- 1Royal Brompton Hospital, Imaging Centre, Cardiac Magnetic Resonance Unit, London, United Kingdom
- 2Third Department of Cardiology, Aristotle University of Thessaloniki, Thessaloniki, Greece
- 3Unit of Inherited Cardiac Conditions, First Cardiology Department, University of Athens, Athens, Greece
Background: Cardiac magnetic resonance (CMR) combined with late gadolinium enhancement (LGE) has revealed a non-negligible increased incidence of myocardial fibrosis (MF) in athletes compared to healthy sedentary controls.
Objective: The aim of this systematic research and meta-analysis is to investigate and present our perspective regarding CMR indices in athletes compared to sedentary controls, including T1 values, myocardial extracellular volume (ECV) and positive LGE indicative of non-specific fibrosis, also to discuss the differences between young and veteran athletes.
Methods: The protocol included searching, up to October 2021, of MEDLINE, EMBASE, SPORTDiscus, Web of Science and Cochrane databases for original studies assessing fibrosis via CMR in athletes. A mean age of 40 years differentiated studies' athletic populations to veteran and young.
Results: The research yielded 14 studies including in total 1,312 individuals. There was a statistically significant difference in LGE fibrosis between the 118/759 athletes and 16/553 controls (Z = 5.2, P < 0.001, I2 = 0%, PI = 0.45). Notably, LGE fibrosis differed significantly between 546 (14.6%) veteran and 140 (25.7%) young athletes (P = 0.002). At 1.5T, T1 values differed between 117 athletes and 48 controls (P < 0.0001). A statistically significant difference was also shown at 3T (110 athletes vs. 41 controls, P = 0.0004), as well as when pooling both 1.5T and 3T populations (P < 0.00001). Mean ECV showed no statistically significant difference between these groups.
Conclusions: Based on currently available data, we reported that overall LGE based non-specific fibrosis and T1 values differ between athletes and sedentary controls, in contrast to ECV values. Age of athletes seems to have impact on the incidence of MF. Future prospective studies should focus on the investigation of the underlying pathophysiological mechanisms.
Introduction
Athletic training is known to induce morphological and functional cardiovascular adaptations of cardiac chambers, collectively known as athlete's heart (1). Apart from the widely established benefits of exercise, recent evidence suggests there may be some overlap between physiology and pathophysiology, and occasionally, with cardiac diseases. The duration of exposure to demanding training and the type of sports may play a role in these processes (2). Cardiac magnetic resonance (CMR) has been increasingly used in establishing an accurate diagnosis given that exercise may lead to cardiac remodeling that in certain situations can be clinically challenging to differentiate from various cardiomyopathies (3, 4).
CMR is a non-radiating imaging modality with high spatial resolution, which not only is the reference standard for functional and morphological assessment but also has the benefit of tissue characterization by exploiting gadolinium-based contrast late enhancement (LGE) as a marker of myocardial fibrosis (MF) (5, 6). Gadolinium-based contrast compounds freely enter the extracellular space but not intact myocardial cells. Under pathologic conditions, cellular death and fibrotic processes lead to expansion of extracellular space, while cell damage means that myocardial cell membranes become permeable to contrast. These phenomena significantly alter the kinetics of gadolinium, leading to higher peak uptake from the myocardium and delayed washout (7). Interestingly specific patterns of LGE have been sporadically detected in athletic individuals, although data so far are not consistent, coming from small-sample studies that frequently lack comparisons with sedentary controls or “lifelong,” veteran athletic individuals which could provide further insight in the underlying pathophysiological mechanisms (8).
Other important techniques, such as native T1 and extracellular volume (ECV) may prove clinically relevant in athletic individuals providing additional information regarding cellular and extracellular pathology, even though they have not yet been widely studied in these populations (9). Native T1 times quantify the time required for the net magnetization vector of a myocardial area to return to pre-excitation levels. Native T1 depends highly on the tissue composition (10). Elevated T1 times have been reported in several myocardial pathologic conditions. Pertinent to the topic of this review, T1 values increase in situations of increased free water within expanded interstitial space and could be used to detect and quantify interstitial myocardial fibrosis (11). The measurement of pre- and post-contrast T1 times enable the CMR-based calculation of ECV with good correlation to the actual histology-derived collagen volume fraction (12). The ratio of intra- to extracellular space shifts significantly in myocardial fibrosis, and ECV is uniquely capable of detecting such changes. It thus constitutes an ideal modality for the clinical applications described in the present review (13).
