- 1Department of Radiology, West China Hospital, Sichuan University, Chengdu, Sichuan, China
- 2Department of Endocrinology, West China Hospital, Sichuan University, Chengdu, Sichuan, China
Background: This study aims to investigate the association of epicardial adipose tissue (EAT) accumulation with cardiac function and atrioventricular coupling in a cohort of postmenopausal women assessed by cardiac magnetic resonance imaging (CMR).
Materials and methods: Overall, 283 postmenopausal women (mean age 61.5 ± 9.1 years) who underwent CMR examination were enrolled. Participants were classified into four groups by the quartile of EAT volume. EAT volume was quantified on short-axis cine stacks covering the entire epicardium. CMR-derived cardiac structure and function, including left atrial (LA)- volume, emptying fraction, deformation, and left ventricular (LV)- mass, volume, ejection fraction, and deformation, were compared among the four groups of graded EAT volume.
Results: Left ventricular mass (LVM) and LV remodeling index were both increased in the group with the highest EAT volume, compared to those in the lowest quartile (p = 0.016 and p = 0.003). The LV global longitudinal strain (LV-GLS), circumferential strain (LV-GCS), and LA- reservoir strain (LA-RS), conduit strain (LA-CS), and booster strain (LA-BS), were all progressively decreased from the lowest quartile of EAT volume to the highest (all p < 0.05). Multivariable linear regression analyses showed that EAT was independently associated with LV-GLS, LA-RS, LA-CS, and LA-BS after adjusting for body mass index and other clinical factors.
Conclusion: Epicardial adipose tissue accumulation is independently associated with subclinical LV and LA function in postmenopausal women. These associations support the role of EAT in mediating deleterious effects on cardiac structure and function.
Introduction
During menopausal transition, women are susceptible to metabolic alterations. Redistribution of body fat from the subcutaneous area to the intra-abdominal visceral area is an important metabolic change for women after menopause. Previous studies have shown that epicardial adipose tissue (EAT) volume tends to be expanded particularly in postmenopausal women (1, 2). EAT was indicated to be associated with hypertension, coronary microvascular dysfunction, and diastolic filling restriction in women but not in men (1, 3, 4). In addition, studies have shown that in the presence of hemodynamic stress, women tend to present more frequently with left ventricular (LV) hypertrophy, smaller LV volumes, and preserved ejection fraction, compared to age-matched men (5, 6). These notable sex differences indicate that estrogen deficiency and EAT might play a role in mediating cardiac abnormalities in postmenopausal women.
Cardiac magnetic resonance (CMR) imaging has been widely used in the evaluation of heart structure and function. CMR tissue tracking has been validated to have excellent producibility and reproducibility in evaluating LV myocardial deformation. Recently, the importance of left atrial (LA) phasic function and feature tracking strain has been increasingly recognized. A prior study indicated that LA dysfunction preceded the onset of heart failure (7). CMR-derived phasic LA function and strain have been suggested to be able to serve as sensitive imaging biomarkers in the assessment and stratification of diastolic dysfunction (8, 9). Furthermore, CMR has been validated to be able to quantify the epicardial fat tissue using a simple volumetric technique on a standard clinical steady-state free-precession sequence (10).
To the best of our knowledge, few studies on this subject have assessed EAT volume and cardiac structure and function by using CMR. Therefore, we aimed to investigate the association of EAT accumulation with cardiac function and atrioventricular coupling in a cohort of postmenopausal women by CMR.
Materials and methods
Study population
This study was approved by the Biomedical Research Ethics Committee of our hospital and conducted in accordance with the Declaration of Helsinki. Written informed consent was waived due to the retrospective nature of this study.
In this cross-sectional study, we included a cohort of 283 postmenopausal women who underwent CMR examination between January 2015 and June 2021. The menopausal status of the participants was recorded according to self-report. Women with both natural menopause and surgical menopause were included. The demographic and clinical characteristics of the included individuals were recorded according to digital medical records. Triglyceride-to-high density lipoprotein cholesterol ratio (TG/HDL) was calculated to indicate insulin resistance level (11).
Exclusion criteria were as follows: (a) patients with pericardial effusion; (b) atrial fibrillation; (c) obstructive coronary artery disease and myocardial infarction, (d) myocarditis and pericarditis, (e) moderate to severe valvular disease, (f) primary and secondary cardiomyopathies, and (g) poor image quality and unavailable to derive CMR parameters.
Cardiac magnetic resonance protocol
All CMR scans were performed using a 3.0T scanner (Siemens Healthcare, Erlangen, Germany). Balanced steady-state free-precession sequence was used to obtain cine images. Three long-axis views (2-chamber, 3-chamber, 4-chamber) and consecutive short-axis slices covering the entire LV were obtained with the following parameters: temporal resolution, 33.22 ms; repetition time, 2.77 ms; echo time, 1.31 ms; field of view, 234 mm × 280 mm and slice thickness, 8 mm.
Imaging analysis
Measurement of left ventricular structure and function
All CMR parameters were assessed using a commercially available software (CVI42; Circle Cardiovascular Imaging, Inc., Calgary, AB, Canada). LV structural parameters, including LV end-diastolic volume (LV-EDV), LV end-systolic volume (LV-ESV), and LV mass (LVM), were attained by manually tracing the endocardial and epicardial contours of the left ventricle at the end-diastolic and end-systolic phases on the short-axis stacks. LV functional parameters included LVEF and LV myocardial strain. LV myocardial strain indices were acquired by loading the short-axis stacks and the two-chamber and four-chamber long-axis images into the feature tracking module. The software then computes the LV global radial (LV-GRS), circumferential (LV-GCS), and longitudinal peak strain (LV-GLS) (12).
Measurement of left atrial structure and function
Left atrial parameters were obtained as previously described (13). LA structural parameters included maximum LA volume (LAVmax), minimum LA volume (LAVmin), and pre-atrial contraction LA volume (LAVac). LA functional parameters included phasic volumetric-based LA emptying fractions and LA strain-based indices. LA endocardial and epicardial borders were manually delineated in the two- and four-chamber long-axis images using LV end-diastole as a reference phase. LA appendage and pulmonary veins were excluded from the LA volume. Then, the software automatically traced the atrial border in the subsequent phases. Manual adjustments were performed to obtain optimal tracking of the LA border. The software then computes the LA peak longitudinal reservoir strain (LA-RS), conduit strain (LA-CS), and booster strain (LA-BS). Total LA emptying fraction (LAEFT), a measure of reservoir function, was calculated as (LAVmax–LAVmin)/LAVmax. Passive LA emptying fraction (LAEFP), a measure of conduit function, was calculated as (LAVmax–LAVac)/LAVmax. Booster LA emptying fraction (LAEFB), a measure of atrial contractile pump function, was calculated as (LAVac–LAVmin)/LAVac. Representative images of LV and LA longitudinal strain are shown in Figure 1.
