- 1Department of Biochemistry, Saveetha Dental College and Hospitals, Saveetha Institute of Medical and Technical Sciences, Saveetha University, Chennai, India
- 2Cancer Science Laboratory, Department of Biotechnology, School of Bioengineering, SRM Institute of Science and Technology, Chennai, India
- 3Department of Bioscience Research & Medicine-Cardiology, The University of Tennessee Health Science Center, Memphis, TN, United States
Owing to myocardial abnormalities, cardiac ailments are considered to be the major cause of morbidity and mortality worldwide. According to a recent study, membranous vesicles that are produced naturally, termed as “exosomes”, have emerged as the potential candidate in the field of cardiac regenerative medicine. A wide spectrum of stem cells has also been investigated in the treatment of cardiovascular diseases (CVD). Exosomes obtained from the stem cells are found to be cardioprotective and offer great hope in the treatment of CVD. The basic nature of exosomes is to deal with the intracellular delivery of both proteins and nucleic acids. This activity of exosomes helps us to rely on them as the attractive pharmaceutical delivery agents. Most importantly, exosomes derived from microRNAs (miRNAs) hold great promise in assessing the risk of CVD, as they serve as notable biomarkers of the disease. Exosomes are small, less immunogenic, and lack toxicity. These nanovesicles harbor immense potential as a therapeutic entity and would provide fruitful benefits if consequential research were focused on their upbringing and development as a useful diagnostic and therapeutic tool in the field of medicine.
Introduction
Cardiac problems and their related diseases are considered to be the major cause of morbidity and mortality around the World (1, 2). Reports suggest that 17.7 million population succumb to death every year due to the risk of cardiovascular diseases (CVDs), which claims 31% of the total deaths worldwide (1). According to the recent studies (3–5), naturally produced membranous vesicles have the potential capability of targeting the recipient cells specifically and delivering their bioactive constituents. Owing to this characteristic, these vesicles could emerge as a potential candidate in the field of regenerative medicine. This non-living entity but bioactive, termed as “exosomes”, shows promising preliminary results in mending a broken heart with the help of stem cells; thereby, proving their advantage over the use of other cell types. Exosomes are looked upon as a best tool with great emphasis on their nature of selectivity and the ease of uptake by target cells, thereby creating a platform bestowed with immense opportunities in cell and tissue-specific targeting (6–8).
The cellular waste from the lining cells are known to be discarded by means of releasing secretory vesicles called as exosomes (9, 10). Recent studies (4, 11–14) have indicated that exosomes are escalated into crucial agents in cell–cell signaling and find application in normal physiology, such as myocardial angiogenesis, cardiac development, and the formation of vesicles during the maturation of reticulocytes. Exosomes bring about intracellular communication by way of particular modes, such as direct interaction by cell-to-cell contact, electrical, as well as long range signals and some chemical molecular interaction processed extracellularly (15–17). It has been established that one of the essential features required for the regulation of functions of the heart is the use of extracellular vesicles (EVs), precisely the exosomes (15, 18–20). The terminology “exosomes” were proposed by Johnstone et al. (21) while investigating the vesicle formation that are involved in the formation of reticulocytes. The exosome synthesis and release are diagrammatically represented (Figure 1A).
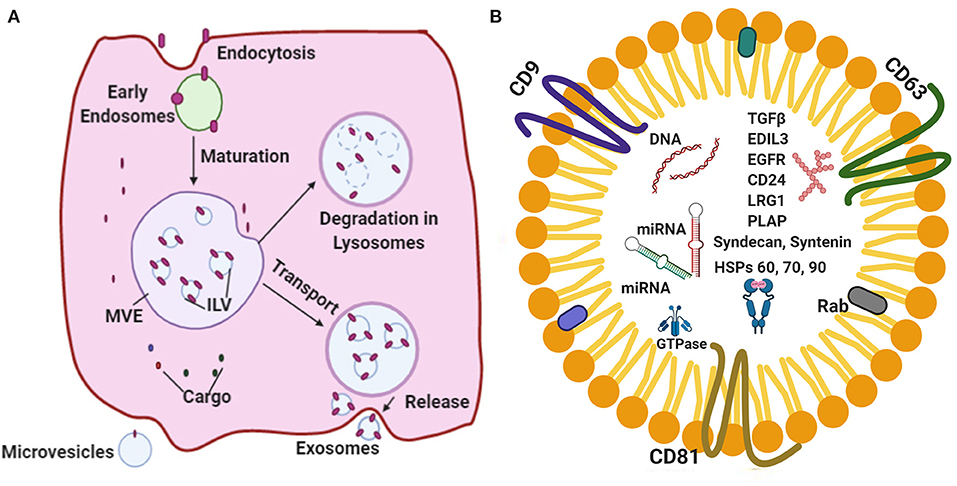
Figure 1. (A) A schematic representation of the major vital components of the endosomal pathway synthesis and release. (B) Represents standard exosomal -markers that have been reported to serve as biomarkers for the identification of certain diseases. Exosomal cargo composed of various proteins, mRNA, miRNA and DNA.
Exosomes are nanosized EVs that range from 30 to 150 nm in size with a floating density of 1.13–19 g/ml, as determined by using the ultracentrifugation technique on a linear sucrose density gradient (2–0.25 M of sucrose) (22–25). According to International Society for Extracellular Vesicles (ISEV) the membrane vesicles classified three categories apoptotic bodies (ABs), membrane vesicles (MVs) and exosomes. Exosomes possess unique characteristic features, such as a fluid lipid bilayer, surface proteins/receptors, mRNAs, microRNA (miRNA), specific set of proteins, transcription factors, and other substances (26, 27). Exosomes composed of various signaling molecules, miRNAs and nucleic acids are clearly depicted (Figure 1B). A previous study (15) indicated that the exosomal contents are highly regulated in the case of stress and disease conditions, which reflects the nature of the parent cell.
Exosomes belong to the unique subtype of membrane vesicles that are released from the vital endocytic compartment of live cells. The EVs develop from budding after the invagination of plasma membrane and are synthesized through the endosomal pathway (27, 28). The EVs with membranous structures are typically, otherwise, called as exosomes, microvesicles, microparticles, ectosomes, oncosomes, apoptotic bodies, and so on (29–31). The United States is known to be the major contributor and lead the World in terms of for the research on exosomes (32).
Various theories attribute to the mechanism of action of exosomes. First, exosomes possess proteomic potency that exerts a potent diverse effect on the immune responses from both humoral and cell-mediated components of the immune system (33–35). Second, exosomes play a pivotal role in disease-modulating capacity due to the transfer of miRNAs (36, 37). Third, exosomes are of endosomal origin predominantly and are composed of small (~30–140 nm) particles enclosed by a lipid bilayer (13). Finally, they are classified further as a subset of EVs that are secreted precisely by most of the cell types (16). The isolation and purification of exosomes are still underdeveloped (14, 16, 38, 39). The isolation of exosomes from raw biological fluids seems to be a herculean task as they are similar to the size of other components in the biological fluids, such as lipoprotein, chylomicrons, and macrovesicles, which overlap with that of the exosomes (17, 39, 40).
Storage and Preservation of Exosomes
The suitable temperature to store exosomes has been found to be at −29°C (40). The anti-freezing agents are found to be helpful in preventing the formation of ice crystals inside the exosomes, increase their shelf-life, and help in storing them at −80°C or in liquid nitrogen (41–43). The entire protein content and representative functional analysis would be preserved in the exosomes if they are analyzed immediately after isolation (44). The isolation of exosomes was found to be easier from conditioned culture media and the entire process seems to be not complicated; however, different types of EVs are often co-isolated owing to the size overlap and the lack of cell-specific biomarkers (45). Many techniques have been devised for the purification of exosomes and these are also known to impact the yield, density, and function of recovered EVs (46–48). These techniques have been classified broadly into two subgroups: conventional methods and microfluidics-based methods (14, 49).
The conventional methods include size exclusion, chromatography, ultracentrifugation, immunoaffinity, ultrafiltration, and polymer-based precipitation (16, 50). These are well-established methods and are used widely, but known for lesser efficiency or reduced yield (51). In contrast, microfluidics-based method has gained momentum in the rapidly evolving isolation platforms as they possess many advantages, such as low sample consumption, increased sensitivity, easy to use, and tremendous speed when compared with conventional methods (52–54).
The purification of exosomes using the conventional method has been the most dependent mode over the past decade in research laboratories and clinics. The main principle underlying the method of isolation of exosomes is based on their physical property or their functions. As a result, they have been classified into three subgroups: density-based, size-based, and function-based isolation (16, 55).
In order to process small amount of fluid, microfluidics work on micron-sized channels (μL to pL) (52, 56). The microfluidic devices are fabricated with a specific polymer named polydimethylsiloxane (PDMS) (24, 57, 58). PDMS is known to be optically transparent and biocompatible (59). On the basis of application and separation approach required for the experiment, different components comprise the microfluidic device, such as microchannels, microvalves, connecting tubes, micropumps, and micromixers (60). Recent studies (52, 53, 61) have proved that microfluidics are capable of sorting exosomes with increased purity and sensitivity. At the same time, cutting down costs, reducing the amount of reagents utilized, and most importantly, bringing down the duration of time invested in the protocol drastically (62).
In general terms, microfluidics-based method has been classified broadly into two methods: active and passive (63). The former method relies on the exertion of external forces for its application, whereas the latter one is dependent mostly on the use of hydrodynamic and surface forces (64).
Method of Exosome Uptake by Cells
The uptake of exosomes could be denoted as the process in which exosome signals are transferred to the recipient cell by a three-step mechanism that involves the interaction of receptors, membrane fusion, and endocytosis (65). In addition, exosome uptake was measured quantitatively by using fluorescent EGFP Renilla protein (66). The exosome uptakes through the following processes are studied widely: clathrin-mediated, caveolin-mediated, macropinocytosis, phagocytosis, involvement of lipid rafts, and membrane fusion (67–72).
Exosome Loading
Numerous methods have been proposed for loading exosomes that are classified widely into two different strategies: cargo loading after isolation and cargo loading during formation (73, 74). Exosomes composed of various types of cargo molecules DNA, RNA, protein, miRNA and lipids (75). The exosomal cargo loading is an important phenomenon for circulation and cell-cell communication. Endosomal cargo loading is differs in various physiological and pathological conditions. The endosomal sorting complex required for transport (ESCRT) a pivotal roles in synthesis and cargo loading (76). ESCRT family of proteins such as Tsg101, Hrs, CHMP4, STAM1, VPS4, VTA1, nSMase 2, PLD. CD9 and ALIX are important roles in cargo loading (77–81). N-linked glycosylation directed the glycoprotein sorting in extracellular membrane vesicles (82). Lipids plays a crucial roles in exosome biogenesis includes Phosphotidylserine (PS), phosphotidylethanolamine (PE), phosphatidylcholaine (PC), phosphatidylinositals (PIs), phosphatidic acid (PA), cholesterol, ceramide and spingomyelins (83, 84).
Exosome Classification
Exosomes have been classified broadly as natural exosomes (13) and engineered exosomes (85) (Figure 2). Naturally occurring exosomes are further classified into animal-derived exosomes (16) and plant-derived exosomes (86). As exosomes are synthesized under normal and diseased or tumor conditions, animal exosomes are subdivided further into normal exosomes and tumor exosomes or oncosomes (30, 87). Some of the normal cell type exosomes are derived from mesenchymal stem cells (MSCs) (88) and immune cells, such as T cells, B cells, macrophages dendritic cells, and natural killer cells (89). Among these, MSCs play a vital role in the disease development and are also involved in the damage and repair of tissues with definitive therapeutic prospects on CVD and certain neurological diseases (90). In addition, normal exosomes occur in body fluids, such as saliva, plasma, urine, milk, bile, ascites, and express diagnostic and therapeutic properties (13, 91, 92). Tumor exosomes are not only associated with tumor growth and metastasis, but also helps in identifying the disease conditions, thereby acting as ‘diagnostic markers’ (35, 93, 94).
