- 1Klinik für Innere Medizin II, Universitätsklinikum Ulm, Ulm, Germany
- 2Department of Cardiology, Charité-University-Medicine (Campus Virchow – Klinikum), Berlin, Germany
- 3Department of Medicine and Surgery, University of Milan-Bicocca, Milan, Italy
Right ventricular (RV) systolic function has an important role in the prediction of adverse outcomes, including mortality, in a wide range of cardiovascular (CV) conditions. Because of complex RV geometry and load dependency of the RV functional parameters, conventional echocardiographic parameters such as RV fractional area change (FAC) and tricuspid annular plane systolic excursion (TAPSE), have limited prognostic power in a large number of patients. RV longitudinal strain overcame the majority of these limitations, as it is angle-independent, less load-dependent, highly reproducible, and measure regional myocardial deformation. It has a high predictive value in patients with pulmonary hypertension, heart failure, congenital heart disease, ischemic heart disease, pulmonary embolism, cardiomyopathies, and valvular disease. It enables detection of subclinical RV damage even when conventional parameters of RV systolic function are in the normal range. Even though cardiac magnetic resonance-derived RV longitudinal strain showed excellent predictive value, echocardiography-derived RV strain remains the method of choice for evaluation of RV mechanics primarily due to high availability. Despite a constantly growing body of evidence that support RV longitudinal strain evaluation in the majority of CV patients, its assessment has not become the part of the routine echocardiographic examination in the majority of echocardiographic laboratories. The aim of this clinical review was to summarize the current data about the predictive value of RV longitudinal strain in patients with pulmonary hypertension, heart failure and valvular heart diseases.
Introduction
The right ventricle (RV) has a complex anatomy and position in the thorax and therefore its assessment has long been limited and included a few conventional parameters that are easy to measure. Studies reported a significant prognostic effect of these RV parameters in a wide range of cardiovascular (CV) conditions such as pulmonary hypertension, congenital heart disease, pulmonary embolism, heart failure, cardiomyopathies, and valvular disease (1–6). Interestingly, RV parameters were reported as significant independent predictors of outcome in the general population and patients with arterial hypertension (7, 8). The development of imaging techniques provided detailed insight into RV structure, function and mechanics. Cardiac magnetic resonance and 3D echocardiography significantly improved our knowledge about RV structure and function, but speckle-tracking imaging enabled efficient and prompt echocardiographic assessment of RV mechanics (9).
RV longitudinal strain represents the cornerstone of RV mechanics evaluation with excellent reproducibility and high predictive value in patients with different aforementioned CV diseases (10–13). It has higher sensitivity and specificity in the detection of subclinical RV damage in patients with cardiomyopathies, cardiac amyloidosis and cancer (14–16). Although cardiac magnetic resonance provides more possibilities, that include the accurate evaluation of RV volumes and function, its relatively low availability and high level of competence that this kind of imaging demands, represent the main obstacles for its adoption in everyday clinical practice. Echocardiography-derived RV strain remains the method of choice for the evaluation of RV mechanics. Despite a constantly growing body of evidence that support RV longitudinal strain evaluation in the majority of CV patients, its assessment has not become part of the routine echocardiographic examination in majority of echocardiographic laboratories.
The aim of this clinical review was to summarize the current data about the predictive value of RV longitudinal strain in patients with pulmonary hypertension, heart failure, and valvular diseases in order to emphasize the importance of its evaluation in routine echocardiographic examination in these conditions.
Right Ventricular Strain—Basic Characteristics
The retrosternal position of the RV and its unique crescent-shaped geometry with inflow and outflow tracts in almost the same plane is challenging for echocardiographic assessment (17). Several echocardiographic views should be used for appropriate evaluation of RV structure and function and not all of them are part of the basic echocardiographic examination that underwent the majority of CV patients. Therefore, practically all parameters that are recommended by the guidelines (RV diameters, TAPSE, FAC, s′, E/e′, sPAP) are assessed in a 4-chamber view (18), which is easy to access.
The evaluation of RV longitudinal strain is also performed in 4-chamber view, which certainly represents an advantage because it does not demand additional time for acquisition (Figure 1). RV wall is significantly thinner than in the left ventricle (LV) and consists of only two layers that are predominantly longitudinally and obliquely directed in the free wall (17). The deep subendocardial fibers have longitudinal direction from base to apex, while the superficial subepicardial fibers normally have circumferential direction, parallel to the AV groove (19), but they turn obliquely as they approach to the apex of the heart and continue onto the LV. The continuity of RV and LV fibers significantly contributes to ventricular interdependence (19), which is important for both RV and LV function.
