- 1Department of Cardiology, Royal Prince Alfred Hospital, Sydney, NSW, Australia
- 2Central Clinical School, The University of Sydney School of Medicine, Sydney, NSW, Australia
- 3Charles Perkins Centre, Heart Research Institute, Sydney, NSW, Australia
- 4Heart Centre for Children, The Sydney Children's Hospital Network, Sydney, NSW, Australia
- 5The Children's Hospital at Westmead Clinical School, Sydney, NSW, Australia
- 6Department of Cardiology, The Royal Melbourne Hospital, Melbourne, VIC, Australia
- 7The University of Melbourne School of Medicine, Melbourne, VIC, Australia
- 8Murdoch Children's Research Institute, Melbourne, VIC, Australia
- 9Green Lane Paediatric and Congenital Cardiac Service, Starship Hospital, Auckland, New Zealand
- 10Paediatric Cardiac Service, Queensland Children's Hospital, Brisbane, QLD, Australia
- 11The University of Queensland School of Medicine, Brisbane, QLD, Australia
- 12Discipline of Exercise and Sports Science, Sydney School of Health Sciences, Faculty of Medicine and Health, The University of Sydney, Sydney, NSW, Australia
- 13The George Washington University School of Medicine and Health Sciences, Washington, DC, United States
- 14Division of Cardiovascular Surgery, Children's National Hospital, Washington, DC, United States
Background: People with a Fontan circulation usually have moderately impaired exercise performance, although a subset have high physical performance (“Super-Fontan”), which may represent a low-risk phenotype.
Methods: People with a “Super-Fontan” phenotype were defined as achieving normal exercise performance [≥80% predicted peak oxygen uptake (VO2) and work rate] during cardiopulmonary exercise testing (CPET) and were identified from the Australian and New Zealand Fontan Registry. A Fontan control group that included people with impaired exercise performance (<80% predicted VO2 or work rate) was also identified based on a 1:3 allocation ratio. A subset of participants were prospectively recruited and completed a series of physical activity, exercise self-efficacy, and health-related quality of life questionnaires.
Results: Sixty CPETs (“Super-Fontan”, n = 15; control, n = 45) were included. A subset (“Super-Fontan”, n = 10; control, n = 13) completed a series of questionnaires. Average age was 29 ± 8 years; 48% were males. Exercise capacity reflected by percent predicted VO2 was 67 ± 17% in the entire cohort. Compared to the “Super-Fontan” phenotype, age at Fontan completion was higher in controls (4.0 ± 2.9 vs. 7.2 ± 5.3 years, p = 0.002). Only one (7%) person in the “Super-Fontan” group had a dominant right ventricle compared to 15 (33%) controls (p = 0.043). None of those in the “Super-Fontan” group were obese, while almost a quarter (22%) of controls were obese based on body mass index (p = 0.046). Lung function abnormalities were less prevalent in the “Super-Fontan” group (20 vs. 70%, p = 0.006). Exercise self-efficacy was greater in the “Super-Fontan” group (34.2 ± 3.6 vs. 27.9 ± 7.2, p = 0.02). Self-reported sports participation and physical activity levels during childhood and early adulthood were higher in the “Super-Fontan” group (p < 0.05). The total average time spent participating in structured sports and physical activity was 4.3 ± 2.6 h/wk in the “Super-Fontan” group compared to 2.0 ± 3.0 h/wk in controls, p = 0.003. There were no differences in self-reported current total physical activity score or health-related quality of life between groups (p ≥ 0.05).
Conclusions: The “Super-Fontan” phenotype is associated with a healthy weight, lower age at Fontan completion, better exercise self-efficacy, and higher overall levels of sport and physical activity participation during physical development.
Introduction
Francis Fontan first described the Fontan procedure in 1971 as a surgical method to treat babies born with tricuspid atresia (1). The procedure involves redirecting venous return directly into the pulmonary arteries resulting in no subpulmonary pump. The Fontan procedure has evolved with the advancement of medicine and surgical techniques in an attempt to optimize long-term outcomes, and although clinical outcomes have improved significantly, rates of morbidity and premature death are still high.
Exercise intolerance is common in people living with a Fontan circulation. Peak oxygen uptake (VO2) is the primary index of exercise tolerance (i.e., exercise capacity) and has significant prognostic value in patients with congenital heart disease (2). People with a Fontan circulation have reduced percent predicted peak VO2, which on average ranges from 60 to 65% (3, 4). However, there is extensive variability between patients, and it is acknowledged that a subgroup—“Super-Fontans”—have superior exercise performance (exercise and work capacity) compared to the majority of the Fontan population (5).
Currently, there is limited information about this subset of people who have superior physical performance. Since we originally described this unique phenotype (5), other centers have also characterized a similar subset of Fontan patients with normal exercise capacity (6, 7). Importantly, higher exercise capacity in people with a Fontan circulation appears to be associated with better prognosis and end-organ function (7–9). Understanding the factors associated with normal exercise capacity in this unique subset of patients can potentially aid in risk stratification and the identification of therapeutic targets. The aim of this study was to characterize factors associated with superior exercise performance in people with the “Super-Fontan” phenotype.
Methods
People in the Australian and New Zealand Fontan Registry with recorded cardiopulmonary exercise testing (CPET) results and a “Super-Fontan” phenotype were identified and included in this study. People with impaired exercise capacity were also identified as controls based on a 1:3 allocation. Exercise and work capacity was measured by peak VO2 and work rate, respectively. To account for sex, height, and weight differences, peak VO2 and work rate are expressed as a percentage of predicted normal values (10, 11). Participants were categorized into a “Super-Fontan” (5) or a control group. The “Super-Fontan” group was defined as achieving normal exercise and work capacity (≥80% predicted) (5, 6, 12–15). The control group consisted of Fontan subjects who had reduced exercise or work capacity (<80% predicted).
We decided to include work capacity as a criterion as obese patients may have normal exercise capacity but present with limited work capacity and exercise intolerance. Participants were excluded if their CPET was conducted on a treadmill or if results were considered to be submaximal effort defined as a peak respiratory exchange ratio <1.0 (16). A subset of participants (study group) completed a series of health-related quality of life, physical activity, and exercise self-efficacy questionnaires. Clinical and demographic information, including dominant ventricular morphology, type of Fontan procedure, patent fenestration, sex, and age at Fontan completion, were obtained from the Australian and New Zealand Fontan Registry database and medical records when available. This study was approved by the Royal Children's Hospital Melbourne Human Research Ethics Committee (38,172).
Exercise Self-Efficacy and Quality of Life
Exercise self-efficacy was assessed using the Exercise Self-Efficacy Scale (17). The total score was calculated as the sum of all questions, with a higher score reflecting greater exercise self-efficacy, which assesses an individual's beliefs in their ability to continue exercising regularly.
Health-related quality of life was measured using the PedsQL Adult Quality of Life Inventory Version 4. The items of each question were reversed scored, and linearly transformed in accordance with the scoring guidelines. In addition to the total score, the physical health summary score and psychosocial health summary score were also calculated, with higher scores suggesting better health-related quality of life.