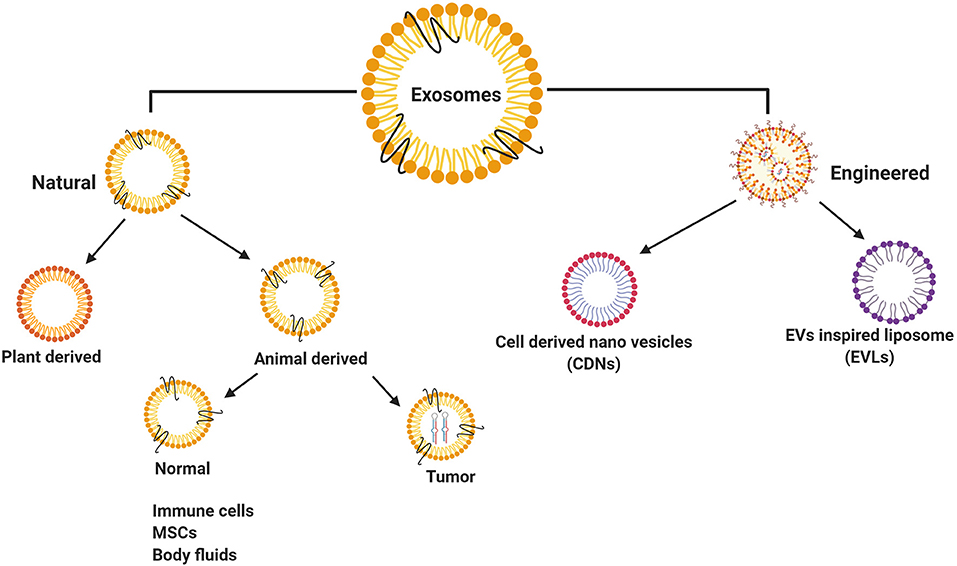
Figure 2. Classification of exosomes isolated from different resources. Natural exosomes have showed in animal/human derived exosomes, plant derived exosomes and engineered exosomes.
Biogenesis and Release of Exosomes
Initially, exosomes are synthesized with the inward budding of the cell membrane that gives rise to early endosomes processed by a secondary inward budding of the endosomal membrane to develop into numerous intraluminal vesicles (ILVs), also referred to as the late endosomes (13). Late endosomes enclosing ILVs are also known as multivesicular bodies (MVBs). In the end, the MVBs tend to fuse with the cell membrane bringing about the release of ILVs in an exocytotic manner outward into the extracellular environment (16). Originally, these ILVs are referred to as “Exosomes” (13, 80, 95–97).
Exosomes are released from the cell by either constitutive or inducible process. The constitutive secretory pathway is controlled by RAB GTPases (Rab 27a/b, Rab 11, and Rab 35), heterotrimeric G protein, WNT5A, glycosphingolipids, and flotillins (98–104). Furthermore, the inducible secretion pathway is controlled by stress stimuli that involve an aberrant intracellular calcium release, thrombin, DNA damage, hypoxia, lipopolysaccharides, and heat shock (99, 105–107).
Although the release is governed by either of these factors, ultimately exosome secretion necessitates its fusion with the plasma membrane (108). The membrane fusion of MVBs is facilitated by a combination of soluble N-ethylmaleimide sensitive factor attachment protein receptor (SNARE) proteins localized on MVBs that interact with target SNAREs localized on the intracellular side of the plasma membrane, which results in a membrane-binding SNARE complex paving pathway for membrane fusion (109–111).
A few pilot studies (112–115) have demonstrated that the exosomal RNAs are quite functional in their recipient cells by showcasing the composition of exosomes composed of double-stranded DNA (dsDNA), mRNA, and non-coding RNA. A study (81) depicts the efficient intercellular communication that arises due to the exchange of exosome contents across cells. In addition, evidence shows that the process of protein and RNA sorting into exosomes is dependent mostly on multiple pathophysiological factors, such as stress and disease (116, 117). This type of tailor-made exosomes with modified functional constraints due to any disease may serve as biomarkers for the diagnosis and prognosis of various diseases namely CVD (15, 20, 118).
Stem Cells
Since the first study describing the potential of skeletal muscle in order to repair the heart, a wide spectrum of stem cells has been investigated for the treatment of CVD (119). Stem cells are capable of self-renewal with the ability to differentiate themselves into numerous cell types. Stem cells could be guided to become specific cells that can help to regenerate and repair diseased or damaged tissues (120). Stem cells are unipotent, multipotent, and pluripotent (121). Different types of stem cells exist and are classified based on their source, such as embryonic stem cells, umbilical cord blood stem cells, cardiac stem cells, MSCs, and so on (122).
The cell-based therapies were studied widely in cardiac tissue regeneration and the most significant type of cells identified are as follows: skeletal myoblasts (SKM) (123), bone marrow derived cells (BMCs) (124), induced pluripotent stem cells (iPSCs) (125), endothelial progenitor cells (EPCs) (126), and cardiac progenitor cells (CPCs) (127, 128). Among the three therapies identified, exosome-based therapy has emerged as the novel focal point to treat CVD (129). The various cell types that are studied widely in the case of cardiac repair in vitro and clinical trials are ESCs, iPSCs and multipotent/unipotent adult stem cell lineages, such as MSCs, cancer stem cells (CSCs), and cardiosphere-derived cells (CDCs) (130, 131).
Pluripotent stem cells are capable of differentiating into any type of cells in the body and used for tissue repair (132). Therefore, these stem cells were investigated widely as a harbinger of hope in cardiac regenerative therapy. To support this fact, Adamiak et al. (2) have stated that EVs derived from murine iPSC (miPSC–EVs) offer cytoprotective properties to cardiac cells in vitro and are capable of inducing superior cardiac repair in vivo associated with left ventricular function, vascularization, and amelioration of apoptosis and hypertrophy. A recent study (133) has revealed that the treatment using iPSC-derived exosomes induces the embryoid body differentiation and remodeled the cardiomyocyte hypertrophic cells.
Stem Cell-Derived Exosomes
Pluripotent stem cells that are generated from adult somatic cells or blood cells by a process of genetic programming are called as iPSCs, wherein they revert into an embryonic-like pluripotent nature (134, 135). Owing to the clinical hurdles, such as safety and ethical issues, the use of human iPSCs for transplantation therapy to offer cardiac protection and restore function has been looked upon as a nightmare rather than a reality. Therefore, most of the research studies have been focused on secretomes (cell-secreted EVs) apart from iPSC injection/transplantation (133, 136). MSCs are the most magnificent producers of exosomes among the various cell types (90, 137, 138). The treatment of diseases based on the mechanism of MSC-derived exosomes has emerged as the future of scientific research. It has been put forth that therapeutic exosomes in tissue regeneration occupy the hotspot which might hold great promise for a long term.
In the 1970s, Friedenstein (47) described a population of bone marrow stromal cells that are capable of mesodermal differentiation and trophic support of hematopoiesis (48), Caplan (139) was the one who coined the term “Mesenchymal stem cells” in the early 1990s. MSCs constitute a heterogeneous subset of stromal regenerative cells and could be harvested easily from several types of adult tissues (140). MSCs have attained a prominent place by being known as the most promising of all therapy tools owing to their relatively simple procedure for cell isolation, self-renewal capacity, differentiation, ability, low immunogenicity, multipotency, and secretion of mediators that support tissue renovation or its substitution (140). MSC-derived exosomes seem to be enriched highly in certain biologically active molecules, such as RNAs and proteins (54). These MSCs are well equipped to maintain homeostasis within the tissue and respond to external stimuli. Studies have revealed that MSC-derived exosomes promote protective effects against myocardial I/R injury through several mechanisms that might include cardiac regeneration, anti-apoptosis, anti-inflammatory effects, cardiac remodeling, anti-vascular remodeling, and neovascularization (141–144). A recent discovery suggested that cardiac progenitor-derived exosomes inhibited cardiomyocyte apoptosis and improves the patients hailing in myocardial infarction and left ventricular ejection function (145).
As per a previous study (61), adipose-derived stem cells (ADSCs) are stromal vascular fragment of the adipose tissue in origin and have proved to be credible and reliable in terms of clinical implications. These cells have inherent differentiation properties that could give rise to diverse cell lineages and are capable of secreting high levels of protein that are known to play an important role in immunoregulation, revascularization, angiogenesis, coetaneous wound healing, and regeneration of worn-out tissues (146–148).
Apart from the secreted proteins, these cells are capable of releasing exosomes that are designated as small EVs with a multivesicular endosomal origin (13, 45). ADSC-Exos normoxic or hypoxic environment have been isolated successfully by using in vitro methods (149, 150). The work by Ribeiro-Rodrigues et al. (151) have demonstrated that cardiomyocyte-derived exosomes during ischemic condition gave rise to a new vessel formation and on further investigation revealed the proangiogenic effect, which was attributed partially to relatively expressed miR-143 and miR-222 in exosomes.
Another variant, known as a cardiac telocyte cell, that belong to the novel class of stromal cells was known to induce the growth and differentiation of CSCs/CPCs during organogenesis and brought about improvement in cardiac function (152). In addition, the transplantation of cardiac telocyte cells led to an enhancement of angiogenesis coupled with a decrease in cardiac fibrosis, which was involved in heart physiology and regeneration (153). Cardioprotective factors that enable beneficial effects to damaged heart were secreted by cardiac telocyte-derived exosomes (154).
A previous research work carried out by Kervadec et al. (155) stated that exosomes that are secreted by human ESC-derived cardiovascular progenitors (hESC-pg) were capable of providing equal benefits of cardioprotective effects in contrast to hESC-pg alone injected in a mouse with post-infarct heart failure (HF). Kervadec et al. (155) also identified over 927 upregulated genes in the heart owing to the treatment with hESC-pg and their derivative exosomes, which led to an increase in cardiac function by 78%. Khan et al. (156) have also observed that mouse ESC-derived exosomes help in restoring the cardiac function in acute myocardial infarction (MI)-infected mouse model. In addition, the study even reported that miR-294 was responsible for neovascularization, improved survival of cardiomyocytes, and reduced fibrosis post infarction.
Therapeutic efficacies of iPSC-derived exosomes have contributed to neovascularization and survival of cardiomyocytes in experimental animal models of CVDs, as demonstrated by Jung et al. (157). Besides these exosomes, iPSC-derived cardiovascular progenitor cells (iPSC-pg) have proven to be effective in the treatment of congenital cardiac failure (CCF), attributed mainly to the specificity of 16 highly abundant miRNAs that were known to be evolutionarily conserved mRNAs and involved in pathways associated with tissue repair (158). Khan et al. (156) have demonstrated that the mouse ESC-derived exosomes are capable of endogenous repair and preserve the functions of the heart when delivered by intramyocardial injection, as soon as left anterior descending ligation in an infraction-afflicted murine model. In particular, the researchers attributed the success toward ESC-derived exosomes, which were responsible for the beneficial effects in therapy to exosomal miR-294 (19, 156, 159). Cardiac progenitor-derived exosomes composed of pregnancy associated plasma protein A cleavage of insulin-like growth factor binding protein-4 and insulin-like growth factor-1 and activated the cell proliferation in Akt and ERK1/2 pathways in cardiomyocytes (160). In addition, cardiac progenitor-derived exosomes inhibited caspase activation and cardiomyocyte protection (160).
Beneficial Role of Stem Cell-Derived Exosomes in CVD
Research data suggest that exosomes obtained from stem cells aid in cardioprotection, which promotes therapeutic remedy in the treatment of CVD (136, 161). Lai et al. (162) have demonstrated that human embryonic stem cells (hESC) secreted 50–100 nm membrane vesicles using an ex vivo Langendorff model of ischemia/reperfusion (I/R) injury, wherein they observed that these purified MSC-derived exosomes help in reducing the infarct size in mouse cardiac tissue.
Arslan et al. (163) showed that a single intravenous injection of exosomes (5 mm) before reperfusion reduced the size of infarct by ~45% in mice. It was also reported that the treatment with exosomes helped to restore depleted energy and redox rate potential in mouse health within 30 min after I/R, which was proven by the presence of increased levels of ATP and NADH levels with a decrease in oxidative stress (164). The studies conclude that MSC-derived exosomes harbor all necessary enzymes that are required for the generation of ATP (165, 166). The report also suggest that exosome treatment leads to the reduction in systematic inflammation in mice model after treatment with myocardial I/R (167) (Figure 3). Thus, MSC-derived exosomes could be a powerful therapeutic solution for patients diagnosed with acute myocardial infarction (167). The cardiac progenitor cells plays crucial role in angiogenic and cardiogenic properties in high-glucose conditions in both in vitro and animal models (168).
The data obtained by Wang et al. (169) revealed the supreme cardioprotective nature, inclusive of cell survival and angiogenesis by human endometrium-derived MSC (En-MSC). Mounting clinical and experimental evidences suggest the possibility that MSC with the ability to differentiate into different cell lineages could serve as a promising therapy to treat patients with cardiac dysfunction (170–172). Hematopoietic stem cell (HSC)-derived exosomes provide support to repair cardiac tissues by differentiating into cardiomyocytes (173, 174).
The research work published by Tseliou et al. (175) stated that the exosomes derived from cardiospheres (CDCs) seem to transform inert dermal fibroblasts into therapeutically active cells, which could bring about a decrease in the size of the scar and improve cardiac function in a chronic myocardial infarction model. CDCs are known to stimulate the regeneration of angiogenesis and functional improvement in the infarcted myocardium (176). Exosomes from CDCs could inhibit apoptosis significantly and are capable of promoting the proliferation of cardiomyocytes apart from enhancing angiogenesis (6).