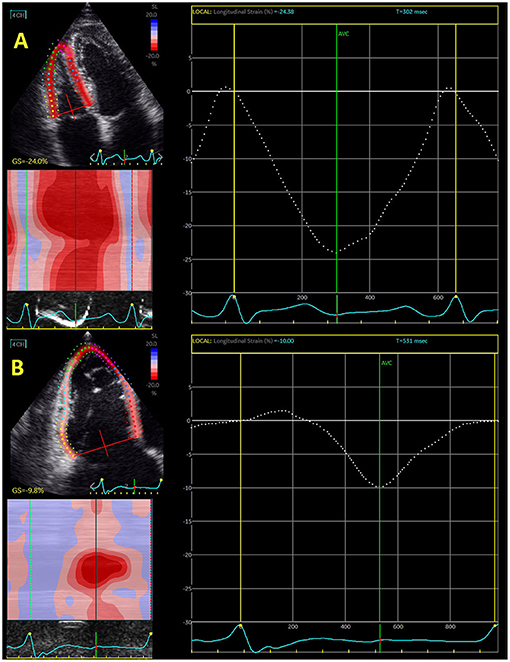
Figure 1. Echocardiography-derived right ventricular global longitudinal strain in control subjects (A) and patient with severe functional tricuspid regurgitation (B).
The technical aspects of image acquisition for speckle-tracking assessment are specific because they need to provide optimal images for post processing and high level of reproducibility for strain assessment. The suggested frame rate is between 40 and 90 frames per second for analysis of cardiac deformation. With increase of heart rate requires a higher frame rate to allow optimal speckle-tracking echocardiography. The acquisition of high-quality images is necessary for strain analysis, which considers the optimal gain settings and breath-hold techniques to clearly define the endocardial and epicardial borders and to avoid artifact associated to rib or lung motions and translational heart movement. RV focused apical 4-chamber view is necessary for RVLS evaluation. The optimal RV focused view should provide the visualization of the whole RV, the RV-free wall, and avoid foreshortening of the RV apex.
There is still no consensus about the region of interest that should be included in evaluation of RVLS. Some authors suggest a full RV chamber six-segmental model including the interventricular septum into the calculation of RVLS, whereas more authors accepted a three-segmental model, which involves only the RV free wall (20). The six-segment model is more feasible than three-segment model due to easier tracking of the whole RV. Absolute LS values derived from the free wall of the RV are significantly higher than from the whole RV. The authors that promote three-segmental model claim that the septum is traditionally seen as part of the LV and therefore should not be included into RVLS assessment.
The normal values of RVLS are still a matter of debate. Study that included significant number of patients reported lower RVLS values in men than in women, independently of the three- or six-segment model (21). Reference limits of normality for RVLS were −20% for men and −20.3% for women, whereas for RV free-wall LS limits were −22.5% for men and −23.3% for women (21).
There are several commercially available software platforms that enable post-process evaluation of raw data. EchoPac (GE) and TomTec are the most frequently used in clinical and research circumstances. EchoPac provided six-segment model and curve for each RV segment (including interventricular septum) and three-segmental model can be derived from these curves involving only segments of RV free wall. However, it is vendor dependent tool and can be used only for evaluation of echocardiographic images obtained by GE machines. TomTec is a vendor-independent software that can measure all echocardiographic images and provides automatically three-segment model for RV free-wall LS evaluation (separate values for free-wall RV and interventricular septum).
RV strain has several advantages: angle-independency, less load-dependency, and accuracy in measurement of regional myocardial deformation. The major limitation of 2D RV strain is motion-dependent speckles loss outside the imaging plane, especially due to excessive motion of the RV lateral wall. Nevertheless, this has been largely overcome with the introduction of software that provides dedicated RV longitudinal strain analysis and separate evaluation of RV free-wall longitudinal strain and RV global longitudinal strain. The important limitations are also lack of agreement about method that should be used for RVLS evaluation (three- vs. six-model) and unknown normal values for RVLS. Advantages and limitations are presented in Table 1. Even though all parameters of RV systolic function—TAPSE, FAC, and RV free-wall longitudinal function were reported as independent predictors of overall and CV mortality in patients with different CVD, RV free-wall longitudinal strain was proven to be the only independent predictor in analysis adjusted for multiple demographic, clinical and echocardiographic characteristics (22, 23). Study that used echocardiographic and CMR evaluation of RV function showed good correlation between TAPSE, s′, FAC, and global RV longitudinal strain and CMR-derived RV ejection fraction (24). Free-wall RV longitudinal strain showed the highest diagnostic accuracy and very high sensitivity and specificity to predict reduced EV ejection fraction (RVEF) <45% (24).
Rv Strain in Pulmonary Arterial Hypertension
The majority of studies investigated the predictive role of RV longitudinal strain in patients with idiopathic pulmonary arterial hypertension (PAH), but the number of researches that examined patients with pulmonary hypertension in pulmonary diseases (COPD, interstitial fibrosis) and connective tissue diseases (erythematous lupus, systemic sclerosis) is constantly increasing.
In patients with PAH, conventional parameters of RV systolic function, including TAPSE, FAC, s′, and RVEF, may be normal despite abnormal RV strain, which is an additional reason for the usage of RV strain in these patients. RV longitudinal strain can identify subclinical RV dysfunction at the early phase of disease and may serve as an important marker of subtle RV systolic dysfunction when conventional parameters, primarily TAPSE, are in the normal range (25, 26).