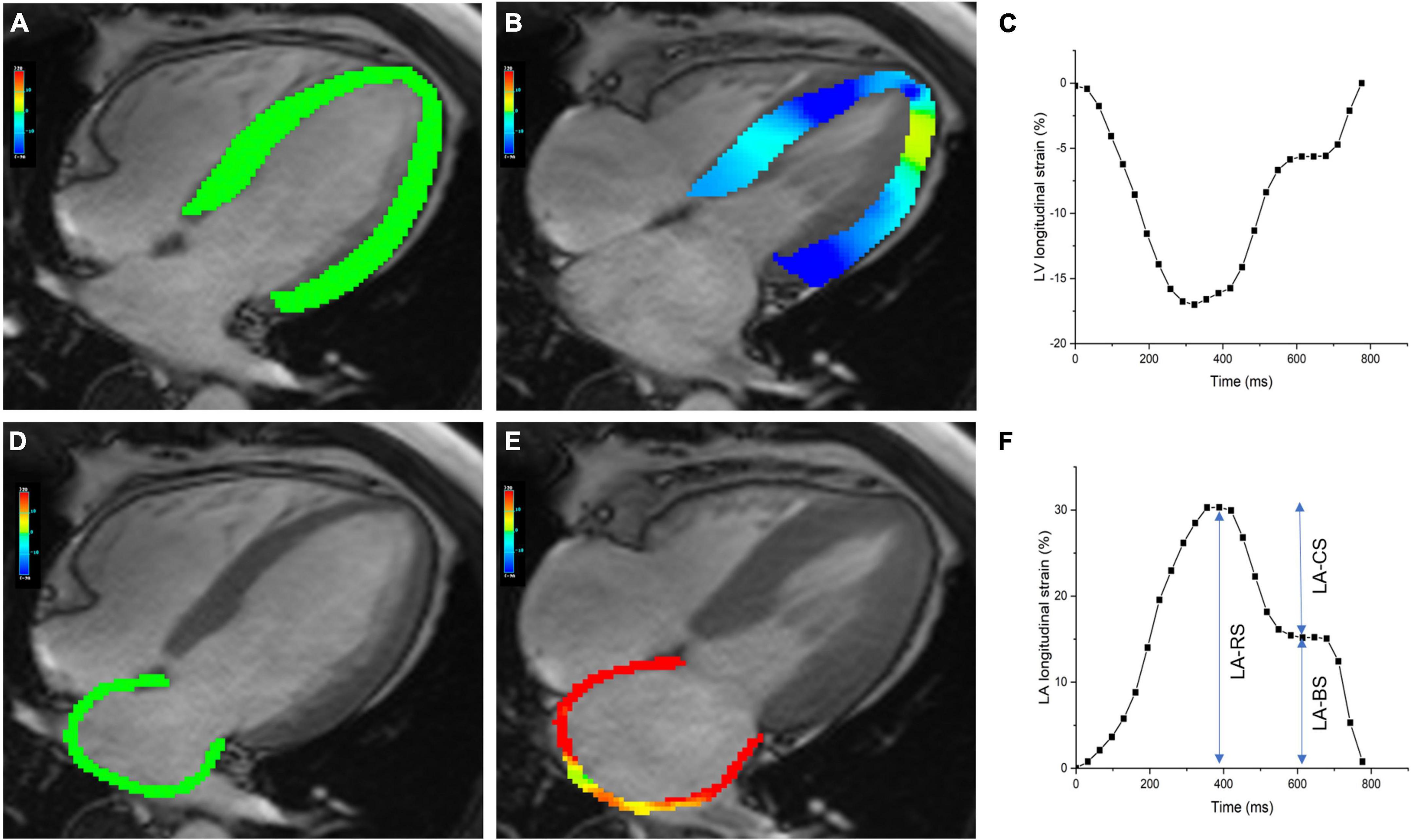
Figure 1. Cardiac magnetic resonance (CMR)-derived left ventricular and atrial longitudinal strain. Panels (A,B) show the pseudocolor maps of LV longitudinal strain in the four-chamber view at the end-diastolic and end-systolic phases. Panel (C) shows representative plot of LV longitudinal strain. Panels (D,E) show the pseudocolor maps of LA longitudinal strain in the four-chamber view at the end-diastolic and end-systolic phases. Panel (F) shows a plot of LA longitudinal strain, along with measures of reservoir, conduit, and booster strain. LA-RS, LA reservoir strain; LA-CS, LA conduit strain; LA-BS, LA booster strain.
Measurement of epicardial adipose tissue volume
The measurement of EAT volume has been previously described (10, 14). The areas of EAT were delineated on consecutive short-axis cine images. Epicardial border and visceral pericardial border on each slice from the level of the mitral valve to the apical slice were manually traced (Figure 2). Then the EAT volume was calculated by summation of the results of each slice’s area multiplied by the slice thickness based on the modified Simpson’s rule.