Adult Stem Cells (ASC) Based Therapy
Endothelial progenitor cells, a class of unipotent adult stem cells, have been looked upon as a therapeutic progenitor for the post-injury treatment of cardiac muscles (177, 178). A group of researchers (179) have demonstrated that adult human CD34+ stem cells isolated and purified from mobilized peripheral blood mononuclear cells (PBMCs) secrete exosomes (CD34 Exo) and are capable of inducing angiogenic activity in isolated endothelial cells and in murine models (179, 180). A study by Sahoo et al. (179) also reported a similar finding that human CD34+ stem cells have the ability to secrete cup-shaped exosomes that expressed CD63, phosphatidylserine, and TSG101. Recent striking discoveries shows that CD34-Exo are internalized easily by endothelial cells in the ischemic tissue, wherein they tend to induce cell cycle, angiogenesis, and cell proliferation (6, 181, 182).
On the basis of in vivo results, the scientists noted that both CD34+ cells and CD34+ exosomes induced the formation of vessel, such as endothelial cells, influenced by appropriately elevated proportion of endothelial cells in the Matrigel plug. A previous study (183) reveals that CD34+ exosomes form a key paracrine component of CD34+ cells induced vessel cell growth. Exosomes purified from body fluids and somatic cells have also been reported to be capable of being implemented as novel therapeutic options for certain CVD (36).
Stroke and Stem Cell-Derived Exosomal Therapy
Stroke is a malfunction of the heart that leads to death and disability. Stroke is a form of degenerative disease associated with aging in individuals. Stem cell therapy could pave the way for a biological treatment alternative to the traditional therapy based on pharmacology (184). MSCs are chosen widely because of their differentiation ability and the ease with which they can be isolated from various adult tissues. In addition, adult MSCs pose less problems when compared with ESCs in terms of tumorigenesis and ethical issues (185, 186). In this regard, an evidence (139) claimed that the beneficial effects of stem cell transplantation are based on their ability to secrete bioactive molecules, namely, the exosomes. In a myocardial infarction model, the utilization of exosomes obtained from myc-transformed MSCs was known to reduce the infarction size (187). A previous study (138, 188) has focused mainly on the role of EVs in MSC transplantation and their therapeutic effects. The major subtypes of EVs are exosomes, microvesicles, and apoptotic bodies.
MSC-derived exosomes could be isolated steadily from MSC-conditioned medium. They are more effective compared with direct MSC transplantation used in the treatment of various ailments, such as CVD, acute kidney injury, liver injury, and lung injury (125, 185).
Therapeutic Application of Exosomes
Exosomes contain various molecules, such as miRNA, that are capable of mediating biological function by means of gene regulation (185). miRNAs belong to a novel class of small non-coding RNAs (189, 190). Evidence show that miRNA are packaged into exosomes. They help to mediate cell–cell communication by acting as paracrine messengers (191). Exosomes serve as a new therapeutic target and could act as a biomarker for various disorders including cardiac disease (190). Mesenchymal stem cell derived exosomes (MSC-exo) used for various aging related diseases (192).
Exosomal miR-146a could be used as a highly specific blood biomarker that will help in the diagnosis and risk stratification of patients suffering from peripartum cardiomyopathy (115). Another studies (15, 193) has claims that the impairment of myocardial angiogenesis in diabetic patients occurs due to the secretion of anti-angiogenic exosomes released from cardiomyocytes. Sepsis-induced cardiomyopathy has been attributed to the high number of exosomes and their intrinsic properties (194).
Exosomes along with their biologically active cargos would offer apparent prognostic information about various diseases, such as chronic inflammation (195), cardiovascular and renal diseases (196), neurodegenerative diseases (197), lipid metabolic disorders (198), and tumors (199). Exosomes resemble the paracrine factors in both human and mouse CPCs (200) and stimulate heart protection inclusive of miRNAs both in vitro and in vivo. Gray et al. (201) reported that CPC-derived exosomes in response to hypoxia also led to the upregulation of 11 miRNAs compared with normal exosomes. In the previous work of Ong et al. (202), CPCs that overexpressed hypoxia inducible factor 1α (HIF-1α) help in improving the survival rate of transplants and the success was attributed mainly to the increased levels of miR126 and miR120 present within the exosomes, which had activated the prosurvival kinases and induced the glycolytic switch in the recipient CPCs.
Human CD34+ stem cell-secreted exosomes portrayed angiogenic activity, which was independent of its action in both in vitro and in vivo studies. This supports the notion that exosomes from stem cells could be an important component of therapeutic angiogenesis (11, 203). Ischemic heart disease (IHD) is caused by the blockage of blood flow to the heart arteries, resulting in reduced blood supply to the heart (204). It seems to be one of the most common cause of death, of over 9 million deaths per year worldwide (205). To cure this disease, stem cell therapy using somatic multipotent and pluripotent stem cells presents a valid approach to harness cardiac regeneration and myocardial function recovery (206). Giricz et al. (207) have demonstrated that EVs released from rat heart after ischemic preconditioning is essential and plays a crucial role in the transmission of remote conditioning signals for cardioprotection.
Exosomes deal with the transport and intracellular delivery of proteins and nucleic acids naturally. This helps us to rely on them as the attractive pharmaceutical delivery agents (208). Numerous commercial organizations have started developing exosome-based cancer diagnostics lately, such as Caris life sciences, exosomes diagnostics, and Humsa Bio Med. However, in the field of cardiovascular medicine exosomes remain an unexplored world that is committed to pioneer in order to reach the zenith of fruitful therapy.
Exosomes are known to offer atherosclerotic protein by means of cell–cell communication. Chen et al. (209) were able to trace the presence of RNAs in the supernatant of human MSCs that might be correlated to exosomes. They were able to witness a cardioprotective effect offered by these exosomes when infused into the rat model. Ibrahim et al. (210) have claimed the exosomes as critical agents that are capable of bringing about cardiac regeneration influenced by cardiosphere-mediated cell therapies. CPCs seem to possess antifibrotic effects by way of transferring the exosomes into fibroblasts therapy promoting angiogenesis and the survival of cardiac myocytes in vitro (85).
Exosomes that have been isolated from CDCs expressing Lamb 2b was known to contain cardiomyocyte-specific peptide on their surface and, therefore, led to the increased uptake by cardiomyocytes (85). Exosomes that were isolated from hiPSC-ECs contained miR-199b-5p and are known to promote angiogenesis (211). Exosomes have been synthesized naturally within the body and promote cell–cell communication, molecular therapy for cancer treatment (212), and in the diagnostics of various skeletal disorders, such as osteoarthritis (213), Osteochondral regeneration (214), myocardial I/R injury (162), limb ischemia, and pulmonary hypertension (215). Exosomes have been explored widely to act as the natural drug delivery vehicles, because they can travel safely in extracellular fluids trespassing immune cells and deliver the cargo to designated target cells with both utmost efficiency and a high degree of specificity (216, 217).
When it comes to exosomes as a notable therapeutic delivery system, it offers a few benefits in terms of specificity, safety, and stability. Exosomes are capable of delivering cargo to specific targets located at distant sites and could be employed to deliver small interfering RNA (siRNA) or other pharmaceutically active compounds (218). The miniature size of exosomes, being native to animals, helps them in avoiding phagocytosis easily, capable of fusing diligently with the cell membrane and escape the engulfing by lysosomes. As if the exosomes are a natural product of our body, which results in minimal or low immune response (219). In addition, exosomes possess a hydrophilic core that make them apt to deliver water-soluble drugs (220). ADSC-exosomes seem to play prominent tissue engineering and regenerative therapies (61). Exosomes have been used for therapeutic applications of cardiac ischemic diseases (221).
Transplantation of stem/progenitor cells have been declared as one of the promising therapeutic strategies for CVDs with the ability to replace lost cardiomyocytes and improve contractility (132, 222). Interestingly, exosomes have been stated to bring about communication among the cardiac fibroblasts, endothelial cells, and cardiomyocytes via delivering a wide array of contents inclusive of proteins and nucleic acids, such as RNA and DNA (108). This seems to be essential in order to support myocardium with oxygen and nutrients detrimental in normal heart and, thereby, mediate homeostasis of the heart maintaining structural integrity. In addition, exosome-enriched miRNAs nurture great promise for predicting the risk of developing CVD. Li et al. (55) have identified and listed plasma exosomal miR-422a and miR-125b2-3p, which might serve as a blood-based biomarker for the diagnosis and monitoring of patients suffering from ischemic stroke (IS). Recently, mesenchymal stem cell derived exosomes used in therapeutic purposes in SARS-CoV-2 infected cases (223). Recently, the exosomes which induces the autophagy and metastasis of tumor cells (224). Exciting results indicated that a long-term treatment of low-dose of Ticagcelor enhances the release of cardioprotective-exosomes through increasing cell proliferation in cardiomyocytes in vitro models (225).
Advantages of Exosomes
Exosomes are smaller in size and being nanovesicles, they are less complex and less fragile than parent cells. They could be easily modulated/modified, engineered, manufactured, and stored. They evince no risk of tumor formation and are considered to be less immunogenic. They offer safety to their molecular cargos by providing the protection against enzymatic and non-enzymatic degradation (226).
First, the use of exosomes helps to avoid problems pertaining to cells that have defective or mutant DNA (227). Second, their small size makes it feasible to circularize easily through capillaries, whereas cells used in cell-based therapies, such as MSCs, might be large in size to traverse through the capillaries. Third, level of MSCs might diminish quickly after transplantation, whereas exosomes might be able to achieve a higher benefit than the transplanted MSCs (24). Fourth, exosomes lack toxicity and immunogenicity (228). It has been evident from various studies (229–232) that exosomes and their contents have the innate ability to govern cell survival, proliferation, migration, and differentiation in the damaged heart; thereby, reducing the risk of CVD-related deaths.
Limitations/Disadvantages of Exosomes
It is really tough to note that a remarkable challenge lies ahead in developing scalable and reproducible protocols for isolation, purification, and storage of exosomes. In addition, there is a great deal of efforts necessary to provide improvised techniques and frame essential or vital norms for the analysis of the quality of exosomes. The suitable method to isolate sterile exosomes should be in compliance with good manufacturing practices capable of reproducible purity and potency (23). It is also difficult to set up a particular unit for determining the dose of exosomes, dosage regimen, particle numbers, and the route of administration (233). There is a great deal of effort and efficiency required to design a successful protocol to identify, quantify, and characterize the major exosomal component that are responsible for the biological effect in a particular disease. In addition, proposing the mechanism of action through qualified potency assays in case of relevant disease in vitro and in animal models is a tedious task requiring a lot of perfection with utmost efficiency (234).
Future Perspectives
Exosomal research an emerging area of study which implies various advantages for therapeutic and diagnostic applications in cardiovascular disorder and other disease conditions. The future perspectives is need to address the route of administration of exosomes, dose fixation, biological half-life will be identified. The engineered exosomes are required to be tested in toxicity, immunogenicity and allergic potentials.
Conclusion
Exosomes has a wide array of sources from stem cells to body fluids. Not only the regeneration potency of the exosomes, but also the cardioprotective roles of exosomes have been researched in many in vivo and in vitro findings. Exosomes are best studied for multi-targeted system biology for repair and regeneration as it harbors number of molecules. Based on the research purpose, the required therapeutic exosomes can be developed. To achieve this, a sensitive technique dissection and detailed analysis of exosomes components are required which will ultimately lead us to early diagnosis and standard treatment. This detailed review shows that the exosomes are effective and efficient drug delivery models. They also seem to be a promising path in the field of regenerative medicine. The stem cell-based therapy has emerged as the promising and safe therapeutic strategy for CVD. In conclusion, exosomes derived from stem cells is capable of exerting beneficial therapeutic effects on myocardial regeneration, thereby help in the treatment of CVD. In addition, clinical trials are required to prove the therapeutic efficacy of exosomes in CVD.
Author Contributions
SJ and DG wrote the manuscript. JR and KP edited the manuscript. All authors contributed to the article and approved the submitted version.
Funding
This study was supported by Department of Science and Technology (DST) Science Engineering Research Board (SERB), Government of India grant number EEQ/2017/000567 to KP, DST-SERB grant number EEQ/2019/000568 to SJ, and National Institute of Health R01 grant HL141345 to JR.
Conflict of Interest
The authors declare that the research was conducted in the absence of any commercial or financial relationships that could be construed as a potential conflict of interest.
Publisher's Note
All claims expressed in this article are solely those of the authors and do not necessarily represent those of their affiliated organizations, or those of the publisher, the editors and the reviewers. Any product that may be evaluated in this article, or claim that may be made by its manufacturer, is not guaranteed or endorsed by the publisher.
Acknowledgments
We gratefully thank Mr. M. Vijayaraman for correcting English language in this article.