Cardiopulmonary Exercise Testing and Spirometry
Center specific CPET protocols were performed on an electronically braked cycle ergometer as part of routine clinical care. In addition to measures of peak VO2, work rate, and pre-exercise spirometry, CPET parameters including minute ventilation (VE), carbon dioxide production (VCO2), blood pressure, VO2 at the anaerobic threshold, heart rate (HR), and arterial oxygen saturation indicated by pulse oximetry were obtained when available. Predicted maximal oxygen pulse (ml/beat)—a surrogate for stroke volume—was calculated by dividing predicted maximal VO2 by predicted maximal HR (220–age) (18). A cardiovascular limitation to exercise performance was indicated by a chronotropic index (cardiovascular index) above the upper limit of normal (19) or a reduced peak oxygen pulse (<80% predicted). Chronotropic index was calculated as ΔHR (beats/min)/ΔVO2 (L/min) (19, 20). Maximal voluntary ventilation (MVV) was estimated as forced expiratory volume in 1 s (FEV1) x 40, and breathing reserve was calculated as MVV–peak VE or (MVV–peak VE)/MVV x 100. A mechanical ventilatory limitation to exercise was suggested by a breathing reserve of <15% or <11 L/min (18, 21). Peak circulatory power was calculated as peak VO2 (mL/kg/min) x peak systolic blood pressure (mm Hg). Ventilatory inefficiency was suggested by a peak VE/VCO2 ratio of >40. A significant fall in oxygen saturation was considered as a decrease of ≥5%.
Spirometry parameters were considered as abnormal if values were below the lower limit of normal calculated from the Global Lung Initiative regression equations (22). Lung function was defined as normal, obstruction, restriction, or mixed defect in accordance with the American Thoracic Society/European Respiratory Society algorithm (23). In the absence of total lung capacity measured by plethysmography, ventilatory restriction was suggested if forced vital capacity (FVC) was below the lower limit of normal. Mixed defect was suggested if the FEV1/FVC ratio and FVC were below the lower limit of normal.
Physical Activity Across the Lifespan
To assess structured sport and physical activity participation across the lifespan, we used a modified version of the Kriska long-term recall physical activity questionnaire (24). Participants were asked to recall the sports and physical activities they participated in across multiple age ranges. For each sport and physical activity reported, the years in each age range, duration (hours) per month, and months per year were recorded. Sports and physical activities were categorized as childhood (ages 4–12 years), high school and early adulthood (ages 13–21 years), older adulthood (ages 22+ years), and physical activity across the lifespan (four to the age at questionnaire completion). The total hours of sport or physical activity participation were summated for each category and indexed as an average per week (h/wk).
Current Level of Physical Activity
Self-reported current levels of physical activity and sedentary time were assessed using the International Physical Activity Questionnaire (IPAQ) Long-Form. The IPAQ scores were not truncated, and metabolic minutes per week (MET-min/week) were calculated in accordance with the IPAQ Scoring Manual. Sedentary activity was reflected by sitting time (minutes) per day.
Statistical Analysis
Statistical analysis was performed using IBM SPSS version 26 software (IBM Corp, Armonk, NY, USA). Data are presented as mean ± standard deviation or number (%) unless specified otherwise. The Shapiro-Wilk test or visual inspection of histograms and Q-Q plots were conducted to assess for normal distribution. An independent t-test or Mann–Whitney U was used as appropriate to compare differences between the “Super-Fontan” group and the control group. Proportions were compared using Pearson Chi-Square. A p-value of < 0.05 was considered as statistically significant.
Results
Participant Demographics
Detailed participant demographics and characteristics are shown in Table 1. Of the 60 people with a Fontan circulation included in the CPET analysis, 15 had a “Super-Fontan” phenotype, and 35 had impaired exercise performance. The average age was 28.7 ± 7.6 years, and 48% were males.
The average body mass index (BMI) was 25.9 kg/m2, and 20 people (33%) were overweight or obese. None who had the “Super-Fontan” phenotype were obese based on BMI compared to 22% in the control group (p = 0.046). The majority (70%) had a total cavopulmonary connection, and the average age at Fontan procedure was 6.4 ± 5.0 years. The age at Fontan procedure was lower in the “Super-Fontan” group compared to the control group (4.0 ± 2.9 vs. 7.2 ± 5.3 years, p = 0.002). Thirty-seven (62%) had a dominant left ventricle, 16 (27%) had a dominant right ventricle, 3 (5%) had biventricular morphology, and 4 (7%) had indeterminate ventricles. A dominant right ventricle was associated with impaired exercise performance (p = 0.043). One person (7%) in the “Super-Fontan” group had a Fontan conversion from an atriopulmonary connection to an extracardiac conduit type circulation. There were no statistically significant differences in age, sex, BMI, type of Fontan procedure, or patent fenestration between groups (p ≥ 0.05 for all).
Twenty-three Fontan participants completed the questionnaires. Of the 23 Fontan study group participants, 10 (43%) were in the “Super-Fontan” group, and 13 (57%) were in the control group. There were no differences between groups in baseline demographics for the subset of study participants who completed the questionnaires (p ≥ 0.05 for all).
Exercise Self-Efficacy and Health-Related Quality of Life
The average total exercise self-efficacy score was higher in the “Super-Fontan” group compared to the control group (34.2 ± 3.6 vs. 27.9 ± 7.2, p = 0.02).
There was no statistically significant difference in total health-related quality of life score between the “Super-Fontan” group and the control group (78.9 ± 13.0 vs. 68.2 ± 18.8, p = 0.14). There was also no statistically significant difference between the “Super-Fontan” and control groups in the physical health summary score (80.3 ± 11.7 vs. 67.1 ± 21.9, p = 0.1) or psychosocial health summary score (78.2 ± 15.4 vs. 68.8 ± 19.6, p = 0.23).
Cardiopulmonary Exercise Testing and Spirometry
Detailed CPET and spirometry results are shown in Tables 2, 3. In the study group, the average time from the CPET to the questionnaires was 2.1 ± 1.9 years, and there was no difference between groups (p = 0.5).
The average percent predicted peak VO2 and work rate for the entire cohort was 67 ± 17% and 72 ± 22%, respectively. There was no statistically significant difference in percent predicted maximum HR between the “Super-Fontan” and control groups (83 ± 9% vs. 76 ± 15%, p = 0.09). Peak circulatory power, HR reserve (HRR), peak VE, and VO2 at anaerobic threshold (percentage of predicted VO2) were higher in the “Super-Fontan” group (p < 0.05 for all). There was no difference in exercise-induced desaturation between groups (p = 0.5).
Of the 40 participants (“Super-Fontan,” n = 11; control, n = 29) where the chronotropic index could be calculated, 7 (18%) participants had values outside the normal range. All participants in the “Super-Fontan” group had a chronotropic index within the normal range. In the control group, 4 (14%) participants had a high chronotropic index, and 3 (10%) had a low chronotropic index suggesting cardiovascular limitation and chronotropic insufficiency as inhibitors to exercise performance, respectively. The “Super-Fontan” group also had a higher percent predicted oxygen pulse compared to the control group (109 ± 16% vs. 80 ± 20%, p < 0.001). When markers of cardiovascular limitation were combined [low peak oxygen pulse (<80% predicted) or a high chronotropic index], no patient with the “Super-Fontan” phenotype had evidence of a cardiovascular limitation to exercise capacity compared to 62% in the control group (p < 0.001).