Systematic Review
Systematic Review Rationale-Objectives
In a previous systematic review involving only 65 athletes (14), van de Schoor et al. predominantly identified MF in the intraventricular septum and the right ventricular (RV) insertion points. Although the underlying mechanisms are widely undetermined, the summarized evidence supported genetic predisposition, silent myocarditis, pulmonary artery pressure overload, and prolonged exercise-induced repetitive micro-injury as possible contributors (15). More recently, Zhang et al. (16) performed a meta-analysis of athletic individuals and sedentary controls who underwent CMR, focusing however only on general MF prevalence without looking into different patterns, and without discriminating different athletic age groups, or sex-specific data. According to the results, 21.1% of athletes had evidence of LGE, compared to just 3.2% in sedentary controls. The heterogeneity of the 12 included studies was acceptable (P = 0.34), while the difference in prevalence was statistically significant, suggesting a correlation between MF and intense athletic training.
In view of updated data on the topic of CMR based assessment, using both contrast and non-contrast techniques, we performed a revised systematic search and meta-analysis, with a three-fold aim: To focus on updated, peer-reviewed data, report a risk of bias assessment, which was unfortunately missing from the recent meta-analysis (16), and extract data on other CMR-derived techniques, such as native T1 mapping as a sensitive marker of interstitial fibrosis and ECV quantification, as marker of myocardial tissue remodeling, based on studies using both 1.5 and 3 Tesla (T) scanners. Of note, structured assessment of risk of bias and exclusion of low-quality data is crucial, since recruitment bias can have an enormous effect on the prevalence of LGE in an athlete population.
Systematic Review Strategy
The systematic research protocol was registered in the PROSPERO database (ID: CRD42021273996). MEDLINE, EMBASE, SPORTDiscus, Web of Science and Cochrane databases were systematically searched with the use of PubMed, Google Scholar and Cochrane Reviews search Engines between January 2000 up to October 2021. Studies were eligible for inclusion in the systematic review if they evaluated one or more of the following parameters in endurance sports athletes: (a) The presence of late gadolinium enhancement, (b) Native T1 values and (c) ECV. The Oxford Dictionary definition was used to identify endurance sports (“a sport that involves continuous high intensity exercise”) (17). Only studies reported in English were assessed for inclusion. As for the exclusion criteria, studies lacking a control arm of age- and sex-matched individuals were excluded from the systematic review, as were studies in which either controls or athletes had been included on the basis of having symptoms or signs of cardiac pathology (e.g., premature ventricular contractions) were excluded. When multiple studies reported on data from the same research group, only one was kept, unless it is explicitly stated that there was no overlap. A detailed presentation of the systematic protocol is described in Supplementary Material. Bias assessment was performed via the Newcastle-Ottawa quality assessment scale (NOS) for cohort studies (18). The Review Manager (RevMan) Version 5.3 and SPSS (IBM Corp. Released 2015. IBM SPSS Statistics for Windows, Version 23.0. Armonk, NY: IBM Corp.) were used for statistical analysis. Random effect was the model of choice for all pooled analyses with Z-value for overall effect and I2 for heterogeneity. Heterogeneity was considered significant at the level of p < 0.10. T1 and ECV, were separately assessed for 1.5 and 3 Tesla and afterwards in total. The incidence of LGE in younger vs. veteran athletes (cut-off set at 40y) was compared in a sub-analysis through the chi-square test. Reported means and standard deviations (SD) are pooled from study data. Data was mathematically transformed if needed. P-values of <0.05 were considered statistically significant unless otherwise stated.
Results
LGE in Athletes and Sedentary Controls
Fourteen (14) studies (4, 19–31) matching the pre-specified inclusion criteria were found and included in the updated review and meta-analysis (Table 1). A risk of bias assessment was performed using the Newcastle Ottawa Scale (Supplementary Table 1). In total, 759 athletes and 553 sedentary controls were included in the updated meta-analysis. Out of these, 118 (16.6%) athletes and 12 (2.3%) controls had LGE, a difference in proportions that proved statistically significant (Z = 5.2, P < 0.001). Study heterogeneity regarding LGE was low (I2 = 0%, PI = 0.45) (Figure 1). Most of the studies either included only male athletes and controls or reported sex-specific data. Forest plots could be constructed for male (4, 21, 22, 24–29, 31) and female (21, 22, 31) athletes. In total, LGE data regarding 460 male athletes were compared to those of 315 sedentary controls. The Z-overall effect was estimated at 4.76 with P < 0.001. Heterogeneity was low (I2 = 0%, textitPI = 0.80). Regarding females, 119 athletes were compared with 82 sedentary controls. No statistically significant difference was observed (P = 0.10) for a Z-overall effect of 1.67, while heterogeneity was not negligible (I2 = 44%, PI = 0.18).