Small study that included 42 patients with PAH investigated the influence of different echocardiographic parameters on 4-year outcome and reported that RV free-wall longitudinal strain showed the best specificity and sensitivity in prediction of CV events in this population, significantly better than TAPSE, FAC, s′, and RV index of myocardial performance (27). PAH patients with RV free-wall longitudinal strain <-19.4% experienced fewer cardiovascular events than those with RV free-wall strain ≥-19.4% (27). Another study that investigated 51 PAH patients demonstrated that RV global longitudinal strain ≥-15.5% was independent predictor of adverse clinical events and mortality (28). The large study that included 575 patients with confirmed or suspected PAH reported significant decline in RV free-wall longitudinal strain proportional to worse functional class, shorter 6-min walk distance, higher N-terminal pro-B-type natriuretic peptide level, and the presence of right heart failure (29). RV free-wall longitudinal strain predicted survival after adjustment for pulmonary pressure, pulmonary vascular resistance, and right atrial pressure (29). Haeck et al. (30) involved 150 PH patients and demonstrated that RV free-wall strain ≥-19%, unlike TAPSE and FAC, was an independent predictor of all-cause mortality in these patients. All findings are summarized in Table 2.
Badagliacca et al. (31) recently identified 3 different RV free-wall strain patterns in PAH patients based on the time period from peak-systolic strain to return to the baseline point-set for the basal and mid-RV free wall segments. The authors reported that the shape of the RV strain curve and the longer time necessary to return RV strain to the baseline point were associated with worse outcome. Therefore, pattern 3, which corresponded to slow and steady gradual movement of a strain-derived curve, had the worst event-free survival during follow-up of 24 months (31) (Table 2).
Not only RV strain at baseline, but also its change during therapy and follow-up is important for prognosis of PAH patients. Hardegree et al. (32) reported that ≥5% improvement in RV free-wall longitudinal strain had >7-fold lower mortality during the follow-up of 4 years.
Meta-analysis that involved 1,169 PH patients revealed that RV free-wall strain >-19% had a significantly higher risk for the combined endpoint (mortality and PH-related events), while patients with >-22% had a significantly higher risk for all-cause mortality (33). It showed that a relative reduction of RV longitudinal strain <10% was insignificant for survival after multivariate analysis, while a relative reduction >10% of RV longitudinal strain represented significant and independent risk factor of adverse outcome in PH patients (33) (Table 4).
The role of CMR-derived RV longitudinal strain in prediction of adverse outcome in PAH patients is insufficiently known because of scarce data. Recent study that included 80 PAH patients provided CMR analysis of all RV and right atrial (RA) structural, functional and strain parameters and showed that RV longitudinal strain, TAPSE, FAC, and RA longitudinal strain were predictors of adverse outcome in these patients (34). Sato et al. (35) showed the improvement of CMR-derived RV strain during ambrisentan and tadalafil combination therapy in PAH patients with systemic sclerosis (Table 3).
RV Strain in Heart Failure
RV dysfunction is known indicator of poor survival in patients with heart failure (HF) (55). Conventional echocardiographic parameters for assessment of RV systolic function such as TAPSE and FAC have already been proven as independent predictors of adverse outcome in HF (56). Dini et al. (57) showed not only that a reduced RV function at baseline was independent predictor of poor outcome, but also that the reversibility of abnormal RV function, assessed by TAPSE, was related with a better prognosis, independently of LVEF improvement, LV reverse remodeling, and cardiac resynchronization therapy. Study that included patients after acute myocardial infarction reported that FAC, used for evaluation of RV systolic function, was associated with an increased risk of all-cause mortality, CV death, sudden death, HF, and stroke (58). Another investigation that used tissue Doppler parameter for evaluation of RV systolic function (s′) in patients with HF with preserved LVEF showed that s′ <5 cm/s was predictor of CV mortality, recurrent HF, and ischemic stroke (59).
Investigations proved that RV longitudinal strain is deteriorated at early stage of HF (3). Data from the literature indicate that RV longitudinal strain provides a more accurate and less preload dependent estimation of the overall performance of the RV in HF patients (3). The majority of data are focused on patients with HF with reduced LVEF (HFrEF) (45, 46, 60). However, data regarding HF with preserved LVEF (HFpEF) also emphasize the important role of RV longitudinal strain in these patients (3). Table 3 summarizes all findings in this field.
Study that involved patients with HFrEF referred for heart transplantation reported that free-wall and global RV longitudinal strains, NT-pro-BNP, FAC, and LV end-diastolic volume were independent predictors of combined outcome (hospitalization for acute heart failure, cardiovascular death, heart transplantation, intra-aortic balloon pump implantation, and ventricular assist device implantation) (45). Free-wall RV longitudinal strain was the strongest predictor of combined outcome, even stronger than LV global longitudinal strain, which support importance of RV strain in prognostic stratification in HF patients (45). Another investigation showed that only RV free-wall longitudinal strain, but not TAPSE, FAC, and s′, was independent predictor of CV events (hospitalization and CV mortality) in HFrEF patients during 3-year follow-up (47). Carluccio et al. (48) revealed that RV free-wall longitudinal strain was a better predictor than TAPSE in HFrEF patients with the best discriminatory value of RV free-wall longitudinal strain for the prediction of adverse outcomes was −15.3%. Small study revealed that RV longitudinal strain <-16% in HFrEF patients was a good predictor of functional capacity improvement (VO2 peak) in HFrEF patients who were referred for cardiac rehabilitation (49). Another investigation found that RV free-wall strain was a predictor of adverse CV events (death, cardiac transplantation, and hospitalization due to HF) independently of age, LVEF, tricuspid s′, E/e′ septal, and right atrial volume index in a population of patients with chronic HFrEF who were followed for 5 years (11). The cut-off value used for RV free-wall strain was −14.8%. Study that combined CMR and echocardiographic evaluation of the RV in HFrEF patients showed that echocardiography-derived RV free-wall longitudinal strain was a better predictor of overall and CV mortality than TAPSE, FAC, and CMR-derived RVEF, RV longitudinal strain during 4.7-year follow-up period (50). Figure 2 illustrates RV free-wall longitudinal strain in control subjects and HFrEF patient.
