- 1Division of Cardiology, Department of Internal Medicine II, Vienna General Hospital, Medical University of Vienna, Vienna, Austria
- 2Department of Dermatology, Landesklinikum Wiener Neustadt, Wiener Neustadt, Austria
- 3Department of Internal Medicine I, Cardiology, Klinikum Bamberg, Bamberg, Germany
- 4Department of Emergency Medicine, Vienna General Hospital, Medical University of Vienna, Vienna, Austria
- 5Department of Internal Medicine, Cardiology and Nephrology, Landesklinikum Wiener Neustadt, Wiener Neustadt, Austria
Background and Rationale: Mild therapeutic hypothermia (MTH) is a concept to reduce infarct size and improve outcome after ST-segment elevation myocardial infarction (STEMI). In the STATIM trial, we investigated MTH as an additional therapy for STEMI patients. In the intention-to-treat set, 101 patients were included. No difference in primary and secondary endpoints measured by cardiac magnetic resonance imaging was found. Platelet activation and plasmatic coagulation are key in the pathophysiology of STEMI. In the present study, we investigated the effect of MTH on primary and secondary hemostasis in STEMI patients.
Methods and Results: Platelet function and morphology were assessed by routine blood count, aggregometry testing, and flow cytometry. Soluble platelet markers were determined by enzyme-linked immunosorbent assay (ELISA) testing. Plasmatic coagulation was measured throughout the study. Platelet count remained unchanged, irrespective of treatment, whereas platelet size decreased in both patient groups. Platelet aggregometry indicated increased platelet reactivity in the MTH group. Furthermore, higher adenosine diphosphate (ADP) plasma levels were found in MTH patients. Expression of glycoprotein IIb/IIIa was increased on platelets of STEMI patients treated with MTH. Lower patient temperatures correlated with longer clotting times and resulted in reduced pH. Lower pH values were positively correlated with longer clotting times.
Conclusion: Present data indicate longer clotting times and higher platelet reactivity in STEMI patients treated with MTH. These changes did not correspond to clinical bleeding events or larger infarct size.
Introduction
Acute coronary syndrome (ACS) is amongst the leading causes of death worldwide (1). Infarct size is one of the best predictors of long-term outcome in ST-elevation myocardial infarction (STEMI) patients (2). While hyperthermia is associated with an unfavorable outcome (3), mild therapeutic hypothermia (MTH) has been studied in STEMI patients as adjunct to primary percutaneous coronary intervention (pPCI) to reduce final infarct size. Several studies tested MTH in various other clinical settings such as stroke (4), neonatal hypoxic encephalopathy (5) and myocardial infarction (6–9). In clinical routine, MTH is primarily used in patients suffering from cardiac arrest (10). However, a large trial did not indicate a mortality benefit for MTH in cardiac arrest patients (11). A meta-analysis including six randomized controlled trials showed a significant reduction of infarct size in myocardial infarction patients with anterior wall involvement by MTH. However, no effect on mortality or infarct size in the overall patient cohort could be observed (12). In the STATIM trial, 120 patients were included, of whom 101 remained in the final analysis. We did not observe any difference in primary and secondary endpoints by cardiac magnetic resonance (CMR) imaging 2 ± 4 and 195 ± 15 days after pPCI (13). Platelet activation, atherothrombosis and fibrin deposition are key mechanisms in STEMI, not only causing coronary artery occlusion but also microvascular obstruction, which leads to extended myocardial damage and unfavorable outcome (14). The impact of MTH on human platelet function and coagulation is limited. Differing results were reported on the effect of MTH on coagulation parameters in cardiac arrest patients (15, 16), and platelet reactivity was not changed by MTH in patients with cardiogenic shock (17). In the present prospective randomized controlled trial, we investigated the effect of MTH on primary and secondary hemostasis in STEMI patients.
Materials and Methods
Patients and Study Design
The present study is a substudy of the randomized, controlled, open-label, end-point blinded STATIM trial (13). One hundred twenty STEMI patients were allocated to MTH or normothermia. Randomization was conducted by an emergency physician in an out-of-hospital setting. Study patients were assigned 1:1 to target temperature management or normothermia. One hundred one patients remained in the intention-to-treat data set (54 normothermia group, 47 MTH group). Inclusion criteria were: (1) Age between 18 and 75, (2) at least 30 min of continuous typical chest pain, (3) anterior or inferior STEMI with ST-segment elevation of >0.2 mV in two contiguous leads, (4) duration of symptoms <6 h prior to first medical contact (FMC), and (5) immediate access to the catheterization laboratory. Exclusion criteria were: Cardiac arrest, a tympanic temperature below 35°C at presentation, history of myocardial infarction or PCI/coronary artery bypass grafting, chronic (New York Heart Association II–IV) or acute heart failure (Killip class II–IV), thrombolysis, infection, end-stage kidney disease, hepatic failure, recent stroke, hematological dyscrasias, oral anticoagulant treatment, severe pulmonary disease, known allergy to medications used in the trial, and being female of childbearing age (13). The MTH group received MTH treatment by surface cooling (EMCOOLS Flex.Pad®, EMCOOLS Emergency Medical Cooling Systems AG) and administration of ice cold saline (10 ml/kg for anterior STEMI; 20 ml/kg for inferior STEMI) at FMC. After arrival in the catheterization laboratory, an endovascular cooling catheter (Accutrol 14 Fr catheter and InnerCool RTx endovascular console, ZOLL Medical Corporation) was placed. Target temperature was set to 34 °C and maintained for 60 min after reperfusion. For shivering control, buspirone and meperidine were administered. Bleeding events were monitored for the first 45 days after study inclusion and classified according to the TIMI bleeding criteria (18).
Routine Blood Tests
Blood draws were obtained at predetermined time points (FMC, pPCI, and 72 h after pPCI). Arterial blood was collected for blood gas analysis during pPCI. Patients did not receive any emergency medication prior to the FMC blood draws. Blood was immediately anticoagulated with EDTA (ethylenediaminetetraacetic acid), citrate or heparin, and transferred to the Clinical Department of Laboratory Medicine (General Hospital Vienna). Dilution effects were considered by normalizing consecutive blood draws to hematocrit from FMC. Extrinsic and common pathway of blood coagulation was tested by measuring prothrombin time (prothrombin time, given as percentage). Partial thromboplastin time (given as seconds) was used for testing the intrinsic and common pathway. All pH values shown in this study were measured at time of reperfusion.