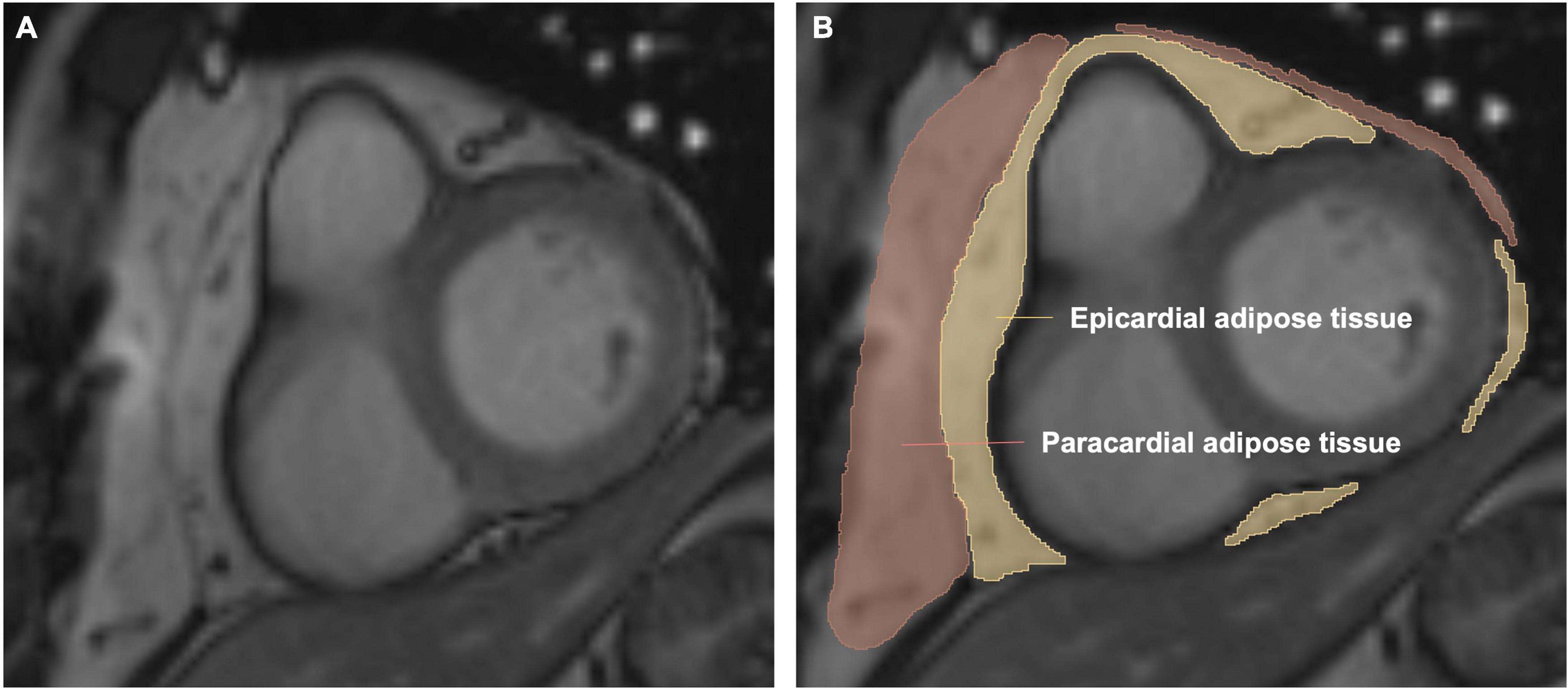
Figure 2. Volumetric assessment of the epicardial adipose tissue (EAT) on short-axis slices. Panels (A,B) are representative images of one short-axis slice. Epicardial adipose tissue is shown in yellow. Paracardial adipose tissue is shown in red. Pericardial adipose tissue is epicardial adipose tissue plus paracardial adipose tissue. The EAT volume was calculated by summation of the results of each slice’s area multiplied by the slice thickness based on the modified Simpson’s rule.
Morphological LA and LV parameters and the EAT volume were indexed to body surface area (BSA). Reproducibility was assessed for EAT volume and LA and LV strain parameters. To determine the intra-observer reproducibility, one observer (SH) performed all the measurements at first and repeated in 30 randomly selected CMR scans 1 month later. And inter-observer reproducibility was evaluated by comparing the measurements from the same collection of images by another experienced observer (KS).
Statistical analysis
Statistical analyses were conducted with SPSS (Version 19; IBM, Armonk, NY, USA) and Graphpad Prism (Version 7.0a, GraphPad Software Inc., San Diego, CA, USA). Baseline characteristics and CMR indices were all summarized across the quartile of EAT volume. Continuous data are expressed as the means ± SDs or medians with interquartile ranges. Categorical data are expressed as numbers (percentages). Continuous variables were compared among the four groups of EAT volume using one-way analysis of variance (ANOVA). Dichotomous variables were compared among groups by using χ test. Pearson’s or Spearman correlation analyses were performed to evaluate the bivariable correlations among EAT volume and other CMR parameters as appropriate. Multivariable linear regression analyses were conducted using body mass index (BMI), EAT and clinical factors as independent variables and LA and LV myocardial longitudinal strain as dependent variables. Candidate variables with no collinearity and a p-value < 0.1 in the univariate analyses as well as factors based on clinical grounds were included in the multivariable models. The intraclass correlation coefficient (ICC) was used to assess the inter- and intra- observer reproducibility. Two-sided p < 0.05 was considered statistically significant.
Results
Baseline characteristics of the included participants
In total, 283 postmenopausal women were enrolled in this study. Baseline demographic and clinical characteristics were summarized across the quartiles of EAT volume in Table 1. The ranges of EAT volume of the four groups were Q1: <37.5 ml/m2, Q2: 37.5∼48.9 ml/m2, Q3: 48.9∼62.0 ml/m2, and Q4: > 62.0 ml/m2, respectively. The average age of the study cohort was 61.5 ± 9.1 years. Six women in the study population had surgical menopause. There were graded increases in BMI and TG/HDL across the quartiles of EAT. And BMI was significantly correlated with EAT volume (r = 0.354). EAT was inversely correlated with the level of HDL cholesterol (r = −0.214). Age was found to be related to EAT volume (r = 0.399). Women with higher EAT volumes were significantly older than those in the lower quartile. Presence of hypertension was more frequent in participants with a larger EAT volume. The presence of diabetes mellitus had a graded increase from the lowest to the highest quartile of EAT, but the difference was not statistically significant.
Comparisons of cardiac magnetic resonance-derived cardiac structure and function among quartiles of epicardial adipose tissue volume
Cardiac structural and functional parameters by CMR according to the quartile distribution of EAT volume are presented in Table 2 and Figure 3. The mean EAT volumes of the four groups were Q1 = 29.7 ± 5.9 ml/m2 vs. Q2 = 42.7 ± 3.3 ml/m2 vs. Q3 = 54.8 ± 4.1 ml/m2 vs. Q4 = 73.3 ± 9.6 ml/m2. LVM and LV remodeling index were both increased in the group with the highest EAT volume, compared to those in the lowest quartile. LV-EDV and LV-ESV did not show any difference across the four groups. There was no significant difference in LVEF among the groups. The LV-GLS and LV-GCS, but not LV-GRS, was significantly reduced in the highest quartile of EAT.