Thirty-seven people with a Fontan circulation (“Super-Fontan,” n = 10; control, n = 27) had baseline spirometry recorded, 16 (43%) had normal spirometry function, 20 (54%) had evidence of ventilatory restriction, and 1 (3%) had a pattern suggestive of mixed defect. The “Super-Fontan” group tended to have higher percent predicted FVC compared to the control group (85 ± 8% vs. 78 ± 10%, p = 0.05). Lung function abnormalities at rest were associated with impaired exercise performance; 2 (20%) patients in the “Super-Fontan” group had ventilatory or mixed defect compared to 19 (70%) in the control group (p = 0.006).
The average breathing reserve was 36 ± 18%, and five out of the 37 patients had a mechanical ventilatory limitation to exercise performance. The majority (80%) who had a mechanical ventilatory limitation also had evidence of ventilatory restriction at rest.
Physical Activity Across the Lifespan
One of the participants in the control group had an incomplete questionnaire and was excluded from the analysis. Results for the Kriska physical activity questionnaire are shown in Table 4; Figure 1. Childhood physical activity was higher in the “Super-Fontan” group compared to the control group (3.9 ± 3.3 h/wk vs. 2.0 ± 3.4 h/wk, p = 0.04). The average h/wk of sports and physical activity participation during high school and early adulthood was 5.2 ± 4.4 h/wk in the “Super-Fontan” group and 2.1 ± 3.1 h/wk in the control group, p = 0.04. There was no statistically significant difference in sport and physical activity participation during older adulthood. The overall average duration of sport and physical activity participation indexed per week was higher in the “Super-Fontan” group compared to the control group (4.3 ± 2.6 h/wk vs. 2.0 ± 3.0 h/wk, p = 0.003).
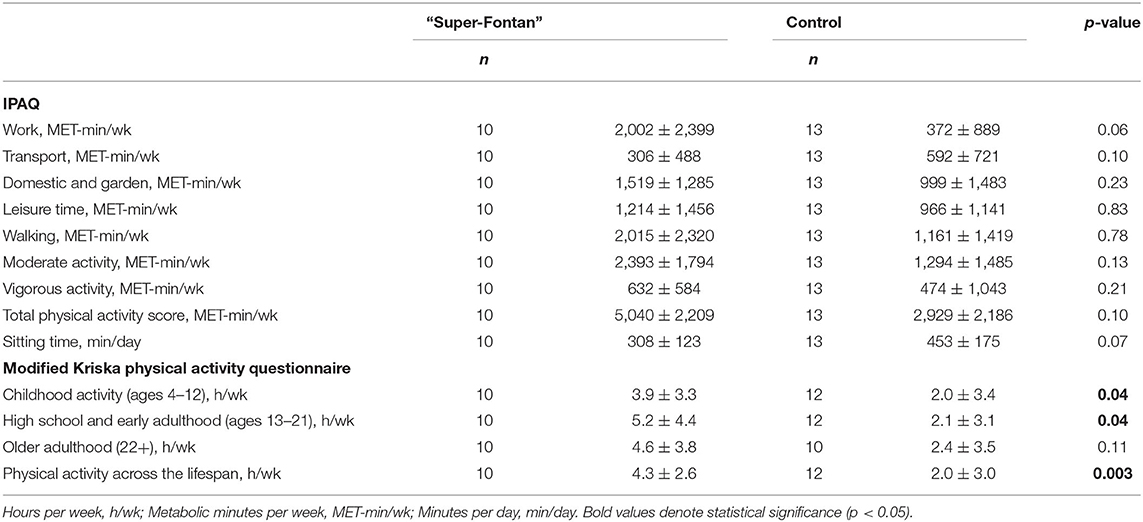
Table 4. The international physical activity questionnaire (IPAQ) and modified Kirska questionnaire results.
Current Physical Activity Levels
The detailed IPAQ results are shown in Table 4. The average self-reported MET-min/wk was higher at all physical activity intensities and sub-domains, except for the transport domain in the “Super-Fontan” group, although this did not achieve statistical significance (Figure 2). Sitting time tended to be lower in the “Super-Fontan” group compared to the control group (308 ± 123 vs. 453 ± 175 min/day, p = 0.07).
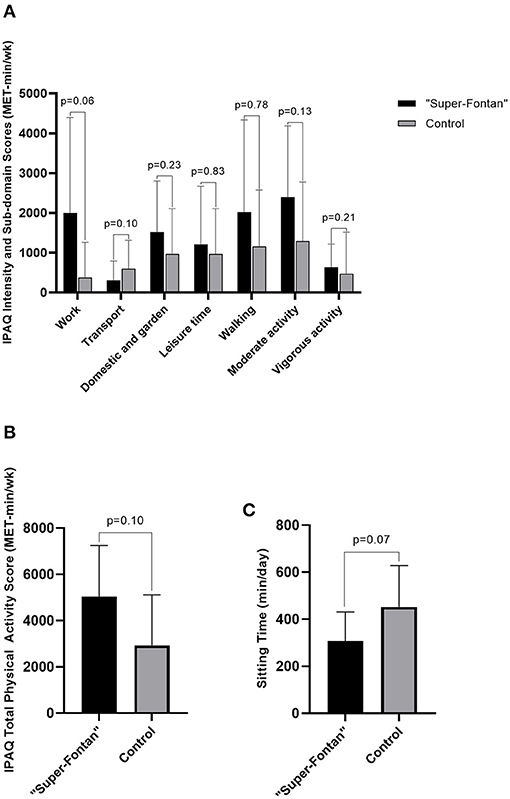
Figure 2. International physical activity questionnaire (IPAQ) scores. (A) IPAQ intensity and sub-domain scores, (B) IPAQ total physical activity score, (C) Sitting time. Metabolic minutes per week (MET-min/wk); minutes per day (min/day).
Discussion
Despite an absent subpulmonary ventricle in the Fontan circulation, a subset of the population (“Super-Fontans”) can still achieve normal exercise performance, which is associated with increased levels of physical activity early in life, a healthy weight, and earlier age at Fontan completion.
Factors Associated With Superior Physical Performance
In this study, the age at Fontan completion was lower in those with a “Super-Fontan” phenotype, and the absence of obesity or a dominant right ventricle were associated with normal exercise performance. This contrasts with previous findings that showed no differences between exercise performance Fontan phenotypes with these factors (6, 7). Our study describes an older Fontan cohort compared to previous series, and the conflicting findings might be attributed to an era effect. Alternatively, later age at Fontan completion, dominant right ventricular morphology, and obesity may manifest as important factors that impair the ability to achieve “normal” exercise performance later in life when circulatory function is more susceptible to compromise and maladaptation.
Similar to our findings, a review by Daley et al. found that earlier age at Fontan completion is associated with preserved long-term exercise capacity (25). A large multi-center series in a contemporary Fontan cohort also corroborates this; each year Fontan completion was delayed, percent predicted VO2 and HRR decreased by 1.5 percentage points and 4.1 beats/min, respectively (26). This association may be explained by enhanced reversal of adverse cardiac remodeling and offsetting volume overload with earlier age at Fontan completion (27, 28). Indeed, patients with a later age at Fontan completion present with evidence of greater ventricular dysfunction and atrioventricular valve insufficiency (29). Conversely, one study found a positive correlation between age at Fontan completion, and percent predicted peak VO2 (30). Although later age at Fontan completion is accompanied by an extended period of volume overload and cyanosis, it may allow for pulmonary artery catch-up growth and a larger conduit to optimize flow (31, 32); however, this theory requires verification.
