mol Biol. (2019) 60:650–8. doi: 10.1165/rcmb.2018-0197OC
84. Shinge SAU, Zhang D, Achu Muluh T, Nie Y, Yu F. Mechanosensitive piezo1 channel evoked-mechanical signals in atherosclerosis. J Inflamm Res. (2021) 14:3621–36. doi: 10.2147/JIR.S319789
85. Randolph GJ. Proliferating macrophages prevail in atherosclerosis. Nat Med. (2013) 19:1094–5. doi: 10.1038/nm.3316
86. Randolph GJ. Mechanisms that regulate macrophage burden in atherosclerosis. Circ Res. (2014) 114:1757–71. doi: 10.1161/CIRCRESAHA.114.301174
87. Mantella LE, Quan A, Verma S. Variability in vascular smooth muscle cell stretch-induced responses in 2D culture. Vasc Cell. (2015) 7:7. doi: 10.1186/s13221-015-0032-0
88. Qiu J, Zheng Y, Hu J, Liao D, Gregersen H, Deng X, et al. Biomechanical regulation of vascular smooth muscle cell functions: from in vitro to in vivo understanding. J R Soc Interface. (2014) 11:20130852. doi: 10.1098/rsif.2013.0852
89. Liu G, Hitomi H, Hosomi N, Lei B, Nakano D, Deguchi K, et al. Mechanical stretch augments insulin-induced vascular smooth muscle cell proliferation by insulin-like growth factor-1 receptor. Exp Cell Res. (2011) 317:2420–8. doi: 10.1016/j.yexcr.2011.07.016
90. Zheng Q, Zou Y, Teng P, Chen Z, Wu Y, Dai X, et al. Mechanosensitive channel piezo1 senses shear force to induce KLF2/4 expression via CaMKII/MEKK3/ERK5 axis in endothelial cells. Cells. (2022) 11:2191. doi: 10.3390/cells11142191
91. Porto Ribeiro T, Barbeau S, Baudrimont I, Vacher P, Freund-Michel V, Cardouat G, et al. Piezo1 channel activation reverses pulmonary artery vasoconstriction in an early rat model of pulmonary hypertension: the role of Ca(2+) influx and Akt-eNOS pathway. Cells. (2022) 11:2349. doi: 10.3390/cells11152349
92. Jufri NF, Mohamedali A, Avolio A, Baker MS. Mechanical stretch: physiological and pathological implications for human vascular endothelial cells. Vasc Cell. (2015) 7:8. doi: 10.1186/s13221-015-0033-z
93. Lambert M, Capuano V, Olschewski A, Sabourin J, Nagaraj C, Girerd B, et al. Ion channels in pulmonary hypertension: a therapeutic interest? Int J Mol Sci. (2018) 19:3162. doi: 10.3390/ijms19103162
94. Fernandez RA, Wan J, Song S, Smith KA, Gu Y, Tauseef M, et al. Upregulated expression of STIM2, TRPC6, and Orai2 contributes to the transition of pulmonary arterial smooth muscle cells from a contractile to proliferative phenotype. Am J Physiol Cell Physiol. (2015) 308:C581–93. doi: 10.1152/ajpcell.00202.2014
95. Zhang N, Dou YQ, Han M. [Roles of smooth muscle 22alpha in vascular homeostasis and vascular remodeling]. Sheng Li Xue Bao. (2021) 73:82–8.