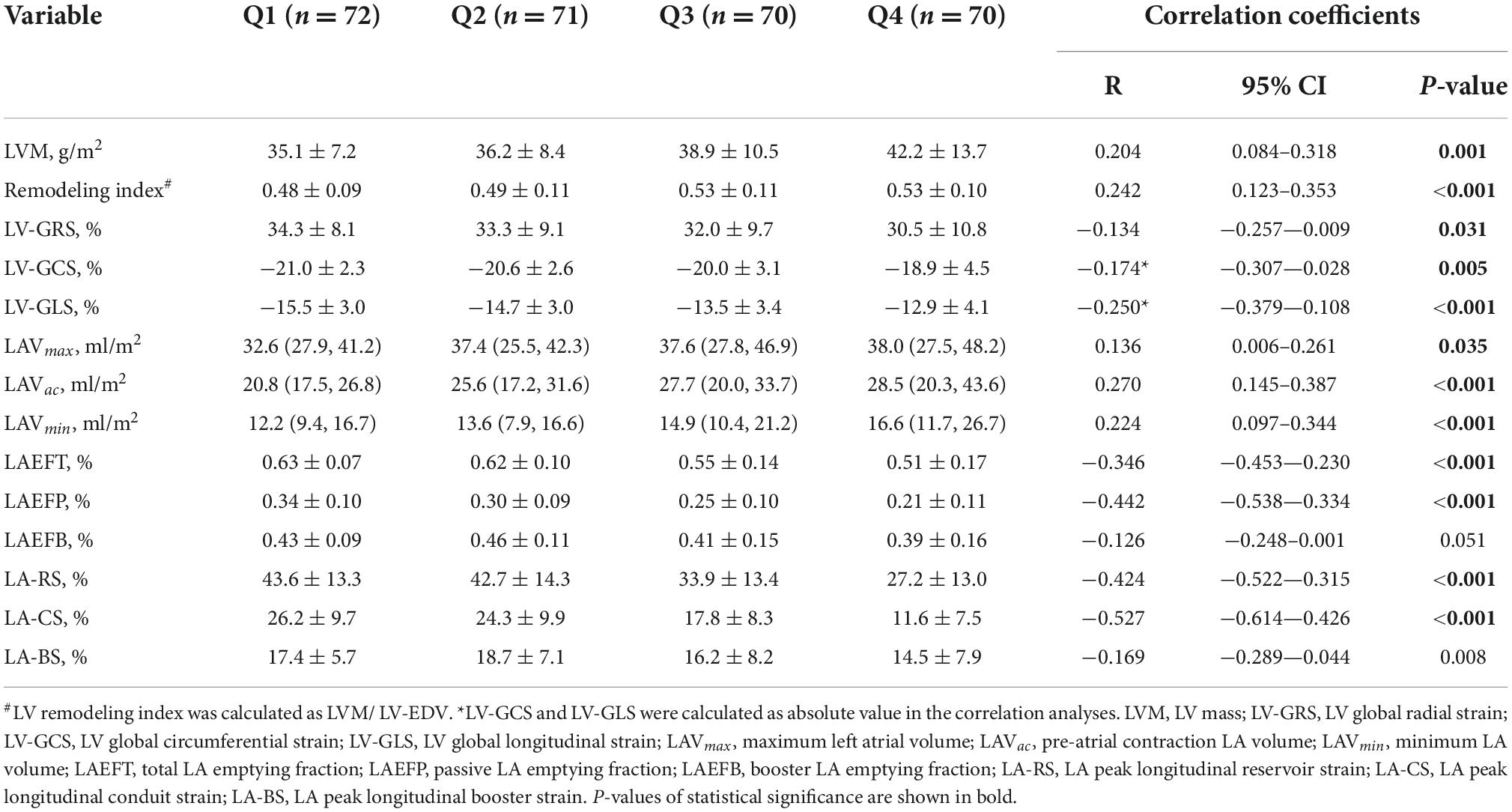
Table 2. Cardiac structural and functional parameters by cardiac magnetic resonance (CMR) and their correlations with epicardial adipose tissue (EAT) volume.
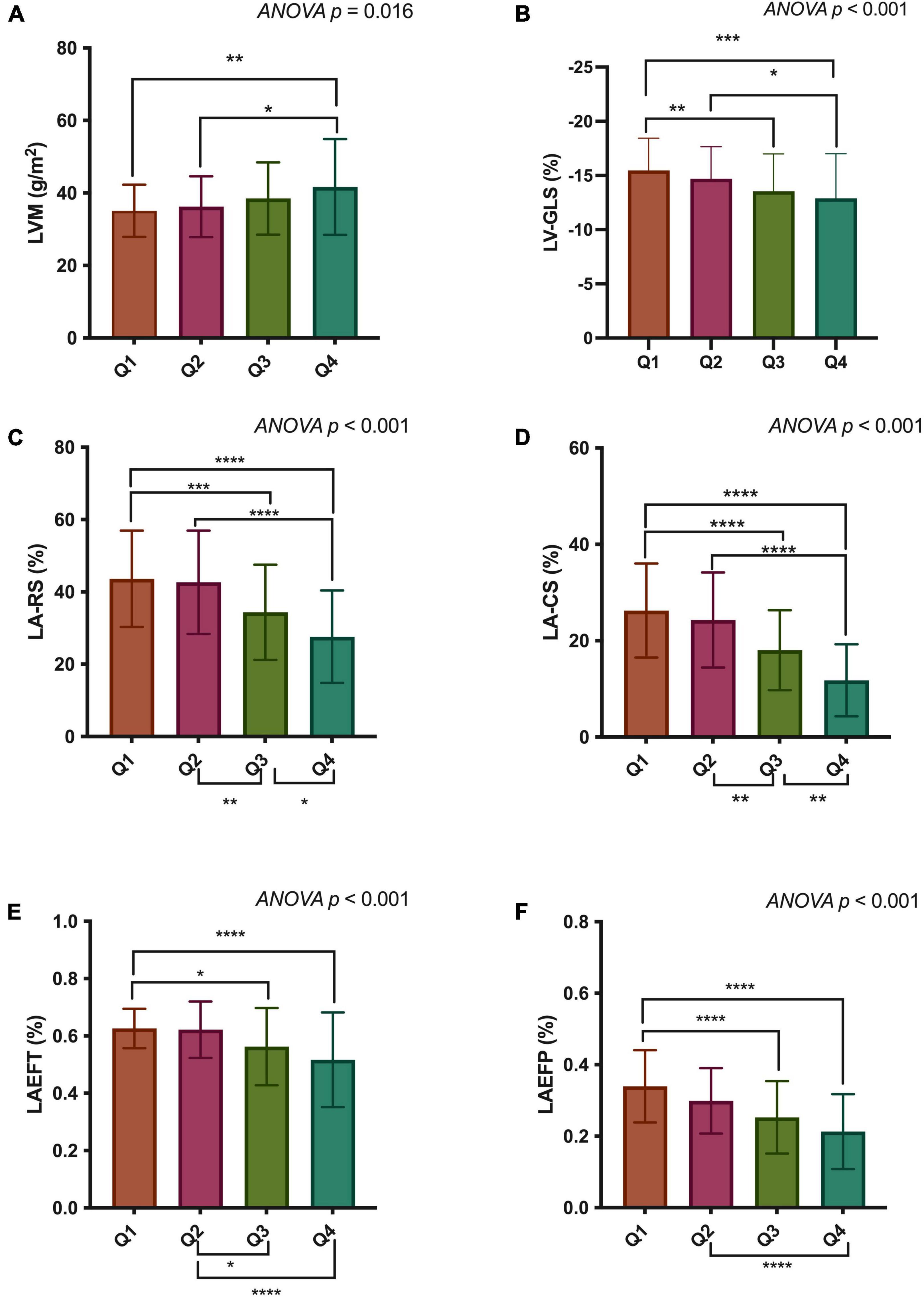
Figure 3. Comparisons of LVM (A), LV-GLS (B), LA-RS (C), LA-CS (D), LAEFT (E), and LAEFP (F) among quartiles of epicardial adipose tissue (EAT) volume. LVM, left ventricular mass; LV-GLS, LV global longitudinal strain; LA-RS, left atrial reservoir strain; LA-CS, LA conduit strain; LAEFT, total LA emptying fraction; LAEFP, passive LA emptying fraction. *p < 0.05; **p < 0.01, ***p < 0.001; ****p < 0.0001.