We have previously reported increased adiposity is associated with a higher risk of adverse outcomes in people with a Fontan circulation (33). None of the people with the “Super-Fontan” phenotype were obese based on BMI compared to 22% in the comparator group with impaired exercise performance. The latest Pediatric Heart Network results also support this finding (34). This may be related to the impact of excess adiposity on respiratory muscle pump function and increased mechanical loading, which may impair exercise performance. In addition, the importance of maintaining a healthy body weight to preserve low pulmonary vascular resistance is increasingly recognized (32). Importantly, higher BMI often co-exists with increased visceral adiposity (epicardial and intra-abdominal fat), which may be particularly pathological—visceral adiposity is positively associated with pulmonary vascular resistance and inversely associated with ejection fraction and cardiac index in the Fontan circulation (35, 36). Of course, BMI may not be a robust measure of adiposity in the setting of complex congenital heart disease, where myopenia is common (33, 37, 38). The association between adiposity and pulmonary vascular resistance may be attributed to the adverse effects of pro-inflammatory adipokines (39), co-existing obstructive sleep apnea (40, 41), or decreased adiponectin (39, 42). The mechanisms underlying the association between adiposity and Fontan hemodynamics warrant further investigation.
We showed that an absence of a dominant right ventricle was associated with the “Super-Fontan” phenotype. A linear association between ventricular morphology and exercise capacity has previously been reported (43, 44). However, when categorized into exercise performance phenotypes (i.e., normal exercise performance vs. impaired exercise performance), series from Cincinnati Children's Hospital and the Children's Hospital in Philadelphia (a younger population than ours) found no association between ventricular morphology and a superior exercise performance phenotype (6, 7). It would seem plausible that a systemic right ventricle (compared to a systemic left ventricle) would be less likely to adapt to progressive hemodynamic perturbations over time and become more susceptible to circulatory demise and exercise intolerance. Long-term follow-up studies show dominant right ventricular morphology to be associated with worse clinical outcomes (45, 46).
We did not find any differences with regard to type of Fontan circulation, fenestration patency, or sex between the “Super-Fontan” phenotype and those with impaired exercise performance. However, although not statistically significant, there was a higher percentage of females in the “Super-Fontan” group compared to those with impaired exercise performance. Previous studies have also shown that a higher proportion of females are able to achieve better exercise performance (6, 7, 34), and have superior long term-outcomes (47, 48). The mechanisms underlying these sex differences are unclear and warrant further investigation.
Of note, while not statistically significant, and there were no differences in oxygen saturation, 20% of people with impaired exercise performance had a patent fenestration. It is unknown if this is associated with institutional bias toward fenestration or if these patients reflect a higher risk cohort requiring a fenestration at Fontan completion.
There were also no statistically significant differences in health-related quality of life measures between the “Super-Fontan” and control group in this study. However, the associations between quality of life and exercise performance are inconsistent across studies and warrant further investigation (49–51). This may be related to adults with a Fontan circulation accommodating to exercise intolerance over time. Supporting this notion, even asymptomatic people with congenital heart disease (New York Heart Association Functional Class I) have objectively impaired exercise capacity (2). Furthermore, the benefits of physical activity and exercise training on quality of life (particularly in the psychosocial domains) are potentially related to the social engagement that accompanies participation rather than better physical function.
Exercise Response and Lung Function
HRR was greater in those with the “Super-Fontan” phenotype compared to the control group. Importantly, diminished HRR may be associated with arrhythmia-related mortality (9). This may explain why the combination of peak VO2 and HRR has a more substantial prognostic value for 5-year survival (52), with peak VO2 likely associated with heart-failure-related mortality. We also found higher peak circulatory power on average in people with the “Super-Fontan” phenotype, which is associated with better outcomes (53, 54). Collectively, the “Super-Fontan” exercise response reflects a low-risk Fontan phenotype.
Overall, most of our Fontan cohort had normal range chronotropic index implying an appropriate HR response relative to the metabolic load. Only three patients had a low chronotropic index with impaired exercise capacity indicating chronotropic insufficiency—It has been postulated that an inappropriate HR response is not a primary limiting factor to exercise performance unless severe chronotropic incompetence is present (55, 56). Exercise performance in the Fontan circulation appears predominately limited by preload deprivation that inhibits stroke volume augmentation. Over half of our Fontan patients with impaired peak VO2 show evidence of a cardiovascular (stroke volume) limitation to exercise performance denoted by an elevated chronotropic response or low peak oxygen pulse. Of course, the reduced oxygen pulse and elevated chronotropic index can also reflect impaired peripheral muscle oxygen extraction, which is also present in the Fontan circulation (57).
Lung function is commonly impaired in people with a Fontan circulation and is associated with prognosis and exercise capacity (58–60). The majority of our Fontan cohort with baseline spirometry results recorded had evidence of ventilatory restriction or mixed defect. Baseline spirometry abnormalities were less prevalent in the “Super-Fontan” phenotype compared to those with impaired exercise performance. However, despite abnormal lung function at rest, most patients in this study had ample breathing reserve, potentially because exercise performance in Fontan patients is predominantly impaired by cardiovascular limitations prior to encroaching upon ventilatory constraints. Only five people had evidence of mechanical ventilatory limitation during exercise, with no difference between the “Super-Fontan” and control groups. Of note, it is likely ventilatory limitations to exercise performance is underappreciated using breathing reserve in isolation as a surrogate. The addition of exercise flow-volume loops will perhaps reveal more Fontan patients with ventilatory limitations to exercise performance.
Exercise Self-Efficacy and Physical Activity
Although self-reported physical activity levels were higher and sedentary (sitting) time was lower in the “Super-Fontan” group compared to the controls, this did not achieve statistical significance. This may be attributed to the duration between CPET and the administration of the questionnaires (~2 years), which likely does not truly reflect their current levels of physical activity. Furthermore, while the IPAQ shows moderate validity compared to accelerometers (61), in the setting of congenital heart disease, patients often overestimate their physical activity levels using the IPAQ long-form (62).
Indeed, Powell et al. reported that 77% of patients with the “Super-Fontan” phenotype participated in regular physical activity compared to 10% in those with impaired exercise performance (6). This is in accordance with our previous reports that show many of those with a “Super-Fontan” phenotype or positive exercise capacity trajectory regularly participate in moderate-to-vigorous sports and physical activity (5, 63). Importantly, increased physical activity levels could be attributed to higher exercise self-efficacy in the “Super-Fontan” cohort. Preceding studies have shown an association between physical activity levels and exercise self-efficacy (64).
