- 1Division of Cardiology, School of Medicine, Johns Hopkins University, Baltimore, MD, United States
- 2Virginia Commonwealth University Medical Center, Richmond, VA, United States
Stress cardiomyopathy (Takotsubo syndrome) is a reversible syndrome stemming from myocardial injury leading to systolic dysfunction and is usually noted in the setting of a stressful event, be it an emotional or physical trigger. While the exact pathophysiology behind stress cardiomyopathy is yet unknown, there is ample evidence suggesting that neurocardiogenic mechanisms may play an important role. Although historically stress cardiomyopathy was generally thought to be a relatively benign condition, there is growing recognition of the cardiovascular complications associated with it despite its reversibility. Our review aims to shed light onto key cardiovascular imaging modalities used to diagnose stress cardiomyopathy while highlighting the role that imaging plays in assessing disease severity, identifying complications, dictating treatment approaches, and in short-term and long-term prognosis.
Background
Takotsubo syndrome, also known as stress cardiomyopathy (stress CM), apical ballooning syndrome, or broken heart syndrome, is increasingly recognized as an important cause of acute reversible myocardial injury and acute systolic dysfunction (1). The origin of the name “takotsubo” refers to octopus traps used in Japan, which resemble the apical ballooning pattern often observed on fluoroscopic left ventriculogram in the classic presentation of stress CM (2). Previously thought to be a rare diagnosis, stress CM currently comprises around 2% of all the patients presenting with concern for acute coronary syndrome (ACS) (3).
Stress CM was initially thought to be a relatively benign, reversible condition, but as prevalence has increased it has become apparent that it is often associated with significant morbidity and mortality (1). There is increasing recognition of associated cardiovascular complications such as left ventricular (LV) outflow tract obstruction, mitral regurgitation, and heart failure which may all lead to cardiogenic shock (4).
In an era where the application of multimodality cardiovascular imaging has been increasing, our review will aim to highlight the diagnosis of stress CM with a particular focus on imaging.
Definition and Diagnosis
Stress CM is defined as a syndrome of transient and acute LV systolic and diastolic dysfunction usually related to a profound emotional or physical stress within the prior 5 days (1, 5). The syndrome is suspected when observed regional wall motion abnormalities (RWMA) cannot be explained by corresponding coronary artery occlusions. The typical pattern of LV RWMA that is described includes apical hypokinesia, akinesia, or dyskinesia which gives the apical ballooning shape with relative basal hyperkinesis. Other forms of stress CM include “reverse Takotsubo” or “atypical Takotsubo” which are characterized by mid-ventricular or basal hypokinesis (6). Focal stress CM is another rare form described by its focal LV RWMA which makes it very hard to distinguish from myocarditis or myocardial infarction (7). Clinically, stress CM often presents with ST-segment changes on electrocardiography (ECG), elevation in troponins, a significant increase in serum natriuretic peptides, and characteristic imaging findings. However, coronary angiography remains an essential diagnostic modality to definitively exclude ischemic etiologies for RWMA (1).
Multiple diagnostic criteria have been proposed: the most commonly used are the Heart Failure Association of the European Society of Cardiology diagnostic criteria for Takotsubo Syndrome (8), the International Takotsubo Diagnostic Criteria (InterTAK) (9), and the Revised Mayo Clinic Criteria (10). The criteria are summarized in Table 1. The Japanese Society of Echocardiography (JSE) and the European Association of Cardiovascular Imaging (EACVI) published a joint consensus document in 2020 providing a comprehensive review of the various cardiovascular imaging modalities that can be utilized in diagnosing and prognosticating stress CM. They incorporated the InterTAK criteria into a simplified diagnostic algorithm that helps evaluate chest pain and/or dyspnea and rule out several cardiac causes before getting to stress CM (11).
Pathophysiology
The exact pathophysiology leading to stress CM still remains unknown. There is a known neuro-cardiovascular link that may contribute to stress CM, but recently neuroimaging studies have given us more insight (12). The central autonomic nervous system (CANS) is vital for cardiovascular regulation. Prior to adopting the terms Takotsubo and stress CM, clinicians have identified instances of transient systolic dysfunction as well as dynamic EKG changes in relation to intracranial pathology such as stroke or hemorrhages (12). This phenomenon has been later described as “neurogenic stunning” and was also seen in patients undergoing electroconvulsive therapy (ECT) (13). As described by de Chazal et al. in a recent review, in the acute phase, there is increased blood flow in the hippocampus, brainstem, and basal ganglia with return to normal upon resolution of the syndrome (1). However, it is still unknown why some emotional stressors cause myocardial dysfunction while other stressors do not. Stress induces activation of complex neocortical limbic pathways which leads to the activation of brainstem noradrenergic neurons and stress related neuropeptides (NPY) produced by the arcuate nucleus of the hypothalamus. Acute stressors induce brain activation, which increases the bioavailability of norepinephrine, cortisol, and NPY. Both circulating epinephrine and norepinephrine released from the medulla of the adrenal glands or locally from sympathetic nerve terminals are known to be significantly increased during the acute phase of stress CM. The catecholamine and NPY surge can lead to the apical ballooning physiology through multiple mechanisms including direct catecholamine toxicity, epicardial, and microvascular coronary spasm or vasoconstriction, and increased cardiac afterload (14). Experimental data have shown that β2-adrenoceptors are more frequently expressed in an apical than the basal segment of the LV, while a reverse distribution is present for norepinephrine β1-adrenoceptors and sympathetic and sympathetic nerve terminals of the neuro-cardiac axis, which are dense at the base but not the apex (15). Previously, it was widely thought that the catecholamine surge is solely responsible for the cardiomyopathy; however, it is increasingly recognized that alternate mechanisms may play more of a role. Catecholamine levels often increase in response to cardiac dysfunction and hypotension. It is not yet clear how norepinephrine and NPY contribute mechanistically to stress CM. One hypothesis maintains that the cardio-depressant effects of catecholamines may lead to supply demand mismatch at the level of the myocardium and eventually myocardial stunning (1). Thus, it is plausible that in individuals with higher levels of NPY/norepinephrine, an intense stressor may predispose them to develop stress CM (1).
Risk Factors
Estrogen deficiency in post-menopausal women appears to be one of the strongest risk factors associated with stress CM. This is often superimposed on a background of anxiety or panic disorder (16). Diabetes has also been described as a risk factor, as 10–25% of all the patients presenting with stress CM have concomitant diabetes (17). One of the non-cardiac risk factors frequently associated with stress CM is asthma, particularly after the use of medical interventions such as beta-2 agonists, racemic epinephrine, and intubation. It is not fully understood whether it is the asthma itself or the treatment that triggers the syndrome (18). Marijuana use is also associated with stress CM with a subsequent higher mortality (19). Prior studies suggest that cannabinoids induce NPY expression from the arcuate nucleus of the hypothalamus which can lead to neurogenic myocardial stunning (20). Finally, as we continue to assimilate further data on the COVID-19 pandemic, there is increasing evidence of acute or chronic cardiac injury in the setting of a COVID-19 infection. Although stress CM has been reported in the setting of COVID-19, other pathologies as a cause of cardiac injury are more common including supply/demand mismatch ischemia, microvascular thromboses, myocarditis, or cytokine storm from a hyperinflammatory state (21–24).
Echocardiographic Findings
Left Ventricular Wall Motion and Systolic Function
In the acute phase of stress CM, an echocardiographic assessment is an essential first step toward the diagnosis. The typical echocardiographic findings are characterized by the presence of a large area of dysfunctional myocardium which extends beyond one coronary artery territory. In classic stress CM, symmetrical wall motion abnormalities are seen with akinesis or dyskinesis of the apical and mid-ventricular segments of the anterior, lateral, septal, and inferior walls with hyperdynamic function of the basal segments (25) (Figure 1). Other variants such as “mid-ventricular” or “inverted” stress cardiomyopathy can also be seen. The mid-ventricular variant is characterized by akinesis of the mid-ventricular segments with mild hypokinesis or normal contraction of the apical segments and hypercontractility of the base. The inverted variant has two different forms: the first manifests as preserved apical function and severe hypokinesis of the rest of the walls while the second form is characterized by hypokinesis only in the basal segments (often called “reverse Takotsubo”) (25).