References
1. Organization WH. World Health Statistics 2016: Monitoring Health for the Sdgs Sustainable Development Goals. Geneva: World Health Organization (2016).
2. Adamiak M, Cheng G, Bobis-Wozowicz S, Zhao L, Kedracka-Krok S, Samanta A, et al. Induced pluripotent stem cell (iPSC)-derived extracellular vesicles are safer and more effective for cardiac repair than iPSCs. Circ Res. (2018) 122:296–309. doi: 10.1161/CIRCRESAHA.117.311769
3. Bai J, Duan J, Liu R, Du Y, Luo Q, Cui Y, et al. Engineered targeting tLyp-1 exosomes as gene therapy vectors for efficient delivery of siRNA into lung cancer cells. Asian J Pharm Sci. (2020) 15:461–71. doi: 10.1016/j.ajps.2019.04.002
4. Rogers RG, Ciullo A, Marban E, Ibrahim AG. Extracellular vesicles as therapeutic agents for cardiac fibrosis. Front Physiol. (2020) 11:479. doi: 10.3389/fphys.2020.00479
5. Lu Y, Chan YT, Tan HY Li S, Wang N, Feng Y. Epigenetic regulation in human cancer: the potential role of epi-drug in cancer therapy. Mol Cancer. (2020) 19:79. doi: 10.1186/s12943-020-01197-3
6. Mathiyalagan P, Liang Y, Kim D, Misener S, Thorne T, Kamide CE, et al. Angiogenic mechanisms of human CD34(+) stem cell exosomes in the repair of ischemic hindlimb. Circ Res. (2017) 120:1466–76. doi: 10.1161/CIRCRESAHA.116.310557
7. Meurer S, Wimmer AE, Leur EV, Weiskirchen R. Endoglin trafficking/exosomal targeting in liver cells depends on N-glycosylation. Cells. (2019) 8:997 doi: 10.3390/cells8090997
8. Liang C, Liu Y, Xu H, Huang J, Shen Y., Chen F, et al. Exosomes of human umbilical cord MSCs protect against hypoxia/reoxygenation-induced pyroptosis of cardiomyocytes via the miRNA-100-5p/FOXO3/NLRP3 pathway. Front Bioeng Biotechnol. (2020) 8:615850. doi: 10.3389/fbioe.2020.615850
9. Trams EG, Lauter CJ, Norman S Jr., Heine U. Exfoliation of membrane ecto-enzymes in the form of micro-vesicles. Biochimica et Biophysica Acta. (1981) 645:63–70. doi: 10.1016/0005-2736(81)90512-5
10. Pan BT, Teng K, Wu C, Adam M, Johnstone RM. Electron microscopic evidence for externalization of the transferrin receptor in vesicular form in sheep reticulocytes. J Cell Biol. (1985) 101:942–8. doi: 10.1083/jcb.101.3.942
11. Olejarz W, Kubiak-Tomaszewska G, Chrzanowska A, Lorenc T. Exosomes in angiogenesis and anti-angiogenic therapy in cancers. Int J Mol Sci. (2020). 21, 5840–6478. doi: 10.3390/ijms21165840
12. Chen Q, Huang M, Wu J, Jiang Q, Zheng X. Exosomes isolated from the plasma of remote ischemic conditioning rats improved cardiac function and angiogenesis after myocardial infarction through targeting Hsp70. Aging. (2020) 12:3682–93. doi: 10.18632/aging.102837
13. Kalluri R, Lebleu VS. The biology, function, and biomedical applications of exosomes. Science. (2020) 367:6478 doi: 10.1126/science.aau6977
14. Dash M, Palaniyandi K, Ramalingam S, Sahabudeen S, Raja NS. Exosomes isolated from two different cell lines using three different isolation techniques show variation in physical and molecular characteristics. Biochim Biophys Acta Biomembr. (2021) 1863:183490. doi: 10.1016/j.bbamem.2020.183490
15. Ailawadi S, Wang X, Gu H, Fan GC. Pathologic function and therapeutic potential of exosomes in cardiovascular disease. Biochim Biophys Acta. (2015) 1852:1–11. doi: 10.1016/j.bbadis.2014.10.008
16. Gurunathan S, Kang MH, Jeyaraj M, Qasim M, Kim JH. Review of the isolation, characterization, biological function, and multifarious therapeutic approaches of exosomes. Cells. (2019) 8:307 doi: 10.3390/cells8040307
17. Gurunathan S, Kang MH, Kim JH. A comprehensive review on factors influences biogenesis, functions, therapeutic and clinical implications of exosomes. Int J Nanomedicine. (2021) 16:1281–312. doi: 10.2147/IJN.S291956
18. Mancuso T, Barone A, Salatino A, Molinaro C, Marino F, Scalise M, et al. Unravelling the biology of adult cardiac stem cell-derived exosomes to foster endogenous cardiac regeneration and repair. Int J Mol Sci. (2020) 21:3725 doi: 10.3390/ijms21103725
19. Tikhomirov R, Donnell BR, Catapano F, Faggian G, Gorelik J, Martelli F, et al. Exosomes: from potential culprits to new therapeutic promise in the setting of cardiac fibrosis. Cells. (2020) 9:592 doi: 10.3390/cells9030592
20. Sluijter JPG, Davidson SM, Boulanger CM, Buzas EI, De Kleijn DPV, Engel FB, et al. Extracellular vesicles in diagnostics and therapy of the ischaemic heart: position paper from the working group on cellular biology of the heart of the european society of cardiology. Cardiovasc Res. (2018) 114:19–34. doi: 10.1093/cvr/cvx211
21. Johnstone RM, Bianchini A, Teng K. Reticulocyte maturation and exosome release: transferrin receptor containing exosomes shows multiple plasma membrane functions. Blood. (1989) 74:1844–51. doi: 10.1182/blood.V74.5.1844.bloodjournal7451844
22. Vlassov AV, Magdaleno S, Setterquist R, Conrad R. Exosomes: current knowledge of their composition, biological functions, and diagnostic and therapeutic potentials. Biochim Biophys Acta. (2012) 1820:940–8. doi: 10.1016/j.bbagen.2012.03.017
23. Sluijter JP, Verhage V, Deddens JC, Van Den Akker F, Doevendans PA. Microvesicles and exosomes for intracardiac communication. Cardiovasc Res. (2014) 102:302–11. doi: 10.1093/cvr/cvu022
24. Johnsen KB, Gudbergsson JM, Skov MN, Pilgaard L, Moos T, Duroux M. A comprehensive overview of exosomes as drug delivery vehicles - endogenous nanocarriers for targeted cancer therapy. Biochim Biophys Acta. (2014) 1846:75–87. doi: 10.1016/j.bbcan.2014.04.005
25. Buzas EI, Gyorgy B, Nagy G, Falus A, Gay S. Emerging role of extracellular vesicles in inflammatory diseases. Nat Rev Rheumatol. (2014) 10:356–64. doi: 10.1038/nrrheum.2014.19
26. Zhu H, Fan GC. Extracellular/circulating microRNAs and their potential role in cardiovascular disease. Am J Cardiovasc Dis. (2011) 1:138–49.
27. Emanueli C, Shearn AI, Angelini GD, Sahoo S. Exosomes and exosomal miRNAs in cardiovascular protection and repair. Vascul Pharmacol. (2015) 71:24–30. doi: 10.1016/j.vph.2015.02.008
28. Yanez-Mo M, Siljander PR, Andreu Z, Zavec AB, Borras FE, Buzas EI, et al. Biological properties of extracellular vesicles and their physiological functions. J Extracell Vesicles. (2015). 4:27066. doi: 10.3402/jev.v4.27066
29. Théry C, Witwer KW, Aikawa E, Alcaraz MJ, Anderson JD, Andriantsitohaina R, et al. Minimal information for studies of extracellular vesicles 2018 (MISEV2018): a position statement of the International Society for Extracellular Vesicles and update of the MISEV2014 guidelines. J Extracell Vesicles. (2018) 7:1535750. doi: 10.1080/20013078.2018.1461450
30. Bertolini I, Terrasi A, Martelli C, Gaudioso G, Di Cristofori A. Storaci AM, et al. A GBM-like V-ATPase signature directs cell-cell tumor signaling and reprogramming via large oncosomes. EBioMedicine. (2019) 41:225–35. doi: 10.1016/j.ebiom.2019.01.051
31. Surman M, Kedracka-Krok S, Hoja-Lukowicz D, Jankowska U, Drozdz A, Stepien EL, et al. Mass spectrometry-based proteomic characterization of cutaneous melanoma ectosomes reveals the presence of cancer-related molecules. Int J Mol Sci. (2020) 21:2934. doi: 10.3390/ijms21082934
32. Wang B, Xing D, Zhu Y, Dong S, Zhao B. The state of exosomes research: a global visualized analysis. Biomed Res Int. (2019) 2019:1495130. doi: 10.1155/2019/1495130
33. Toh WS, Zhang B, Lai RC, Lim SK. Immune regulatory targets of mesenchymal stromal cell exosomes/small extracellular vesicles in tissue regeneration. Cytotherapy. (2018) 20:1419–26. doi: 10.1016/j.jcyt.2018.09.008
34. Börger V, Bremer M, Ferrer-Tur R, Gockeln L, Stambouli O, Becic A, et al. Mesenchymal stem/stromal cell-derived extracellular vesicles and their potential as novel immunomodulatory therapeutic agents. Int J Mol Sci. (2017) 18:1450. doi: 10.3390/ijms18071450
35. Zhang L, Yu D. Exosomes in cancer development, metastasis, and immunity. Biochim Biophys Acta Rev Cancer. (2019) 1871:455–68. doi: 10.1016/j.bbcan.2019.04.004
36. Beltrami C, Besnier M, Shantikumar S, Shearn AI, Rajakaruna C, Laftah A, et al. Human pericardial fluid contains exosomes enriched with cardiovascular-expressed MicroRNAs and promotes therapeutic angiogenesis. Mol Ther. (2017) 25:679–93. doi: 10.1016/j.ymthe.2016.12.022
37. Ferguson S, Kim S, Lee C, Deci M, Nguyen J. The Phenotypic Effects of Exosomes Secreted from Distinct Cellular Sources: a Comparative Study Based on miRNA Composition. AAPS J. (2018) 20:67. doi: 10.1208/s12248-018-0227-4
38. Wu X, Qu D, Weygant N, Peng J, Houchen CW. Cancer stem cell marker DCLK1 correlates with tumorigenic immune infiltrates in the colon and gastric adenocarcinoma microenvironments. Cancers. (2020) 12:274. doi: 10.3390/cancers12020274
39. Zhu L, Sun HT, Wang S, Huang SL, Zheng Y, Wang CQ, et al. Isolation and characterization of exosomes for cancer research. J Hematol Oncol. (2020) 13:152. doi: 10.1186/s13045-020-00987-y
40. Yu B, Zhang X, Li X. Exosomes derived from mesenchymal stem cells. Int J Mol Sci. (2014) 15:4142–57. doi: 10.3390/ijms15034142
41. Bahr MM, Amer MS, Abo-El-Sooud K, Abdallah AN, El-Tookhy OS. Preservation techniques of stem cells extracellular vesicles: a gate for manufacturing of clinical grade therapeutic extracellular vesicles and long-term clinical trials. Int J Vet Sci Med. (2020) 8:1–8. doi: 10.1080/23144599.2019.1704992
42. Jeyaram A, Jay SM, Preservation and storage stability of extracellular vesicles for therapeutic applications. AAPS J. (2017) 20:1. doi: 10.1208/s12248-017-0160-y
43. Wu Y, Deng W, Klinke DJ II. Exosomes: improved methods to characterize their morphology, RNA content, and surface protein biomarkers. Analyst. (2015) 140:6631–42. doi: 10.1039/C5AN00688K
44. Maroto R, Zhao Y, Jamaluddin M, Popov VL, Wang H, Kalubowilage M, et al. Effects of storage temperature on airway exosome integrity for diagnostic and functional analyses. J Extracell Vesicles. (2017) 6:1359478. doi: 10.1080/20013078.2017.1359478
45. Raposo G Stoorvogel W. Extracellular vesicles: exosomes, microvesicles, and friends. J Cell Biol. (2013) 200:373–83. doi: 10.1083/jcb.201211138
46. Baranyai T, Herczeg K, Onodi Z, Voszka I, Modos K, Marton N, et al. Isolation of exosomes from blood plasma: qualitative and quantitative comparison of ultracentrifugation and size exclusion chromatography methods. PLoS ONE. (2015) 10:e0145686. doi: 10.1371/journal.pone.0145686
47. Friedenstein AJ, Chailakhjan RK, Lalykina KS. The development of fibroblast colonies in monolayer cultures of guinea-pig bone marrow and spleen cells. Cell Tissue Kinet. (1970) 3:393–403. doi: 10.1111/j.1365-2184.1970.tb00347.x
48. White IA, Sanina C, Balkan W, Hare JM. Mesenchymal stem cells in cardiology. Methods Mol Biol. (2016) 1416:55–87. doi: 10.1007/978-1-4939-3584-0_4