We found that participation in regular structured sports and physical activity from childhood to early adulthood was significantly higher in those with a “Super-Fontan” phenotype compared to those with impaired exercise performance. Total overall participation in sports and physical activity was also higher in the “Super-Fontan” group. While exercise training interventions can increase peak VO2 (65, 66), it appears that participation in regular sports and physical activity from a younger age lays the foundation to achieve a low-risk “Super-Fontan” phenotype. This is consistent with the findings of Ohuchi et al. who showed that increased physical activity levels during childhood—reflected by a positive exercise capacity trajectory—were associated with better adult Fontan physiology, hepatic function, and prognosis (67). In another series, children and adolescents with a Fontan circulation who participated in sports during middle and high school had better lung function and exercise capacity (68). Regular sports, exercise training, or physical activity may be particularly crucial during childhood when organs, and especially the pulmonary vasculature, are likely more sensitive to adaptation in this period of rapid growth and development (67).
The association with regular moderate-to-vigorous physical activity participation and the “Super-Fontan”—superior exercise performance and low-risk—phenotypical expression may be attributed to multiple mechanisms. Regular participation in moderate-to-vigorous intensity sports and physical activity is important for the development of skeletal muscle mass and prevention of Fontan-associated myopenia (37). Deficiencies in skeletal muscle mass have important implications for ventricular function (37), and exercise capacity (57, 69). Higher appendicular muscle mass provides structural support to reduce venous compliance and enhances skeletal muscle pump function, facilitating preload and ventricular filling (70, 71). Indeed, increasing skeletal muscle mass through resistance exercise training improves ventricular filling, cardiac output, peak VO2, and reduces respiratory dependence in people with a Fontan circulation (72).
A primary constraint to ventricular filling appears to be elevated pulmonary vascular resistance. To maintain low pulmonary vascular resistance, there must be an adequately developed pulmonary circulation. However, pulmonary artery growth essentially ceases after Fontan completion (73), likely due to the combination of reduced pulsatility and pulmonary flow. We have previously shown that lower limb exercise can transiently increase (pulsatile) pulmonary flow (74). It is likely that engaging in regular long-term physical activity or exercise training (particularly during childhood), which transiently increases pulmonary flow (and may also alter the flow profile), facilitates pulmonary vascular development. Furthermore, periodic increases in ventricular filling associated with exercise may augment volume load to the chronically preload deprived ventricle and attenuate progressive “disuse hypofunction”. This phenomenon is observed when volume load is restored following atrial septal defect closure in adults, reversing diastolic dysfunction (31).
Of course, the association between the “Super-Fontan” phenotype and physical activity during childhood, and early adulthood, may simply reflect that those with superior exercise performance are more capable of participating in regular sports and physical activity, especially from an earlier age.
Clinical Implications and Future Directions
While there appear to be common characteristics associated with the “Super-Fontan” phenotype, some patients in this subset still have features—such as a dominant right ventricle, atriopulmonary connection, or patent fenestration—that are expected to impede exercise performance. This suggests that extracardiac and potentially modifiable factors contribute to the expression of the “Super-Fontan” phenotype.
A key finding of our study is that exercise-self efficacy and regular participation in structured sports and physical activity from a young age is significantly higher in those with the “Super-Fontan” phenotype. This highlights the need to promote exercise training, sports, and physical activity in people with a Fontan circulation from early in life. Those who participated in sports from a young age probably also have higher exercise self-efficacy, which establishes a foundation for life-long physical activity habits. To date, exercise training is the most effective therapy for improving peak VO2 in people who have a Fontan circulation (70). While exercise training recommendations are now available for people with a Fontan circulation (65, 66, 75), they are predominantly based on clinical experience and expert opinion.
The forthcoming multi-center randomized controlled Fontan Fitness Intervention Trial (F-FIT) will hopefully provide more conclusive evidence to aid the development of future exercise training recommendations for people living with a Fontan circulation.
Limitations
It is important to note that the retrospective design of this study can only show association and not causation. A key limitation in this study is the reliability of long-term physical activity questionnaires, which is subject to recall error. However, the reliability of long-term physical activity recall appears to be reasonable, with a previous study reporting an intraclass correlation coefficient of ~0.4 (76).
CPET is a specialized assessment that requires a high degree of clinical expertise to perform and interpret. This may restrict the results of this study to patients in the care of expert centers, which are predominately located in major cities. Indeed, we previously reported that <8% of people in the Australian and New Zealand Fontan Registry had recorded serial CPET documented (63). Therefore, our sample may be subjected to selection bias. The spirometry and CPET data were also derived from the tabulated reports available, and we had limited access to the flow-volume curves or primary CPET data. This restricted our ability to visually inspect the acceptability and repeatability of spirometry maneuvers, verify anaerobic threshold selection, or standardize the processing of CPET parameters. However, the spirometry and CPET studies were predominantly performed in “expert” experienced centers, and our results reflect the available reports used in clinical practice.
Our study is also limited in sample size, which increases the risk of a type II error, and we may not be able to detect some important associations.
Ideally, the function of the single ventricle and “Super-Fontan” status should be evaluated during upright exercise with invasive haemodynamic measures, but this is technically challenging. Currently, we and others have defined the “Super-Fontan” phenotype as achieving ≥80% predicted VO2 and/or work rate (5–7, 15), which can be influenced by the regression prediction equation selected and remains an arbitrary threshold.
Conclusions
The “Super-Fontan” phenotype is associated with a healthy weight, younger age of Fontan completion (around 4 years), and higher overall levels of sport and physical activity participation during childhood and early adulthood. The “Super-Fontan” phenotype exercise response was accompanied by a higher HRR, oxygen pulse, peak circulatory power, and a later anaerobic threshold onset.
Data Availability Statement
The original contributions presented in the study are included in the article/supplementary material, further inquiries can be directed to the corresponding author/s.
Ethics Statement
The studies involving human participants were reviewed and approved by the Royal Children's Hospital Melbourne Human Research Ethics Committee. The patients/participants provided their written informed consent to participate in this study.
Author Contributions
DT, Yd'U, DC, and RC contributed to the conception and design of the study. DT drafted the manuscript and acquired and analyzed the data. All authors critically reviewed the manuscript, contributed to the interpretation of the results, and approved the submission of the manuscript.
Funding
DT was supported by a Grant from Additional Ventures, the National Heart Foundation of Australia Vanguard Grant (102277), the Medical Research Future Fund–Cardiovascular Health Mission–Congenital Heart Disease Grant (ARGCHDG000016), and the Paulette Isabel Jones PhD Completion Scholarship.
Conflict of Interest
The authors declare that the research was conducted in the absence of any commercial or financial relationships that could be construed as a potential conflict of interest.
Publisher's Note
All claims expressed in this article are solely those of the authors and do not necessarily represent those of their affiliated organizations, or those of the publisher, the editors and the reviewers. Any product that may be evaluated in this article, or claim that may be made by its manufacturer, is not guaranteed or endorsed by the publisher.
Acknowledgments
We are grateful for the assistance of Karin du Plessis and Nimani Fernando for their help with identifying potential participants.