LAVmin and LAVac were markedly enlarged in participants with larger EAT volumes. But no significant difference was found in LAVmax among the groups. Regarding volume-based LA function, LAEFT and LAEFP were markedly decreased in the higher EAT group. No significant difference was observed in LAEFB among the four groups. The strain-based LA function indices, including LA-RS, LA-CS, and LA-BS, all progressively decreased from the lowest quartile of EAT volume to the highest.
Associations between epicardial adipose tissue and cardiac structural and functional indices
Univariate correlation analyses of EAT and other CMR indices are presented in Table 2. EAT volume had a weak correlation with LVM (r = 0.204) and the remodeling index (r = 0.242). LV-GLS (r = −0.250), LV-GCS (r = −0.174), and LV-GRS (r = −0.134) were inversely correlated with EAT volume. Volume-based LA function indices were negatively related to EAT volume, with only LAEFT (r = −0.346) and LAEFP (r = −0.442) being statistically significant. LA-RS (r = −0.424) and LA-CS (r = −0.527), and LA-BS (r = −0.169) were also correlated with EAT volume.
In the multivariable linear regression analyses, age, systolic blood pressure, heart rate, hypertension, diabetes, dyslipidemia, menopausal age, surgical menopause, TG/HDL, BMI, and EAT volume were included as independent variables. Among them, BMI (β = −0.261), EAT volume (β = −0.149) and diabetes (β = −0.286) were found to be independently associated with LV-GLS. Furthermore, EAT volume was also found to be independently correlated with LA-RS (β = −0.277), LA-CS (β = −0.324), and LA-BS (β = −0.210) (Table 3).
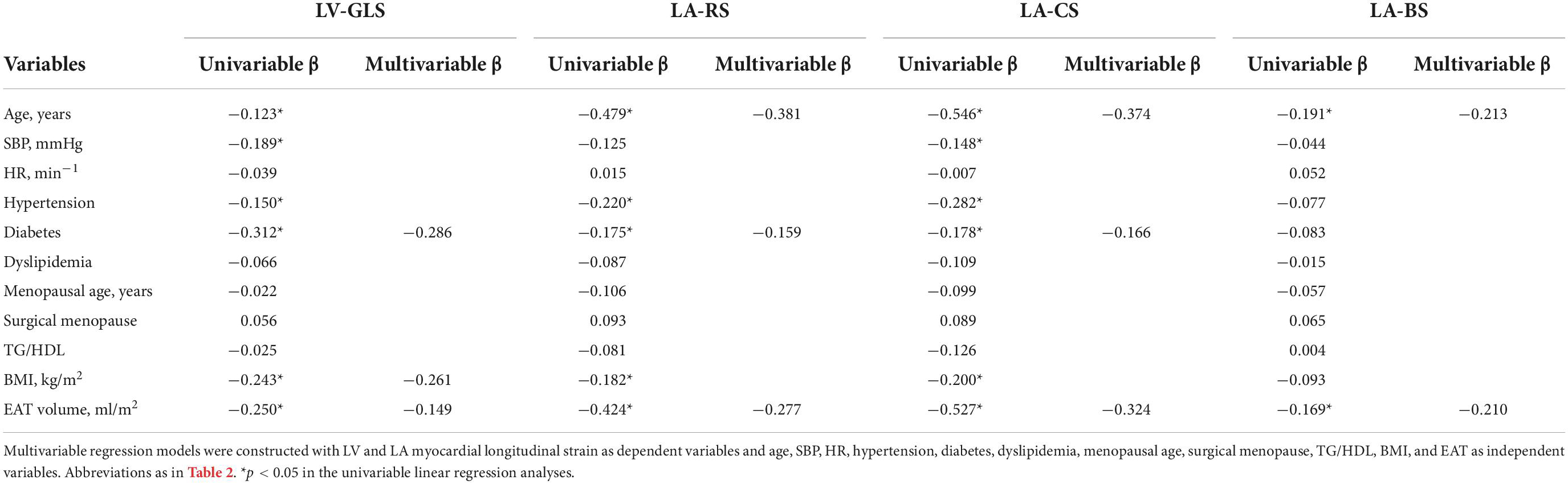
Table 3. Multivariable linear regression of epicardial adipose tissue (EAT) and cardiac function parameters.
Intra- and inter-observer reproducibility of cardiac magnetic resonance parameters
The intra-observer and inter-observer reproducibility of EAT volume, and LA and LV strains were considered excellent (ICCs ranged from 0.882 to 0.952) (Supplementary Table 1).
Discussion
In this study, we used CMR to explore the associations of EAT with cardiac functional and structural parameters. First, we found that LV global longitudinal strain was progressively reduced with the increasing of EAT volume. Second, the phasic emptying function and deformation of LA were also found to be gradually decreased from the lowest quartile of EAT volume to the highest. Third, EAT was correlated with LV GLS and LA strains independent of age, systolic blood pressure, heart rate, hypertension, diabetes, dyslipidemia, menopausal age, surgical menopause, TG/HDL, and BMI.