49. Wu M, Ouyang Y, Wang Z, Zhang R, Huang PH, Chen C, et al. Isolation of exosomes from whole blood by integrating acoustics and microfluidics. Proc Natl Acad Sci U S A. (2017) 114:10584–9. doi: 10.1073/pnas.1709210114
50. Ekwueme EC, Mohiuddin M, Yarborough JA, Brolinson PG, Docheva D, Fernandes HAM, et al. Prolotherapy induces an inflammatory response in human tenocytes in vitro. Clin Orthop Relat Res. (2017) 475:2117–27. doi: 10.1007/s11999-017-5370-1
51. Caradec J, Kharmate G, Hosseini-Beheshti E, Adomat H, Gleave M, Guns E. Reproducibility and efficiency of serum-derived exosome extraction methods. Clin Biochem. (2014) 47:1286–92. doi: 10.1016/j.clinbiochem.2014.06.011
52. Iliescu FS, Vrtacnik D, Neuzil P, Iliescu C. Microfluidic technology for clinical applications of exosomes. Micromachines. (2019) 10:392. doi: 10.3390/mi10060392
53. Kanwar SS, Dunlay CJ, Simeone DM, Nagrath S. Microfluidic device (ExoChip) for on-chip isolation, quantification and characterization of circulating exosomes. Lab Chip. (2014) 14:1891–900. doi: 10.1039/C4LC00136B
54. Kim GB, Shon OJ, Seo MS, Choi Y, Park WT, Lee GW. Mesenchymal stem cell-derived exosomes and their therapeutic potential for osteoarthritis. Biology. (2021) 10:285. doi: 10.3390/biology10040285
55. Li P, Kaslan M, Lee SH, Yao J, Gao Z. Progress in exosome isolation techniques. Theranostics. (2017) 7:789–804. doi: 10.7150/thno.18133
56. Whitesides GM. The origins and the future of microfluidics. Nature. (2006) 442:368–73. doi: 10.1038/nature05058
57. Chiriaco MS, Bianco M, Nigro A, Primiceri E, Ferrara F, Romano A, et al. Lab-on-chip for exosomes and microvesicles detection and characterization. Sensors. (2018) 18:3175. doi: 10.3390/s18103175
58. Zhu Q, Heon M, Zhao Z, He M. Microfluidic engineering of exosomes: editing cellular messages for precision therapeutics. Lab Chip. (2018) 18:1690–703. doi: 10.1039/C8LC00246K
59. Ng JM, Gitlin I, Stroock AD, Whitesides GM. Components for integrated poly(dimethylsiloxane) microfluidic systems. Electrophoresis. (2002) 23:3461–73. doi: 10.1002/1522-2683(200210)23:20<3461::AIDELPS3461>3.0.CO;2-8
60. Cheruvanky A, Zhou H, Pisitkun T, Kopp JB, Knepper MA, Yuen PS, et al. Rapid isolation of urinary exosomal biomarkers using a nanomembrane ultrafiltration concentrator. Am J Physiol Renal Physiol. (2007) 292:F1657–61. doi: 10.1152/ajprenal.00434.2006
61. Mizuno H. Adipose-derived stem cells for regenerative medicine in the field of plastic and reconstructive surgery. J Oral Biosci. (2013) 55:132–6. doi: 10.1016/j.job.2013.04.005
62. Kurian TK, Banik S, Gopal D, Chakrabarti S, Mazumder N. Elucidating methods for isolation and quantification of exosomes: a review. Mol Biotechnol. (2021) 63:249–66. doi: 10.1007/s12033-021-00300-3
63. Wang C, Zhang C, Liu L, AX, Chen B, Li Y, et al. Macrophage-derived mir-155-containing exosomes suppress fibroblast proliferation and promote fibroblast inflammation during cardiac injury. Mol Ther. (2017) 25:192–204. doi: 10.1016/j.ymthe.2016.09.001
64. Salafi T, Zeming KK, Zhang Y. Advancements in microfluidics for nanoparticle separation. Lab Chip. (2016) 17:11–33. doi: 10.1039/C6LC01045H
65. Li X, Corbett AL, Taatizadeh E, Tasnim N, Little JP, Garnis C, et al. Challenges and opportunities in exosome research-Perspectives from biology, engineering, and cancer therapy. APL Bioeng. (2019) 3:011503. doi: 10.1063/1.5087122
66. Toribio V, Morales S, Lopez-Martin S, Cardenes B, Cabanas C, Yanez-Mo M. Development of a quantitative method to measure EV uptake. Sci Rep. (2019) 9:10522. doi: 10.1038/s41598-019-47023-9
67. Feng D, Zhao WL, Ye YY, Bai XC, Liu RQ, Chang LF, et al. Cellular internalization of exosomes occurs through phagocytosis. Traffic. (2010) 11:675–87. doi: 10.1111/j.1600-0854.2010.01041.x
68. Costa Verdera H, Gitz-Francois JJ, Schiffelers RM, Vader P. Cellular uptake of extracellular vesicles is mediated by clathrin-independent endocytosis and macropinocytosis. J Control Release. (2017) 266:100–8. doi: 10.1016/j.jconrel.2017.09.019
69. Fitzner D, Schnaars M, Van Rossum D, Krishnamoorthy G, Dibaj P, Bakhti M, et al. Selective transfer of exosomes from oligodendrocytes to microglia by macropinocytosis. J Cell Sci. (2011) 124:447–58. doi: 10.1242/jcs.074088
70. Stephens L, Ellson C, Hawkins P. Roles of PI3Ks in leukocyte chemotaxis and phagocytosis. Curr Opin Cell Biol. (2002) 14:203–13. doi: 10.1016/S0955-0674(02)00311-3
71. Sezgin E, Levental I, Mayor S, Eggeling C. The mystery of membrane organization: composition, regulation and roles of lipid rafts. Nat Rev Mol Cell Biol. (2017) 18:361–74. doi: 10.1038/nrm.2017.16
72. Jahn R, Lang T, Südhof TC. Membrane fusion. Cell. (2003) 112:519–33. doi: 10.1016/S0092-8674(03)00112-0
73. Van Der Meel R, Fens MH, Vader P, Van Solinge WW, Eniola-Adefeso O, Schiffelers RM. Extracellular vesicles as drug delivery systems: lessons from the liposome field. J Control Release. (2014) 195:72–85. doi: 10.1016/j.jconrel.2014.07.049
74. Li SP, Lin ZX, Jiang XY, Yu XY. Exosomal cargo-loading and synthetic exosome-mimics as potential therapeutic tools. Acta Pharmacol Sin. (2018) 39:542–51. doi: 10.1038/aps.2017.178
75. Hessvik NP, Llorente A. Current knowledge on exosome biogenesis and release. Cell Mol Life Sci. (2018) 75:193–208. doi: 10.1007/s00018-017-2595-9
76. Juan T, Furthauer M. Biogenesis and function of ESCRT-dependent extracellular vesicles. Semin Cell Dev Biol. (2018) 74:66–77. doi: 10.1016/j.semcdb.2017.08.022
77. Baietti MF, Zhang Z, Mortier E, Melchior A, Degeest G, Geeraerts A, et al. Syndecan-syntenin-ALIX regulates the biogenesis of exosomes. Nat Cell Biol. (2012) 14:677–85. doi: 10.1038/ncb2502
78. Tamai K, Tanaka N, Nakano T, Kakazu E, Kondo Y, Inoue J, et al. Exosome secretion of dendritic cells is regulated by Hrs, an ESCRT-0 protein. Biochem Biophys Res Commun. (2010) 399:384–90. doi: 10.1016/j.bbrc.2010.07.083
79. Colombo M, Moita C, Van Niel G, Kowal J, Vigneron J, Benaroch P, et al. Analysis of ESCRT functions in exosome biogenesis, composition and secretion highlights the heterogeneity of extracellular vesicles. J Cell Sci. (2013) 126:5553–65. doi: 10.1242/jcs.128868
80. Kowal J, Tkach M, Thery C. Biogenesis and secretion of exosomes. Curr Opin Cell Biol. (2014) 29:116–25. doi: 10.1016/j.ceb.2014.05.004
81. Villarroya-Beltri C, Baixauli F, Gutierrez-Vazquez C, Sanchez-Madrid F, Mittelbrunn M. Sorting it out: regulation of exosome loading. Semin Cancer Biol. (2014) 28:3–13. doi: 10.1016/j.semcancer.2014.04.009
82. Liang Y, Eng WS, Colquhoun DR, Dinglasan RR, Graham DR, Mahal LK. Complex N-linked glycans serve as a determinant for exosome/microvesicle cargo recruitment. J Biol Chem. (2014) 289:32526–37. doi: 10.1074/jbc.M114.606269
83. Skotland T, Sandvig K, Llorente A. Lipids in exosomes: current knowledge and the way forward. Prog Lipid Res. (2017) 66:30–41. doi: 10.1016/j.plipres.2017.03.001
84. Llorente A, Skotland T, Sylvanne T, Kauhanen D, Rog T, Orlowski A, et al. Molecular lipidomics of exosomes released by PC-3 prostate cancer cells. Biochim Biophys Acta. (2013) 1831:1302–9. doi: 10.1016/j.bbalip.2013.04.011
85. Mentkowski KI, Lang JK. Exosomes engineered to express a cardiomyocyte binding peptide demonstrate improved cardiac retention in vivo. Sci Rep. (2019) 9:10041. doi: 10.1038/s41598-019-46407-1
86. Garaeva L, Kamyshinsky R, Kil Y, Varfolomeeva E, Verlov N, Komarova E, et al. Delivery of functional exogenous proteins by plant-derived vesicles to human cells in vitro. Sci Rep. (2021) 11:6489. doi: 10.1038/s41598-021-85833-y
87. Minciacchi VR, Freeman MR, Di Vizio D. Extracellular vesicles in cancer: exosomes, microvesicles and the emerging role of large oncosomes. Semin Cell Dev Biol. (2015) 40:41–51. doi: 10.1016/j.semcdb.2015.02.010
88. Xunian Z, Kalluri R. Biology and therapeutic potential of mesenchymal stem cell-derived exosomes. Cancer Sci. (2020) 111:3100–10. doi: 10.1111/cas.14563
89. Wu R, Gao W, Yao K, Ge J. Roles of exosomes derived from immune cells in cardiovascular diseases. Front Immunol. (2019) 10:648. doi: 10.3389/fimmu.2019.00648
90. Ma ZJ, Yang JJ, Lu YB, Liu ZY, Wang XX. Mesenchymal stem cell-derived exosomes: Toward cell-free therapeutic strategies in regenerative medicine. World J Stem Cells. (2020) 12:814–40. doi: 10.4252/wjsc.v12.i8.814
91. Reis LA, Borges FT, Simoes MJ, Borges AA, Sinigaglia-Coimbra R, Schor N. Bone marrow-derived mesenchymal stem cells repaired but did not prevent gentamicin-induced acute kidney injury through paracrine effects in rats. PLoS ONE. (2012) 7:e44092. doi: 10.1371/journal.pone.0044092
92. Keller S, Ridinger J, Rupp AK, Janssen JW, Altevogt P. Body fluid derived exosomes as a novel template for clinical diagnostics. J Transl Med. (2011) 9:86. doi: 10.1186/1479-5876-9-86
93. Pritchard A, Tousif S, Wang Y, Hough K, Khan S, Strenkowski J, et al. Lung tumor cell-derived exosomes promote M2 macrophage polarization. Cells. (2020) 9:1303. doi: 10.3390/cells9051303
94. Sinha D, Roy S, Saha P, Chatterjee N, Bishayee A. Trends in Research on Exosomes in Cancer Progression and Anticancer Therapy. Cancers. (2021) 13:326. doi: 10.3390/cancers13020326
95. Akers JC, Gonda D, Kim R, Carter BS, Chen CC. Biogenesis of extracellular vesicles (EV): exosomes, microvesicles, retrovirus-like vesicles, and apoptotic bodies. J Neurooncol. (2013) 113:1–11. doi: 10.1007/s11060-013-1084-8
96. Yang JM, Gould SJ. The cis-acting signals that target proteins to exosomes and microvesicles. Biochem Soc Trans. (2013) 41:277–82. doi: 10.1042/BST20120275
97. Pant S, Hilton H, Burczynski ME. The multifaceted exosome: biogenesis, role in normal and aberrant cellular function, and frontiers for pharmacological and biomarker opportunities. Biochem Pharmacol. (2012) 83:1484–94. doi: 10.1016/j.bcp.2011.12.037
98. Ostrowski M, Carmo NB, Krumeich S, Fanget I, Raposo G, Savina A, et al. Rab27a and Rab27b control different steps of the exosome secretion pathway. Nat Cell Biol. (2010) 12:19–30. doi: 10.1038/ncb2000
99. Savina A, Vidal M, Colombo MI. The exosome pathway in K562 cells is regulated by Rab11. J Cell Sci. (2002) 115:2505–15 doi: 10.1242/jcs.115.12.2505
100. Hsu C, Morohashi Y, Yoshimura S, Manrique-Hoyos N, Jung S, Lauterbach MA, et al. Regulation of exosome secretion by Rab35 and its GTPase-activating proteins TBC1D10A-C. J Cell Biol. (2010) 189:223–32. doi: 10.1083/jcb.200911018
101. Vidal MJ, Stahl PD. The small GTP-binding proteins Rab4 and ARF are associated with released exosomes during reticulocyte maturation. Eur J Cell Biol. (1993) 60:261–7.