References
1. Fontan F, Baudet E. Surgical repair of tricuspid atresia. Thorax. (1971) 26:240–8. doi: 10.1136/thx.26.3.240
2. Diller GP, Dimopoulos K, Okonko D, Li W, Babu-Narayan SV, Broberg CS, et al. Exercise intolerance in adult congenital heart disease: comparative severity, correlates, and prognostic implication. Circulation. (2005) 112:828–35. doi: 10.1161/CIRCULATIONAHA.104.529800
3. Muller J, Christov F, Schreiber C, Hess J, Hager A. Exercise capacity, quality of life, and daily activity in the long-term follow-up of patients with univentricular heart and total cavopulmonary connection. Eur Heart J. (2009) 30:2915–20. doi: 10.1093/eurheartj/ehp305
4. Paridon SM, Mitchell PD, Colan SD, Williams RV, Blaufox A, Li JS, et al. A cross-sectional study of exercise performance during the first 2 decades of life after the fontan operation. J Am Coll Cardiol. (2008) 52:99–107. doi: 10.1016/j.jacc.2008.02.081
5. Cordina R, du Plessis K, Tran D, D'Udekem Y. Super-Fontan: is it possible? J Thorac Cardiovasc Surg. (2018) 155:1192–4. doi: 10.1016/j.jtcvs.2017.10.047
6. Powell AW, Chin C, Alsaied T, Rossiter HB, Wittekind S, Mays WA, et al. The unique clinical phenotype and exercise adaptation of fontan patients with normal exercise capacity. Can J Cardiol. (2020) 36:1499–507. doi: 10.1016/j.cjca.2019.11.006
7. Weinreb SJ, Dodds KM, Burstein DS, Huang J, Rand EB, Mancilla E, et al. End-Organ function and exercise performance in patients with fontan circulation: what characterizes the high performers? J Am Heart Assoc. (2020) 9:E016850. doi: 10.1161/JAHA.120.016850
8. Ohuchi H, Negishi J, Noritake K, Hayama Y, Sakaguchi H, Miyazaki A, et al. Prognostic value of exercise variables in 335 patients after the fontan operation: a 23-year single-center experience of cardiopulmonary exercise testing. Congenit Heart Dis. (2015) 10:105–16. doi: 10.1111/chd.12222
9. Diller GP, Giardini A, Dimopoulos K, Gargiulo G, Muller J, Derrick G, et al. Predictors of morbidity and mortality in contemporary fontan patients: results from a multicenter study including cardiopulmonary exercise testing in 321 patients. Eur Heart J. (2010) 31:3073–83. doi: 10.1093/eurheartj/ehq356
10. Jones NL, Makrides L, Hitchcock C, Chypchar T, McCartney N. Normal standards for an incremental progressive cycle ergometer test. Am Rev Respir Dis. (1985) 131:700–8.
12. Guazzi M, Bandera F, Ozemek C, Systrom D, Arena R. Cardiopulmonary exercise testing: what is its value? J Am Coll Cardiol. (2017) 70:1618–36. doi: 10.1016/j.jacc.2017.08.012
13. Puente-Maestu L. Reference values in adults. In: Ward SA, Palange P, editors. Clinical Exercise Testing. European Respiratory Monograph (2007). p. 165–85.
14. Levett DZH, Jack S, Swart M, Carlisle J, Wilson J, Snowden C, et al. Perioperative cardiopulmonary exercise testing (CPET): consensus clinical guidelines on indications, organization, conduct, and physiological interpretation. Br J Anaesth. (2018) 120:484–500. doi: 10.1016/j.bja.2017.10.020
15. Alsaied T, Rathod RH, Aboulhosn JA, Budts W, Anderson JB, Baumgartner H, et al. Reaching consensus for unified medical language in fontan care. ESC Heart Failure. (2021) 8:3894–905. doi: 10.1002/ehf2.13294
16. Del Buono MG, Arena R, Borlaug BA, Carbone S, Canada JM, Kirkman DL, et al. Exercise intolerance in patients with heart failure: JACC state-of-the-art review. J Am Coll Cardiol. (2019) 73:2209–25. doi: 10.1016/j.jacc.2019.01.072
17. Kroll T, Kehn M, Ho P-S, Groah S. The SCI exercise self-efficacy scale (ESES): development and psychometric properties. Int J Behav Nutr Phys Act. (2007) 4:34. doi: 10.1186/1479-5868-4-34
18. Sietsema KE, Stringer WW, Sue DY, Ward S. Wasserman & Whipp's: Principles of Exercise Testing and Interpretation: Including Pathophysiology and Clinical Applications. Philadelphia: Lippincott Williams & Wilkins (2020).
19. Sirichana W, Neufeld EV, Wang X, Hu SB, Dolezal BA, Cooper CB. Reference values for chronotropic index from (1280) incremental cycle ergometry tests. Med Sci Sports Exerc. (2020) 52:2515–21. doi: 10.1249/MSS.0000000000002417
20. Neder JA, Nery LE, Peres C, Whipp BJ. Reference values for dynamic responses to incremental cycle ergometry in males and females aged 20 to 80. Am J Respir Crit Care Med. (2001). 164:1481–6. doi: 10.1164/ajrccm.164.8.2103007
21. Arena R, Sietsema KE. Cardiopulmonary exercise testing in the clinical evaluation of patients with heart and lung disease. Circulation. (2011) 123:668–80. doi: 10.1161/CIRCULATIONAHA.109.914788
22. Quanjer PH, Stanojevic S, Cole TJ, Baur X, Hall GL, Culver BH, et al. Multi-ethnic reference values for spirometry for the 3-95-yr age range: the global lung function 2012 equations. Eur Respir J. (2012) 40:1324–43. doi: 10.1183/09031936.00080312
23. Pellegrino R, Viegi G, Brusasco V, Crapo RO, Burgos F, Casaburi R, et al. Interpretative strategies for lung function tests. Eur Respir J. (2005) 26:948–68. doi: 10.1183/09031936.05.00035205
24. Kriska AM, Sandler RB, Cauley JA, LaPorte RE, Hom DL, Pambianco G. The assessment of historical physical activity and its relation to adult bone parameters. Am J Epidemiol. (1988) 127:1053–63. doi: 10.1093/oxfordjournals.aje.a114881
25. Daley M, D'Udekem Y. In patients undergoing fontan completion, does a younger age at operation result in better long-term exercise capacity and prognosis? Interact Cardiovasc Thorac Surg. (2018) 28:301–5. doi: 10.1093/icvts/ivy219
26. Madan P, Stout KK, Fitzpatrick AL. Age at fontan procedure impacts exercise performance in adolescents: results from the pediatric heart network multicenter study. Am Heart J. (2013) 166:365–72 e1. doi: 10.1016/j.ahj.2013.04.013
27. Sluysmans T, Sanders SP, Velde Mvd, Matitiau A, Parness IA, Spevak PJ, et al. Natural history and patterns of recovery of contractile function in single left ventricle after fontan operation. Circulation. (1992) 86:1753–61. doi: 10.1161/01.CIR.86.6.1753
28. Uemura H, Yagihara T, Kawashima Y, Yamamoto F, Nishigaki K, Matsuki O, et al. What factors affect ventricular performance after a fontan-type operation? J Thorac Cardiovasc Surg. (1995) 110:405–15. doi: 10.1016/S0022-5223(95)70237-7