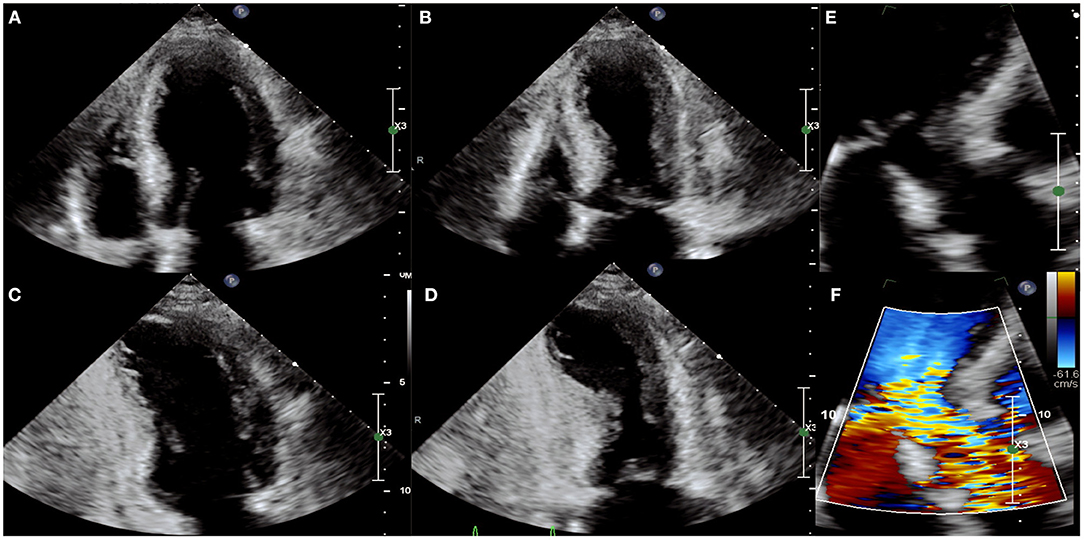
Figure 1. Echocardiographic images of a person with stress cardiomyopathy with left ventricular outflow track obstruction (LVOT) and systolic anterior motion (SAM) of the mitral valve. The apical-4-chamber view in diastole (A) and systole (B) and 2-chamber view in diastole (C) and systole (D) showing classic apical ballooning with akinesis and hyperdynamic basal segments. (E,F) Illustrate SAM and turbulence across the LVOT indicative of LVOT obstruction.
Echocardiography also demonstrates reduced LV ejection fraction (EF) with variable severity and in most cases recovers as the myocardial stunning resolves (2). The degree of the EF reduction varies depending on the level of myocardial impairment which can often correspond with pre-existing comorbidities, sex, and age. While the degree of myocardial impairment can sometimes be severe, this is not typically reflected by the degree of elevation of cardiac biomarkers, which may be only modestly elevated. The discrepancy between troponin levels and RWMAs can help clinicians to differentiate between ACS and stress CM. More recently, the product of Troponin I level and echocardiographic LVEF has been utilized as an index to differentiate stress CM from ST elevation myocardial infarction (STEMI). A value ≥250 is indicative of a STEMI, with a sensitivity and specificity of 95 and 87%, respectively (26).
Left ventricular EF has been found to be an independent predictor of major cardiovascular complications and can help identify stress CM patients at higher risk particularly in those aged >75 years (27). Elderly patients demonstrate significantly lower LV systolic function compared to younger patients (defined as <75 years) (25). If RWMAs persist, then it is reasonable to evaluate with a cardiac MRI to rule out necrosis or other pathologies.
Assessment of LV longitudinal strain may have an important prognostic value in the acute phases of stress CM. Decreased global longitudinal strain (GLS) in patients with stress CM was found to correlate with higher in-hospital mortality (28).
LV Diastolic Function
LV diastolic dysfunction is common in stress CM (29). Global diastolic dysfunction has been observed in some patients in the early phases of stress CM which is evident by impaired left ventricular relaxation and increased E/e' ratio, a non-invasive marker of LV filling pressures (30, 31). In the Takotsubo Italian Network (TIN) registry, E/e' ratios were higher in patients who went on to develop acute decompensated heart failure and was found to be an independent predictor of in-hospital mortality (32). Of all the complications associated with stress CM, acute decompensated heart failure is the most common early complication; thus, an early assessment of the E/e' ratio may allow for early identification of patients at higher risk of decompensation and could serve as a useful tool to guide management. As E/e' ratio elevations can be transient, the ratio itself could be used as a marker for functional recovery when followed serially across the course of the syndrome.
Mitral Regurgitation (MR)
The mechanism of MR in stress CM is not completely understood. Systolic anterior motion of the anterior mitral leaflet (SAM) has been reported in 40–50% of patients with stress CM and may be associated with significant MR (33) (Figure 1). MR in stress CM is typically described in cases where reduction in LVEF is severe and LV volume is high and likely represents functional MR due to papillary muscle displacement and tethering of the mitral leaflets in the setting of a dilated and dysfunctional LV (33). In the TIN registry, the presence of MR appeared to be a powerful prognostic marker associated with cardiogenic shock and in-hospital morbidity and mortality (32).
LV Outflow Tract Obstruction (LVOTO)
LVOTO, diagnosed by echocardiogram, may result from basal hypercontractility with or without systolic anterior motion of the anterior mitral leaflet causing dynamic obstruction of the LV outflow tract (LVOT), and is seen in as many as 12.5–25% of cases (32, 34). Significant LVOTO is described as a peak instantaneous LVOT gradient ≥ 30 mmHg. The LVOT gradient has important clinical and therapeutic implications in patients with stress CM; in this cohort, inotropic agents and diuretics should be avoided as the basal hypercontractility induced by catecholamines and the reduction in preload can lead to an exacerbation in gradient with subsequent hemodynamic collapse and cardiogenic shock. LVOTO is associated with increased LV afterload and systolic wall tension which may lead to subendocardial ischemia. In this setting, beta blockade may be beneficial in reducing inotropy and reduced myocardial demand. Alpha-1 agonism with phenylephrine can increase systemic vascular resistance and thus help reduce the effect of dynamic LVOT obstruction, ultimately reducing LV afterload and wall stress (25). Patients with severe LVOTO and stress CM are at higher risk for free wall rupture and life-threatening arrhythmias.
Right Ventricular (RV) Function
Electrocardiographic signs of RV strain warrant special attention with regards to evaluation of RV function in stress CM.
The prevalence of RV involvement has been reported to be around 14% in one study; however, this may be an underestimation due to difficulty imaging the RV (25). In patients with biventricular ballooning, RV contraction often mirrors that of the LV (35). The pattern described is the opposite of the well-described McConnell's sign seen in massive pulmonary emboli which manifests itself as RV apical hyperkinesis and basal and mid hypokinesis to akinesis. The pattern of RV involvement was eventually called “reverse McConnell's sign” (36). There is conflicting evidence on whether RV involvement predicts worse outcomes. In the TIN registry, RV involvement was more prevalent in patients with major complications. No significant correlation has been found between tricuspid annular systolic excursion (TAPSE) and major adverse events (32).
Contrast Echocardiography
Per the updated American Society of Echocardiography guidelines (2018) and European Association of Cardiovascular Imaging (2017) guidelines, contrast echocardiography is a recommended tool to evaluate for the presence of an LV thrombus in situations where it is not detected on non-contrast echocardiography (37). However, if contrast echocardiography fails to detect a thrombus in cases with a high clinical suspicion for a thrombus, cardiac MRI would provide a higher sensitivity and specificity at detecting LV thrombi.
Cardiac Magnetic Resonance Imaging (CMR)
Cine CMR Sequence
Cine CMR sequences allow for more detailed assessment of LV and RV function, ventricular wall motion abnormalities, and possible complications of stress CM (38, 39). While the “apical ballooning” pattern of wall motion accounts for around 75–80% of patients, stress CM can also present in the form of other less common variants, namely the mid-ventricular variant or the inverted variant which are discussed earlier (40, 41) (Figure 2). The cine sequence can also be used to demonstrate LVOTO with or without systolic anterior motion of the mitral valve leaflet as well as functional mitral regurgitation (39). These complications can be further assessed with phase contrast velocity imaging in order to generate quantitative values such as LV outflow tract gradient.