Previous studies on EAT mainly focused on EAT thickness around the right ventricular free wall or pericardial adipose tissue (epicardial adipose tissue plus paracardial adipose tissue) measured by echocardiogram or CT. Kim et al. found that pericardial adipose tissue (PAT) was more strongly associated with the subclinical LV dysfunction than BMI and waist circumference (15). However, EAT is embryologically different from paracardial adipose tissue. And EAT is anatomically more closely connected to the coronary arteries and myocardium than paracardial adipose tissue (16). The proinflammatory cytokines released by EAT can directly impair the myocardium, due to the absence of a fascial plane between the two structures (17). A previous echocardiographic study that included 1,004 participants found that only EAT was significantly correlated with diastolic dysfunction, whereas, PAT was not associated with the decreased diastolic function (18). Therefore, we focused on investigating the specific association of EAT, not PAT, with the cardiac structure and function during the same CMR examination.
Association of epicardial adipose tissue with volumetric- and strain-based phasic left atrial function
Impairment of LA function has been proposed to precede the development of heart failure in a large longitudinal population of asymptomatic participants (7). In our study, we found that LAEFT and LAEFP, but not LAEFB, gradually decreased as the EAT volume accumulated. Evidence about the booster function of LA is still conflicting (19, 20). Even though the volumetric booster function was not significantly different among the groups, the booster strain along with the other two components of LA strain, demonstrated significant differences across the quartile of EAT in our study. This is because that strain-based indices are more sensitive in evaluating atrial mechanics than volumetric indices (21). As for the reservoir function, it is an indicator for LA compliance, reflecting the relaxation of LA during LV systole. The reservoir strain has been previously demonstrated to be correlated with LV filling pressure (22) and the incidence of heart failure (7). The LA-CS of the conduit phase also had a strong association with EAT volume. Considering that conduit function is mainly influenced by the LV relaxation, the abnormality of conduit strain could indicate an early stage of LV diastolic dysfunction.
In a prior study, the researchers demonstrated that LA strain was correlated with EAT in patients with coexisting obesity and diabetes (23). However, possibly due to the small sample size of this study, no significant correlation was observed between EAT and LA function when the patient group and control group were evaluated separately. The researchers assumed that this might indicate that abnormal LA function only occurs when the EAT volume is over a certain amount. Our study was supportive of this assumption. The volume- and strain-based LA function were all significantly reduced in the highest amount of EAT compared to the low quartile of EAT volume.
Association of epicardial adipose tissue with left ventricular systolic function
The relationship between EAT and LV diastolic function has been well-established in several echocardiographic studies (24). However, evidence about the association between EAT and LV systolic function remains to be elucidated. Several studies using speckle tracking strain analysis by echocardiography demonstrated that EAT is inversely correlated with LV global longitudinal strain (25, 26). Consistent with these results, we observed that longitudinal strain was reduced in the high EAT group. We assumed that the link between EAT and longitudinal strain could be explained by microvascular dysfunction and interstitial fibrosis induced by adipokines and cytokines secreted by EAT (25). These abnormalities mainly affect the subendocardial layer of the myocardium, leading to a reduction in longitudinal LV mechanics in the subclinical stage of disease.
Potential mechanisms underlying the influence of epicardial adipose tissue on cardiac structure and function
Several relevant mechanisms have been proposed to explain the associations between EAT and cardiac structure and function (27). The first is that EAT could impair LV diastolic filling by a regional mechanical force. Findings of impaired diastolic function and enlarged LA volume in the absence of LV hypertrophy observed in uncomplicated obesity suggest the mechanical role of local adipose depot around the ventricles (28).
Furthermore, EAT could also affect LV structure and function by a paracrine pathway due to the anatomic proximity of EAT to the myocardium (29). A recent study by Ng et al. which quantified the intramyocardial fat content and myocardial interstitial fibrosis by CMR, suggested that the redundant EAT might impair the contractile function by mediating an increase in myocardial fat accumulation and interstitial fibrosis (25).
Third, in our diverse population of postmenopausal women with a relatively high presence of hypertension, obesity and diabetes, there was a possible systemic inflammatory effect induced by these comorbidities that caused the expansion of EAT volume and abnormalities in the heart (30, 31). Under the influence of systemic inflammation, EAT can release various pro-inflammatory cytokines. Thus, the EAT can further aggravates these systemic inflammatory influences on the myocardium and has a deleterious impact on cardiac structure and function. However, a prior clinical study by Woerden et al. did not observe significant association between EAT and the level of C-reactive protein or leucocytes (32). Possibly because the effect of EAT is too small to be reflected via peripheral venepuncture. Also, the sample size is relatively small.
As our correlation analyses showed, age and EAT were both independent factors that inversely correlated with LV-GLS and LA strains. Several previous studies also found a strong relation between age and EAT (14, 33). In the study by de Vos et al. age-adjusted regression analyses showed that EAT was positively related to weight, BMI, waist circumference, waist-to-hip ratio and subclinical coronary atherosclerosis (34). However, we were unable to examine the effect of aging process on EAT expansion and other cardiac abnormalities in this study.
Limitations
This study has several limitations. First, this was a cross-sectional study. Therefore, we were unable to demonstrate a causal relationship between the increased EAT, laboratory biomarkers, co-morbidities and abnormalities in cardiac structure and function. Second, data on waist circumference, waist to hip ratio or abdominal visceral adipose fat are lacking in the majority of included participants, since this was a retrospective study. Inclusion of these data would have made our study more comprehensive. Third, this study only included postmenopausal women. Whether these results could also be applied to men or premenopausal women needs to be elucidated. Finally, due to the lack of long-term follow-up data, we were unable to evaluate the prognostic role of EAT in our study population. Further longitudinal studies are required to examine the potential of EAT in predicting cardiovascular outcomes.
Conclusion
The accumulation of EAT is independently associated with LV and LA function in postmenopausal women. These associations support the role of EAT in mediating deleterious effects on cardiac structure and function. The assessment of EAT volume may facilitate clinicians to have added information on the impairment of cardiac function. Researchers could commit themselves to developing medicine targeting the epicardial fat tissue to prevent the cardiac remodeling and dysfunction.
Data availability statement
The raw data supporting the conclusions of this article will be made available by the authors, without undue reservation.
Ethics statement
The studies involving human participants were reviewed and approved by West China Hospital of Sichuan University Biomedical Research Ethics Committee. Written informed consent for participation was not required for this study in accordance with the national legislation and the institutional requirements.
Author contributions
SH and Z-GY designed the study. SH was the major contributor to the manuscript drafting and revisions. LJ and JW were responsible for data collecting and sorting. SH and KS analysed the CMR parameters and interpreted the results. W-FY and W-LQ performed the statistical analyses and prepared the tables and figures. YL and YR helped to revise the manuscript critically for important intellectual content. Z-GY supervised the whole work and revised the manuscript. All authors approved the final manuscript.
Funding
This work was supported by the 1⋅3⋅5 project for disciplines of excellence, West China Hospital, Sichuan University (ZYGD18013).