Platelet Aggregometry
Platelet function was measured by aggregometry (Multiplate® Analyzer, Roche Diagnostics). Heparin anticoagulated blood was obtained for platelet aggregation measurements at FMC and at pPCI. For stimulation, arachidonic acid (ASPI-test), adenosine diphosphate (ADP, ADP-test) or thrombin receptor activating peptide-6 (TRAP-6, TRAP-test) were used, and diluted according to manufacturer's protocol (ASPI-test, ADP-test, TRAP-test; Roche Diagnostics).
Flow Cytometry
Blood samples were immediately transferred to the laboratory facilities and incubated with the following fluorochrome-labeled antibodies at room temperature for 30 min: PAC-1 (glycoprotein IIb/IIIa) (BD Biosciences Pharmingen), CD45, CD14, CD16, glycoprotein Ib (CD42b), P-selectin (CD62p), PECAM-1 (CD31) and integrin alpha-V (CD51) (Biolegend). Erythrocytes were lysed by addition of BD FACS lysing solution (Becton Dickinson). Samples were analyzed using a 6-color BD Canto II (Becton Dickinson). Forward and side scatter were used to divide cell debris/platelets from viable cells. Platelets were identified by forward and sideward scatter as well as glycoprotein Ib (CD42b) positivity. Monocyte-platelet-aggregates (MPA) were defined by co-aggregation of monocytes and platelets. CD45 and CD14 were used for monocyte identification as previously described (19).
Plasma Measurements
ADP plasma levels were evaluated by an ADP Assay Kit (Abcam). sP-Selectin (soluble P-selectin) was determined utilizing a Human sP-selectin Platinum ELISA (Thermo Scientific). For the quantification of thrombospondin (TSP-1) a Human Thrombospondin-1 Quantikine ELISA Kit (R&D Systems) was used. Soluble CD40L concentration was determined using a Human sCD40L (soluble CD40 ligand) Platinum ELISA Kit (Thermo Scientific). All assays were performed according to the manufacturers' protocol. All plates were measured by a VersaMax microplate reader (Molecular Devices). ADP Assay kit plates were read on a Varioskan Flash microplate reader (Thermo Scientific).
Cardiac Magnetic Resonance Imaging
A 1.5 T system cardiac magnetic resonance (CMR) (Avanto Fit, Siemens Medical Systems) was performed for each patient 4 ± 2 days after pPCI to measure left ventricular function, infarct size, microvascular obstruction and myocardial salvage index. A second CMR was performed 195 ± 15 days after PCI. The detailed protocol is described elsewhere (13, 20).
Statistics
Results are presented as mean ± standard deviation (SD) or median [interquartile range, IQR]. For normally distributed data, paired Students t-test was applied; otherwise, Wilcoxon signed rank test was used. The comparison between two groups was performed by a two-tailed unpaired t-test for normally distributed data, otherwise Mann-Whitney-U-test was employed. We used Δ values to compare value change over time between normothermia and MTH groups. Δ values of tested parameters were calculated by subtracting FMC values from pPCI values, followed by a two-tailed unpaired t-test or Mann-Whitney-U-test. Kolmogorov-Smirnov test and visual assessment of histograms were applied to test for normal distribution. An ANOVA with repeated measures was used to compare more than two groups. For multiple testing, Bonferroni-Holm correction was employed. Pearson correlation (r) was used for normally distributed data, otherwise data were calculated by Spearman rank correlation (rs). Chi-square test for independence was used to calculate a relationship between two categorical variables. A p < 0.05 was considered statistically significant. Statistical analyses were performed using IBM SPSS Statistics 26.0 (IBM). Figures were generated using GraphPad Prism 9.0 (GraphPad).
Results
Patient Characteristics and Outcome Parameters
In the STATIM trial, 120 patients were recruited between 2013 and 2016. 19 study patients were excluded after randomization. Causes for exclusion were: STEMI criteria not fulfilled (8 patients), Killip Class >I (1 patient), no availability of the cath lab (1 patient), history of myocardial infarction (2 patients), symptom onset >6 h (2 patients) and not suitable for CMR (3 patients). The intention-to-treat set consisted of 101 patients, of whom 47 patients were assigned to MTH treatment. A detailed flow chart of the STATIM trial is published in (13). Previous medication of patients including angiotensin converting enzyme inhibitor (ACE-I), acetylsalicylic acid (ASA), angiotensin receptor blocker (ARB), and statin intake is listed in Table 1. Statin treatment was significantly more prevalent in patients of the normothermia group (normothermia: n = 12, MTH: n = 3; p < 0.05; Table 1). Regular intake of any other medication including antidiabetic drugs (12 patients), vitamin D supplements (5 patients), non-steroidal anti-inflammatory drugs (NSAID) without ASA (4 patients), antidepressant drugs (6 patients), proton pump inhibitor (9 patients), amiodarone (1 patient), thyroid replacement medication (2 patients), alpha blockers (4 patients) was overall very low and showed no group differences. There was no correlation between any pre-existing medication of patients and tested parameters in this study (data not shown). All patients received acetylsalicylic acid (ASA), heparin and prasugrel or ticagrelor by the emergency physician prior to pPCI. Patients in the MTH group had a significantly lower temperature at reperfusion (normothermia: 36.3 ± 0.6°C, MTH: 34.4 ± 0.6°C; p < 0.0001; Table 1). There was no difference in the number of bleeding events. Detailed patient characteristics are displayed in Table 1. Measurements of routine laboratory testing did not differ between both groups at the time of reperfusion as illustrated in Table 2. An extended list of laboratory parameters is published elsewhere (13).
Plasmatic Coagulation, Platelet Count, and Mean Platelet Volume
Patients in the MTH group displayed lower prothrombin time measured at pPCI, indicating a longer clotting time under MTH (normothermia: 88.42 ± 18.42%, MTH: 77.83 ± 15.15%; p < 0.01; Figure 1A). No significant differences were detected regarding partial thromboplastin time (normothermia: 121.86 ± 46.61 s, MTH: 132.98 ± 43.46 s; not significant; Figure 1B).