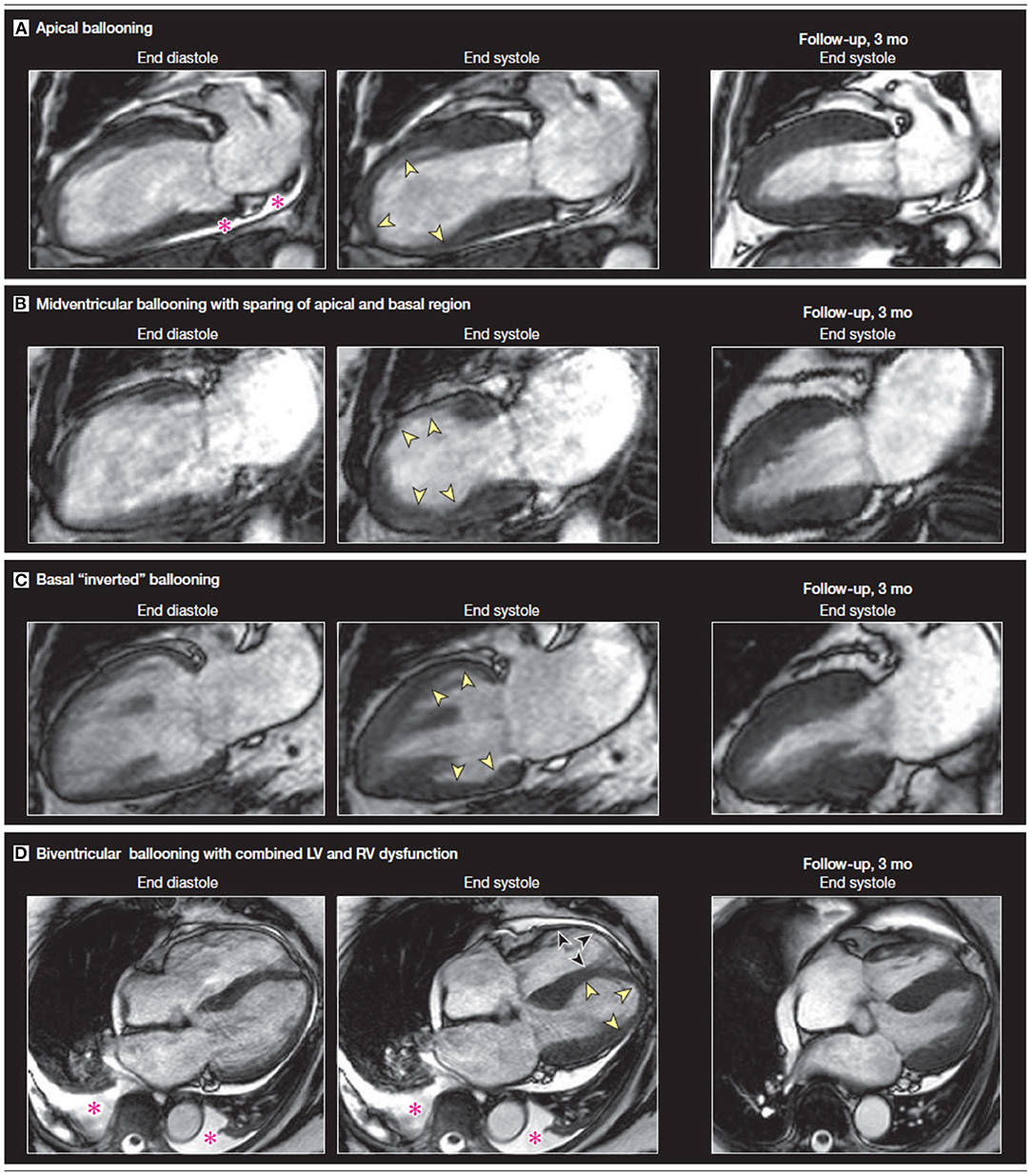
Figure 2. A comparison of vertical long axis CMR cine sequence images on diagnosis and at 3-month follow-up showing a recovery of wall motion abnormalities. The four sets of images display four different variants associated with stress CM. The pink asterisk denotes a pericardial effusion. Yellow arrows indicate apical akinesis while the black arrows indicate RV apical akinesis in the biventricular ballooning variant. Figure adapted from Figure 2 on Clinical Characteristics and cardiovascular magnetic resonance findings in stress (Takotsubo) cardiomyopathy by Eitel et al. (41).
Additionally, CMR allows for more quantitative assessment of RV function. RV involvement has been assessed by CMR in multiple studies: patients with RV dysfunction were older, had with longer hospital stays, had more frequent preceding stressful events, had significantly more pleural effusions, and had a lower LV EF compared to those that did not have RV involvement (41, 42).
Recently, CMR post-processing techniques called feature/tissue tracking CMR (FT-CMR) have been developed that enable strain analysis for the quantification of myocardial deformation. A study by Stiermaier et al. evaluated 141 patients with stress CM and found that reduced global circumferential (GCS) and global longitudinal strain (GLS) were associated with the apical ballooning variant while reduced global radial strain (GRS) was associated with the basal ballooning variant (43). Additionally, the study posited that GLS may be useful as a prognostic indicator as LV strain measures worse than −14.75% were associated with adverse outcomes (43).
Myocardial Edema
In the setting of myocardial injury, tissue inflammation may increase resulting in localized myocardial edema (41). Changes in the tissue water content can be evaluated via a CMR T2-weighted imaging protocol (Figure 3) which allows for assessment of the distribution of myocardial edema and can be helpful in differentiating between cardiac pathologies such as stress CM, ACS, and myocarditis.
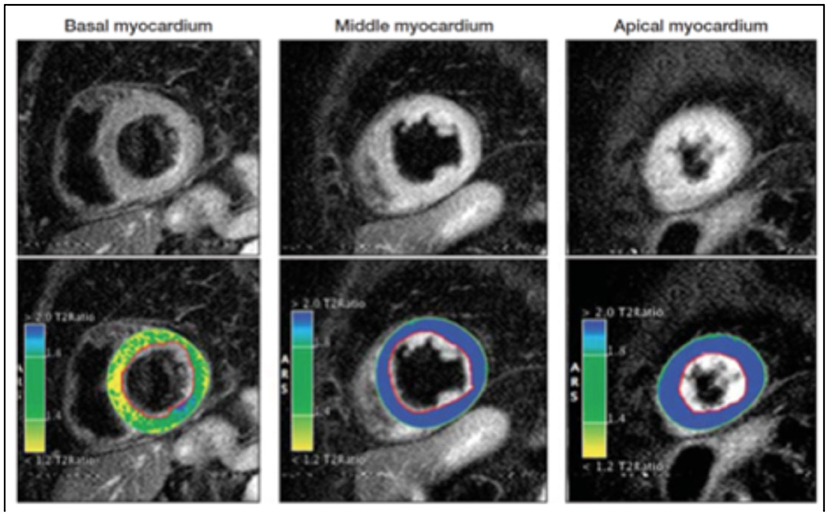
Figure 3. T2-weighted sequences demonstrating variability in signal intensity based on the affected myocardial regions. The color coding resembles varying signal intensity ratios between myocardium and skeletal tissue with blue indicating a ratio ≥ 1.9 (indicating edema) while yellow/green indicates a ratio <1.9, indicative of normal myocardium. These images illustrate predominant myocardial edema in the mid-ventricular and apical segments. Adapted from Clinical Characteristics and cardiovascular magnetic resonance findings in stress (Takotsubo) cardiomyopathy by Eitel et al. (41).
By employing the black blood T2-weighted (fast spin echo) triple-inversion recovery (IR) sequence, fat, and flowing blood can be suppressed allowing the contrast signal to differentiate between normal myocardium and edematous tissue (44). This process can be quantified by calculating the ratio of the signal intensity (SI) between the myocardium and skeletal tissue, with a cut-off value of over 1.9 considered significant for edema.
The pattern of edema in stress CM classically overlaps with the dysfunctional ventricular regions noted on the cine CMR sequence and does not correspond to territories served by epicardial vessels that would generally be seen in ACS (41). The signal distribution tends to be transmural and circumferential over the affected area, unlike in myocarditis where it tends to be more diffuse and heterogenous with a propensity to have higher signal intensity in the sub-epicardial or mid-myocardial tissue.
It is important to note, however, that T2-weighted triple IR can be prone to artifacts from various factors such as arrhythmias, blood pooling, or breathing motion artifact. Therefore, studies investigating techniques that use motion correction algorithms such as extracellular volume (ECV) mapping and parametric T1 or T2 mapping have shown to improve diagnostic accuracy and provide further quantitative evidence in evaluating myocardial edema (15, 45–47). In patients with myocardial infarction, the intensity of T2 signaling tends to take months or even years to decline in the infarcted and surrounding tissue depending on the severity of the injury. In stress cardiomyopathy, there has been variable data with some studies showing a normalization of signaling as the myocardial function returns to normal, while other studies have shown a slower return to baseline, despite recovery in LV myocardial function (15, 45–48). These novel techniques could prove to be viable alternatives to evaluate myocardial inflammation and characterize disease severity without using gadolinium contrast and warrant further studies with larger patient cohorts.
Although the pathophysiology of myocardial inflammation in stress CM is not well-known, increased myocardial edema on imaging may indicate a direct inflammatory process from the syndrome, a secondary effect stemming from elevated sympathetic drive, or microvascular ischemia associated with stress CM (41, 48, 49).