Conflict of interest
The authors declare that the research was conducted in the absence of any commercial or financial relationships that could be construed as a potential conflict of interest.
Publisher’s note
All claims expressed in this article are solely those of the authors and do not necessarily represent those of their affiliated organizations, or those of the publisher, the editors and the reviewers. Any product that may be evaluated in this article, or claim that may be made by its manufacturer, is not guaranteed or endorsed by the publisher.
Supplementary material
The Supplementary Material for this article can be found online at: https://www.frontiersin.org/articles/10.3389/fcvm.2022.1015983/full#supplementary-material
Abbreviations
EAT, epicardial adipose tissue; LV, left ventricular; CMR, cardiac magnetic resonance; LA, left atrial; LVEF, left ventricular ejection fraction; eGFR, estimated glomerular filtration rate; EDV, end-diastolic volume; ESV, end-systolic volume; LVM, LV mass; GRS, global radial strain; GCS, global circumferential strain; GLS, global longitudinal strain; RS, reservoir strain; CS, conduit strain; BS, booster strain; LAVmax, maximum LA volume; LAVmin, minimum LA volume; LAVac, pre-atrial contraction LA volume; LAEFT, total LA emptying fraction; LAEFP, passive LA emptying fraction; LAEFB, booster LA emptying fraction; BSA, body surface area; BMI, body mass index; ICC, intraclass correlation coefficient; PAT, pericardial adipose tissue.
References
1. Kim S-A, Kim M-N, Shim W-J, Park S-M. Epicardial adipose tissue is related to cardiac function in elderly women, but not in men. Nutr Metab Cardiovasc Dis. (2017) 27:41–7. doi: 10.1016/j.numecd.2016.11.001
2. Baragetti A, Pisano G, Bertelli C, Garlaschelli K, Grigore L, Fracanzani AL, et al. Subclinical atherosclerosis is associated with Epicardial Fat Thickness and hepatic steatosis in the general population. Nutr Metab Cardiovasc Dis. (2016) 26:141–53. doi: 10.1016/j.numecd.2015.10.013
3. Alam MS, Green R, de Kemp R, Beanlands RS, Chow BJW. Epicardial adipose tissue thickness as a predictor of impaired microvascular function in patients with non-obstructive coronary artery disease. J Nucl Cardiol. (2013) 20:804–12. doi: 10.1007/s12350-013-9739-6
4. Shim IK, Cho K-I, Kim H-S, Heo J-H, Cha TJ. Impact of gender on the association of epicardial fat thickness, obesity, and circadian blood pressure pattern in hypertensive patients. J Diabetes Res. (2015) 2015:924539. doi: 10.1155/2015/924539
5. Kararigas G, Dworatzek E, Petrov G, Summer H, Schulze TM, Baczko I, et al. Sex-dependent regulation of fibrosis and inflammation in human left ventricular remodelling under pressure overload: Sex-specific LV gene regulation in pressure overload. Eur J Heart Fail. (2014) 16:1160–7. doi: 10.1002/ejhf.171
6. Regitz-Zagrosek V, Kararigas G. Mechanistic pathways of sex differences in Cardiovascular Disease. Physiol Rev. (2017) 97:1–37. doi: 10.1152/physrev.00021.2015
7. Habibi M, Chahal H, Opdahl A, Gjesdal O, Helle-Valle TM, Heckbert SR, et al. Association of CMR-measured LA function with heart failure development: results from the MESA study. JACC Cardiovasc Imaging. (2014) 7:570–9. doi: 10.1016/j.jcmg.2014.01.016
8. Aquaro GD, Pizzino F, Terrizzi A, Carerj S, Khandheria BK, Di Bella G. Diastolic dysfunction evaluated by cardiac magnetic resonance: the value of the combined assessment of atrial and ventricular function. Eur Radiol. (2019) 29:1555–64. doi: 10.1007/s00330-018-5571-3
9. Peters DC, Lamy J, Sinusas AJ, Baldassarre LA. Left atrial evaluation by cardiovascular magnetic resonance: sensitive and unique biomarkers. Eur Heart J Cardiovasc Imaging. (2021) 23:14–30. doi: 10.1093/ehjci/jeab221
10. Nelson AJ, Worthley MI, Psaltis PJ, Carbone A, Dundon BK, Duncan RF, et al. Validation of cardiovascular magnetic resonance assessment of pericardial adipose tissue volume. J Cardiovasc Magn Reson. (2009) 11:1–8. doi: 10.1186/1532-429X-11-15
11. Zhao Q, Cheng Y-J, Xu Y-K, Zhao ZW, Liu C, Sun TN, et al. Comparison of various insulin resistance surrogates on prognostic prediction and stratification following percutaneous coronary intervention in patients with and without type 2 diabetes mellitus. Cardiovasc Diabetol. (2021) 20:190. doi: 10.1186/s12933-021-01383-7
12. Shi K, Yang M-X, Huang S, Yan WF, Yan WF, Li Y, et al. Effect of diabetes mellitus on the development of left ventricular contractile dysfunction in women with heart failure and preserved ejection fraction. Cardiovasc Diabetol. (2021) 20:185.
13. Zhang Y, Li X-M, Shen M-T, Huang S, Li Y, Yang Z-G. Atrioventricular coupling and left atrial abnormality in type 2 diabetes mellitus with functional mitral regurgitation patients verified by cardiac magnetic resonance imaging. Cardiovasc Diabetol. (2022) 21:100. doi: 10.1186/s12933-022-01536-2
14. Huang S, Li Y, Jiang L, Ren Y, Wang J, Shi K, et al. Impact of Type 2 diabetes mellitus on epicardial adipose tissue and myocardial microcirculation by MRI in postmenopausal women. J Magn Reson Imaging. (2022) 56:1404–13.