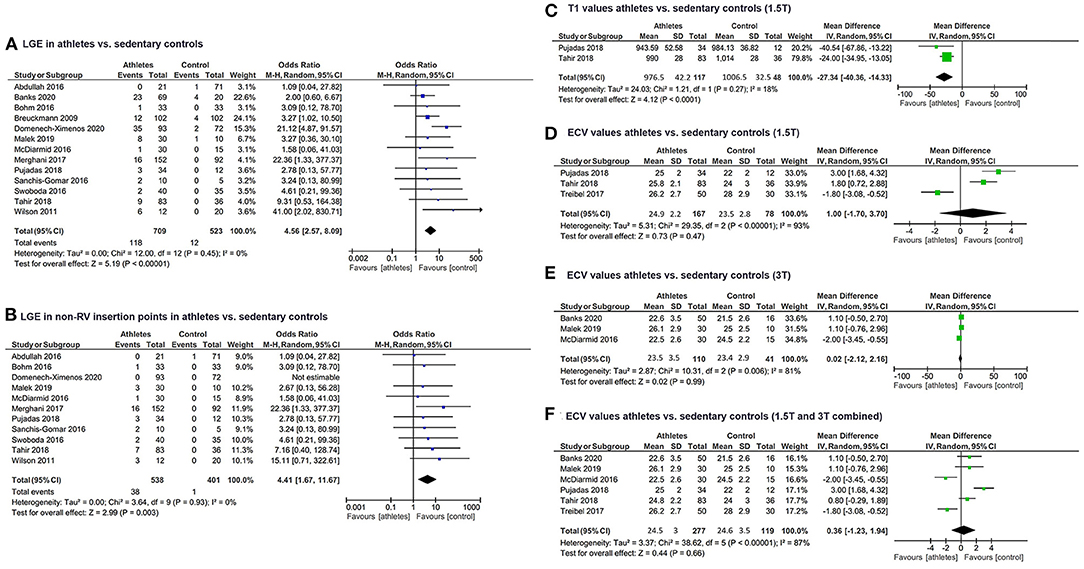
Figure 1. Forest plots of (A) LGE prevalence in athletes and sedentary controls, (B) LGE prevalence excluding RV insertion point LGE in athletes and sedentary controls, (C) Native T1 values from 1.5T CMR scans in athletes and sedentary controls, (D) ECV from 1.5T CMR scans in athletes and sedentary controls, (E) ECV from 3T CMR scans in athletes and sedentary controls, (F) Pooled ECV in athletes and sedentary controls. LGE, late gadolinium enhancement; RV, right ventricle; CMR, cardiac magnetic resonance; ECV, extracellular volume.
Regarding the comparison of young vs. veteran athletes, LGE data were available for 10 studies. A mean age over 40 years (546 athletes total, 14.6% exhibited LGE) and three studies with a mean age below 40 years (140 athletes total, 25.7% exhibited LGE). The chi-square test revealed a statistically significant difference of LGE incidence between the two groups of veteran and young athletes (chi-square = 9.7, P = 0.002).
LGE in RV insertion points is increasingly considered a non-specific finding of unknown significance (21, 22). A focused sub-analysis investigating the incidence of non-RV insertion point LGE revealed that the prevalence is also substantially higher in athletes (38 out of 538 included, 7%) compared to controls (one out of 401 included, 0.3%, Z = 2.99, P = 0.003).