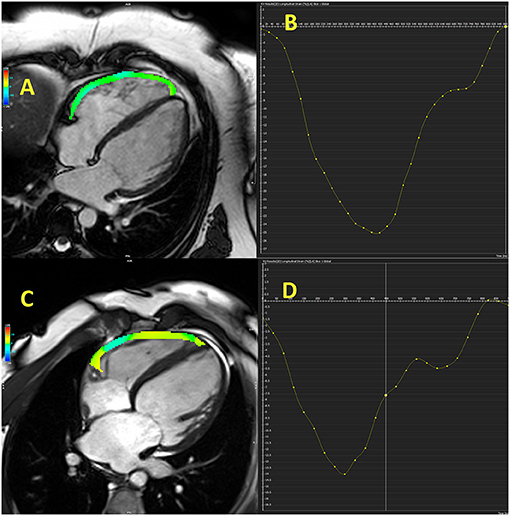
Figure 2. Cardiac magnetic resonance-derived right ventricular free-wall longitudinal strain in control subject (A,B) and patient with heart failure with reduced ejection fraction (C,D).
Iacoviello et al. (60) demonstrated that both global and free-wall RV longitudinal strain were independent predictors of all-cause and CV mortality in chronic HFrEF patients during 3-year follow-up. Even though TAPSE, FAC, and s′ were predictors of mortality in univariate analysis, they were not included in multivariate analysis. On the other hand, Carluccio et al. (11) found superior predictive value of RV free-wall strain over RV global strain in HFrEF patients over 30-month follow-up. Global RV longitudinal strain did not remain independent predictor of composite outcome (all-cause death/HF-related hospitalization) in the models that included LV parameters (LVEF, LV longitudinal strain, left atrial volume index) and other RV parameters (tricuspid regurgitation severity, RV dimensions, systolic pulmonary pressure), whereas RV free-wall strain remained an independent predictor in all models (51) (Table 3).
The investigation that combined echocardiographic analysis with myocardial histologic analysis reported that RV free-wall longitudinal strain was the main determinant of myocardial fibrosis, which may explain high prognostic value of this parameter in HF patients (52). RV free-wall strain had the highest diagnostic accuracy for detecting severe myocardial fibrosis, much better than TAPSE, right atrial longitudinal strain and VO2 peak (52).
In patients with acute HF decompensation RV free-wall longitudinal strain was an independent predictor of cardiac events (CV death or unplanned hospitalization due to HF worsening) (46). Other echocardiographic parameters such as LVEF, TAPSE, FAC, RV and LV global longitudinal strains were not proven to be the independent predictors of outcome in these patients (46). The cut-off value for RV free-wall longitudinal strain that showed the highest sensitivity and specificity was −13.1% (46). In the similar group of patients with acute HF authors found that global RV longitudinal strain was a predictor of all-cause mortality regardless of LV global longitudinal strain and clinical characteristics (53). Patients with reduced biventricular longitudinal strain (>-9% for LV and >-12% for RV) had the worst prognosis (53). The predictive power of RV global longitudinal strain was more significant in the absence of PH (Table 3).
Bosch et al. (54) reported gradual and significant deterioration of RV longitudinal strain from controls across HFpEF to HFrEF patients. The authors reported that RV free-wall longitudinal strain, sPAP and their ratio were independent predictors of composite endpoint (mortality and HF hospitalization) in the whole population of HF patients (61). Unfortunately, the separate analyses of HFrEF and HFpEF patients were not performed and therefore predictive value of RV longitudinal strain in HFpEF remains to be determined.
RV Strain in Valvular Heart DiseaseS
The effect of RV function in patients with valvular disease has been investigated in the last several years and it has become evident that effect of RV dysfunction on outcome in these patients is not irrelevant. The majority of studies reported deterioration of RV longitudinal strain in patients with valvular diseases (61, 62), but some recent investigations revealed a significant predictive value of RV strain in patients with aortic stenosis, mitral and tricuspid regurgitation (12, 13, 40, 63). Data are still scarce but warrant a special attention due to constantly increasing number of transcatheter interventions in valvular diseases and importance to develop new scores for better risk stratification in patients who undergo these interventions. Table 4 summarized all findings.