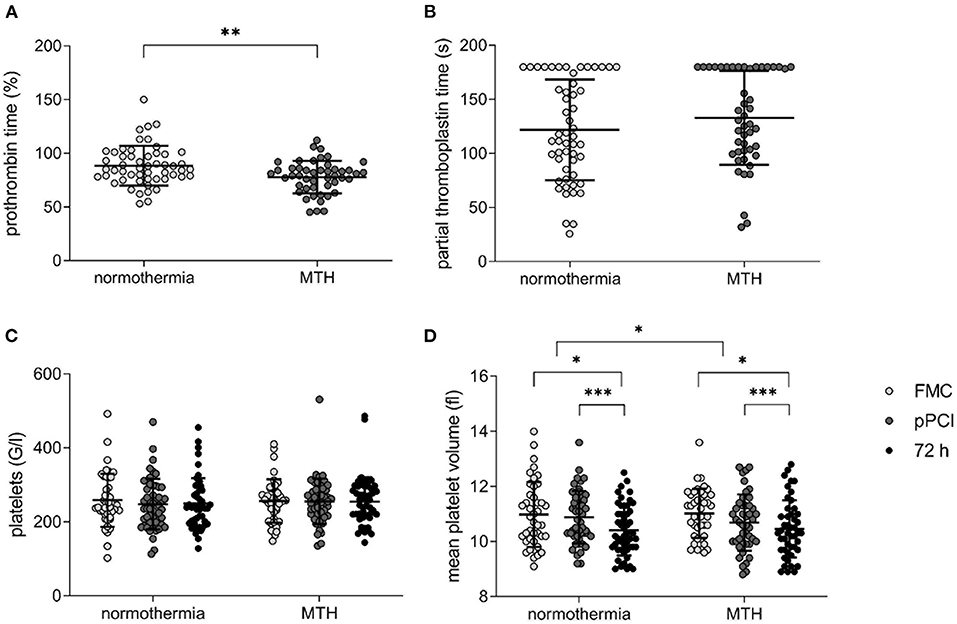
Figure 1. Platelet count, mean platelet volume and bleeding times in normothermia and MTH patients. (A) prothrombin time in percent (normothermia n = 53, MTH n = 47) and (B) partial thromboplastin time in seconds (normothermia n = 53, MTH n = 47) measured at pPCI. (C) platelet count in G/l (equals 1,000/ml; normothermia n = 51, MTH n = 45) and (D) MPV in fl (normothermia n = 51, MTH n = 45) from FMC until 72 h after pPCI. For (A), unpaired t-test was used. For (B), Mann-Whitney-U-test was used. For (C,D), ANOVA with repeated measures was used. First medical contact (FMC), mild therapeutic hypothermia (MTH), mean platelet volume (MPV), primary percutaneous coronary intervention (pPCI). *p < 0.05, **p < 0.01, ***p < 0.001.
Platelet count was not different between the groups and remained unchanged over time from FMC until 72 h after pPCI (Figure 1C). Mean platelet volume declined in both groups from FMC to 72 h after pPCI. This decline in volume was greater in the MTH group (Δ normothermia: −0.12 ± 1.5 fl, Δ MTH: −0.28 ± 1.53 fl; p < 0.05; Figure 1D).
Platelet Reactivity of MTH Patients Is Increased ex vivo Compared to Normothermia
Inhibition of (1) cyclooxygenase 1 (ASPI-test), (2) ADP receptor P2Y12 (ADP-test) and (3) and thrombin receptor (TRAP-test) were determined in platelets from FMC and pPCI blood samples. While cyclooxygenase 1 was significantly inhibited between FMC and pPCI in the normothermia STEMI cohort (FMC 59.89 ± 37.66 U vs. pPCI 47.39 ± 27.69 U; p < 0.05; Figure 2A), no inhibition was observed in the MTH cohort. TRAP-induced platelet reactivity increased significantly from FMC to pPCI in the MTH group (FMC 106.89 ± 32.67 U vs. pPCI 133.58 ± 27.18 U; p < 0.0001; Figure 2C), but remained unchanged in the normothermia group, resulting in a significant difference between the groups (Δ normothermia: 6.5 ± 37.21 U, Δ MTH: 26.68 ± 32.31 U; p < 0.05; Figure 2C). ADP-mediated platelet activation behaved in the same way (decrease in the normothermia group and increase in the MTH group at FMC and pPCI) but differences were not significant (Figure 2B).
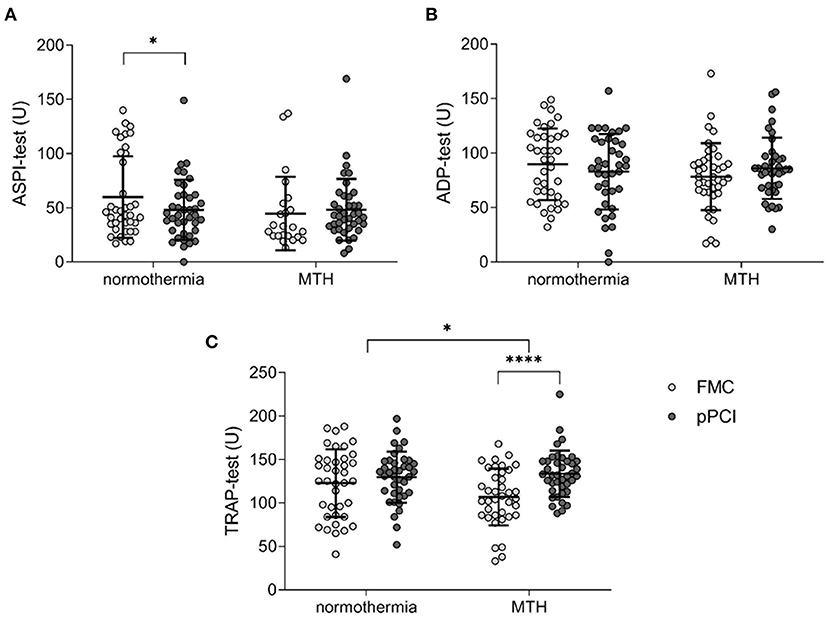
Figure 2. Platelet aggregometry. (A) ASPI-test (normothermia n = 38, MTH n = 35), (B) ADP-test (normothermia n = 38, MTH n = 35), and (C) TRAP-test (normothermia n = 38, MTH n = 34) were performed with whole blood obtained at first medical contact and pPCI. Results are illustrated as area under the curve units. For (A), Wilcoxon signed rank test was used. For (B,C), paired and unpaired t-tests were used. First medical contact (FMC), mild therapeutic hypothermia (MTH), primary percutaneous coronary intervention (pPCI), units (U). *p < 0.05, ****p < 0.0001.