T1 and ECV in Athletes vs. Sedentary Controls
Data regarding alternative non-contrast markers such as T1 and ECV derived from 3T and 1.5T MRI were extracted separately from included studies. Two studies (28, 31) had 1.5T T1 data available for a total of 117 athletes (mean T1 976.5 ± 42.2 msec) and 48 controls (1006.5 ± 32.5 msec) showed a statistically significant difference (Z = 4.12, P < 0.0001, I2 = 18%, PI = 0.27). T1 data with 3T scanners were similar—three studies (20, 26, 27) including 110 athletes (mean T1 1179.9 ± 42.1 msec) and 41 controls (mean T1 1200.2 ± 31 msec) also yielded a statistically significant difference in T1 values between athletes and sedentary controls (Z = 3.55, P = 0.0004, I2 = 0%, PI = 0.86) (Figure 1). When pooled into one forest plot, data from 1.5T and 3T CMR scans revealed a significant difference in T1 values between the two groups, while having no heterogeneity (Z = 6.15, P < 0.00001, I2 = 0%, PI = 0.66). Controls' mean T1 was estimated at 1095.7 ± 101.7 ms, whereas athletes' mean T1 was 1075.1 ± 110.4 msec.
ECV quantified via 1.5T MRI scanners (23, 28, 31) in three studies did not differ significantly (Z = 0.73, P = 0.47, I2 = 93%, PI = PI < 0.00001) between the total 167 athletes (mean ECV 24.9 ± 2.2%) and 78 sedentary controls (mean ECV 23.5 ± 2.8%). MRI scans at 3T (20, 26, 27) comparing ECV between 110 athletes (mean ECV 23.5 ± 3.5%) to 41 sedentary controls (mean ECV 23.4 ± 2.9%) were also characterized by a not statistically significant mean difference (Z = 0.02, P = 0.99, I2 = 81%, PI < 0.006) (Figure 1). The same tendency was observed when pooling mean ECV of athletes (24.5 ± 3%) and control groups (24.6 ± 3.5%) scanned with 1.5T and 3T and comparing them, as the mean difference had a Z = 0.42 and P = 0.66 (I2 = 87%, PI < 0.00001).
Discussion
All available evidence indicates that the prevalence of MF, as documented by LGE in CMR scans, is significantly higher in athletes compared to sedentary controls. The pooled frequency of 16.6% is lower than the 21.1% reported by Zhang et al. (16), with the difference owed to the updated set of studies, which include new data, updated numbers from some research teams and exclude some previous, non-peer-reviewed datasets.
In a close examination of the included studies, LGE was indeed considerably more frequent in athletes compared to controls, even when excluding RV insertion point fibrosis. Studies that included younger athletes also had a significantly higher prevalence of LGE compared to studies including veteran athletes. This is an interesting finding that is open to interpretation. It is not inconceivable that the differences in recruitment strategy between studies that led to an age disparity also caused differences in the prevalence of LGE. Importantly, Domenech-Ximenos et al., one of the included studies with the lowest mean athlete age, only administered gadolinium-based contrast to a sub-set of participating patients, potentially introducing bias in LGE results (21). Overall, the long-term effects of endurance exercise on the heart are widely unknown. Endurance exercise is associated with a transient increase of biomarkers of cardiac damage and there is growing evidence that lifelong male athletes aged above 40 years show a higher prevalence of a higher coronary plaque burden, and a different MF pattern compatible with subclinical infarction compared with relatively sedentary healthy controls (32). As no adequate data were available for females, no sex-specific conclusions could be safely drawn from our analysis. Further, ideally prospective studies with sizeable athlete populations are required to determine the effect of duration exercise on the incidence, pattern, and extent of MF as well as its prognostic relevance.
Quite interestingly, native T1 values were consistently shown to be significantly decreased in athletes, both in 1.5T and 3T magnetic fields. It has been suggested that in athletic left ventricular hypertrophy (LVH), native T1 seems to be decreased suggesting that physiological athletic LVH represents enhanced cellular hypertrophy, unlike any other sort of LVH mechanism (6). The ECV as a marker of myocardial tissue remodeling and excessive collagen deposition is also a robust measure of diffuse MF and fairly interesting from a pathophysiological perspective. It would not be unreasonable to assume that, in the setting of exercise-induced cardiomyocyte hypertrophy (6), the ECV is expected to be reduced. That said, the ECV was not found significantly increased in athletes compared to controls, and indeed some studies yielded the opposite result (23, 27). Whether this is the result of study selection bias, relatively small numbers of included so far studies, or whole-heart sub-clinical expansion of ECV through increased collagen deposition in maladapted athletes' hearts (33) is an interesting research question that deserves further investigation.