Aortic Stenosis
Study that investigated RV free-wall longitudinal strain before and 1 year after transcatheter or surgical aortic valve replacement (TAVR or SAVR) showed that RV longitudinal strain did not significantly improve after TAVR, but it significantly deteriorated in SAVR group, even though TAVR group had worse baseline clinical characteristics (more patients in NYHA class III and IV and significantly higher EuroSCORE) (36). However, it must be noted that RV strain improved in TAVR group for 2.4% in absolute values, which was still not enough to reach statistical significance in a relative small sample (n = 101). Interestingly, TAPSE and FAC also significantly reduced after SAVR and remained the same in TAVR group (36). This difference potentially could be due to pericardiotomy during SAVR. Another investigation that included patients after SAVR revealed that RV free-wall longitudinal strain >-15% had high sensitivity and specificity for development of low output cardiac syndrome in the first 30 days after surgery, which may qualify RV strain for risk stratification undergoing SAVR (37). The same group of authors recently provided results form long follow-up and showed that RV free-wall longitudinal strain was independent predictor of low output cardiac syndrome, but not of in-hospital mortality, hospital stay, or vasoplegic syndrome (38).
Dahou et al. (63) investigated patients with low-flow low-gradient aortic stenosis and found that 2-year survival in patients with RV free-wall longitudinal strain >-13% was significantly lower than in those with RV free-wall longitudinal strain <-13%. RV free-wall longitudinal strain >-13% was an independent predictor of mortality in these patients, regardless age, aortic stenosis severity, myocardial infarction and LV longitudinal strain (63). Stress RV longitudinal strain, measured during low dose dobutamine stress, has incremental prognostic value over LV longitudinal strain, LVEF and RV strain at rest (63) (Table 4).
Research that included HF patients due to severe aortic stenosis undergoing TAVR reported that RV global longitudinal strain and RV-arterial coupling (TAPSE/sPAP and RV longitudinal strain/sPAP) provided better risk stratification than other RV echocardiographic parameters during long-term follow up of 10 years (39). TAPSE, FAC, s′, sPAP, tricuspid regurgitation and tricuspid annulus diameter were not proven to be the independent predictors of 10-year mortality after TAVR (39).
Recent large study that involved 334 patients who underwent TAVR reported no significant change in RV free-wall longitudinal strain at 1-year follow-up, in both groups with baseline impaired and normal RV systolic function (12). However, RV longitudinal strain was associated with 1-year all-cause mortality. The authors found a 6% higher risk for 1-year mortality for each unit increase in RV longitudinal strain. Other parameters of RV systolic function (TAPSE, FAC, s′), as well as LVEF, existence of tricuspid regurgitation and sPAP were not independent predictors of 1-year mortality in these patients (12). These findings support the routine assessment of RV longitudinal strain in patients undergoing SAVR or TAVR in order to improve the risk stratification (Table 4).
Mitral Regurgitation
RV systolic function is a very important in patients undergoing mitral valve surgery and some studies showed its strong predictive value for long-term mortality in a large population of patients who underwent cardiac surgery (valve, valve + CABG, CABG) (64). RV systolic function, assessed by TAPSE, FAC, and s′, remained depressed shortly after mitral valve surgery (65).
Study that involved patients who underwent mitral valve surgery reported significant deterioration of RV free-wall longitudinal strain in these patients (40). The authors found smaller reductions in patients who underwent robotic-assisted mitral valve repair than in patients who underwent standard mitral valve repair. The patients who underwent robotic-assisted mitral valve repair also showed greater recovery in RV longitudinal strain at 1-year follow-up comparing with pre-surgery values (40). Similar investigation that involved patients who underwent surgical mitral valve repair showed that only resolution of RV longitudinal strain at 1 month predicted the subsequent myocardial recovery (41). TAPSE, FAC, and s′ did not have any role in this prediction. Patients with preserved RV systolic function had a lower risk of hospitalization due to HF compared to those with reduced RV function (41) (Table 4).
Large research that involved 568 patients with severe ischemic mitral regurgitation who underwent surgical valve repair and 131 patients with concomitant tricuspid valve repair demonstrated that the RV continued to dilate while the LV reduced during follow-up after surgery (42). RVEF decreased, while LVEF increased during 6.3-year follow-up. RV longitudinal strain showed continuous deterioration during follow-up period. The largest decline in RV longitudinal strain occurred in the first month after surgery, while it remained constant after first year of follow-up (42). RV longitudinal strain, FAC and TAPSE were significantly lower in patients who underwent tricuspid valve repair than in those who did not undergo tricuspid valve repair. RV strain and FAC did not significantly recover during follow-up, while TAPSE significantly improved in both groups—with and without tricuspid valve repair. These findings showed that surgical treatment of functional mitral and tricuspid regurgitation along with revascularization failed to induce improve RV function and reverse remodeling (Table 4).
Functional mitral regurgitation has been currently treated with interventional “edge-to-edge” technique and study that investigated patients with moderate and severe mitral regurgitation, who underwent MitraClip procedure, demonstrated no acute improvement (within 7 days) in CMR-derived RVEF, RV end-diastolic volume and tricuspid regurgitation (66).
Findings from aforementioned studies warrant further examinations to identify optimal timing and approach of intervention for functional mitral and tricuspid regurgitation.