102. Ekstrom EJ, Bergenfelz C, Von Bulow V, Serifler F, Carlemalm E, Jonsson G, et al. WNT5A induces release of exosomes containing pro-angiogenic and immunosuppressive factors from malignant melanoma cells. Mol Cancer. (2014) 13:88. doi: 10.1186/1476-4598-13-88
103. Phuyal S, Hessvik NP, Skotland T, Sandvig K, Llorente A. Regulation of exosome release by glycosphingolipids and flotillins. FEBS J. (2014) 281:2214–27. doi: 10.1111/febs.12775
104. Blanc L, Vidal M. New insights into the function of Rab GTPases in the context of exosomal secretion. Small GTPases. (2018) 9:95–106. doi: 10.1080/21541248.2016.1264352
105. Lespagnol A, Duflaut D, Beekman C, Blanc L, Fiucci G, Marine JC, et al. Exosome secretion, including the DNA damage-induced p53-dependent secretory pathway, is severely compromised in TSAP6/Steap3-null mice. Cell Death Differ. (2008) 15:1723–33. doi: 10.1038/cdd.2008.104
106. Heijnen HFG, Schiel AE, Fijnheer R, Geuze HJ, Sixma JJ. Activated platelets release two types of membrane vesicles: microvesicles by surface shedding and exosomes derived from exocytosis of multivesicular bodies and ?-granules. Blood. (1999) 94:3791–9. doi: 10.1182/blood.V94.11.3791
107. Lin F, Zeng Z, Song Y, Li L, Wu Z, Zhang X, et al. YBX-1 mediated sorting of miR-133 into hypoxia/reoxygenation-induced EPC-derived exosomes to increase fibroblast angiogenesis and MEndoT. Stem Cell Res Ther. (2019) 10:263. doi: 10.1186/s13287-019-1377-8
108. Gurung S, Perocheau D, Touramanidou L, Baruteau J. The exosome journey: from biogenesis to uptake and intracellular signalling. Cell Commun Signal. (2021) 19:47. doi: 10.1186/s12964-021-00730-1
109. Chaineau M, Danglot L, Galli T. Multiple roles of the vesicular-SNARE TI-VAMP in post-Golgi and endosomal trafficking. FEBS Lett. (2009) 583:3817–26. doi: 10.1016/j.febslet.2009.10.026
110. Fader CM, Sanchez DG, Mestre MB, Colombo MI. TI-VAMP/VAMP7 and VAMP3/cellubrevin: two v-SNARE proteins involved in specific steps of the autophagy/multivesicular body pathways. Biochim Biophys Acta. (2009) 1793:1901–16. doi: 10.1016/j.bbamcr.2009.09.011
111. Srivatsav AT, Kapoor S. The emerging world of membrane vesicles: functional relevance, theranostic avenues and tools for investigating membrane function. Front Mol Biosci. (2021) 8:640355. doi: 10.3389/fmolb.2021.640355
112. Mathivanan S, Simpson RJ. ExoCarta: a compendium of exosomal proteins and RNA. Proteomics. (2009) 9:4997–5000. doi: 10.1002/pmic.200900351
113. Kim DK, Kang B, Kim OY, Choi DS, Lee J, Kim SR, et al. EVpedia: an integrated database of high-throughput data for systemic analyses of extracellular vesicles. J Extracell Vesicles. (2013) 2:20384. doi: 10.3402/jev.v2i0.20384
114. Thakur BK, Zhang H, Becker A, Matei I, Huang Y, Costa-Silva B, et al. Double-stranded DNA in exosomes: a novel biomarker in cancer detection. Cell Res. (2014) 24:766–9. doi: 10.1038/cr.2014.44
115. Halkein J, Tabruyn SP, Ricke-Hoch M, Haghikia A, Nguyen NQ, Scherr M, et al. MicroRNA-146a is a therapeutic target and biomarker for peripartum cardiomyopathy. J Clin Invest. (2013) 123:2143–54. doi: 10.1172/JCI64365
116. Saeedi S, Israel S, Nagy C, Turecki G. The emerging role of exosomes in mental disorders. Transl Psychiatry. (2019) 9:122. doi: 10.1038/s41398-019-0459-9
117. Poe AJ, Knowlton AA. Exosomes as agents of change in the cardiovascular system. J Mol Cell Cardiol. (2017) 111:40–50. doi: 10.1016/j.yjmcc.2017.08.002
118. Zhang J, Cui X, Guo J, Cao C, Zhang Z, Wang B, et al. Small but significant: Insights and new perspectives of exosomes in cardiovascular disease. J Cell Mol Med. (2020) 24:8291–303. doi: 10.1111/jcmm.15492
119. Hare JM, Chaparro SV. Cardiac regeneration and stem cell therapy. Curr Opin Organ Transplant. (2008) 13:536–42. doi: 10.1097/MOT.0b013e32830fdfc4
120. Kwon SG, Kwon YW, Lee TW, Park GT, Kim JH. Recent advances in stem cell therapeutics and tissue engineering strategies. Biomater Res. (2018) 22:36. doi: 10.1186/s40824-018-0148-4
121. Singh VK, Saini A, Kalsan M, Kumar N, Chandra R. Describing the stem cell potency: the various methods of functional assessment and in silico diagnostics. Front Cell Dev Biol. (2016) 4:134. doi: 10.3389/fcell.2016.00134
122. Hipp J, Atala A. Sources of stem cells for regenerative medicine. Stem Cell Rev. (2008) 4:3–11. doi: 10.1007/s12015-008-9010-8
123. Durrani S, Konoplyannikov M, Ashraf M, Haider KH. Skeletal myoblasts for cardiac repair. Regen Med. (2010) 5:919–32. doi: 10.2217/rme.10.65
124. Afzal MR, Samanta A, Shah ZI, Jeevanantham V, Abdel-Latif A, Zuba-Surma EK, et al. Adult bone marrow cell therapy for ischemic heart disease: evidence and insights from randomized controlled trials. Circ Res. (2015) 117:558–75. doi: 10.1161/CIRCRESAHA.114.304792
125. Fan C, Zhang E, Joshi J, Yang J, Zhang J, Zhu W. Utilization of human induced pluripotent stem cells for cardiac repair. Front Cell Dev Biol. (2020) 8:36. doi: 10.3389/fcell.2020.00036
126. Mudyanadzo TA. Endothelial progenitor cells and cardiovascular correlates. Cureus. (2018) 10:e3342. doi: 10.7759/cureus.3342
127. Sebastiao MJ, Serra M, Pereira R, Palacios I, Gomes-Alves P, Alves PM. Human cardiac progenitor cell activation and regeneration mechanisms: exploring a novel myocardial ischemia/reperfusion in vitro model. Stem Cell Res Ther. (2019) 10:77. doi: 10.1186/s13287-019-1174-4
128. Barreto S, Hamel L, Schiatti T, Yang Y, George V. Cardiac progenitor cells from stem cells: learning from genetics and biomaterials. Cells. (2019) 8:1536. doi: 10.3390/cells8121536
129. Balbi C, Vassalli G. Exosomes: beyond stem cells for cardiac protection and repair. Stem Cells. (2020) 38:1387–99. doi: 10.1002/stem.3261
130. Muller P, Lemcke H, David R. Stem cell therapy in heart diseases - cell types, mechanisms and improvement strategies. Cell Physiol Biochem. (2018) 48:2607–55. doi: 10.1159/000492704
131. Willerson JT. Stem cell therapy for cardiovascular diseases: a progressive journey. Curr Opin Cardiol. (2015) 30:205–12. doi: 10.1097/HCO.0000000000000156
132. Abou-Saleh H, Zouein FA, El-Yazbi A, Sanoudou D, Raynaud C, Rao C, et al. The march of pluripotent stem cells in cardiovascular regenerative medicine. Stem Cell Res Ther. (2018) 9:201. doi: 10.1186/s13287-018-0947-5
133. Kurtzwald-Josefson E, Zeevi-Levin N, Rubchevsky V, Bechar Erdman N, Schwartz Rohaker O, Nahum O, et al. Cardiac fibroblast-induced pluripotent stem cell-derived exosomes as a potential therapeutic mean for heart failure. Int J Mol Sci. (2020) 21:7215. doi: 10.3390/ijms21197215
134. Malik N, Rao MS. A review of the methods for human iPSC derivation. Methods Mol Biol. (2013) 997:23–33. doi: 10.1007/978-1-62703-348-0_3
135. Fischer B, Meier A, Dehne A, Salhotra A, Tran TA, Neumann S, et al. A complete workflow for the differentiation and the dissociation of hiPSC-derived cardiospheres. Stem Cell Res. (2018) 32:65–72. doi: 10.1016/j.scr.2018.08.015
136. Jung JH, Fu X, Yang PC. Exosomes generated from iPSC-derivatives: new direction for stem cell therapy in human heart diseases. Circ Res. (2017) 120:407–17. doi: 10.1161/CIRCRESAHA.116.309307
137. Yeo RW, Lai RC, Zhang B, Tan SS, Yin Y, Teh BJ, et al. Mesenchymal stem cell: an efficient mass producer of exosomes for drug delivery. Adv Drug Deliv Rev. (2013) 65:336–41. doi: 10.1016/j.addr.2012.07.001
138. Nikfarjam S, Rezaie J, Zolbanin NM, Jafari R. Mesenchymal stem cell derived-exosomes: a modern approach in translational medicine. J Transl Med. (2020) 18:449. doi: 10.1186/s12967-020-02622-3
139. Caplan AI, Dennis JE. Mesenchymal stem cells as trophic mediators. J Cell Biochem. (2006) 98:1076–84. doi: 10.1002/jcb.20886
140. Samsonraj RM, Raghunath M, Nurcombe V, Hui JH, Van Wijnen AJ, Cool SM. Concise review: multifaceted characterization of human mesenchymal stem cells for use in regenerative medicine. Stem Cells Transl Med. (2017) 6:2173–85. doi: 10.1002/sctm.17-0129
141. Cui X, He Z, Liang Z, Chen Z, Wang H, Zhang J. Exosomes from adipose-derived mesenchymal stem cells protect the myocardium against ischemia/reperfusion injury through wnt/beta-catenin signaling pathway. J Cardiovasc Pharmacol. (2017) 70:225–31. doi: 10.1097/FJC.0000000000000507
142. Kang K, Ma R, Cai W, Huang W, Paul C, Liang J, et al. Exosomes secreted from CXCR4 overexpressing mesenchymal stem cells promote cardioprotection via akt signaling pathway following myocardial infarction. Stem Cells Int. (2015) 2015:659890. doi: 10.1155/2015/659890
143. Joo HS, Suh JH, Lee HJ, Bang ES, Lee JM. Current knowledge and future perspectives on mesenchymal stem cell-derived exosomes as a new therapeutic agent. Int J Mol Sci. (2020) 21:727. doi: 10.3390/ijms21030727
144. Golpanian S, Wolf A, Hatzistergos KE, Hare JM. Rebuilding the damaged heart: mesenchymal stem cells, cell-based therapy, and engineered heart tissue. Physiol Rev. (2016) 96:1127–68. doi: 10.1152/physrev.00019.2015
145. Barile L, Lionetti V, Cervio E, Matteucci M, Gherghiceanu M, Popescu LM, et al. Extracellular vesicles from human cardiac progenitor cells inhibit cardiomyocyte apoptosis and improve cardiac function after myocardial infarction. Cardiovasc Res. (2014) 103:530–41. doi: 10.1093/cvr/cvu167
146. Kapur SK., Katz AJ, Review of the adipose derived stem cell secretome. Biochimie. (2013) 95:2222–8. doi: 10.1016/j.biochi.2013.06.001
147. Argentati C, Morena F, Bazzucchi M, Armentano I, Emiliani C, Martino S. Adipose stem cell translational applications: from bench-to-bedside. Int J Mol Sci. (2018) 19:3475. doi: 10.3390/ijms19113475
148. Lasocka I, Jastrzebska E, Szulc-Dabrowska L, Skibniewski M, Pasternak I., Kalbacova MH, et al. The effects of graphene and mesenchymal stem cells in cutaneous wound healing and their putative action mechanism. Int J Nanomedicine. (2019) 14:2281–99. doi: 10.2147/IJN.S190928
149. Han YD, Bai Y, Yan XL, Ren J, Zeng Q, Li XD, et al. Co-transplantation of exosomes derived from hypoxia-preconditioned adipose mesenchymal stem cells promotes neovascularization and graft survival in fat grafting. Biochem Biophys Res Commun. (2018) 497:305–12. doi: 10.1016/j.bbrc.2018.02.076