ADP Levels Are Increased in STEMI Patients Treated With MTH
We measured ADP levels, sP-selectin, TSP-1 and sCD40L at FMC and pPCI by ELISA. Normothermia patients displayed decreased levels of ADP at pPCI compared to FMC (FMC 0.94 ± 0.56 nmol/μl vs. pPCI 0.78 ± 0.43 nmol/μl; p < 0.05; Figure 3A), while MTH patients remained unchanged, with a significant intergroup delta difference in ADP levels from FMC to pPCI (Δ normothermia: −0.16 ± 0.46 nmol/μl, Δ MTH: −0.01 ± 0.4 nmol/μl; p < 0.05; Figure 3A). Soluble P-selectin, TSP-1, sCD40L did not differ between patients treated with MTH and normothermia patients (Figures 3B–D). However, TSP-1 (normothermia: FMC 4,450.99 ± 4,466.21 ng/ml vs. pPCI 2,343.75 ± 1,554.46 ng/ml; p < 0.01; MTH: FMC 5,185.68 ± 4,154.88 ng/ml vs. pPCI 3,701.96 ± 5,208.03 ng/ml; p < 0.05; Figure 3C) and sCD40L (normothermia: FMC 3.97 ± 2.34 ng/ml vs. pPCI 2.79 ± 2.25 ng/ml; p < 0.01; MTH: FMC 3.17 ± 1.95 ng/ml vs. pPCI 2.24 ± 2.25 ng/ml; p < 0.01; Figure 3D) plasma levels decreased in both groups from FMC to pPCI. It has been shown that angiotensin receptor blockade and statins reduced sCD40L in patients (21) and p-selectin levels in animals (22). We did not detect any differences in soluble platetet activation marker levels between patients with and without such preexisting medication (data not shown).
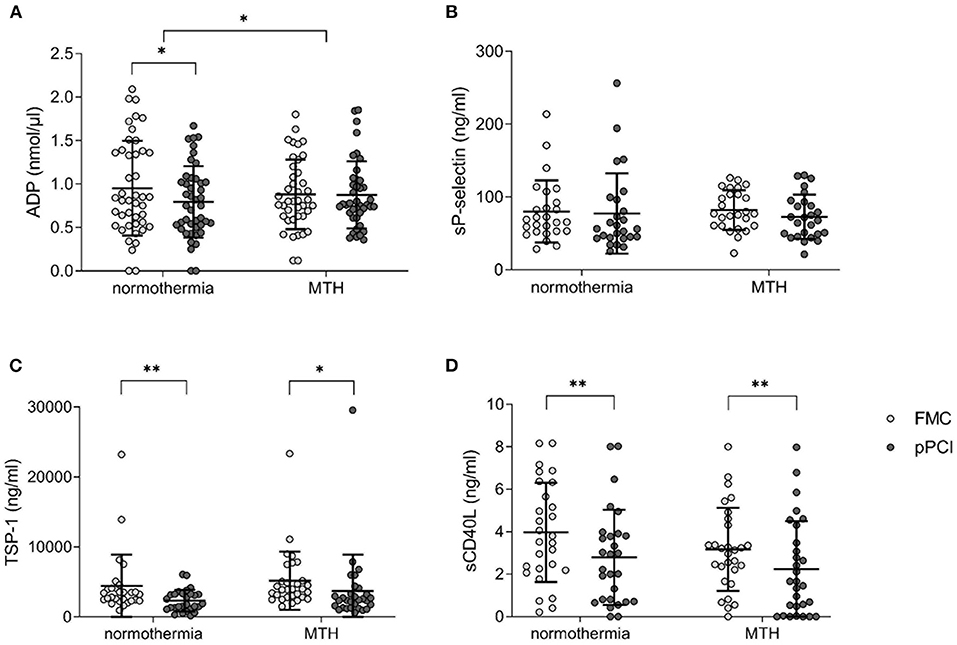
Figure 3. Soluble platelet activation markers. (A) ADP concentration given in nmol/μl (normothermia n = 44, MTH n = 41). (B) sP-selectin (normothermia n = 26, MTH n = 26), (C) TSP-1 (normothermia n = 28, MTH n = 30), and (D) sCD40L (normothermia n = 28, MTH n = 29) concentrations are presented as ng/ml from FMC and pPCI plasma. For (A), paired and unpaired t-tests were used. For (B–D), Wilcoxon signed rank tests were used. Adenosine diphosphate (ADP), first medical contact (FMC), mild therapeutic hypothermia (MTH), primary percutaneous coronary intervention (pPCI), soluble CD40 ligand (sCD40L), soluble P-selectin (sP-selectin), thrombospondin-1 (TSP-1). *p < 0.05, **p < 0.01.
MTH Influences PAC-1 and P-Selectin Platelet Receptor Expression
Platelet expression of PAC-1 and P-selectin at FMC and at pPCI were assessed by flow cytometry. PAC-1 expression decreased from FMC to pPCI in normothermia patients while it increased in MTH patients (Δ normothermia: −81.65 ± 381.25 MFI (mean fluorescence intensity), Δ MTH: 57.56 ± 471.99 MFI; p < 0.05; Figure 4A).
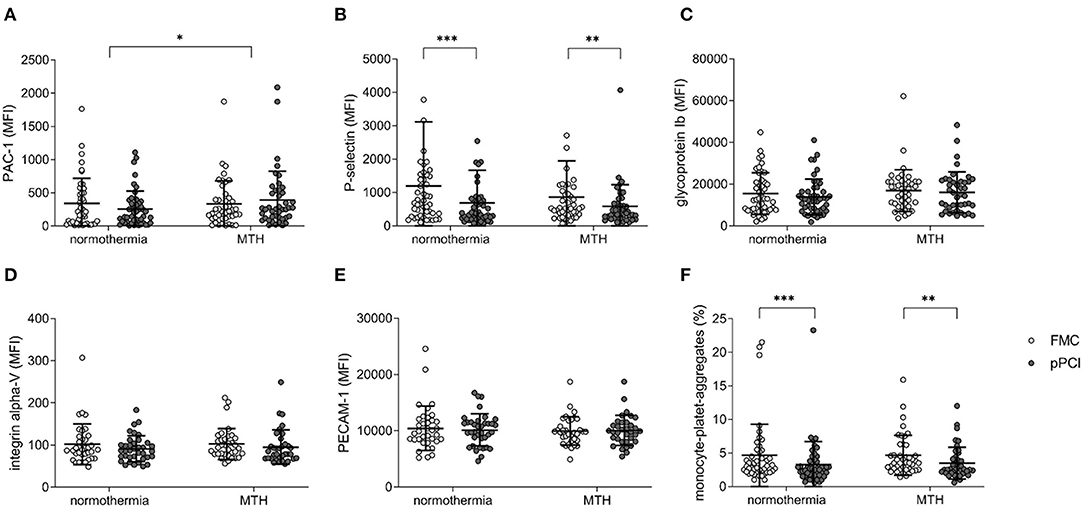
Figure 4. Platelet receptor expression. (A) Glycoprotein IIb/IIIa (PAC-1; normothermia n = 46, MTH n = 43), (B) P-selectin (CD62p; normothermia n = 46, MTH n = 43), (C) Glycoprotein Ib (CD42b; normothermia n = 46, MTH n = 43), (D) Integrin α-V (CD51; normothermia n = 36, MTH n = 35), (E) PECAM-1 (CD31; normothermia n = 36, MTH n = 34) expression on platelets at FMC and pPCI comparing normothermia and MTH patients. Data are expressed as MFI. (F) MPA (normothermia n = 40, MTH n = 42) in the normothermia and MTH group in percentage of total platelet counts. For (A–F), Wilcoxon signed rank tests and Mann-Whitney-U-tests were used. First medical contact (FMC), mean fluorescence intensity (MFI), monocyte-platelet-aggregates (MPA) mild therapeutic hypothermia (MTH), procaspase activating compound-1 (PAC-1), platelet endothelial cell adhesion molecule-1 (PECAM-1), primary percutaneous coronary intervention (pPCI). *p < 0.05, **p < 0.01, ***p < 0.001.