29. Anderson PAW, Sleeper LA, Mahony L, Colan SD, Atz AM, Breitbart RE, et al. Contemporary outcomes after the fontan procedure: a pediatric heart network multicenter study. J Am Coll Cardiol. (2008) 52:85–98. doi: 10.1016/j.jacc.2008.01.074
30. Bolin EH, Maskatia SA, Tate AL, Petit CJ. Older age at completion of fontan procedure is associated with improved percentage of predicted maximum oxygen uptake. Tex Heart Inst J. (2015) 42:333–40. doi: 10.14503/THIJ-14-4178
31. Gewillig M, Brown SC. The fontan circulation after 45 years: update in physiology. Heart. (2016) 102:1081–6. doi: 10.1136/heartjnl-2015-307467
32. Gewillig M, Brown SC, van de Bruaene A, Rychik J. Providing a framework of principles for conceptualising the fontan circulation. Acta Paediatrica. (2020) 109:651–8. doi: 10.1111/apa.15098
33. Cao JY, Tran D, Briody J, Attard C, Hassan EB, Simm P, et al. Impact of adiposity on clinical outcomes in people living with a fontan circulation. Int J Cardiol. (2021) 329:82–8. doi: 10.1016/j.ijcard.2020.12.066
34. Goldberg DJ, Zak V, McCrindle BW, Ni H, Gongwer R, Rhodes J, et al. Exercise capacity and predictors of performance after fontan: results from the pediatric heart network fontan 3 study. Pediatr Cardiol. (2021) 42:158–68. doi: 10.1007/s00246-020-02465-1
35. Lubert AM, Lu JC, Rocchini AP, Norris MD, Yu S, Agarwal PP, et al. Relation of increased epicardial fat after fontan palliation to cardiac output and systemic ventricular ejection fraction. Am J Cardiol. (2018) 121:862–6. doi: 10.1016/j.amjcard.2017.12.026
36. Lubert AM, Alsaied T, Trout AT, Dillman JR, Goldstein BH. Relation of visceral fat and haemodynamics in adults with fontan circulation. Cardiol Young. (2020) 30:995–1000. doi: 10.1017/S1047951120001419
37. Tran D, D'Ambrosio P, Verrall CE, Attard C, Briody J, D'Souza M, et al. Body composition in young adults living with a fontan circulation: the myopenic profile. J Am Heart Assoc. (2020) 9:E015639. doi: 10.1161/JAHA.119.015639
38. Sandberg C, Johansson K, Christersson C, Hlebowicz J, Thilen U, Johansson B. Sarcopenia is common in adults with complex congenital heart disease. Int J Cardiol. (2019) 296:57–62. doi: 10.1016/j.ijcard.2019.06.011
39. Summer R, Walsh K, Medoff BD. Obesity and pulmonary arterial hypertension: is adiponectin the molecular link between these conditions? Pulm Circ. (2011) 1:440–7. doi: 10.4103/2045-8932.93542
40. Watson NF, Bushnell T, Jones TK, Stout K. A novel method for the evaluation and treatment of obstructive sleep apnea in four adults with complex congenital heart disease and fontan repairs. Sleep Breath. (2009) 13:421–4. doi: 10.1007/s11325-009-0260-8
41. Krimly A, Jain CC, Egbe A, Alzahrani A, Al Najashi K, Albert-Brotons D, et al. The pulmonary vascular bed in patients with functionally univentricular physiology and a fontan circulation. Cardiol Young. (2021) 31:1241–50. doi: 10.1017/S104795112100192X
42. Medoff BD. Fat, fire and muscle–the role of adiponectin in pulmonary vascular inflammation and remodeling. Pulmonary Pharmacology & Therapeutics. (2013) 26:420–6. doi: 10.1016/j.pupt.2012.06.006
43. Ohuchi H, Yasuda K, Hasegawa S, Miyazaki A, Takamuro M, Yamada O, et al. Influence of ventricular morphology on aerobic exercise capacity in patients after the fontan operation. J Am Coll Cardiol. (2001) 37:1967–74. doi: 10.1016/S0735-1097(01)01266-9
44. Giardini A, Hager A, Pace Napoleone C, Picchio FM. Natural history of exercise capacity after the fontan operation: a longitudinal study. Ann Thorac Surg. (2008) 85:818–21. doi: 10.1016/j.athoracsur.2007.11.009
45. Khairy P, Fernandes SM, Mayer JE, Triedman JK, Walsh EP, Lock JE, et al. Long-Term survival, modes of death, and predictors of mortality in patients with fontan surgery. Circulation. (2008) 117:85–92. doi: 10.1161/CIRCULATIONAHA.107.738559
46. Oster ME, Knight JH, Suthar D, Amin O, Kochilas LK. Long-Term outcomes in single-ventricle congenital heart disease. Circulation. (2018) 138:2718–20. doi: 10.1161/CIRCULATIONAHA.118.036821
47. D'Udekem Y, Iyengar AJ, Galati JC, Forsdick V, Weintraub RG, Wheaton GR, et al. Redefining expectations of long-term survival after the fontan procedure: twenty-five years of follow-up from the entire population of Australia and New Zealand. Circulation. (2014) 130 (11 Suppl 1):S32–8. doi: 10.1161/CIRCULATIONAHA.113.007764
48. Dennis M, Zannino D, du Plessis K, Bullock A, Disney PJS, Radford DJ, et al. Clinical outcomes in adolescents and adults after the fontan procedure. J Am Coll Cardiol. (2018) 71:1009–17. doi: 10.1016/j.jacc.2017.12.054
49. D'Udekem Y, Cheung MM, Setyapranata S, Iyengar AJ, Kelly P, Buckland N, et al. How good is a good fontan? quality of life and exercise capacity of fontans without arrhythmias. Ann Thorac Surg. (2009) 88:1961–9. doi: 10.1016/j.athoracsur.2009.07.079
50. Gratz A, Hess J, Hager A. Self-estimated physical functioning poorly predicts actual exercise capacity in adolescents and adults with congenital heart disease. Eur Heart J. (2009) 30:497–504. doi: 10.1093/eurheartj/ehn531
51. Hager A, Hess J. Comparison of health related quality of life with cardiopulmonary exercise testing in adolescents and adults with congenital heart disease. Heart. (2005) 91:517. doi: 10.1136/hrt.2003.032722
52. Inuzuka R, Diller G-P, Borgia F, Benson L, Tay ELW, Alonso-Gonzalez R, et al. Comprehensive use of cardiopulmonary exercise testing identifies adults with congenital heart disease at increased mortality risk in the medium term. Circulation. (2012) 125:250. doi: 10.1161/CIRCULATIONAHA.111.058719
53. Giardini A, Specchia S, Berton E, Sangiorgi D, Coutsoumbas G, Gargiulo G, et al. Strong and independent prognostic value of peak circulatory power in adults with congenital heart disease. Am Heart J. (2007) 154:441–7. doi: 10.1016/j.ahj.2007.05.009
54. Madan N, Beachler L, Konstantinopoulos P, Worley S, Sun Z, Latson LA. Peak circulatory power as an indicator of clinical status in children after fontan procedure. Pediatr Cardiol. (2010) 31:1203–8. doi: 10.1007/s00246-010-9799-1
55. Claessen G, La Gerche A, Van De Bruaene A, Claeys M, Willems R, Dymarkowski S, et al. Heart rate reserve in fontan patients: chronotropic incompetence or hemodynamic limitation? J Am Heart Assoc. (2019) 8:E012008. doi: 10.1161/JAHA.119.012008