In conclusion, we report interesting data based on the latest evidence in MF assessment from both contrast based and non-contrast CMR techniques. The present systematic review and meta-analysis contains updated data about LGE prevalence along with a comprehensive risk of bias assessment, in combination with a novel meta-synthesis of data regarding T1 and ECV values in the endurance athletes. Non-specific MF in athletic individuals is a somewhat frequent finding in highly trained athletes and there seem to be variation attributable to age (and therefore potentially the duration of exposure to exercise) and sex. Contrast based non-specific LGE and native T1 are found to be particularly useful in discrimination of athletic vs. sedentary individuals. Further data are certainly required to elucidate the underlying physiological and pathophysiological mechanisms. More importantly, whether these non-specific LGE patterns in athletes are actually associated with adverse events-particularly for underrepresented athlete groups such as women and veteran athletes, as well as the effect of deconditioning on the fibrotic process, are all certainly topics for further research.
Data Availability Statement
The raw data supporting the conclusions of this article will be made available by the authors, without undue reservation.
Author Contributions
EA and CV conceived and designed the present systematic review. AA and PP also contributed meaningfully to the design of the systematic review and meta-analysis. DM, AT, and CB performed the systematic literature search. DM, AT, CB, and AA performed the analysis of data. EA, DM, AT, and CB wrote the initial draft versions of the manuscript. PP, AA, and CV performed significant revisions. All authors provided critical feedback and helped shape the research, analysis, and manuscript.
Conflict of Interest
The authors declare that the research was conducted in the absence of any commercial or financial relationships that could be construed as a potential conflict of interest.
Publisher's Note
All claims expressed in this article are solely those of the authors and do not necessarily represent those of their affiliated organizations, or those of the publisher, the editors and the reviewers. Any product that may be evaluated in this article, or claim that may be made by its manufacturer, is not guaranteed or endorsed by the publisher.
Supplementary Material
The Supplementary Material for this article can be found online at: https://www.frontiersin.org/articles/10.3389/fcvm.2021.784474/full#supplementary-material
Abbreviations
CMR, cardiac magnetic resonance; LGE, late gadolinium enhancement; ECV, extracellular volume; min, minutes; RV, right ventricular; CAD, coronary artery disease; LAD, left anterior descending artery; LCA, left coronary artery, RCA, right coronary artery; IVS, interventricular septum.
References
1. Sharma S, Merghani A, Mont L. Exercise and the heart: the good, the bad, the ugly. Eur Heart J. (2015) 36:1445–53. doi: 10.1093/eurheartj/ehv090
2. La Gerche. Can intense endurance exercise cause myocardial damage and fibrosis? Curr Sports Med Rep. (2013) 12:63–9. doi: 10.1249/JSR.0b013e318287488a
3. Gati S, Sharma S, Pennell D. The role of cardiovascular magnetic resonance imaging in the assessment of highly trained athletes. JACC Cardiovasc Imaging. (2018) 11(2 Pt 1):247–59. doi: 10.1016/j.jcmg.2017.11.016
4. Bohm P, Schneider G, Linneweber L, Rentzsch A, Kramer N, Abdul-Khaliq H, et al. Right and left ventricular function and mass in male elite master athletes: a controlled contrast-enhanced cardiovascular magnetic resonance study. Circulation. (2016) 133:1927–35. doi: 10.1161/CIRCULATIONAHA.115.020975
5. La Gerche A, Taylor AJ, Prior DL. Athlete's heart: the potential for multimodality imaging to address the critical remaining questions. JACC Cardiovasc Imaging. (2009) 2:350–63. doi: 10.1016/j.jcmg.2008.12.011