No group difference was detected for P-selectin (Figure 4B), glycoprotein Ib (Figure 4C), integrin alpha-V (Figure 4D), PECAM-1 (Figure 4E), and MPA (Figure 4F). P-selectin expression was decreased in both groups at FMC and pPCI (normothermia: FMC 1,192.59 ± 1,924.01 MFI vs. pPCI 696.17 ± 989.27 MFI; p < 0.001; MTH: FMC 858.60 ± 1,092.52 MFI vs. pPCI 586.58 ± 647.66 MFI; p < 0.01; Figure 4B). In both groups, MPA decreased from FMC to pPCI (normothermia: FMC 4.65 ± 4.66 % vs. pPCI 3.25 ± 3.49 %; p < 0.001; MTH: FMC 4.66 ± 2.99 % vs. pPCI 3.50 ± 2.38 %; p < 0.01; Figure 4F).
MTH Is Associated With a Lower pH Value and Longer Clotting Times
Body temperature at reperfusion correlated positively with prothrombin time (n = 97; r = 0.35; p < 0.001; Figure 5A). The same trend was observed for partial thromboplastin time, but was not significant (n = 97; rs = −0.20; not significant; Figure 5B).
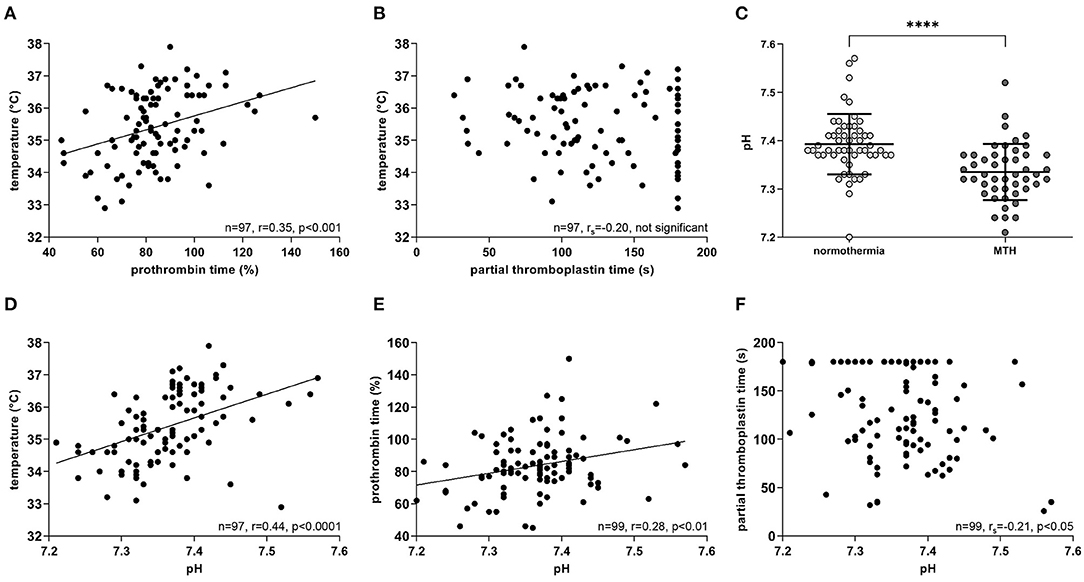
Figure 5. Correlations of temperature, bleeding time and pH. All measurements were at the time of reperfusion during pPCI. (A) Temperature correlated positively with prothrombin time (n = 97), but not with, (B) partial thromboplastin time (n = 97). (C) MTH patients had significantly lower pH values compared to the normothermia group (normothermia n = 53, MTH n = 47). (D) pH correlated positively with temperature (n = 97) and (E) prothrombin time (n=99), whereas negatively with, (F) partial thromboplastin time (n = 99). For (A,D,E), Pearson correlation was used. For (B,F) Spearman rank correlation was used. For (C), Mann-Whitney-U-test was used. Celsius (°C), mild therapeutic hypothermia (MTH), primary percutaneous coronary intervention (pPCI), seconds (s). ****p < 0.0001.
Blood pH measured at pPCI was significantly lower in patients treated with MTH than in normothermia patients (normothermia: 7.39 ± 0.06 mmol/l, MTH: 7.33 ± 0.06 mmol/l; p < 0.0001; Figure 5C). Lower blood pH correlated significantly with lower patient temperatures (n = 97; r = 0.44; p < 0.0001; Figure 5D). Furthermore, pH correlated positively with prothrombin time (n = 99; r = 0.28; p < 0.01; Figure 5E) and on the contrary, negatively with partial thromboplastin time (n = 99; rs = −0.21; p < 0.05; Figure 5F).
Discussion
In the present study, we sought to investigate platelet function and plasma coagulation of STEMI patients in response to MTH in a controlled, prospective trial. We are demonstrating opposing effects of MTH on primary and secondary hemostasis—higher platelet reactivity and longer clotting times in cooled patients. The observation of attenuated platelet inhibition by dual antiplatelet therapy under conditions of MTH is important because effective platelet inhibition is an essential treatment step prior to implantation of stents.
MTH was hoped to reduce infarct size in STEMI. Up to now, none of the published trials except for a small feasibility trial (8) could prove a significant reduction of infarct size (6, 13). Patients presenting early after symptom onset with a large myocardium at risk who reach a target temperature below 35°C early may benefit most from MTH (12). Therefore, MTH was initiated at FMC in the STATIM trial, and 80% of the patients reached the target temperature at reperfusion. However, no difference in CMR-measured outcome variables could be observed (13).
The effect of MTH on hemostasis and the occurrence of hemorrhage is controversial. Several studies suggested a prolonged clot initiation during hypothermia in cardiac arrest patients (16, 23). Two meta-analyses did not detect a higher risk for bleeding due to MTH, neither in cardiac arrest (24), nor in STEMI (12). In the STATIM trial, we also did not observe more bleedings in patients treated with MTH compared to normothermia patients.