56. Goldberg DJ, Avitabile CM, McBride MG, Paridon SM. Exercise capacity in the fontan circulation. Cardiol Young. (2013) 23:824–30. doi: 10.1017/S1047951113001649
57. Cordina R, O'Meagher S, Gould H, Rae C, Kemp G, Pasco JA, et al. Skeletal muscle abnormalities and exercise capacity in adults with a fontan circulation. Heart. (2013) 99:1530–4. doi: 10.1136/heartjnl-2013-304249
58. Alonso-Gonzalez R, Borgia F, Diller GP, Inuzuka R, Kempny A, Martinez-Naharro A, et al. Abnormal lung function in adults with congenital heart disease: prevalence, relation to cardiac anatomy, and association with survival. Circulation. (2013) 127:882–90. doi: 10.1161/CIRCULATIONAHA.112.126755
59. Turquetto ALR, Caneo LF, Agostinho DR, Oliveira PA, Lopes M, Trevizan PF, et al. Impaired pulmonary function is an additional potential mechanism for the reduction of functional capacity in clinically stable fontan patients. Pediatr Cardiol. (2017) 38:981–90. doi: 10.1007/s00246-017-1606-9
60. Callegari A, Neidenbach R, Milanesi O, Castaldi B, Christmann M, Ono M, et al. A restrictive ventilatory pattern is common in patients with univentricular heart after fontan palliation and associated with a reduced exercise capacity and quality of life. Congenit Heart Dis. (2019) 14:147–55. doi: 10.1111/chd.12694
61. Wanner M, Probst-Hensch N, Kriemler S, Meier F, Autenrieth C, Martin BW. Validation of the long international physical activity questionnaire: influence of age and language region. Prev Med Rep. (2016) 3:250–6. doi: 10.1016/j.pmedr.2016.03.003
62. Larsson L, Johansson B, Wadell K, Thilén U, Sandberg C. Adults with congenital heart disease overestimate their physical activity level. Int J Cardiol Heart Vasc. (2018) 22:13–7. doi: 10.1016/j.ijcha.2018.11.005
63. Tran DL, Rodrigues C, du Plessis K, Zannino D, Davis GM, Celermajer DS, et al. Decline is not inevitable: exercise capacity trajectory in an Australian and New Zealand fontan cohort. Heart Lung Circ. (2021) 30:1356–63. doi: 10.1016/j.hlc.2021.01.004
64. Acosta-Dighero R, Torres-Castro R, Rodríguez-Núñez I, Rosales-Fuentes J, Vilaró J, Fregonezi G, et al. Physical activity assessments in children with congenital heart disease: a systematic review. Acta Paediatrica. (2020) 109:2479–90. doi: 10.1111/apa.15478
65. Tran D, Maiorana A, Ayer J, Lubans DR, Davis GM, Celermajer DS, et al. Recommendations for exercise in adolescents and adults with congenital heart disease. Prog Cardiovasc Dis. (2020) 63:350–66. doi: 10.1016/j.pcad.2020.03.002
66. Tran DL, Maiorana A, Davis GM, Celermajer DS, D'Udekem Y, Cordina R. Exercise testing and training in adults with congenital heart disease: a surgical perspective. Ann Thorac Surg. (2020) 112:1045–54. doi: 10.1016/j.athoracsur.2020.08.118
67. Ohuchi H, Negishi J, Miike H, Toyoshima Y, Morimoto H, Fukuyama M, et al. Positive pediatric exercise capacity trajectory predicts better adult fontan physiology rationale for early establishment of exercise habits. Int J Cardiol. (2019) 274:80–7. doi: 10.1016/j.ijcard.2018.06.067
68. Kodama Y, Koga K, Kuraoka A, Ishikawa Y, Nakamura M, Sagawa K, et al. Efficacy of sports club activities on exercise tolerance among Japanese middle and high school children and adolescents after fontan procedure. Pediatr Cardiol. (2018) 39:1339–45. doi: 10.1007/s00246-018-1900-1
69. Avitabile CM, Goldberg DJ, Leonard MB, Wei ZA, Tang E, Paridon SM, et al. Leg lean mass correlates with exercise systemic output in young fontan patients. Heart. (2018) 104:680–4. doi: 10.1136/heartjnl-2017-311661
70. Baker DW, Tran D, Cordina R. The fontan circulation: is exercise training the solution? Prog Pediatr Cardiol. (2020) 59:101314. doi: 10.1016/j.ppedcard.2020.101314
71. Cordina R, D'Udekem Y. Long-lasting benefits of exercise for those living with a fontan circulation. Curr Opin Cardiol. (2019) 34:79–86. doi: 10.1097/HCO.0000000000000582
72. Cordina RL, O'Meagher S, Karmali A, Rae CL, Liess C, Kemp GJ, et al. Resistance training improves cardiac output, exercise capacity and tolerance to positive airway pressure in fontan physiology. Int J Cardiol. (2013) 168:780–8. doi: 10.1016/j.ijcard.2012.10.012
73. Ovroutski S, Ewert P, Alexi-Meskishvili V, Hölscher K, Miera O, Peters B, et al. Absence of pulmonary artery growth after fontan operation and its possible impact on late outcome. Ann Thorac Surg. (2009) 87:826–31. doi: 10.1016/j.athoracsur.2008.10.075
74. Cordina R, Celermajer DS, D'Udekem Y. Lower limb exercise generates pulsatile flow into the pulmonary vascular bed in the setting of the fontan circulation. Cardiol Young. (2018) 28:732–3. doi: 10.1017/S104795111800015X
75. Budts W, Borjesson M, Chessa M, van Buuren F, Trigo Trindade P, Corrado D, et al. Physical activity in adolescents and adults with congenital heart defects: individualized exercise prescription. Eur Heart J. (2013) 34:3669–74. doi: 10.1093/eurheartj/eht433
Keywords: physical activity, congenital heart disease, exercise training, cardiac rehabilitation, exercise capacity
Citation: Tran DL, Celermajer DS, Ayer J, Grigg L, Clendenning C, Hornung T, Justo R, Davis GM, d'Udekem Y and Cordina R (2021) The “Super-Fontan” Phenotype: Characterizing Factors Associated With High Physical Performance. Front. Cardiovasc. Med. 8:764273. doi: 10.3389/fcvm.2021.764273
Received: 25 August 2021; Accepted: 09 November 2021;
Published: 07 December 2021.
Edited by:
Petru Liuba, Lund University, SwedenReviewed by:
Hideo Ohuchi, National Cerebral and Cardiovascular Center, JapanPaul Clift, University Hospitals Birmingham NHS Foundation Trust, United Kingdom
Copyright © 2021 Tran, Celermajer, Ayer, Grigg, Clendenning, Hornung, Justo, Davis, d'Udekem and Cordina. This is an open-access article distributed under the terms of the Creative Commons Attribution License (CC BY). The use, distribution or reproduction in other forums is permitted, provided the original author(s) and the copyright owner(s) are credited and that the original publication in this journal is cited, in accordance with accepted academic practice. No use, distribution or reproduction is permitted which does not comply with these terms.
*Correspondence: Rachael Cordina, rachael.cordina@sydney.edu.au