Late Gadolinium Enhancement (LGE)
The use of gadolinium-mediated myocardial enhancement has been used to delineate areas of myocardial scarring or replacement fibrosis stemming from myocardial injury and inflammation. Conventionally, when using LGE to delineate areas of fibrosis in patients with a history of myocardial infarction or myocarditis, a threshold of 5 standard deviations (SDs) above the signal intensity seen in remote, unaffected tissue is used to define significant enhancement. In areas affected by myocardial infarction, the LGE pattern is generally predominant in the subendocardial tissue and can spread transmurally depending on the extent of injury, while in myocarditis the distribution tends to dominate in the sub-epicardial region (updated Lake Louise consensus criteria) (50). Previously, it was thought that the absence of LGE was a pathognomonic feature of stress CM that differentiated it from other pathologies such as myocardial infarctions or myocarditis; however, several studies have now shown that stress CM does present with a certain degree of LGE that is usually of reduced intensity compared to other conditions that cause fibrosis (41, 51, 52). Eitel et al. showed no evidence of LGE in any of the 239 patients assessed in one study, while lowering the threshold to 3 SD showed positive findings in 22 patients (9%) (41). These patients had higher troponin levels at presentation compared to those that had negative LGE but there were no differences in the LV ejection fraction, end-diastolic volume, or end-systolic volume. Another study similarly revealed that changing the SD threshold for LGE may yield different results (53).
There are various theories as to what causes the delayed enhancement, with some studies suggesting a delayed washout of contrast due to the presence of myocardial inflammation and interstitial edema. Other studies have used immune-histological evidence to show that affected areas in stress CM have increased collagen-1 which could be indicative of a fibrotic process (52, 53). Perhaps the low level of LGE reflects the reversibility of stress CM and near or complete resolution, which is an essential diagnostic component of this condition. In ischemic cardiomyopathies or non-ischemic cardiomyopathies such as sarcoidosis, higher degrees of LGE are often associated with poorer prognoses due to irreversible myocardial injury (54). In one study, stress CM patients with LGE had a higher frequency of cardiogenic shock and had a slower recovery of wall motion compared to those that did not display LGE (53). Therefore, extent of LGE on CMR may represent a useful prognostic marker in stress CM.
Early Gadolinium Enhancement (EGE)
Within 1–3 min of gadolinium administration, EGE can be accessed via a high T1 inversion time. This technique is essential for the identification of LV thrombus. Thrombi are characterized by having no gadolinium uptake and are seen as a hypodense mass (almost black) in contrast to the gray myocardium. Important CMR parameters are summarized in Table 2.
Nuclear Imaging
Recent studies have reported a role for nuclear imaging findings in the diagnosis of stress CM. Single photon emission computed tomography can assess cardiac stunning using 201 Thallium or 99m Technetium-labeled radiopharmaceuticals and 123I-metalodobenzyl-guanidine (I-123 MIBG) (55). In addition, 18F-fluorodeoxyglucose (FDG) positron emission tomography can be used to detect myocardial glucose metabolism (56). In the acute and sub-acute phases of stress CM, the affected segments of the myocardium show defects in FDG and MIBG despite normal to slightly reduced perfusion (57). MIBG is a norepinephrine analog and uses the same mechanism of norepinephrine in the uptake and storage in presynaptic vesicles. After adrenergic stimulation, MIBG is released in the synaptic cleft but is not metabolized by monoamine oxidase (MOA) and catechol-O-methyltransferase (COMT) enzymes (58). MIBG is also labeled with iodine-123 (123I) as a radiotracer due to its suitability for imaging. With such properties, it is possible to evaluate the cardiac uptake of the MIBG and its distribution (59). Visually and quantitively, two parameters are analyzed: heart to mediastinal ratio (H/MR) and myocardial washout rate (WR) (59).
The evaluation of stress CM by I-123 MIBG scintigraphy demonstrates a defect in MIBG uptake at the level of the apex. Semi-quantitative measures also demonstrate a reduction in H/MR and an increase in washout (59). The use of nuclear imaging for the diagnosis of stress CM is promising but given the presence of alternative more accessible modalities, it is not the first line imaging modality for diagnosis.
Cardiac Multi-Detector Computed Tomography
Cardiac multi-detector computed tomography (MDCT) is a comprehensive imaging modality that is used to assess cardiac function as well as coronary artery lesions (55). MDCT can provide valuable information regarding RWMA and can immediately rule out a coronary artery lesion with a high negative predictive value (60). In clinical practice, excluding the possibility of coronary obstruction or acute plaque rupture with MDCT still remains a challenge (55). Cardiac MDCT may be a promising imaging modality in the evaluation of suspected stress-induced cardiomyopathy. Cardiac MDCT may in particular be an appealing imaging modality when there is a clinical need to evaluate the coronary arteries at the same time or when there is a contraindication to MRI.
Complications
Although the hallmark of stress CM is that myocardial function returns to normal within weeks, many patients endure complications related directly to stress CM or comorbid medical conditions and can have significant morbidity and mortality in the inpatient setting (Figure 4).
Acute Heart Failure
Systolic heart failure is the most common complication encountered by these patients in the acute setting, with an incidence ranging between 3 and 46% (3, 32, 61, 62). This can occur as a result of depressed LV systolic function or secondary to other associated complications such as LVOTO or functional mitral regurgitation. Some patients have progression of heart failure to cardiogenic shock requiring inotropic or vasopressor support or even mechanical support via intra-aortic balloon pumps (IABP) or extracorporeal membrane oxygenation (ECMO), with a very small proportion of cases resulting in death. Biventricular involvement has a tendency to occur in the elderly population, is associated with lower LV ejection fraction, and is an independent predictor of adverse cardiovascular outcomes (41, 63). These patients tend to have longer and complicated hospital stays and have shown to have higher rates of in-hospital and long-term morbidity and mortality (64).
LV Thrombus and Systemic Emboli
Formation of an LV thrombus is a relatively rare but significant complication associated with stress CM, particularly with the apical ballooning variant. Studies have shown an incidence between 2 and 9.2% of patients (65–68). In these cases, likelihood of clot formation is increased due to blood stasis from apical hypokinesis/akinesis increased stasis; furthermore, the adrenergic surge that is often thought to be a contributing mechanism behind stress CM could potentially cause endocardial damage that may initiate a thrombotic process. Stress CM patients with LV thrombus are at increased risk for systemic emboli including cerebrovascular embolic events, which can occur in around 17–25% of cases (66, 68).
Arrhythmias
As cardiac arrhythmias are important potential complications of stress CM that could arise in the acute setting, patients need to be monitored closely via telemetry and serial ECGs in order to evaluate for abnormal rhythms and a prolonged QT interval. Atrial fibrillation can occur in ~4.5–25% of patients and is the most common arrhythmia seen in stress CM patients (69–71). Rarely, patients may develop more dangerous cardiac arrhythmias such as ventricular tachycardia or torsades de pointes, especially if a prolonged QT interval >500 ms is present (72, 73). In one study, men were more likely to have cardiac arrhythmias and a higher incidence of cardiac arrest compared to women (69).
Treatment
Acute Heart Failure and Cardiogenic Shock
To date, there have been no large randomized controlled trials investigating various therapies that are commonly used for the treatment of acute heart failure in stress CM. The primary goal of therapy is to address any acute complications that arise, prophylactically treat to minimize risks of certain complications such as thromboembolism, and closely follow the patient throughout the course of the disease until full recovery and/or normalization of cardiac function. In determining the course of therapy, it is important to delineate the pattern of cardiomyopathy and identify certain features such as the presence of LVOTO that may require adjustment in medication regimen (Figure 5).
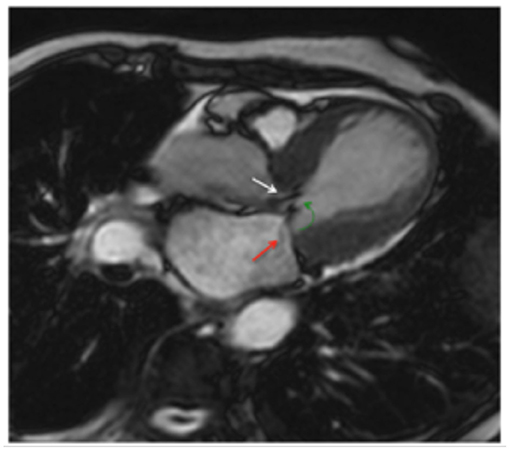
Figure 5. Cine CMR horizontal long-axis view illustrating the systolic “jet” seen in LVOT (white arrow) with concurrent systolic anterior motion of the mitral leaflets (green arrow) and functional mitral regurgitation (red arrow). Adapted from Figure 6 in Plácido et al. (39).
In a patient with normal cardiac output, the standard of treatment involves use of diuretics in order to achieve euvolemia and afterload reduction with arterial vasodilators (e.g., ACE-inhibiters, angiotensin-receptor blockers, angiotensin receptor-neprilysin inhibitor, hydralazine) (1, 74). In patients who are hemodynamically stable with LVOTO, the use of beta-blockers has a negative inotropic effect on basal hypercontractility and reduces the obstruction. In the case of LVOTO, the use of nitrates in order to lower filling pressures may have negative effects by worsening the LVOT gradient and should be avoided (74).