6. Maestrini V, Torlasco C, Hughes R, Moon JC. Cardiovascular magnetic resonance and sport cardiology: a growing role in clinical dilemmas. J Cardiovasc Transl Res. (2020) 13:296–305. doi: 10.1007/s12265-020-10022-7
7. Doltra A, Amundsen BH, Gebker R, Fleck E, Kelle S. Emerging concepts for myocardial late gadolinium enhancement MRI. Curr Cardiol Rev. (2013) 9:185–90. doi: 10.2174/1573403X113099990030
8. Androulakis E, Swoboda PP. The role of cardiovascular magnetic resonance in sports cardiology; current utility and future perspectives. Curr Treat Options Cardiovasc Med. (2018) 20:86. doi: 10.1007/s11936-018-0679-y
9. Robinson AA, Chow K, Salerno M. Myocardial T1 and ECV measurement: underlying concepts and technical considerations. JACC Cardiovasc Imaging. (2019) 12(11 Pt 2):2332–44. doi: 10.1016/j.jcmg.2019.06.031
10. Moon JC, Messroghli DR, Kellman P, Piechnik SK, Robson MD, Ugander M, et al. Myocardial T1 mapping and extracellular volume quantification: a Society for Cardiovascular Magnetic Resonance (SCMR) and CMR Working Group of the European Society of Cardiology consensus statement. J Cardiovasc Magn Reson. (2013) 15:92. doi: 10.1186/1532-429X-15-92
11. Ferreira VM, Piechnik SK, Robson MD, Neubauer S, Karamitsos TD. Myocardial tissue characterization by magnetic resonance imaging: novel applications of T1 and T2 mapping. J Thorac Imaging. (2014) 29:147–54. doi: 10.1097/RTI.0000000000000077
12. Fontana M, White SK, Banypersad SM, Sado DM, Maestrini V, Flett AS, et al. Comparison of T1 mapping techniques for ECV quantification. Histological validation and reproducibility of ShMOLLI versus multibreath-hold T1 quantification equilibrium contrast CMR. J Cardiovasc Magn Reson. (2012) 14:88. doi: 10.1186/1532-429X-14-88
13. Bakogiannis C, Mouselimis D, Tsarouchas A, Papatheodorou E, Vassilikos VP, Androulakis E. Hypertrophic cardiomyopathy or athlete's heart? a systematic review of novel cardiovascular magnetic resonance imaging parameters. Eur J Sport Sci. (2021) 1–30. doi: 10.1080/17461391.2021.2001576
14. van de Schoor FR, Aengevaeren VL, Hopman MT, Oxborough DL, George KP, Thompson PD, et al. Myocardial fibrosis in athletes. Mayo Clin Proc. (2016) 91:1617–31. doi: 10.1016/j.mayocp.2016.07.012
15. Kellman P, Arai AE. Cardiac imaging techniques for physicians: late enhancement. J Magn Reson Imaging. (2012) 36:529–42. doi: 10.1002/jmri.23605
16. Zhang CD, Xu SL, Wang XY, Tao LY, Zhao W, Gao W. Prevalence of myocardial fibrosis in intensive endurance training athletes: a systematic review and meta-analysis. Front Cardiovasc Med. (2020) 7:585692. doi: 10.3389/fcvm.2020.585692
17. Kent M. The Oxford Dictionary of Sports Science and Medicine. Oxford: Oxford University Press (2006).
18. Margulis AV, Pladevall M, Riera-Guardia N, Varas-Lorenzo C, Hazell L, Berkman ND, et al. Quality assessment of observational studies in a drug-safety systematic review, comparison of two tools: the Newcastle-Ottawa Scale and the RTI item bank. Clin Epidemiol. (2014) 6:359–68. doi: 10.2147/CLEP.S66677
19. Abdullah SM, Barkley KW, Bhella PS, Hastings JL, Matulevicius S, Fujimoto N, et al. Lifelong physical activity regardless of dose is not associated with myocardial fibrosis. Circ Cardiovasc Imaging. (2016) 9:e005511. doi: 10.1161/CIRCIMAGING.116.005511
20. Banks L, Altaha MA, Yan AT, Dorian P, Konieczny K, Deva DP, et al. Left ventricular fibrosis in middle-age athletes and physically active adults. Med Sci Sports Exerc. (2020) 52:2500–7. doi: 10.1249/MSS.0000000000002411
21. Domenech-Ximenos B, Sanz-de la Garza M, Prat-Gonzalez S, Sepulveda-Martinez A, Crispi F, Duran-Fernandez K, et al. Prevalence and pattern of cardiovascular magnetic resonance late gadolinium enhancement in highly trained endurance athletes. J Cardiovasc Magn Reson. (2020) 22:62. doi: 10.1186/s12968-020-00660-w
22. Merghani A, Maestrini V, Rosmini S, Cox AT, Dhutia H, Bastiaenan R, et al. Prevalence of subclinical coronary artery disease in masters endurance athletes with a low atherosclerotic risk profile. Circulation. (2017) 136:126–37. doi: 10.1161/CIRCULATIONAHA.116.026964