Tricuspid Regurgitation
Isolated or concomitant functional tricuspid regurgitation has been extensively investigated in last decade. It has been shown that tricuspid regurgitation significantly deteriorated the outcome in patients with mitral regurgitation. However, surgical intervention seems not to improve RV function in these patients (42). Interventional “edge-to-edge” repair significantly increased interest for predictors that may improve outcome after this intervention. Hirasawa et al. (67) reported that reduction of RV free-wall longitudinal strain was proportional to the level of tricuspid regurgitation. Figure 1 shows difference in RV global longitudinal strain between control subjects and patients with severe functional tricuspid regurgitation.
Large study that included 1,089 patients with tricuspid regurgitation showed that reduced RV free-wall longitudinal strain (>-18%), TAPSE (<18.5 mm) and FAC (<35%) were independent predictors for 2-year all-cause mortality (66). The sensitivity and specificity to predict 2-year mortality gradually increased from FAC, across TAPSE, to RV longitudinal strain (43). Similar study that included 896 patients with significant tricuspid regurgitation defined reduced RV function as TAPSE <17 mm, FAC <35%, and RV free-wall longitudinal strain >-23% (22). Mortality was significantly higher in patients with decreased FAC, decreased TAPSE, and impaired RV free wall longitudinal strain. Multivariate analysis showed that RV free-wall longitudinal strain was independently associated with 2.8-year all-cause mortality and incremental to FAC and TAPSE (22). Reduced TAPSE and FAC also represented independent predictors for mortality after adjustment for age, LVEF, RV systolic pressure, significant left-sided valvular disease (22). However, after adjustment for concomitant diseases (diabetes mellitus, chronic kidney disease, coronary artery disease) as well as New York Heart Association class III/IV this independent predictive value vanished for FAC and TAPSE, but not for RV free-wall strain (22) (Table 4).
Investigation that enrolled 250 consecutive patients with severe tricuspid regurgitation investigated RV global and free-wall longitudinal strain and reclassified ~42–56% of patients with normal RV systolic function according to conventional parameters in patients with impaired RV systolic function (13). The authors found that RV free-wall strain >-17% at baseline predicted RV heart failure, whereas patients with RV free-wall strain <-14% at follow-up had significantly better survival (13) (Table 4).
Recent study included 544 patients with severe functional tricuspid regurgitation with a median follow-up of 6 years (43). Patients with CMR-derived RV free-wall longitudinal strain >-16% had significantly lower survival compared with those with lower RV free-wall longitudinal strain (44). CMR-derived RV longitudinal strain was independent predictor of mortality after adjustment for clinical and imaging risk factors, including RV size and ejection fraction (Table 4).
Current studies support the evaluation of RV longitudinal strain due to its high predictive value in patients with tricuspid regurgitation. Data about the effect of interventional “edge-to-edge” tricuspid valve repair are expected and they will provide some new guidelines if RV longitudinal strain should be involved in routine echocardiographic examination and it seems that body of evidence that supports this evaluation is constantly enlarging.
Conclusion
RV longitudinal strain is a significant parameter of RV dysfunction that showed better sensitivity than conventional echocardiographic parameters. Latest investigation demonstrated its role in prediction of adverse outcome in wide range of CV conditions, but the most encouraging data came from studies that were focused on pulmonary hypertension, HFrEF and tricuspid regurgitation. Data about the predictive importance of RV longitudinal strain in HFpEF patients and those with aortic stenosis and mitral regurgitation are still scarce and warrant further investigation. Adding RV longitudinal strain to existing scores might significantly help in the risk stratification, which could induce reclassification of some patients to higher risk group. Existing knowledge about the predictive value of RV longitudinal strain supports its routine echocardiographic evaluation in patients with CV diseases.
Author Contributions
MT wrote the article. NN, LS, JK, and CR reviewed the literature. JK made figures. DB, BG, and DS helped in preparing the text of the manuscript. EB, CC, and WR reviewed the article with important scientific input. All authors contributed to the article and approved the submitted version.
Conflict of Interest
The authors declare that the research was conducted in the absence of any commercial or financial relationships that could be construed as a potential conflict of interest.