Hemostasis is a complex process, involving platelets (primary hemostasis) and fibrin aggregation (secondary hemostasis). In the STATIM trial, secondary hemostasis was evaluated by prothrombin time and partial thromboplastin time. We observed a significantly longer prothrombin time in the MTH group compared to normothermia patients (Figure 1A). Partial thromboplastin time was prolonged in both groups at pPCI due to heparinization, but was not significantly longer in patients who underwent MTH (Figure 1B). Other studies did not observe significant changes in coagulation during MTH (15, 25).
Platelets play a crucial role in the coagulation system and thrombus formation. Growing evidence indicates increased platelet activity under MTH (26, 27). Cooling of platelets in vitro below 15°C resulted in a volume increase and formation of pseudopods (28). Lower body temperature of 32–33°C lead to a decrease in platelet count, possibly caused by hepatic and splenic sequestration (16, 29). Platelet counts of patients in the STATIM trial remained unchanged (Figure 1C). Shape change and granule release are indirectly monitored by measuring MPV. We detected smaller platelets from FMC onwards to pPCI and 72 h in both groups. The drop in platelet size was more pronounced in patients of the MTH group (Figure 1D). In vitro, increased platelet aggregation between 30 and 34°C was shown (26). In ex vivo platelet aggregometry, we found that platelet reactivity decreased from FMC to pPCI in the normothermia group, while it remained unchanged in the MTH group (Figure 2).
Furthermore, we measured the expression of platelet receptors at FMC and pPCI. PAC-1 (glycoprotein IIb/IIIa complex) is a crucial receptor for platelet aggregation. While PAC-1 expression decreased in normothermia patients until pPCI, it increased in MTH patients (Figure 4A). Consistently, increased platelet PAC-1 expression was observed in an in vitro study under hypothermia (30). P-selectin and MPA decreased significantly from FMC to pPCI in both treatment groups (Figures 4B,F). ADP reactivity in aggregometry correlated with platelet PAC-1 and P-selectin receptor expression (Supplementary Figure 1).
STEMI patients receive potent platelet-inhibitory medication early on. In the STATIM trial, acetylsalicylic acid and ADP inhibitors were administered at FMC. Therefore, reduced platelet reactivity is expected. However, it is known that effective platelet receptor inhibition is significantly delayed in STEMI after loading with dual anti-platelet medication (31, 32).
We observed mild reduction of platelet reactivity by aggregometry (Figure 2A) and decreasing PAC-1 expression in the normothermia STEMI patient cohort (Figure 4A). In contrast, MTH partially abrogated platelet inhibition (Figure 2C). This might be one adverse effect of MTH treatment which could explain its failure to improve functional outcome after STEMI. However, no stent thrombosis were observed in the STATIM trial.
ADP was identified as key mediator of hypothermia-induced platelet activation. In vitro experiments with hypothermia showed reduced ADP hydrolysis via decreased E-NTPDase1 activity, which resulted in higher plasmatic ADP levels and platelet activation (33). This is supported by our in vivo data because we observed significantly higher ADP plasma levels in the MTH than in the normothermia cohort (Figure 3A).
Our data demonstrate a significant correlation of lower temperature and lower prothrombin time (Figure 5A). Moreover, lower patient temperatures at reperfusion are associated with lower pH values (Figure 5D). Longer prothrombin time and partial thromboplastin time correlated significantly with lower pH (Figures 5E,F). Additionally, patients in the hypothermia group had significantly lower blood pH (Figure 5C). The decline of pH under hypothermia is considered to be a result of hyperlactataemia (34, 35), which is caused by increased fat metabolism (36). In an in vitro study with whole blood, acidosis impaired clotting times primarily under hypothermia, as it has a substantial effect on thrombin-generating kinetics (37). Our data from STEMI patients are in agreement with these data. We propose that MTH and subsequent changes in pH might impair secondary hemostasis in STEMI patients. However, further studies to clarify the detailed mechanism are warranted.
We did not observe an effect of our findings on hemostasis and bleeding events, which were overall low (Table 1).
In the STATIM trial, CMR was performed 2 ± 4 days after pPCI to measure functional outcome and infarct size (13). None of the platelet activation and plasmatic coagulation measurements correlated with CMR-measured variables.
In conclusion, we found that platelet reactivity was increased in patients treated with MTH, whereby non-degraded ADP in plasma might be an important mediator of platelet activation under hypothermia. Concurrently, secondary hemostasis was slightly impaired under MTH.
Limitations
Temperature changes, while transporting or preparing patient samples could have alleviated group differences. Yet, all samples were processed immediately and no additional cooling or heating steps were applied. Only patients treated with MTH received ice-cold saline, buspirone, and meperidine, which might have an impact on the observed effects. All data from this prospective human STEMI study are observational and do not suffice to draw conclusions on the mechanism of MTH-induced changes in primary and secondary hemostasis.
Data Availability Statement
The original contributions presented in the study are included in the article/Supplementary Material, further inquiries can be directed to the corresponding author/s.
Ethics Statement
The studies involving human participants were reviewed and approved by Ethics Committee of the Medical University of Vienna, Austria (approval number: 1497/2012). The patients/participants provided their written informed consent to participate in this study.
Author Contributions
TS, FS, CT, IL, and AM designed the study and developed the study concept. FS and CT were responsible for patient inclusion in the clinical trial. TS, TH, DS, CT, AM, and IL executed the acquisition of samples. TS, TH, AO and DS were involved in the laboratory processing and methods. TS, TH, AO and AM processed and analyzed the data. TS wrote the original draft and designed all figures and tables. IL and AM reviewed and edited the manuscript. IL and AM supervised TS in the execution of the study and were responsible for acquisition of the funding. All authors contributed to the article and approved the submitted version.
Funding
This study was supported by the FWF Austrian Science Fund (KLI209) and by the Vienna Major Fund (15214).
Conflict of Interest
The authors declare that the research was conducted in the absence of any commercial or financial relationships that could be construed as a potential conflict of interest.
Acknowledgments
We want to thank the staff of the Emergency Department and the Cardiology heart catherization laboratory for the great effort. We especially thank Gerhard Ruzicka, study coordinator of the STATIM trial, for his incredible dedication.