150. Zhang W, Bai X, Zhao B, Li Y, Zhang Y, Li Z, et al. Cell-free therapy based on adipose tissue stem cell-derived exosomes promotes wound healing via the PI3K/Akt signaling pathway. Exp Cell Res. (2018) 370:333–42. doi: 10.1016/j.yexcr.2018.06.035
151. Ribeiro-Rodrigues TM, Laundos TL, Pereira-Carvalho R, Batista-Almeida D, Pereira R, Coelho-Santos V, et al. Exosomes secreted by cardiomyocytes subjected to ischaemia promote cardiac angiogenesis. Cardiovasc Res. (2017) 113:1338–50. doi: 10.1093/cvr/cvx118
152. Tao L, Bei Y, Chen P, Lei Z, Fu S, Zhang H, et al. Crucial role of miR-433 in regulating cardiac fibrosis. Theranostics. (2016) 6:2068–83. doi: 10.7150/thno.15007
153. Zhaofu L, Dongqing C. Cardiac telocytes in regeneration of myocardium after myocardial infarction. Adv Exp Med Biol. (2016) 913:229–39. doi: 10.1007/978-981-10-1061-3_15
154. Fertig ET, Gherghiceanu M, Popescu LM. Extracellular vesicles release by cardiac telocytes: electron microscopy and electron tomography. J Cell Mol Med. (2014) 18:1938–43. doi: 10.1111/jcmm.12436
155. Kervadec A, Bellamy V, El Harane N, Arakelian L, Vanneaux V, Cacciapuoti I, et al. Cardiovascular progenitor-derived extracellular vesicles recapitulate the beneficial effects of their parent cells in the treatment of chronic heart failure. J Heart Lung Transplant. (2016) 35:795–807. doi: 10.1016/j.healun.2016.01.013
156. Khan M, Nickoloff E, Abramova T, Johnson J, Verma SK, Krishnamurthy P, et al. Embryonic stem cell-derived exosomes promote endogenous repair mechanisms and enhance cardiac function following myocardial infarction. Circ Res. (2015) 117:52–64. doi: 10.1161/CIRCRESAHA.117.305990
157. Jung M, Ma Y, Iyer RP, Deleon-Pennell KY, Yabluchanskiy A, Garrett MR, et al. IL-10 improves cardiac remodeling after myocardial infarction by stimulating M2 macrophage polarization and fibroblast activation. Basic Res Cardiol. (2017) 112:33. doi: 10.1007/s00395-017-0622-5
158. El Harane N, Kervadec A, Bellamy V, Pidial L, Neametalla HJ, Perier MC, et al. Acellular therapeutic approach for heart failure: in vitro production of extracellular vesicles from human cardiovascular progenitors. Eur Heart J. (2018) 39:1835–47. doi: 10.1093/eurheartj/ehy012
159. Borden A, Kurian J, Nickoloff E, Yang Y, Troupes CD, Ibetti J, et al. Transient introduction of miR-294 in the heart promotes cardiomyocyte cell cycle reentry after injury. Circ Res. (2019) 125:14–25. doi: 10.1161/CIRCRESAHA.118.314223
160. Barile L, Cervio E, Lionetti V, Milano G, Ciullo A, Biemmi V, et al. Cardioprotection by cardiac progenitor cell-secreted exosomes: role of pregnancy-associated plasma protein-A. Cardiovasc Res. (2018) 114:992–1005. doi: 10.1093/cvr/cvy055
161. Davidson SM, Yellon DM. Exosomes and cardioprotection-a critical analysis. Mol Aspects Med. (2018) 60:104–14. doi: 10.1016/j.mam.2017.11.004
162. Lai RC, Arslan F, Lee MM, Sze NS, Choo A, Chen TS, et al. Exosome secreted by MSC reduces myocardial ischemia/reperfusion injury. Stem Cell Res. (2010) 4:214–22. doi: 10.1016/j.scr.2009.12.003
163. Arslan F, Lai RC, Smeets MB, Akeroyd L, Choo A, Aguor EN, et al. Mesenchymal stem cell-derived exosomes increase ATP levels, decrease oxidative stress and activate PI3K/Akt pathway to enhance myocardial viability and prevent adverse remodeling after myocardial ischemia/reperfusion injury. Stem Cell Res. (2013) 10:301–12. doi: 10.1016/j.scr.2013.01.002
164. Liu Y, Wang M, Liang Y, Wang C, Naruse K, Takahashi K. Treatment of oxidative stress with exosomes in myocardial ischemia. Int J Mol Sci. (2021) 22:1729. doi: 10.3390/ijms22041729
165. Yuan Y, Du W, Liu J, Ma W, Zhang L, Du Z, et al. Stem cell-derived exosome in cardiovascular diseases: macro roles of micro particles. Front Pharmacol. (2018) 9:547. doi: 10.3389/fphar.2018.00547
166. De Andrade Mello P, Coutinho-Silva R, Savio LEB. Multifaceted effects of extracellular adenosine triphosphate and adenosine in the tumor-host interaction and therapeutic perspectives. Front Immunol. (2017) 8:1526. doi: 10.3389/fimmu.2017.01526
167. Chen G, Xu C, Gillette TG, Huang T, Huang P, Li Q, et al. Cardiomyocyte-derived small extracellular vesicles can signal eNOS activation in cardiac microvascular endothelial cells to protect against Ischemia/Reperfusion injury. Theranostics. (2020) 10:11754–74. doi: 10.7150/thno.43163
168. Khaksar M, Sayyari M, Rezaie J, Pouyafar A, Montazersaheb S, Rahbarghazi R. High glucose condition limited the angiogenic/cardiogenic capacity of murine cardiac progenitor cells in in vitro and in vivo milieu. Cell Biochem Funct. (2018) 36:346–56. doi: 10.1002/cbf.3354
169. Wang K, Jiang Z, Webster KA, Chen J, Hu H, Zhou Y, et al. Enhanced cardioprotection by human endometrium mesenchymal stem cells driven by exosomal MicroRNA-21. Stem Cells Transl Med. (2017) 6:209–22. doi: 10.5966/sctm.2015-0386
170. Lemcke H, Gaebel R, Skorska A, Voronina N, Lux CA, Petters J, et al. Mechanisms of stem cell based cardiac repair-gap junctional signaling promotes the cardiac lineage specification of mesenchymal stem cells. Sci Rep. (2017) 7:9755. doi: 10.1038/s41598-017-10122-6
171. Fan XL, Zhang Y, Li X, Fu QL. Mechanisms underlying the protective effects of mesenchymal stem cell-based therapy. Cell Mol Life Sci. (2020) 77:2771–94. doi: 10.1007/s00018-020-03454-6
172. Adamiak M, Sahoo S. Exosomes in myocardial repair: advances and challenges in the development of next-generation therapeutics. Mol Ther. (2018) 26:1635–43. doi: 10.1016/j.ymthe.2018.04.024
173. Burt R, Pearce W, Luo K, Oyama Y, Davidson C., Beohar N, et al. Hematopoietic stem cell transplantation for cardiac and peripheral vascular disease. Bone Marrow Transplant. (2003) 32:S29–31. doi: 10.1038/sj.bmt.1704177
174. Lemcke H, Voronina N, Steinhoff G, David R. Recent progress in stem cell modification for cardiac regeneration. Stem Cells Int. (2018) 2018:1909346. doi: 10.1155/2018/1909346
175. Tseliou E, Fouad J, Reich H, Slipczuk L, De Couto G, Aminzadeh M, et al. Fibroblasts rendered antifibrotic, antiapoptotic, and angiogenic by priming with cardiosphere-derived extracellular membrane vesicles. J Am Coll Cardiol. (2015) 66:599–611. doi: 10.1016/j.jacc.2015.05.068
176. Marban E. Breakthroughs in cell therapy for heart disease: focus on cardiosphere-derived cells. Mayo Clin Proc. (2014) 89:850–8. doi: 10.1016/j.mayocp.2014.02.014
177. Reed DM, Foldes G, Harding SE, Mitchell JA. Stem cell-derived endothelial cells for cardiovascular disease: a therapeutic perspective. Br J Clin Pharmacol. (2013) 75:897–906. doi: 10.1111/j.1365-2125.2012.04361.x
178. Dzau VJ, Gnecchi M, Pachori AS, Morello F, Melo LG. Therapeutic potential of endothelial progenitor cells in cardiovascular diseases. Hypertension. (2005) 46:7–18. doi: 10.1161/01.HYP.0000168923.92885.f7
179. Sahoo S, Klychko E, Thorne T, Misener S, Schultz KM, Millay M, et al. Exosomes from human CD34(+) stem cells mediate their proangiogenic paracrine activity. Circ Res. (2011) 109:724–8. doi: 10.1161/CIRCRESAHA.111.253286
180. Haider KH, Aramini B. Mircrining the injured heart with stem cell-derived exosomes: an emerging strategy of cell-free therapy. Stem Cell Res Ther. (2020) 11:23. doi: 10.1186/s13287-019-1548-7
181. Zuo R, Kong L, Wang M, Wang W, Xu J, Chai Y, et al. Exosomes derived from human CD34(+) stem cells transfected with miR-26a prevent glucocorticoid-induced osteonecrosis of the femoral head by promoting angiogenesis and osteogenesis. Stem Cell Res Ther. (2019) 10:321. doi: 10.1186/s13287-019-1426-3
182. Bian X, Ma K, Zhang C, Fu X. Therapeutic angiogenesis using stem cell-derived extracellular vesicles: an emerging approach for treatment of ischemic diseases. Stem Cell Res Ther. (2019) 10:158. doi: 10.1186/s13287-019-1276-z
183. Li TS, Kubo M, Ueda K, Murakami M, Mikamo A, Hamano K. Impaired angiogenic potency of bone marrow cells from patients with advanced age, anemia, and renal failure. J Thorac Cardiovasc Surg. (2010) 139:459–65. doi: 10.1016/j.jtcvs.2009.07.053
184. Wei L, Wei ZZ, Jiang MQ, Mohamad O, Yu SP. Stem cell transplantation therapy for multifaceted therapeutic benefits after stroke. Prog Neurobiol. (2017) 157:49–78. doi: 10.1016/j.pneurobio.2017.03.003
185. Chang YH, Wu KC, Harn HJ, Lin SZ, Ding DC. Exosomes and Stem Cells in Degenerative Disease Diagnosis and Therapy. Cell Transplant. (2018) 27:349–63. doi: 10.1177/0963689717723636
186. Charitos IA, Ballini A, Cantore S, Boccellino M, Di Domenico M., Borsani E, et al. Stem cells: a historical review about biological, religious, and ethical issues. Stem Cells Int. (2021) 2021:9978837. doi: 10.1155/2021/9978837
187. Chen CJ, Tseng CC, Yen JH, Chang JG, Chou WC, Chu HW, et al. ABCG2 contributes to the development of gout and hyperuricemia in a genome-wide association study. Sci Rep. (2018) 8:3137. doi: 10.1038/s41598-018-21425-7
188. Rani S, Ryan AE, Griffin MD, Ritter T. Mesenchymal stem cell-derived extracellular vesicles: toward cell-free therapeutic applications. Mol Ther. (2015) 23:812–23. doi: 10.1038/mt.2015.44
189. Philippen LE, Dirkx E, Wit JB, Burggraaf K, De Windt LJ, Da Costa Martins PA. Antisense MicroRNA therapeutics in cardiovascular disease: quo vadis? Mol Ther. (2015) 23:1810–8. doi: 10.1038/mt.2015.133
190. Deng L, Blanco FJ, Stevens H, Lu R, Caudrillier A, Mcbride M, et al. MicroRNA-143 activation regulates smooth muscle and endothelial cell crosstalk in pulmonary arterial hypertension. Circ Res. (2015) 117:870–83. doi: 10.1161/CIRCRESAHA.115.306806
191. Mathivanan S, Ji H, Simpson RJ. Exosomes: extracellular organelles important in intercellular communication. J Proteomics. (2010) 73:1907–20. doi: 10.1016/j.jprot.2010.06.006
192. Ahmadi M, Rezaie J. Ageing and mesenchymal stem cells derived exosomes: Molecular insight and challenges. Cell Biochem Funct. (2021) 39:60–6. doi: 10.1002/cbf.3602