References
1. Dong Y, Pan Z, Wang D, Lv J, Fang J, Xu R, et al. Prognostic value of cardiac magnetic resonance-derived right ventricular remodeling parameters in pulmonary hypertension: a systematic review and meta-analysis. Circ Cardiovasc Imaging. (2020) 13:e010568. doi: 10.1161/CIRCIMAGING.120.010568
2. Graziani F, Lillo R, Panaioli E, Pieroni M, Camporeale A, Verrecchia E, et al. Prognostic significance of right ventricular hypertrophy and systolic function in Anderson-Fabry disease. ESC Heart Fail. (2020) 7:1605–14. doi: 10.1002/ehf2.12712
3. Shah AM, Cikes M, Prasad N, Li G, Getchevski S, Claggett B, et al. Echocardiographic features of patients with heart failure and preserved left ventricular ejection fraction. J Am Coll Cardiol. (2019) 74:2858–73. doi: 10.1016/j.jacc.2019.09.063
4. Lyhne MD, Kabrhel C, Giordano N, Andersen A, Nielsen-Kudsk JE, Zheng H, et al. The echocardiographic ratio tricuspid annular plane systolic excursion/pulmonary arterial systolic pressure predicts short-term adverse outcomes in acute pulmonary embolism. Eur Heart J Cardiovasc Imaging. (2021) 22:285–94. doi: 10.1093/ehjci/jeaa243
5. Dini FL, Conti U, Fontanive P, Andreini D, Banti S, Braccini L, et al. Right ventricular dysfunction is a major predictor of outcome in patients with moderate to severe mitral regurgitation and left ventricular dysfunction. Am Heart J. (2007) 154:172–9. doi: 10.1016/j.ahj.2007.03.033
6. Karam N, Stolz L, Orban M, Deseive S, Praz F, Kalbacher D, et al. Impact of right ventricular dysfunction on outcomes after transcatheter edge-to-edge repair for secondary mitral regurgitation. JACC Cardiovasc Imaging. (2021) 14:768–78. doi: 10.1016/j.jcmg.2020.12.015
7. Kawut SM, Barr RG, Lima JA, Praestgaard A, Johnson WC, Chahal H, et al. Right ventricular structure is associated with the risk of heart failure and cardiovascular death: the Multi-Ethnic Study of Atherosclerosis (MESA)–right ventricle study. Circulation. (2012) 126:1681–8. doi: 10.1161/CIRCULATIONAHA.112.095216
8. Tadic M, Cuspidi C, Celic V, Petrovic O, Pencic B, Mancia G, et al. The prognostic importance of right ventricular remodeling and the circadian blood pressure pattern on the long-term cardiovascular outcome. J Hypertens. (2020) 38:1525–30. doi: 10.1097/HJH.0000000000002432
9. Erley J, Tanacli R, Genovese D, Tapaskar N, Rashedi N, Bucius P, et al. Myocardial strain analysis of the right ventricle: comparison of different cardiovascular magnetic resonance and echocardiographic techniques. J Cardiovasc Magn Reson. (2020) 22:51. doi: 10.1186/s12968-020-00647-7
10. Stolfo D, Albani S, Biondi F, De Luca A, Barbati G, Howard L, et al. Global right heart assessment with speckle-tracking imaging improves the risk prediction of a validated scoring system in pulmonary arterial hypertension. J Am Soc Echocardiogr. (2020) 33:1334–44. doi: 10.1016/j.echo.2020.05.020
11. Motoki H, Borowski AG, Shrestha K, Hu B, Kusunose K, Troughton RW, et al. Right ventricular global longitudinal strain provides prognostic value incremental to left ventricular ejection fraction in patients with heart failure. J Am Soc Echocardiogr. (2014) 27:726–32. doi: 10.1016/j.echo.2014.02.007
12. Medvedofsky D, Koifman E, Jarrett H, Miyoshi T, Rogers T, Ben-Dor I, et al. Association of right ventricular longitudinal strain with mortality in patients undergoing transcatheter aortic valve replacement. J Am Soc Echocardiogr. (2020) 33:452–60. doi: 10.1016/j.echo.2019.11.014
13. Ancona F, Melillo F, Calvo F, Attalla El Halabieh N, Stella S, Capogrosso C, et al. Right ventricular systolic function in severe tricuspid regurgitation: prognostic relevance of longitudinal strain. Eur Heart J Cardiovasc Imaging. (2021) jeab030. doi: 10.1093/ehjci/jeab030
14. Steen H, Giusca S, Montenbruck M, Patel AR, Pieske B, Florian A, et al. Left and right ventricular strain using fast strain-encoded cardiovascular magnetic resonance for the diagnostic classification of patients with chronic non-ischemic heart failure due to dilated, hypertrophic cardiomyopathy or cardiac amyloidosis. J Cardiovasc Magn Reson. (2021) 23:45. doi: 10.1186/s12968-021-00711-w
15. Fine NM, White JA, Jimenez-Zepeda V, Howlett JG. Determinants and prognostic significance of serial right heart function changes in patients with cardiac amyloidosis. Can J Cardiol. (2020) 36:432–40. doi: 10.1016/j.cjca.2020.01.020
16. Tadic M, Baudisch A, Haßfeld S, Heinzel F, Cuspidi C, Burkhardt F, et al. Right ventricular function and mechanics in chemotherapy- and radiotherapy-naïve cancer patients. Int J Cardiovasc Imaging. (2018) 34:1581–7. doi: 10.1007/s10554-018-1379-0
17. Tadic M. Multimodality evaluation of the right ventricle: an updated review. Clin Cardiol. (2015) 38:770–6. doi: 10.1002/clc.22443