Supplementary Material
The Supplementary Material for this article can be found online at: https://www.frontiersin.org/articles/10.3389/fcvm.2021.707367/full#supplementary-material
Abbreviations
ACE-I, Angiotensin converting enzyme inhibitor; ACS, Acute coronary syndrome; ADP, Adenosine diphosphate; ARB, Angiotensin receptor blocker; ASA, Acetylsalicylic acid; BMI, Body mass index; CMR, Cardiac magnetic resonance; EDTA, Ethylenediaminetetraacetic acid; ELISA, Enzyme-linked immunosorbent assay; FMC, First medical contact; MFI, Mean fluorescence intensity; MPA, Monocyte-platelet-aggregates; MTH, Mild therapeutic hypothermia; NSAID, Non-steroidal anti-inflammatory drugs; PAC-1, Procaspase activating compound-1; PECAM-1, Platelet endothelial cell adhesion molecule-1; pPCI, Primary percutaneous coronary intervention; sCD40L, Soluble CD40 ligand; sP-selectin, Soluble P-selectin; SD, Standard deviation; STEMI, ST-segment elevation myocardial infarction; TIMI, Thrombolysis in myocardial infarction grade flow; TRAP, Thrombin receptor activating peptide-6; TSP-1, Thrombospondin 1.
References
1. Vedanthan R, Seligman B, Fuster V. Global perspective on acute coronary syndrome: a burden on the young and poor. Circ Res. (2014) 114:1959–75. doi: 10.1161/CIRCRESAHA.114.302782
2. Lonborg J, Vejlstrup N, Kelbæk H, Holmvang L, Jorgensen E, Helqvist S, et al. Final infarct size measured by cardiovascular magnetic resonance in patients with ST elevation myocardial infarction predicts long-term clinical outcome: an observational study. Eur Heart J Cardiovasc Imaging. (2013) 14:387–95. doi: 10.1093/ehjci/jes271
3. Zeiner A, Holzer M, Sterz F, Schörkhuber W, Eisenburger P, Havel C, et al. Hyperthermia after cardiac arrest is associated with an unfavorable neurologic outcome. Arch Intern Med. (2001) 161:2007–12. doi: 10.1001/archinte.161.16.2007
4. Martin-Schild S, Hallevi H, Shaltoni H, Barreto AD, Gonzales NR, Aronowski J, et al. Combined neuroprotective modalities coupled with thrombolysis in acute ischemic stroke: a pilot study of caffeinol and mild hypothermia. J Stroke Cerebrovasc Dis. (2009) 18:86–96. doi: 10.1016/j.jstrokecerebrovasdis.2008.09.015
5. Jacobs SE, Berg M, Hunt R, Tarnow-Mordi WO, Inder TE, Davis PG. Cooling for newborns with hypoxic ischaemic encephalopathy. Cochrane Database Syst Rev. (2013) 2013:CD003311. doi: 10.1002/14651858.CD003311.pub3
6. Erlinge D, Götberg M, Lang I, Holzer M, Noc M, Clemmensen P, et al. Rapid endovascular catheter core cooling combined with cold saline as an adjunct to percutaneous coronary intervention for the treatment of acute myocardial infarction. J Am Coll Cardiol. (2014) 63:1857–65. doi: 10.1016/j.jacc.2013.12.027
7. Noc M, Erlinge D, Neskovic A, Kafedzic S, Merkely B, Zima E, et al. COOL AMI EU pilot trial: a multicentre, prospective, randomised controlled trial to assess cooling as an adjunctive therapy to percutaneous intervention in patients with acute myocardial infarction. EuroIntervention. (2017) 13:e531–9. doi: 10.4244/EIJ-D-17-00279
8. Götberg M, Olivecrona GK, Koul S, Carlsson M, Engblom H, Ugander M, et al. A pilot study of rapid cooling by cold saline and endovascular cooling before reperfusion in patients with ST-elevation myocardial infarction. Circ Cardiovasc Interv. (2010) 3:400–7. doi: 10.1161/CIRCINTERVENTIONS.110.957902
9. Hale SL, Kloner RA. Mild hypothermia as a cardioprotective approach for acute myocardial infarction: laboratory to clinical application. J Cardiovasc Pharmacol Ther. (2011) 16:131–9. doi: 10.1177/1074248410387280
10. Callaway CW, Donnino MW, Fink EL, Geocadin RG, Golan E, Kern KB, et al. Part 8: post–cardiac arrest care. Circulation. (2015) 132:465–82. doi: 10.1161/CIR.0000000000000262
11. Nielsen N, Wetterslev J, Cronberg T, Erlinge D, Gasche Y, Hassager C, et al. Targeted temperature management at 33degreeC versus 36degreeC after cardiac arrest. NEJM. (2013) 369:2197–206. doi: 10.1056/NEJMoa1310519
12. Villablanca PA, Rao G, Briceno DF, Lombardo M, Ramakrishna H, Bortnick A, et al. Therapeutic hypothermia in ST elevation myocardial infarction: a systematic review and meta-analysis of randomised control trials. Heart. (2016) 102:712–9. doi: 10.1136/heartjnl-2015-308559
13. Testori C, Beitzke D, Mangold A, Sterz F, Loewe C, Weiser C, et al. Out-of-hospital initiation of hypothermia in ST-segment elevation myocardial infarction: a randomised trial. Heart. (2018) 105:531–7. doi: 10.1136/heartjnl-2018-313705
14. Symons R, Pontone G, Schwitter J, Francone M, Iglesias JF, Barison A, et al. Long-Term incremental prognostic value of cardiovascular magnetic resonance after ST-segment elevation myocardial infarction. JACC Cardiovasc Imaging. (2018) 11:813–25. doi: 10.1016/j.jcmg.2017.05.023
15. Nielsen AKW, Jeppesen AN, Kirkegaard H, Hvas A-M. Changes in coagulation during therapeutic hypothermia in cardiac arrest patients. Resuscitation. (2016) 98:85–90. doi: 10.1016/j.resuscitation.2015.11.007
16. Spiel AO, Kliegel A, Janata A, Uray T, Mayr FB, Laggner AN, et al. Hemostasis in cardiac arrest patients treated with mild hypothermia initiated by cold fluids. Resuscitation. (2009) 80:762–5. doi: 10.1016/j.resuscitation.2009.03.026
17. Orban M, Mayer K, Morath T, Bernlochner I, Hadamitzky M, Braun S, et al. The impact of therapeutic hypothermia on on-treatment platelet reactivity and clinical outcome in cardiogenic shock patients undergoing primary PCI for acute myocardial infarction: results from the ISAR-SHOCK registry. Thromb Res. (2015) 136:87–93. doi: 10.1016/j.thromres.2015.04.029
18. Cutlip DE, Krucoff MW, Magnus Ohman E, Gabriel Victor Serebruany P, Valgimigli M, Vranckx P, et al. Standardized bleeding definitions for cardiovascular clinical trials a consensus report from the bleeding academic research consortium. Circulation. (2011) 123:2736–47. doi: 10.1161/CIRCULATIONAHA.110.009449