193. Rezaie J, Mehranjani MS, Rahbarghazi R, Shariatzadeh MA. Angiogenic and restorative abilities of human mesenchymal stem cells were reduced following treatment with serum from diabetes mellitus type 2 patients. J Cell Biochem. (2018) 119:524–35. doi: 10.1002/jcb.26211
194. Tabet F, Vickers KC, Cuesta Torres LF, Wiese CB, Shoucri BM, Lambert G, et al. HDL-transferred microRNA-223 regulates ICAM-1 expression in endothelial cells. Nat Commun. (2014) 5:3292. doi: 10.1038/ncomms4292
195. Lasser C, O'neil SE, Shelke GV, Sihlbom C, Hansson SF, Gho YS, et al. Exosomes in the nose induce immune cell trafficking and harbour an altered protein cargo in chronic airway inflammation. J Transl Med. (2016) 14:181. doi: 10.1186/s12967-016-0927-4
196. Gonzalez-Calero L, Martin-Lorenzo M, Alvarez-Llamas G. Exosomes: a potential key target in cardio-renal syndrome. Front Immunol. (2014) 5:465. doi: 10.3389/fimmu.2014.00465
197. Howitt J, Hill AF. Exosomes in the pathology of neurodegenerative diseases. J Biol Chem. (2016) 291:26589–97. doi: 10.1074/jbc.R116.757955
198. Record M, Poirot M, Silvente-Poirot S. Emerging concepts on the role of exosomes in lipid metabolic diseases. Biochimie. (2014) 96:67–74. doi: 10.1016/j.biochi.2013.06.016
199. Salem KZ, Moschetta M, Sacco A, Imberti L, Rossi G, Ghobrial IM, et al. Exosomes in tumor angiogenesis. Methods Mol Biol. (2016) 1464:25–34. doi: 10.1007/978-1-4939-3999-2_3
200. Chen L, Wang Y, Pan Y, Zhang L, Shen C, Qin G, et al. Cardiac progenitor-derived exosomes protect ischemic myocardium from acute ischemia/reperfusion injury. Biochem Biophys Res Commun. (2013) 431:566–71. doi: 10.1016/j.bbrc.2013.01.015
201. Gray WD, French KM, Ghosh-Choudhary S, Maxwell JT, Brown ME, Platt MO, et al. Identification of therapeutic covariant microRNA clusters in hypoxia-treated cardiac progenitor cell exosomes using systems biology. Circ Res. (2015) 116:255–63. doi: 10.1161/CIRCRESAHA.116.304360
202. Ong SG, Lee WH, Huang M, Dey D, Kodo K, Sanchez-Freire V, et al. Cross talk of combined gene and cell therapy in ischemic heart disease: role of exosomal microRNA transfer. Circulation. (2014) 130:S60–9. doi: 10.1161/CIRCULATIONAHA.113.007917
203. Qiu X, Liu J, Zheng C, Su Y, Bao L, Zhu B, et al. Exosomes released from educated mesenchymal stem cells accelerate cutaneous wound healing via promoting angiogenesis. Cell Prolif. (2020) 53:e12830. doi: 10.1111/cpr.12830
204. De Jong OG, Van Balkom BW, Schiffelers RM, Bouten CV, Verhaar MC. Extracellular vesicles: potential roles in regenerative medicine. Front Immunol. (2014) 5:608. doi: 10.3389/fimmu.2014.00608
205. Tibbetts MD, Samuel MA, Chang TS, Ho AC. Stem cell therapy for retinal disease. Curr Opin Ophthalmol. (2012) 23:226–34. doi: 10.1097/ICU.0b013e328352407d
206. Peters PJ, Geuze HJ, Van Der Donk HA, Slot JW, Griffith JM, Stam NJ, et al. Molecules relevant for T cell-target cell interaction are present in cytolytic granules of human T lymphocytes. Eur J Immunol. (1989) 19:1469–75. doi: 10.1002/eji.1830190819
207. Giricz Z, Varga ZV, Baranyai T, Sipos P, Paloczi K, Kittel A, et al. Cardioprotection by remote ischemic preconditioning of the rat heart is mediated by extracellular vesicles. J Mol Cell Cardiol. (2014) 68:75–8. doi: 10.1016/j.yjmcc.2014.01.004
208. Alvarez-Erviti L, Seow Y, Yin H, Betts C, Lakhal S, Wood MJ. Delivery of siRNA to the mouse brain by systemic injection of targeted exosomes. Nat Biotechnol. (2011) 29:341–5. doi: 10.1038/nbt.1807
209. Chen TS, Lai RC, Lee MM, Choo AB, Lee CN, Lim SK. Mesenchymal stem cell secretes microparticles enriched in pre-microRNAs. Nucleic Acids Res. (2010) 38:215–24. doi: 10.1093/nar/gkp857
210. Ibrahim AG, Cheng K, Marban E. Exosomes as critical agents of cardiac regeneration triggered by cell therapy. Stem Cell Reports. (2014) 2:606–19. doi: 10.1016/j.stemcr.2014.04.006
211. Ye M, Ni Q, Qi H, Qian X, Chen J, Guo X, et al. Exosomes derived from human induced pluripotent stem cells-endothelia cells promotes postnatal angiogenesis in mice bearing ischemic limbs. Int J Biol Sci. (2019) 15:158–68. doi: 10.7150/ijbs.28392
212. Syn NL, Wang L, Chow EK, Lim CT, Goh BC. Exosomes in cancer nanomedicine and immunotherapy: prospects and challenges. Trends Biotechnol. (2017) 35:665–76. doi: 10.1016/j.tibtech.2017.03.004
213. Toh WS, Lai RC, Hui JHP, Lim SK. MSC exosome as a cell-free MSC therapy for cartilage regeneration: Implications for osteoarthritis treatment. Semin Cell Dev Biol. (2017) 67:56–64. doi: 10.1016/j.semcdb.2016.11.008
214. Zhang S, Chu WC, Lai RC, Lim SK, Hui JH, Toh WS. Exosomes derived from human embryonic mesenchymal stem cells promote osteochondral regeneration. Osteoarthritis Cartilage. (2016) 24:2135–40. doi: 10.1016/j.joca.2016.06.022
215. Hu GW, Li Q, Niu X, Hu B, Liu J, Zhou SM, et al. Exosomes secreted by human-induced pluripotent stem cell-derived mesenchymal stem cells attenuate limb ischemia by promoting angiogenesis in mice. Stem Cell Res Ther. (2015) 6:10. doi: 10.1186/scrt546
216. Pala R, Anju VT, Dyavaiah M, Busi S, Nauli SM. Nanoparticle-mediated drug delivery for the treatment of cardiovascular diseases. Int J Nanomedicine. (2020) 15:3741–69. doi: 10.2147/IJN.S250872
217. Antimisiaris SG, Mourtas S, Marazioti A. Exosomes and exosome-inspired vesicles for targeted drug delivery. Pharmaceutics. (2018) 10:218. doi: 10.3390/pharmaceutics10040218
218. Tan CY, Lai RC, Wong W, Dan YY, Lim SK, Ho HK. Mesenchymal stem cell-derived exosomes promote hepatic regeneration in drug-induced liver injury models. Stem Cell Res Ther. (2014) 5:76. doi: 10.1186/scrt465
219. Ha D, Yang N, Nadithe V. Exosomes as therapeutic drug carriers and delivery vehicles across biological membranes: current perspectives and future challenges. Acta Pharm Sin B. (2016) 6:287–96. doi: 10.1016/j.apsb.2016.02.001
220. Jiang XC, Gao JQ. Exosomes as novel bio-carriers for gene and drug delivery. Int J Pharm. (2017) 521:167–75. doi: 10.1016/j.ijpharm.2017.02.038
221. Babaei M, Rezaie J, Application of stem cell-derived exosomes in ischemic diseases: opportunity and limitations. J Transl Med. (2021) 19:196. doi: 10.1186/s12967-021-02863-w
222. Fadini GP, Mehta A, Dhindsa DS, Bonora BM, Sreejit G, Nagareddy P, et al. Circulating stem cells and cardiovascular outcomes: from basic science to the clinic. Eur Heart J. (2020) 41:4271–82. doi: 10.1093/eurheartj/ehz923
223. Akbari A, Rezaie J. Potential therapeutic application of mesenchymal stem cell-derived exosomes in SARS-CoV-2 pneumonia. Stem Cell Res Ther. (2020) 11:356. doi: 10.1186/s13287-020-01866-6
224. Salimi L, Akbari A, Jabbari N, Mojarad B, Vahhabi A, Szafert S, et al. Synergies in exosomes and autophagy pathways for cellular homeostasis and metastasis of tumor cells. Cell Biosci. (2020) 10:64. doi: 10.1186/s13578-020-00426-y
225. Casieri V, Matteucci M, Pasanisi EM, Papa A, Barile L, Fritsche-Danielson R, et al. Ticagrelor enhances release of anti-hypoxic cardiac progenitor cell-derived exosomes through increasing cell proliferation in vitro. Sci Rep. (2020) 10:2494. doi: 10.1038/s41598-020-59225-7
226. Dougherty JA, Mergaye M, Kumar N, Chen CA, Angelos MG, Khan M. Potential role of exosomes in mending a broken heart: nanoshuttles propelling future clinical therapeutics forward. Stem Cells Int. (2017) 2017:5785436. doi: 10.1155/2017/5785436
227. Marote A, Teixeira FG, Mendes-Pinheiro B, Salgado AJ. MSCs-derived exosomes: cell-secreted nanovesicles with regenerative potential. Front Pharmacol. (2016) 7:231. doi: 10.3389/fphar.2016.00231
228. Fleury A, Martinez MC, Le Lay S. Extracellular vesicles as therapeutic tools in cardiovascular diseases. Front Immunol. (2014) 5:370. doi: 10.3389/fimmu.2014.00370
229. Dai J, Su Y, Zhong S, Cong L, Liu B, Yang J, et al. Exosomes: key players in cancer and potential therapeutic strategy. Signal Transduct Target Ther. (2020) 5:145. doi: 10.1038/s41392-020-00261-0
230. Shen X, Wang C, Zhu H, Wang Y, Wang X, Cheng X, et al. Exosome-mediated transfer of CD44 from high-metastatic ovarian cancer cells promotes migration and invasion of low-metastatic ovarian cancer cells. J Ovarian Res. (2021) 14:38. doi: 10.1186/s13048-021-00776-2
231. Santoso MR, Ikeda G, Tada Y, Jung JH, Vaskova E, Sierra RG, et al. Exosomes from induced pluripotent stem cell-derived cardiomyocytes promote autophagy for myocardial repair. J Am Heart Assoc. (2020) 9:e014345. doi: 10.1161/JAHA.119.014345
232. Yu H, Wang Z. Cardiomyocyte-derived exosomes: biological functions and potential therapeutic implications. Front Physiol. (2019) 10:1049. doi: 10.3389/fphys.2019.01049
233. Guo J, Jin D, Wu Y, Yang L, Du J, Gong K, et al. The miR 495-UBE2C-ABCG2/ERCC1 axis reverses cisplatin resistance by downregulating drug resistance genes in cisplatin-resistant non-small cell lung cancer cells. EBioMedicine. (2018) 35:204–21. doi: 10.1016/j.ebiom.2018.08.001
Keywords: exosomes, cardiovascular therapy, miRNA, stem cells, cell therapy
Citation: Jayaraman S, Gnanasampanthapandian D, Rajasingh J and Palaniyandi K (2021) Stem Cell-Derived Exosomes Potential Therapeutic Roles in Cardiovascular Diseases. Front. Cardiovasc. Med. 8:723236. doi: 10.3389/fcvm.2021.723236
Received: 10 June 2021; Accepted: 12 July 2021;
Published: 10 August 2021.
Edited by:
Vincenzo Lionetti, Sant'Anna School of Advanced Studies, ItalyReviewed by:
Raj Kishore, Temple University, United StatesJafar Rezaie, Urmia University of Medical Sciences, Iran
Copyright © 2021 Jayaraman, Gnanasampanthapandian, Rajasingh and Palaniyandi. This is an open-access article distributed under the terms of the Creative Commons Attribution License (CC BY). The use, distribution or reproduction in other forums is permitted, provided the original author(s) and the copyright owner(s) are credited and that the original publication in this journal is cited, in accordance with accepted academic practice. No use, distribution or reproduction is permitted which does not comply with these terms.
*Correspondence: Kanagaraj Palaniyandi, muthammal.kanagaraj@gmail.com; Dhanavathy Gnanasampanthapandian, dhanavathy.2006@gmail.com