When treating a patient with cardiogenic shock, the approach to therapy is heavily predicated on the presence or absence of LVOT obstruction. In the absence of LVOT obstruction, inotropic agents such as dobutamine or milrinone can be used to augment cardiac output. Vasopressors such as norepinephrine or vasopressin can be utilized as a second-line agent. There have been retrospective studies and case series suggesting the safety and feasibility of using levosimendan (a Ca2+ sensitizer) as a potential alternative inotropic agent (75, 76); however, clinical trials are needed to further study this agent before adoption in clinical practice. Several case reports have detailed complex stress CM cases requiring mechanical circulatory support via IABP or percutaneous left ventricular assist devices (such as Impella) until recovery (77, 78).
If LVOTO is present independent of concurrent mitral regurgitation, the use of inotropic support can increase basal hypercontractility and worsen the LVOTO, potentially worsening cardiogenic shock. These patients benefit from measures such as administration of IV fluids and/or the use of low-dose beta-adrenergic antagonists such as metoprolol or esmolol for the purpose of negative inotropy on the hyperdynamic basal myocardium which can reduce the LVOT obstruction. Extreme caution must be practiced with use of beta blockers, however, as despite their benefit in reducing LVOTO they can be potentially detrimental by reducing cardiac output further due to negative inotropy. Other therapeutic agents include vasopressors such as phenylephrine or vasopressin which can be administered to increase systemic vascular resistance and thereby reduce obstruction at the LVOT (1). In refractory cases, ECMO may be used to provide support (79).
Much like with acute management of stress CM, chronic management of stress CM has limited evidence with no official guidelines in place to date. There have been several studies ranging from case series to retrospective studies to meta-analyses investigating various heart failure drugs. Some studies favor ACE-inhibitors and angiotensin receptor blockers over beta blockers in terms of potential long-term mortality benefit while others have shown no significant findings (40, 80, 81). The general approach to chronic care of stress CM patients has been to initiate guideline-directed medical therapy as indicated in the care of the general cardiomyopathy patient. In patients with concurrent coronary artery disease, guideline-directed use of aspirin and statin therapies is likely beneficial as well (74).
Thromboembolism
In the 2013 ACCF/AHA guidelines for the management of STEMI, there is a Class IIb recommendation to consider prophylactic anticoagulation in patients with anterior wall myocardial infarctions resulting in hypokinetic or akinetic wall motion abnormalities that could pose a potential high risk of LV thrombus formation (82). In a review article by Heckle et al. (66), data compiled from four studies showed a prevalence of 9.2% for thromboembolic events in patients with stress CM. The study posited that an event rate of 9.2% would roughly equate to a score of at least 5 on the CHA2DS2-Vasc scoring system used to determine the need for anticoagulation in patients with atrial fibrillation; therefore, this could theoretically be extrapolated to initiate prophylactic anticoagulation in patients with stress CM (66). To date, there have not been any randomized clinical trials investigating the efficacy of anticoagulation and duration of therapy as a therapeutic or even prophylactic approach to patients with a LV thrombus in the setting of stress CM. Prior retrospective studies have suggested the use of systemic anticoagulation for at least 3 months in patients with a confirmed LV thrombus.
Prognosis
A retrospective, international, multi-center study by Templin et al., utilized a database called the International Takotsubo Registry to identify risk factors for stress CM as well as and predictors of clinical outcomes in these patients (40). In the study, 21.8% of patients had in-hospital complications including death, which was similar when compared to those with ACS. A multi-variate analysis in this study revealed that physical triggers, acute psychiatric disorders (including anxiety, adjustment disorder, and severe stress), or neurologic disorders (including stroke/TIA, seizures, and headache disorders); a first troponin level >10 times the upper limit of normal; and an LVEF of <45% were all noted to be predictors of higher incidence of in-hospital complications and death. Conversely, older age (>70 years) and the presence of an emotional trigger were associated with a lower incidence of in-hospital complications and death; however, other studies have revealed higher rates of complications in older patients (27, 83).
In-hospital mortality rates for stress CM have ranged between 2 and 5% (32, 83–85). A study in Japan by Isogai et al. (86) compared patients with stress CM due to an out-of-hospital causative factor with those who developed stress CM during hospital admission and found that in-hospital stress CM patients had higher a proportions of co-morbidities along with higher in-hospital mortality (17.9 vs. 5.4%, p < 0.001). Presenting in an unstable condition (i.e., post-cardiac arrest or shock requiring pressor support) or having other non-cardiac acute co-morbidities were also associated with a higher risk of in-hospital mortality. In analyzing long-term outcomes, some studies have shown a higher mortality in patients with stress CM compared to a general age and gender-matched population while others have shown no differences in the long term (87, 88).
Data on recurrence have been limited but current evidence reports a recurrence rate of around 2–4% per year and up to as high as 20% in 10 years (40, 88, 89). There are reports of recurrence presenting with a different anatomical variant of stress CM. Some patients may continue to experience symptoms such as fatigue, shortness of breath, chest pain, and exercise intolerance even with recovery of LVEF (1). There is also evidence that patients can experience psychiatric consequences such as post-traumatic stress disorder as a long-term complication of stress CM (90).
Conclusion
As featured comprehensively in this review, cardiovascular imaging plays a vital role in stress CM and offers many facets of benefits ranging from the initial diagnosis to long-term outcomes. Echocardiography and certain sequences in CMR enable us to differentiate between variants of stress CM, characterize severity of left ventricular dysfunction and identify prognostic features such as LVOTO, mitral regurgitation and RV involvement that often drive our course of therapy. Additionally, several CMR sequences such as feature/tissue tracking, T1/T2 mapping are useful in providing qualitative and quantitative evidence of myocardial injury and degree of inflammation on a cellular and tissue level that can then be translated into prognostic information. As we begin to understand that stress CM is not a benign condition and can often have serious complications in the acute and chronic setting similar to more common cardiac pathologies such as ACS, there is a strong call for further large scale trials in order to investigate and refine our diagnostic and therapeutic approach to this disease.
Author Contributions
All authors listed have made a substantial, direct, and intellectual contribution to the work and approved it for publication.
Conflict of Interest
The authors declare that the research was conducted in the absence of any commercial or financial relationships that could be construed as a potential conflict of interest.
Publisher's Note
All claims expressed in this article are solely those of the authors and do not necessarily represent those of their affiliated organizations, or those of the publisher, the editors and the reviewers. Any product that may be evaluated in this article, or claim that may be made by its manufacturer, is not guaranteed or endorsed by the publisher.
References
1. Medina de Chazal H, Del Buono MG, Keyser-Marcus L, Ma L, Moeller FG, Berrocal D, et al. Stress Cardiomyopathy diagnosis and treatment: JACC state-of-the-art review. J Am Coll Cardiol. (2018) 72:1955–71. doi: 10.1016/j.jacc.2018.07.072
2. Kurisu S, Sato H, Kawagoe T, Ishihara M, Shimatani Y, Nishioka K, et al. Tako-tsubo-like left ventricular dysfunction with ST-segment elevation: a novel cardiac syndrome mimicking acute myocardial infarction. Am Heart J. (2002) 143:448–55. doi: 10.1067/mhj.2002.120403
3. Gianni M, Dentali F, Grandi AM, Sumner G, Hiralal R, Lonn E. Apical ballooning syndrome or takotsubo cardiomyopathy: a systematic review. Eur Heart J. (2006) 27:1523–9. doi: 10.1093/eurheartj/ehl032
4. Kurowski V, Kaiser A, Von Hof K, Killermann DP, Mayer B, Hartmann F, et al. Apical and midventricular transient left ventricular dysfunction syndrome (Tako-Tsubo cardiomyopathy): frequency, mechanisms, and prognosis. Chest. (2007) 132:809–16. doi: 10.1378/chest.07-0608
5. Wittstein IS, Thiemann DR, Lima JAC, Baughman KL, Schulman SP, Gerstenblith G, et al. Neurohumoral features of myocardial stunning due to sudden emotional stress. N Engl J Med. (2005) 352:539–48. doi: 10.1056/NEJMoa043046
6. Tsuchihashi K, Ueshima K, Uchida T, Oh-mura N, Kimura K, Owa M, et al. Transient left ventricular apical ballooning without coronary artery stenosis: a novel heart syndrome mimicking acute myocardial infarction. angina pectoris-myocardial infarction investigations in Japan. J Am Coll Cardiol. (2001) 38:11–8. doi: 10.1016/S0735-1097(01)01316-X
7. Tini G, Limite LR, Arcari L, Cacciotti L, Russo D, Sclafani M, et al. A systematic review on focal takotsubo syndrome: a not-so-small matter. Heart Fail Rev. (2020). doi: 10.1007/s10741-020-09988-y. [Epub ahead of print].