19. Ferrer DG, Jaldín-Fincati JR, Amigone JL, Capra RH, Collino CJ, Albertini RA, et al. Standardized flow cytometry assay for identification of human monocytic heterogeneity and LRP1 expression in monocyte subpopulations: decreased expression of this receptor in nonclassical monocytes. Cytom Part A. (2014) 85:601–10. doi: 10.1002/cyto.a.22455
20. Eitel I, De Waha S, Wöhrle J, Fuernau G, Lurz P, Pauschinger M, et al. Comprehensive prognosis assessment by CMR imaging after ST-segment elevation myocardial infarction. J Am Coll Cardiol. (2014) 64:1217–26. doi: 10.1016/j.jacc.2014.06.1194
21. Han SH, Koh KK, Quon MJ, Lee Y, Shin EK. The effects of simvastatin, losartan, and combined therapy on soluble CD40 ligand in hypercholesterolemic, hypertensive patients. Atherosclerosis. (2007) 190:205–11. doi: 10.1016/j.atherosclerosis.2006.01.021
22. Piqueras L, Kubes P, Alvarez A, O'Connor E, Issekutz AC, Esplugues J V., et al. Angiotensin II induces leukocyte-endothelial cell interactions in vivo via AT1 and AT2 receptor-mediated P-selectin upregulation. Circulation. (2000) 102:2118–23. doi: 10.1161/01.CIR.102.17.2118
23. Kander T, Brokopp J, Friberg H, Schött U. Wide Temperature range testing with ROTEM coagulation analyses. Ther Hypothermia Temp Manag. (2014) 4:125–30. doi: 10.1089/ther.2014.0005
24. Stockmann H, Krannich A, Schroeder T, Storm C. Therapeutic temperature management after cardiac arrest and the risk of bleeding: systematic review and meta-analysis. Resuscitation. (2014) 85:1494–503. doi: 10.1016/j.resuscitation.2014.07.018
25. Jacob M, Hassager C, Bro-Jeppesen J, Ostrowski SR, Thomsen JH, Wanscher M, et al. The effect of targeted temperature management on coagulation parameters and bleeding events after out-of-hospital cardiac arrest of presumed cardiac cause. Resuscitation. (2015) 96:260–7. doi: 10.1016/j.resuscitation.2015.08.018
26. Scharbert G, Kalb ML, Essmeister R, Kozek-Langenecker SA. Mild and moderate hypothermia increases platelet aggregation induced by various agonists: a whole blood in vitro study. Platelets. (2010) 21:44–8. doi: 10.3109/09537100903420269
27. Jeppesen AN, Kirkegaard H, Ilkjær S, Hvas AM. Influence of temperature on thromboelastometry and platelet aggregation in cardiac arrest patients undergoing targeted temperature management. Crit Care. (2016) 20:118. doi: 10.1186/s13054-016-1302-9
28. Winokur R, Hartwig JH. Mechanism of shape change in chilled human platelets. Blood. (1995) 85:1796–804.
29. Hoffmeister KM, Felbinger TW, Falet H, Denis C V., Bergmeier W, Mayadas TN, et al. The clearance mechanism of chilled blood platelets. Cell. (2003) 112:87–97. doi: 10.1016/S0092-8674(02)01253-9
30. Faraday N, Rosenfeld BA. In vitro hypothermia enhances platelet GPIIb-IIIa activation and P-selectin expression. Anesthesiology. (1995) 88:1579–85. doi: 10.1097/00000542-199806000-00022
31. Alexopoulos D, Xanthopoulou I, Gkizas V, Kassimis G, Theodoropoulos KC, Makris G, et al. Randomized assessment of ticagrelor versus prasugrel antiplatelet effects in patients with ST-segment-elevation myocardial infarction. Circ Cardiovasc Interv. (2012) 5:797–804. doi: 10.1161/CIRCINTERVENTIONS.112.972323
32. Parodi G, Valenti R, Bellandi B, Migliorini A, Marcucci R, Comito V, et al. Comparison of prasugrel and ticagrelor loading doses in ST-segment elevation myocardial infarction patients: RAPID (rapid activity of platelet inhibitor drugs) primary PCI study. J Am Coll Cardiol. (2013) 61:1601–6. doi: 10.1016/j.jacc.2013.01.024
33. Straub A, Krajewski S, Hohmann JD, Westein E, Jia F, Bassler N, et al. Evidence of platelet activation at medically used hypothermia and mechanistic data indicating ADP as a key mediator and therapeutic target. Arterioscler Thromb Vasc Biol. (2011) 31:1607–16. doi: 10.1161/ATVBAHA.111.226373
34. Bergman R, Braber A, Adriaanse MA, Van Vugt R, Tjan DHT, Van Zanten ARH. Haemodynamic consequences of mild therapeutic hypothermia after cardiac arrest. Eur J Anaesthesiol. (2010) 27:383–7. doi: 10.1097/EJA.0b013e3283333a7d
35. Raper RF, Cameron G, Walker D, Jane Bowey C. Type B lactic acidosis following cardiopulmonary bypass. Crit Care Med. (1997) 25:46–51. doi: 10.1097/00003246-199701000-00011
36. Aoki M, Nomura F, Stromski ME, Tsuji MK, Fackler JC, Hickey PR, et al. Effects of pH on brain energetics after hypothermic circulatory arrest. Ann Thorac Surg. (1993) 55:1093–103. doi: 10.1016/0003-4975(93)90014-9
Keywords: mild therapeutic hypothermia, myocardial infarction, STEMI, hemostasis, platelets
Citation: Scherz T, Hofbauer TM, Ondracek AS, Simon D, Sterz F, Testori C, Lang IM and Mangold A (2021) Mild Therapeutic Hypothermia Alters Hemostasis in ST Elevation Myocardial Infarction Patients. Front. Cardiovasc. Med. 8:707367. doi: 10.3389/fcvm.2021.707367
Received: 09 May 2021; Accepted: 15 June 2021;
Published: 06 July 2021.
Edited by:
Turgay Celik, VM Medical Park Ankara (Kecioren), TurkeyReviewed by:
Mahmut Ilker Yilmaz, Gulhane School of Medicine, TurkeyMehmet Tolga Dogru, Kirikkale University, Turkey
Copyright © 2021 Scherz, Hofbauer, Ondracek, Simon, Sterz, Testori, Lang and Mangold. This is an open-access article distributed under the terms of the Creative Commons Attribution License (CC BY). The use, distribution or reproduction in other forums is permitted, provided the original author(s) and the copyright owner(s) are credited and that the original publication in this journal is cited, in accordance with accepted academic practice. No use, distribution or reproduction is permitted which does not comply with these terms.
*Correspondence: Irene M. Lang, irene.lang@meduniwien.ac.at