96. Pan XM, Wan RT, Wang YM, Liu SL, He Y, Deng B, et al. Inhibition of chemically and mechanically activated piezo1 channels as a mechanism for ameliorating atherosclerosis with salvianolic acid B. Br J Pharmacol. (2022) 179:3778–814. doi: 10.1111/bph.15826
97. Hassoun PM. Pulmonary arterial hypertension. N Engl J Med. (2021) 385:2361–76. doi: 10.1056/NEJMra2000348
98. Luna-Lopez R, Ruiz Martin A, Escribano Subias P. Pulmonary arterial hypertension. Med Clin. (2022) 158:622–9. doi: 10.1016/j.medcle.2022.05.010
99. Barbeau S, Porto Ribeiro T, Gilbert G, Cardouat G, Baudrimont I, Freund-Michel V, et al. [Involvement of the piezo1 and TRPV4 stretch-activated channels in pulmonary hypertension]. Rev Mal Respir. (2022) 39:79–83. doi: 10.1016/j.rmr.2022.01.013
100. Humbert M, Guignabert C, Bonnet S, Dorfmuller P, Klinger JR, Nicolls MR, et al. Pathology and pathobiology of pulmonary hypertension: state of the art and research perspectives. Eur Respir J. (2019) 53:1801887. doi: 10.1183/13993003.01887-2018
101. Song S, Carr SG, McDermott KM, Rodriguez M, Babicheva A, Balistrieri A, et al. STIM2 (Stromal interaction molecule 2)-mediated increase in resting cytosolic free Ca(2+) concentration stimulates PASMC proliferation in pulmonary arterial hypertension. Hypertension. (2018) 71:518–29. doi: 10.1161/HYPERTENSIONAHA.117.10503
102. Stenmark KR, Frid MG, Graham BB, Tuder RM. Dynamic and diverse changes in the functional properties of vascular smooth muscle cells in pulmonary hypertension. Cardiovasc Res. (2018) 114:551–64. doi: 10.1093/cvr/cvy004
103. Tang H, Yamamura A, Yamamura H, Song S, Fraidenburg DR, Chen J, et al. Pathogenic role of calcium-sensing receptors in the development and progression of pulmonary hypertension. Am J Physiol Lung Cell Mol Physiol. (2016) 310:L846–59. doi: 10.1152/ajplung.00050.2016
104. Li MX, Jiang DQ, Wang Y, Chen QZ, Ma YJ, Yu SS, et al. Signal mechanisms of vascular remodeling in the development of pulmonary arterial hypertension. J Cardiovasc Pharmacol. (2016) 67:182–90. doi: 10.1097/FJC.0000000000000328
105. Wang Z, Chen J, Babicheva A, Jain PP, Rodriguez M, Ayon RJ, et al. Endothelial upregulation of mechanosensitive channel Piezo1 in pulmonary hypertension. Am J Physiol Cell Physiol. (2021) 321:C1010–27. doi: 10.1152/ajpcell.00147.2021
106. John L, Ko NL, Gokin A, Gokina N, Mandala M, Osol G. The Piezo1 cation channel mediates uterine artery shear stress mechanotransduction and vasodilation during rat pregnancy. Am J Physiol Heart Circ Physiol. (2018) 315:H1019–26. doi: 10.1152/ajpheart.00103.2018
107. Cogolludo Moreno L, Villamor E. Mechanisms controlling vascular tone in pulmonary arterial hypertension: implications for vasodilator therapy. Pharmacology. (2007) 79:65–75. doi: 10.1159/000097754
108. Chen J, Rodriguez M, Miao J, Liao J, Jain PP, Zhao M, et al. Mechanosensitive channel Piezo1 is required for pulmonary artery smooth muscle cell proliferation. Am J Physiol Lung Cell Mol Physiol. (2022) 322:L737–60. doi: 10.1152/ajplung.00447.2021
109. Cui X, Pan G, Chen Y, Guo X, Liu T, Zhang J, et al. The p53 pathway in vasculature revisited: a therapeutic target for pathological vascular remodeling? Pharmacol Res. (2021) 169:105683. doi: 10.1016/j.phrs.2021.105683
110. Kuhr FK, Smith KA, Song MY, Levitan I, Yuan JX. New mechanisms of pulmonary arterial hypertension: role of Ca(2)(+) signaling. Am J Physiol Heart Circ Physiol. (2012) 302:H1546–62. doi: 10.1152/ajpheart.00944.2011
Keywords: vascular remodeling, mechanosensitive ion channel Piezo1, endothelial cells, vascular smooth muscle cell, pathology
Citation: Xu H, He Y, Hong T, Bi C, Li J and Xia M (2022) Piezo1 in vascular remodeling of atherosclerosis and pulmonary arterial hypertension: A potential therapeutic target. Front. Cardiovasc. Med. 9:1021540. doi: 10.3389/fcvm.2022.1021540
Received: 17 August 2022; Accepted: 12 September 2022;
Published: 29 September 2022.
Edited by:
Marie Billaud, Brigham and Women's Hospital and Harvard Medical School, United StatesReviewed by:
Swapnil K. Sonkusare, University of Virginia, United StatesJean-Francois Quignard, Université de Bordeaux, France
Copyright © 2022 Xu, He, Hong, Bi, Li and Xia. This is an open-access article distributed under the terms of the Creative Commons Attribution License (CC BY). The use, distribution or reproduction in other forums is permitted, provided the original author(s) and the copyright owner(s) are credited and that the original publication in this journal is cited, in accordance with accepted academic practice. No use, distribution or reproduction is permitted which does not comply with these terms.
*Correspondence: Mingfeng Xia, xiamingfeng@sdutcm.edu.cn; Jing Li, bmsjingl@leeds.ac.uk
†These authors have contributed equally to this work