8. Lyon AR, Bossone E, Schneider B, Sechtem U, Citro R, Underwood SR, et al. Current state of knowledge on Takotsubo syndrome: a position statement from the taskforce on takotsubo syndrome of the heart failure association of the european society of cardiology. Eur J Heart Fail. (2016) 18:8–27. doi: 10.1002/ejhf.424
9. Ghadri JR, Cammann VL, Jurisic S, Seifert B, Napp LC, Diekmann J, et al. A novel clinical score (InterTAK Diagnostic Score) to differentiate takotsubo syndrome from acute coronary syndrome: results from the International Takotsubo Registry. Eur J Heart Fail. (2017) 19:1036–42. doi: 10.1002/ejhf.683
10. Madhavan M, Prasad A. Proposed Mayo Clinic criteria for the diagnosis of Tako-Tsubo cardiomyopathy and long-term prognosis. Herz. (2010) 35:240–3. doi: 10.1007/s00059-010-3339-x
11. Citro R, Okura H, Ghadri JR, Izumi C, Meimoun P, Izumo M, et al. Multimodality imaging in takotsubo syndrome: a joint consensus document of the European Association of Cardiovascular Imaging (EACVI) and the Japanese Society of Echocardiography (JSE). Eur Hear J. (2020) 21:1184–207. doi: 10.1093/ehjci/jeaa149
12. Pollick C, Cujec B, Parker S, Tator C. Left ventricular wall motion abnormalities in subarachnoid hemorrhage: an echocardiographic study. J Am Coll Cardiol. (1988) 12:600–5. doi: 10.1016/S0735-1097(88)80044-5
13. Biso S, Wongrakpanich S, Agrawal A, Yadlapati S, Kishlyansky M, Figueredo V. A review of neurogenic stunned myocardium. Nalivaiko E, ed. Cardiovasc Psychiatry Neurol. (2017) 2017:5842182. doi: 10.1155/2017/5842182
14. Pelliccia F, Kaski JC, Crea F, Camici PG. Pathophysiology of takotsubo syndrome. Circulation. (2017) 135:2426–41. doi: 10.1161/CIRCULATIONAHA.116.027121
15. Haaf P, Garg P, Messroghli DR, Broadbent DA, Greenwood JP, Plein S. Cardiac T1 Mapping and Extracellular Volume (ECV) in clinical practice: a comprehensive review. J Cardiovasc Magn Reson. (2016) 18:89. doi: 10.1186/s12968-016-0308-4
16. Sharkey SW, Windenburg DC, Lesser JR, Maron MS, Hauser RG, Lesser JN, et al. Natural history and expansive clinical profile of stress (Tako-Tsubo) cardiomyopathy. J Am Coll Cardiol. (2010) 55:333–41. doi: 10.1016/j.jacc.2009.08.057
17. Stiermaier T, Santoro F, El-Battrawy I, Möller C, Graf T, Novo G, et al. Prevalence and prognostic impact of diabetes in Takotsubo syndrome: insights from the international, multicenter GEIST registry. Diabetes Care. (2018) 41:1084–8. doi: 10.2337/dc17-2609
18. Pontillo D, Patruno N, Stefanoni R. The tako-tsubo syndrome and bronchial asthma: the chicken or the egg dilemma. J Cardiovasc Med. (2011) 12:149–50. doi: 10.2459/JCM.0b013e32833cdbb0
19. Pacher P, Bátkai S, Kunos G. Cardiovascular pharmacology of cannabinoids. Handb Exp Pharmacol. (2005) 168:599–625. doi: 10.1007/3-540-26573-2_20
20. Bakkali-Kassemi L, El Ouezzani S, Magoul R, Merroun I, Lopez-Jurado M, Errami M. Effects of cannabinoids on neuropeptide Y and β-endorphin expression in the rat hypothalamic arcuate nucleus. Br J Nutr. (2011) 105:654–60. doi: 10.1017/S0007114510004095
21. Siripanthong B, Nazarian S, Muser D, Deo R, Santangeli P, Khanji MY, et al. Recognizing COVID-19–related myocarditis: the possible pathophysiology and proposed guideline for diagnosis and management. Hear Rhythm. (2020) 17:1463. doi: 10.1016/j.hrthm.2020.05.001
22. Chung MK, Zidar DA, Bristow MR, Cameron SJ, Chan T, Harding CV, et al. COVID-19 and cardiovascular disease. Circ Res. (2021) 128:1214–36. doi: 10.1161/CIRCRESAHA.121.317997
23. Jabri A, Kalra A, Kumar A, Alameh A, Adroja S, Bashir H, et al. Incidence of stress cardiomyopathy during the coronavirus disease 2019 pandemic. JAMA Netw Open. (2020) 3:1–7. doi: 10.1001/jamanetworkopen.2020.14780
24. Minhas AS, Scheel P, Garibaldi B, Liu G, Horton M, Jennings M, et al. Takotsubo syndrome in the setting of COVID-19. JACC Case Rep. (2020) 2:1321–5. doi: 10.1016/j.jaccas.2020.04.023
25. Citro R, Lyon AR, Meimoun P, Omerovic E, Redfors B, Buck T, et al. Standard and advanced echocardiography in takotsubo (stress) cardiomyopathy: clinical and prognostic implications. J Am Soc Echocardiogr. (2015) 28:57–74. doi: 10.1016/j.echo.2014.08.020
26. Nascimento FO, Yang S, Larrauri-Reyes M, Pineda AM, Cornielle V, Santana O, et al. Usefulness of the troponin-ejection fraction product to differentiate stress cardiomyopathy from ST-segment elevation myocardial infarction. Am J Cardiol. (2014) 113:429–33. doi: 10.1016/j.amjcard.2013.10.013
27. Citro R, Rigo F, Previtali M, Ciampi Q, Canterin FA, Provenza G, et al. Differences in clinical features and in-hospital outcomes of older adults with tako-tsubo cardiomyopathy. J Am Geriatr Soc. (2012) 60:93–8. doi: 10.1111/j.1532-5415.2011.03730.x
28. Dias A, Franco E, Rubio M, Bhalla V, Pressman GS, Amanullah S, et al. Usefulness of left ventricular strain analysis in patients with takotsubo syndrome during acute phase. Echocardiography. (2018) 35:179–83. doi: 10.1111/echo.13762
29. Akashi YJ, Goldstein DS, Barbaro G, Ueyama T. Takotsubo cardiomyopathy: a new form of acute, reversible heart failure. Circulation. (2008) 118:2754–62. doi: 10.1161/CIRCULATIONAHA.108.767012
30. Nagueh SF, Smiseth OA, Appleton CP, Byrd BF 3rd, Dokainish H, Edvardsen T, et al. Recommendations for the evaluation of left ventricular diastolic function by echocardiography: an update from the American Society of Echocardiography and the European Association of Cardiovascular Imaging. J Am Soc Echocardiogr. (2016) 29:277–314. doi: 10.1016/j.echo.2016.01.011
31. Meimoun P, Passos P, Benali T, Boulanger J, Elmkies F, Zemir H, et al. Assessment of left ventricular twist mechanics in Tako-tsubo cardiomyopathy by two-dimensional speckle-tracking echocardiography. Eur J Echocardiogr. (2011) 12:931–9. doi: 10.1093/ejechocard/jer183
32. Citro R, Rigo F, D'Andrea A, Ciampi Q, Parodi G, Provenza G, et al. Echocardiographic correlates of acute heart failure, cardiogenic shock, and in-hospital mortality in tako-tsubo cardiomyopathy. JACC Cardiovasc Imaging. (2014) 7:119–29. doi: 10.1016/j.jcmg.2013.09.020
33. Izumo M, Nalawadi S, Shiota M, Das J, Dohad S, Kuwahara E, et al. Mechanisms of acute mitral regurgitation in patients with takotsubo cardiomyopathy an echocardiographic study. Circ Cardiovasc Imaging. (2011) 4:392–8. doi: 10.1161/CIRCIMAGING.110.962845
34. El Mahmoud R, Mansencal N, Pilliére R, Leyer F, Abbou N, Michaud P, et al. Prevalence and characteristics of left ventricular outflow tract obstruction in Tako-Tsubo syndrome. Am Heart J. (2008). 156:543–548. doi: 10.1016/j.ahj.2008.05.002
35. Citro R, Caso I, Provenza G, Santoro M, Gregorio G, Bossone E. Right ventricular involvement and pulmonary hypertension in an elderly woman with tako-tsubo cardiomyopathy. Chest. (2010) 137:973–5. doi: 10.1378/chest.09-0923
36. Liu K, Carhart R. “Reverse McConnell's sign?”: A unique right ventricular feature of takotsubo cardiomyopathy. Am J Cardiol. (2013). 111:1232–1235. doi: 10.1016/j.amjcard.2012.12.007
37. Senior R, Becher H, Monaghan M, Agati L, Zamorano J, Vanoverschelde JL, et al. Clinical practice of contrast echocardiography: recommendation by the European Association of Cardiovascular Imaging (EACVI) (2017). Eur Hear J - Cardiovasc Imaging. (2017) 18:1205–1205af. doi: 10.1093/ehjci/jex182
38. Kohan AA, Yeyati EL, Stefano L De, Dragonetti L, Pietrani M, Arenaza DP de, et al. Usefulness of MRI in takotsubo cardiomyopathy: a review of the literature. Cardiovasc Diagn Ther. (2014) 4:138.