23. Treibel TA, Kozor R, Menacho K, Castelletti S, Bulluck H, Rosmini S, et al. Left Ventricular hypertrophy revisited: cell and matrix expansion have disease-specific relationships. Circulation. (2017) 136:2519–21. doi: 10.1161/CIRCULATIONAHA.117.029895
24. Wilson M, O'Hanlon R, Prasad S, Deighan A, Macmillan P, Oxborough D, et al. Diverse patterns of myocardial fibrosis in lifelong, veteran endurance athletes. J Appl Physiol (1985). (2011) 110:1622–6. doi: 10.1152/japplphysiol.01280.2010
25. Breuckmann F, Mohlenkamp S, Nassenstein K, Lehmann N, Ladd S, Schmermund A, et al. Myocardial late gadolinium enhancement: prevalence, pattern, prognostic relevance in marathon runners. Radiology. (2009) 251:50–7. doi: 10.1148/radiol.2511081118
26. Malek LA, Barczuk-Falecka M, Werys K, Czajkowska A, Mroz A, Witek K, et al. Cardiovascular magnetic resonance with parametric mapping in long-term ultra-marathon runners. Eur J Radiol. (2019) 117:89–94. doi: 10.1016/j.ejrad.2019.06.001
27. McDiarmid AK, Swoboda PP, Erhayiem B, Lancaster RE, Lyall GK, Broadbent DA, et al. Athletic cardiac adaptation in males is a consequence of elevated myocyte mass. Circ Cardiovasc Imaging. (2016) 9:e003579. doi: 10.1161/CIRCIMAGING.115.003579
28. Pujadas S, Donate M, Li CH, Merchan S, Cabanillas A, Alomar X, et al. Myocardial remodelling and tissue characterisation by cardiovascular magnetic resonance (CMR) in endurance athletes. BMJ Open Sport Exerc Med. (2018) 4:e000422. doi: 10.1136/bmjsem-2018-000422
29. Sanchis-Gomar F, Lopez-Ramon M, Alis R, Garatachea N, Pareja-Galeano H, Santos-Lozano A, et al. No evidence of adverse cardiac remodeling in former elite endurance athletes. Int J Cardiol. (2016) 222:171–7. doi: 10.1016/j.ijcard.2016.07.197
30. Swoboda PP, McDiarmid AK, Erhayiem B, Broadbent DA, Dobson LE, Garg P, et al. Assessing myocardial extracellular volume by T1 mapping to distinguish hypertrophic cardiomyopathy from athlete's heart. J Am Coll Cardiol. (2016) 67:2189–90. doi: 10.1016/j.jacc.2016.02.054
31. Tahir E, Starekova J, Muellerleile K, von Stritzky A, Munch J, Avanesov M, et al. Myocardial fibrosis in competitive triathletes detected by contrast-enhanced CMR correlates with exercise-induced hypertension and competition history. JACC Cardiovasc Imaging. (2018) 11:1260–70. doi: 10.1016/j.jcmg.2017.09.016
32. Parry-Williams G, Gati S, Sharma S. The heart of the ageing endurance athlete: the role of chronic coronary stress. Eur Heart J. (2021) 42:2737–44. doi: 10.1093/eurheartj/ehab095
Keywords: athletes, myocardial fibrosis, LGE, CMR, meta-analysis, mapping
Citation: Androulakis E, Mouselimis D, Tsarouchas A, Antonopoulos A, Bakogiannis C, Papagkikas P and Vlachopoulos C (2021) The Role of Cardiovascular Magnetic Resonance Imaging in the Assessment of Myocardial Fibrosis in Young and Veteran Athletes: Insights From a Meta-Analysis. Front. Cardiovasc. Med. 8:784474. doi: 10.3389/fcvm.2021.784474
Received: 27 September 2021; Accepted: 30 November 2021;
Published: 21 December 2021.
Edited by:
Thomas A. Treibel, University College London, United KingdomReviewed by:
Stephen C. Kolwicz Jr., Ursinus College, United StatesMariana Vasconcelos, São João University Hospital Center, Portugal
Copyright © 2021 Androulakis, Mouselimis, Tsarouchas, Antonopoulos, Bakogiannis, Papagkikas and Vlachopoulos. This is an open-access article distributed under the terms of the Creative Commons Attribution License (CC BY). The use, distribution or reproduction in other forums is permitted, provided the original author(s) and the copyright owner(s) are credited and that the original publication in this journal is cited, in accordance with accepted academic practice. No use, distribution or reproduction is permitted which does not comply with these terms.
*Correspondence: Emmanuel Androulakis, ZS5hbmRyb3VsYWtpc0ByYmh0Lm5ocy51aw==
†These authors have contributed equally to this work