15. Kim J-S, Kim SW, Lee JS, Lee SK, Abbott R, Lee KY, et al. Association of pericardial adipose tissue with left ventricular structure and function: a region-specific effect? Cardiovasc Diabetol. (2021) 20:26. doi: 10.1186/s12933-021-01219-4
16. Sacks HS, Fain JN. Human epicardial fat: what is new and what is missing? Clin Exp Pharmacol Physiol. (2011) 38:879–87. doi: 10.1111/j.1440-1681.2011.05601.x
17. Packer M. Epicardial adipose tissue may mediate deleterious effects of obesity and inflammation on the myocardium. J Am Coll Cardiol. (2018) 71:2360–72. doi: 10.1016/j.jacc.2018.03.509
18. Christensen RH, Hansen CS, Scholten BJ, Jensen MT, Pedersen BK, Schnohr P, et al. Epicardial and pericardial adipose tissues are associated with reduced diastolic and systolic function in type 2 diabetes. Diabetes Obes Metab. (2019) 21:2006–11. doi: 10.1111/dom.13758
19. Tadic M, Ilic S, Cuspidi C, Ivanovic B, Bukarica L, Kostic N, et al. Left and right atrial phasic function and deformation in untreated patients with prediabetes and type 2 diabetes mellitus. Int J Cardiovasc Imaging. (2015) 31:65–76. doi: 10.1007/s10554-014-0536-3
20. Steele JM, Urbina EM, Mazur WM, Khoury PR, Nagueh SF, Tretter JT, et al. Left atrial strain and diastolic function abnormalities in obese and type 2 diabetic adolescents and young adults. Cardiovasc Diabetol. (2020) 19:163. doi: 10.1186/s12933-020-01139-9
21. Chirinos JA, Sardana M, Ansari B, Satija V, Kuriakose D, Edelstein I, et al. Left atrial phasic function by cardiac magnetic resonance feature tracking is a strong predictor of incident cardiovascular events. Circ Cardiovasc Imaging. (2018) 11:e007512. doi: 10.1161/CIRCIMAGING.117.007512
22. Cameli M, Lisi M, Mondillo S, Padeletti M, Ballo P, Tsioulpas C, et al. Left atrial longitudinal strain by speckle tracking echocardiography correlates well with left ventricular filling pressures in patients with heart failure. Cardiovasc Ultrasound. (2010) 8:14. doi: 10.1186/1476-7120-8-14
23. Evin M, Broadhouse KM, Callaghan FM, McGrath RT, Glastras S, Kozor R, et al. Impact of obesity and epicardial fat on early left atrial dysfunction assessed by cardiac MRI strain analysis. Cardiovasc Diabetol. (2016) 15:164. doi: 10.1186/s12933-016-0481-7
24. Mancio J, Azevedo D, Fragao-Marques M, Falcao-Pires I, Leite-Moreira A, Lunet N, et al. Meta-Analysis of relation of epicardial adipose tissue volume to left atrial dilation and to left ventricular hypertrophy and functions. Am J Cardiol. (2019) 123:523–31. doi: 10.1016/j.amjcard.2018.10.020
25. Ng ACT, Strudwick M, van der Geest RJ, Ng ACC, Gillinder L, Goo SY, et al. Impact of epicardial adipose tissue, left ventricular myocardial fat content, and interstitial fibrosis on myocardial contractile function. Circ Cardiovasc Imaging. (2018) 11:e007372. doi: 10.1161/CIRCIMAGING.117.007372
26. Cho D-H, Joo HJ, Kim M-N, Lim D-S, Shim WJ, Park S-M. Association between epicardial adipose tissue, high-sensitivity C-reactive protein and myocardial dysfunction in middle-aged men with suspected metabolic syndrome. Cardiovasc Diabetol. (2018) 17:95. doi: 10.1186/s12933-018-0735-7
27. Fox CS, Gona P, Hoffmann U, Porter SA, Salton CJ, Massaro JM, et al. Pericardial fat, intrathoracic fat, and measures of left ventricular structure and function: the framingham heart study. Circulation. (2009) 119:1586–91. doi: 10.1161/CIRCULATIONAHA.108.828970
28. Iacobellis G, Ribaudo MC, Leto G, Leto G, Zappaterreno A, Vecci E, et al. Influence of excess fat on cardiac morphology and function: Study in uncomplicated obesity. Obes Res. (2002) 10:767–73.
29. Fitzgibbons TP, Czech MP. Epicardial and perivascular adipose tissues and their influence on cardiovascular disease: basic mechanisms and clinical associations. J Am Heart Assoc. (2014) 3:e000582. doi: 10.1161/JAHA.113.000582
30. Nakanishi K, Fukuda S, Tanaka A, Otsuka K, Taguchi H, Yoshikawa J, et al. Epicardial adipose tissue accumulation is associated with renal dysfunction and coronary plaque morphology on multidetector computed tomography. Circ J. (2015) 80:196–201. doi: 10.1253/circj.CJ-15-0477
31. Cheng K, Chu C, Lee K, Lin TH, Hsieh CC, Chiu CC, et al. Adipocytokines and proinflammatory mediators from abdominal and epicardial adipose tissue in patients with coronary artery disease. Int J Obes. (2008) 32:268–74. doi: 10.1038/sj.ijo.0803726
32. van Woerden G, Gorter TM, Westenbrink BD, Willems TP, van Veldhuisen DJ, Rienstra M. Epicardial fat in heart failure patients with mid-range and preserved ejection fraction: Epicardial fat in heart failure. Eur J Heart Fail. (2018) 20:1559–1566. doi: 10.1002/ejhf.1283
33. Cosson E, Nguyen MT, Rezgani I, Tatulashvili S, Sal M, Berkane N, et al. Epicardial adipose tissue volume and coronary calcification among people living with diabetes: a cross-sectional study. Cardiovasc Diabetol. (2021) 20:35. doi: 10.1186/s12933-021-01225-6
Keywords: epicardial adipose tissue, left atrial strain, left ventricular strain, cardiac magnetic resonance imaging, postmenopausal women
Citation: Huang S, Shi K, Jiang L, Ren Y, Wang J, Yan W-F, Qian W-L, Li Y and Yang Z-G (2022) Adverse association of epicardial adipose tissue accumulation with cardiac function and atrioventricular coupling in postmenopausal women assessed by cardiac magnetic resonance imaging. Front. Cardiovasc. Med. 9:1015983. doi: 10.3389/fcvm.2022.1015983
Received: 10 August 2022; Accepted: 24 October 2022;
Published: 11 November 2022.
Edited by:
Sabina Gallina, University of Studies G. d’Annunzio Chieti and Pescara, ItalyReviewed by:
Neftali Eduardo Antonio-Villa, Instituto Nacional de Cardiologia Ignacio Chavez, MexicoHermann Körperich, Heart and Diabetes Center North Rhine-Westphalia, Germany
Copyright © 2022 Huang, Shi, Jiang, Ren, Wang, Yan, Qian, Li and Yang. This is an open-access article distributed under the terms of the Creative Commons Attribution License (CC BY). The use, distribution or reproduction in other forums is permitted, provided the original author(s) and the copyright owner(s) are credited and that the original publication in this journal is cited, in accordance with accepted academic practice. No use, distribution or reproduction is permitted which does not comply with these terms.
*Correspondence: Zhi-Gang Yang, eWFuZ3pnNjY2QDE2My5jb20=