39. Plácido R, Cunha Lopes B, Almeida AG, Rochitte CE. The role of cardiovascular magnetic resonance in takotsubo syndrome. J Cardiovasc Magn Reson. (2016) 18:1–12. doi: 10.1186/s12968-016-0279-5
40. Templin C, Ghadri JR, Diekmann J, Napp LC, Bataiosu DR, Jaguszewski M, et al. Clinical features and outcomes of takotsubo (Stress) cardiomyopathy. N Engl J Med. (2015) 373:929–38. doi: 10.1056/NEJMoa1406761
41. Eitel I, Knobelsdorff-Brenkenhoff F von, Bernhardt P, Carbone I, Muellerleile K, Aldrovandi A, et al. Clinical characteristics and cardiovascular magnetic resonance findings in stress (Takotsubo) cardiomyopathy. JAMA. (2011) 306:277–86. doi: 10.1001/jama.2011.992
42. Haghi D, Athanasiadis A, Papavassiliu T, Suselbeck T, Fluechter S, Mahrholdt H, et al. Right ventricular involvement in Takotsubo cardiomyopathy. Eur Heart J. (2006) 27:2433–9. doi: 10.1093/eurheartj/ehl274
43. Stiermaier T, Lange T, Chiribiri A, Möller C, Graf T, Villnow C, et al. Left ventricular myocardial deformation in Takotsubo syndrome: a cardiovascular magnetic resonance myocardial feature tracking study. Eur Radiol. (2018) 28:5160–70. doi: 10.1007/s00330-018-5475-2
44. Eitel I, Friedrich MG. T2-weighted cardiovascular magnetic resonance in acute cardiac disease. J Cardiovasc Magn Reson. (2011) 13:13. doi: 10.1186/1532-429X-13-13
45. Thavendiranathan P, Walls M, Giri S, Verhaert D, Rajagopalan S, Moore S, et al. Improved detection of myocardial involvement in acute inflammatory cardiomyopathies using T2 mapping. Circ Cardiovasc Imaging. (2012) 5:102–10. doi: 10.1161/CIRCIMAGING.111.967836
46. Vermes E, Berradja N, Saab I, Genet T, Bertrand P, Pucheux J, et al. Cardiac magnetic resonance for assessment of cardiac involvement in Takotsubo syndrome: do we still need contrast administration? Int J Cardiol. (2020) 308:93–5. doi: 10.1016/j.ijcard.2020.03.039
47. Aikawa Y, Noguchi T, Morita Y, Tateishi E, Kono A, Miura H, et al. Clinical impact of native T1 mapping for detecting myocardial impairment in takotsubo cardiomyopathy. Eur Hear J Cardiovasc Imaging. (2019) 20:1147–55. doi: 10.1093/ehjci/jez034
48. Neil C, Nguyen TH, Kucia A, Crouch B, Sverdlov A, Chirkov Y, et al. Slowly resolving global myocardial inflammation/oedema in Tako-Tsubo cardiomyopathy: evidence from T2-weighted cardiac MRI. Heart. (2012) 98:1278–84. doi: 10.1136/heartjnl-2011-301481
49. Ojha V, Khurana R, Ganga KP, Kumar S. Advanced cardiac magnetic resonance imaging in takotsubo cardiomyopathy. Br J Radiol. (2020) 93:20200514. doi: 10.1259/bjr.20200514
50. Ferreira VM, Schulz-Menger J, Holmvang G, Kramer CM, Carbone I, Sechtem U, et al. Cardiovascular magnetic resonance in nonischemic myocardial inflammation: expert recommendations. J Am Coll Cardiol. (2018) 72:3158–76. doi: 10.1016/j.jacc.2018.09.072
51. Nakamori S, Matsuoka K, Onishi K, Kurita T, Ichikawa Y, Nakajima H, et al. Prevalence and signal characteristics of late gadolinium enhancement on contrast-enhanced magnetic resonance imaging in patients with takotsubo cardiomyopathy. Circ J. (2012) 76:914–21. doi: 10.1253/circj.CJ-11-1043
52. Rolf A, Nef HM, Möllmann H, Troidl C, Voss S, Conradi G, et al. Immunohistological basis of the late gadolinium enhancement phenomenon in tako-tsubo cardiomyopathy. Eur Heart J. (2009) 30:1635–42. doi: 10.1093/eurheartj/ehp140
53. Naruse Y, Sato A, Kasahara K, Makino K, Sano M, Takeuchi Y, et al. The clinical impact of late gadolinium enhancement in Takotsubo cardiomyopathy: serial analysis of cardiovascular magnetic resonance images. J Cardiovasc Magn Reson. (2011) 13:67. doi: 10.1186/1532-429X-13-67
54. Wu KC, Weiss RG, Thiemann DR, Kitagawa K, Schmidt A, Dalal D, et al. Late gadolinium enhancement by cardiovascular magnetic resonance heralds an adverse prognosis in nonischemic cardiomyopathy. J Am Coll Cardiol. (2008) 51:2414–21. doi: 10.1016/j.jacc.2008.03.018
55. Kang Y, Kim YK, Chun EJ, Chor S Il. Potential pathophysiology and clinical workflow of stress- induced cardiomyopathy. Cardiovasc Imaging Asia. (2018) 2:19–27. doi: 10.22468/cvia.2017.00157
56. Cimarelli S, Imperiale A, Ben-Sellem D, Rischner J, Detour J, Morel O, et al. Nuclear medicine imaging of takotsubo cardiomyopathy: typical form and midventricular ballooning syndrome. J Nucl Cardiol. (2008) 15:137–141. doi: 10.1007/BF02976903
57. Lancellotti P, Price S, Edvardsen T, Cosyns B, Neskovic AN, Dulgheru R, et al. The use of echocardiography in acute cardiovascular care: recommendations of the European Association of Cardiovascular Imaging and the Acute Cardiovascular Care Association. Eur Hear J Cardiovasc Imaging. (2015) 16:119–46. doi: 10.1093/ehjci/jeu210
58. Chirumamilla A, Travin MI. Cardiac applications of 123I-mIBG imaging. Semin Nucl Med. (2011) 41:374–87. doi: 10.1053/j.semnuclmed.2011.04.001
59. Squame F, Mansi L, Tinoco Mesquita C, Rezende MF. Nuclear Cardiology Basic and Advanced Concepts in Clinical Practice. Cham: Springer Nature Switzerland (2021).
60. Marano R, De Cobelli F, Floriani I, Becker C, Herzog C, Centonze M, et al. Italian multicenter, prospective study to evaluate the negative predictive value of 16- and 64-slice MDCT imaging in patients scheduled for coronary angiography (NIMISCAD-Non Invasive Multicenter Italian Study for Coronary Artery Disease). Eur Radiol. (2009) 19:1114–23. doi: 10.1007/s00330-008-1239-8
61. Stiermaier T, Moeller C, Oehler K, Desch S, Graf T, Eitel C, et al. Long-term excess mortality in takotsubo cardiomyopathy: predictors, causes and clinical consequences. Eur J Heart Fail. (2016) 18:650–6. doi: 10.1002/ejhf.494
62. Bybee KA, Kara T, Prasad A, Lerman A, Barsness GW, Wright R, Scott, et al. Systematic Review: transient left ventricular apical ballooning: a syndrome that mimics ST-segment elevation myocardial infarction. Ann Intern Med. (2004) 141:858–65. doi: 10.7326/0003-4819-141-11-200412070-00010
63. Citro R, Bossone E, Parodi G, Rigo F, Nardi F, Provenza G, et al. Independent impact of RV involvement on in-hospital outcome of patients with takotsubo syndrome. JACC Cardiovasc Imaging. (2016) 9:894–5. doi: 10.1016/j.jcmg.2015.06.005
64. Kagiyama N, Okura H, Tamada T, Imai K, Yamada R, Kume T, et al. Impact of right ventricular involvement on the prognosis of takotsubo cardiomyopathy. Eur Heart J Cardiovasc Imaging. (2016) 17:210. doi: 10.1093/ehjci/jev145
65. Haghi D, Papavassiliu T, Heggemann F, Kaden JJ, Borggrefe M, Suselbeck T. Incidence and clinical significance of left ventricular thrombus in tako-tsubo cardiomyopathy assessed with echocardiography. QJM. (2008) 101:381–6. doi: 10.1093/qjmed/hcn017
66. Heckle MR, McCoy CW, Akinseye OA, Khouzam RN. Stress-induced thrombus: prevalence of thromboembolic events and the role of anticoagulation in Takotsubo cardiomyopathy. Ann Transl Med. (2018) 6:4–4. doi: 10.21037/atm.2017.11.01
67. Kurisu S, Inoue I, Kawagoe T, Ishihara M, Shimatani Y, Nakama Y, et al. Incidence and treatment of left ventricular apical thrombosis in Tako-tsubo cardiomyopathy. Int J Cardiol. (2011) 146:e58–60. doi: 10.1016/j.ijcard.2008.12.208
68. Santoro F, Stiermaier T, Tarantino N, De Gennaro L, Moeller C, Guastafierro F, et al. Left ventricular Thrombi in Takotsubo syndrome: Incidence, predictors, and management: Results from the GEIST (German Italian stress cardiomyopathy) registry. J Am Heart Assoc. (2017) 6:e006990. doi: 10.1161/JAHA.117.006990
69. Pant S, Deshmukh A, Mehta K, Badheka AO, Tuliani T, Patel NJ, et al. Burden of arrhythmias in patients with Takotsubo Cardiomyopathy (Apical Ballooning Syndrome). Int J Cardiol. (2013) 170:64–8. doi: 10.1016/j.ijcard.2013.10.041
70. Syed FF, Asirvatham SJ, Francis J. Arrhythmia occurrence with takotsubo cardiomyopathy: a literature review. EP Eur. (2011) 13:780–8. doi: 10.1093/europace/euq435
71. Stiermaier T, Santoro F, Eitel C, Graf T, Möller C, Tarantino N, et al. Prevalence and prognostic relevance of atrial fibrillation in patients with Takotsubo syndrome. Int J Cardiol. (2017) 245:156–61. doi: 10.1016/j.ijcard.2017.07.053
72. Migliore F, Zorzi A, Peruzza F, Perazzolo Marra M, Tarantini G, Iliceto S, et al. Incidence and management of life-threatening arrhythmias in Takotsubo syndrome. Int J Cardiol. (2013) 166:261–3. doi: 10.1016/j.ijcard.2012.09.107
73. Madias C, Fitzgibbons TP, Alsheikh-Ali AA, Bouchard JL, Kalsmith B, Garlitski AC, et al. Acquired long QT syndrome from stress cardiomyopathy is associated with ventricular arrhythmias and torsades de pointes. Hear Rhythm. (2011) 8:555–61. doi: 10.1016/j.hrthm.2010.12.012
74. Ghadri J-R, Wittstein IS, Prasad A, Sharkey S, Dote K, Akashi YJ, et al. International expert consensus document on takotsubo syndrome (Part II): diagnostic workup, outcome, and management. Eur Heart J. (2018) 39:2047–62. doi: 10.1093/eurheartj/ehy077
75. Yaman M, Arslan U, Kaya A, Akyol A, Ozturk F, Okudan YE, et al. Levosimendan accelerates recovery in patients with takotsubo cardiomyopathy. Cardiol J. (2016) 23:610–5. doi: 10.5603/CJ.a2016.0100
76. Santoro F, Ieva R, Ferraretti A, Ienco V, Carpagnano G, Lodispoto M, et al. Safety and feasibility of levosimendan administration in takotsubo cardiomyopathy: a case series. Cardiovasc Ther. (2013) 31:e133–7. doi: 10.1111/1755-5922.12047
77. Lisi E, Guida V, Blengino S, Pedrazzi E, Ossoli D, Parati G. Intra-aortic balloon pump for treatment of refractory ventricular tachycardia in Tako-Tsubo cardiomyopathy: a case report. Int J Cardiol. (2014) 174:135–6. doi: 10.1016/j.ijcard.2014.03.102
78. Rashed A, Won S, Saad M, Schreiber T. Use of the Impella 2.5 left ventricular assist device in a patient with cardiogenic shock secondary to takotsubo cardiomyopathy. BMJ Case Rep. (2015) 2015:bcr2014208354. doi: 10.1136/bcr-2014-208354
79. Bonacchi M, Maiani M, Harmelin G, Sani G. Intractable cardiogenic shock in stress cardiomyopathy with left ventricular outflow tract obstruction: is extra-corporeal life support the best treatment? Eur J Heart Fail. (2009) 11:721–7. doi: 10.1093/eurjhf/hfp068
80. Singh K, Carson K, Shah R, Sawhney G, Singh B, Parsaik A, et al. Meta-analysis of clinical correlates of acute mortality in takotsubo cardiomyopathy. Am J Cardiol. (2014) 113:1420–8. doi: 10.1016/j.amjcard.2014.01.419
81. Santoro F, Ieva R, Musaico F, Ferraretti A, Triggiani G, Tarantino N, et al. Lack of efficacy of drug therapy in preventing takotsubo cardiomyopathy recurrence: a meta-analysis. Clin Cardiol. (2014) 37:434–9. doi: 10.1002/clc.22280
82. O'Gara PT, Kushner FG, Ascheim DD, Casey DE Jr, Chung MK, de Lemos JA, et al. 2013 ACCF/AHA guideline for the management of ST-elevation myocardial infarction. Circulation. (2013) 127:e362–425. doi: 10.1161/CIR.0b013e3182742cf6
83. Schneider B, Athanasiadis A, Schwab J, Pistner W, Gottwald U, Schoeller R, et al. Complications in the clinical course of tako-tsubo cardiomyopathy. Int J Cardiol. (2014) 176:199–205. doi: 10.1016/j.ijcard.2014.07.002
84. Deshmukh A, Kumar G, Pant S, Rihal C, Murugiah K, Mehta JL, et al. Prevalence of Takotsubo cardiomyopathy in the United States. Am Heart J. (2012) 164:66–71.e1. doi: 10.1016/j.ahj.2012.03.020
85. Brinjikji W, El-Sayed AM, Salka S. In-hospital mortality among patients with takotsubo cardiomyopathy: a study of the national inpatient sample 2008 to 2009. Am Heart J. (2012) 164:215–21. doi: 10.1016/j.ahj.2012.04.010
86. Isogai T, Yasunaga H, Matsui H, Tanaka H, Ueda T, Horiguchi H, et al. Out-of-hospital versus in-hospital Takotsubo cardiomyopathy: analysis of 3719 patients in the diagnosis procedure combination database in Japan. Int J Cardiol. (2014) 176:413–7. doi: 10.1016/j.ijcard.2014.07.110
87. Sharkey SW, Pink VR, Lesser JR, Garberich RF, Maron MS, Maron BJ. Clinical profile of patients with high-risk tako-tsubo cardiomyopathy. Am J Cardiol. (2015) 116:765–72. doi: 10.1016/j.amjcard.2015.05.054
88. Elesber AA, Prasad A, Lennon RJ, Wright RS, Lerman A, Rihal CS. Four-year recurrence rate and prognosis of the apical ballooning syndrome. J Am Coll Cardiol. (2007) 50:448–52. doi: 10.1016/j.jacc.2007.03.050
89. Schneider B, Athanasiadis A, Sechtem U. Gender-related differences in takotsubo cardiomyopathy. Heart Fail Clin. (2013) 9(2):137–46, vii. doi: 10.1016/j.hfc.2012.12.005
90. Salmoirago-Blotcher E, Rosman L, Wittstein IS, Dunsiger S, Swales HH, Aurigemma GP, et al. Psychiatric history, post-discharge distress, and personality characteristics among incident female cases of takotsubo cardiomyopathy: a case–control study. Hear Lung J Cardiopulm Acute Care. (2016) 45:503–9. doi: 10.1016/j.hrtlng.2016.07.008
Keywords: cardiomyopathy, CMR (cardiovascular magnetic resonance), echocardiography, stress cardiomyopathy, imaging
Citation: Zghyer F, Botheju WSP, Kiss JE, Michos ED, Corretti MC, Mukherjee M and Hays AG (2022) Cardiovascular Imaging in Stress Cardiomyopathy (Takotsubo Syndrome). Front. Cardiovasc. Med. 8:799031. doi: 10.3389/fcvm.2021.799031
Received: 21 October 2021; Accepted: 15 December 2021;
Published: 28 January 2022.
Edited by:
Fabrizio Ricci, University of Studies G. d'Annunzio Chieti and Pescara, ItalyReviewed by:
Luca Arcari, M.G. Ospedale Vannini, ItalyAnwar A. Chahal, University of Pennsylvania, United States
Sylvia J. Buchmann, Vivantes Klinikum Spandau, Germany
Copyright © 2022 Zghyer, Botheju, Kiss, Michos, Corretti, Mukherjee and Hays. This is an open-access article distributed under the terms of the Creative Commons Attribution License (CC BY). The use, distribution or reproduction in other forums is permitted, provided the original author(s) and the copyright owner(s) are credited and that the original publication in this journal is cited, in accordance with accepted academic practice. No use, distribution or reproduction is permitted which does not comply with these terms.
*Correspondence: Allison G. Hays, YWxsaXNvbi5oYXlzJiN4MDAwNDA7Z21haWwuY29t