18. Rudski LG, Lai WW, Afilalo J, Hua L, Handschumacher MD, Chandrasekaran K, et al. Guidelines for the echocardiographic assessment of the right heart in adults: a report from the American Society of Echocardiography endorsed by the European Association of Echocardiography, a registered branch of the European Society of Cardiology, and the Canadian Society of Echocardiography. J Am Soc Echocardiogr. (2010) 23:685–713. doi: 10.1016/j.echo.2010.05.010
19. Haddad F, Hunt SA, Rosenthal DN, Murphy DJ. Right ventricular function in cardiovascular disease, part I: anatomy, physiology, aging, and functional assessment of the right ventricle. Circulation. (2008) 117:1436–48. doi: 10.1161/CIRCULATIONAHA.107.653576
20. Badano LP, Muraru D, Parati G, Haugaa K, Voigt JU. How to do right ventricular strain. Eur Heart J Cardiovasc Imaging. (2020) 21:825–7. doi: 10.1093/ehjci/jeaa126
21. Muraru D, Onciul S, Peluso D, Soriani N, Cucchini U, Aruta P, et al. Sex- and method-specific reference values for right ventricular strain by 2-dimensional speckle-tracking echocardiography. Circ Cardiovasc Imaging. (2016) 9:e003866. doi: 10.1161/CIRCIMAGING.115.003866
22. Prihadi EA, van der Bijl P, Dietz M, Abou R, Vollema EM, Marsan NA, et al. Prognostic implications of right ventricular free wall longitudinal strain in patients with significant functional tricuspid regurgitation. Circ Cardiovasc Imaging. (2019) 12:e008666. doi: 10.1161/CIRCIMAGING.118.008666
23. Li Y, Wang Y, Ye X, Kong L, Zhu W, Lu X. Clinical study of right ventricular longitudinal strain for assessing right ventricular dysfunction and hemodynamics in pulmonary hypertension. Medicine. (2016) 95:e5668. doi: 10.1097/MD.0000000000005668
24. Focardi M, Cameli M, Carbone SF, Massoni A, De Vito R, Lisi M, et al. Traditional and innovative echocardiographic parameters for the analysis of right ventricular performance in comparison with cardiac magnetic resonance. Eur Heart J Cardiovasc Imaging. (2015) 16:47–52. doi: 10.1093/ehjci/jeu156
25. Puwanant S, Park M, Popović ZB, Tang WHW, Farha S, George D, et al. Ventricular geometry, strain, and rotational mechanics in pulmonary hypertension. Circulation. (2010) 121:259–66. doi: 10.1161/CIRCULATIONAHA.108.844340
26. van Kessel M, Seaton D, Chan J, Yamada A, Kermeen F, Hamilton-Craig C, et al. Prognostic value of right ventricular free wall strain in pulmonary hypertension patients with pseudo-normalized tricuspid annular plane systolic excursion values. Int J Cardiovasc Imaging. (2016) 32:905–12. doi: 10.1007/s10554-016-0862-8
27. Motoji Y, Tanaka H, Fukuda Y, Ryo K, Emoto N, Kawai H, et al. Efficacy of right ventricular free-wall longitudinal speckle-tracking strain for predicting long-term outcome in patients with pulmonary hypertension. Circ J. (2013) 77:756–63. doi: 10.1253/circj.CJ-12-1083
28. Park JH, Park MM, Farha S, Sharp J, Lundgrin E, Comhair S, et al. Impaired global right ventricular longitudinal strain predicts long-term adverse outcomes in patients with pulmonary arterial hypertension. J Cardiovasc Ultrasound. (2015) 23:91–9. doi: 10.4250/jcu.2015.23.2.91
29. Fine NM, Chen L, Bastiansen PM, Frantz RP, Pellikka PA, Oh JK, et al. Outcome prediction by quantitative right ventricular function assessment in 575 subjects evaluated for pulmonary hypertension. Circ Cardiovasc Imaging. (2013) 6:711–21. doi: 10.1161/CIRCIMAGING.113.000640
30. Haeck ML, Scherptong RW, Marsan NA, Holman ER, Schalij MJ, Bax JJ, et al. Prognostic value of right ventricular longitudinal peak systolic strain in patients with pulmonary hypertension. Circ Cardiovasc Imaging. (2012) 5:628–36. doi: 10.1161/CIRCIMAGING.111.971465
31. Badagliacca R, Pezzuto B, Papa S, Poscia R, Manzi G, Pascaretta A, et al. Right ventricular strain curve morphology and outcome in idiopathic pulmonary arterial hypertension. JACC Cardiovasc Imaging. (2021) 14:162–72. doi: 10.1016/j.jcmg.2020.08.017
32. Hardegree EL, Sachdev A, Villarraga HR, Frantz RP, McGoon MD, Kushwaha SS, et al. Role of serial quantitative assessment of right ventricular function by strain in pulmonary arterial hypertension. Am J Cardiol. (2013) 111:143–8. doi: 10.1016/j.amjcard.2012.08.061
33. Hulshof HG, Eijsvogels TMH, Kleinnibbelink G, van Dijk AP, George KP, Oxborough DL, et al. Prognostic value of right ventricular longitudinal strain in patients with pulmonary hypertension: a systematic review and meta-analysis. Eur Heart J Cardiovasc Imaging. (2019) 20:475–84. doi: 10.1093/ehjci/jey120
34. Leng S, Dong Y, Wu Y, Zhao X, Ruan W, Zhang G, et al. Impaired cardiovascular magnetic resonance-derived rapid semiautomated right atrial longitudinal strain is associated with decompensated hemodynamics in pulmonary arterial hypertension. Circ Cardiovasc Imaging. (2019) 12:e008582. doi: 10.1161/CIRCIMAGING.118.008582
35. Sato T, Ambale-Venkatesh B, Lima JAC, Zimmerman SL, Tedford RJ, Fujii T, et al. The impact of ambrisentan and tadalafil upfront combination therapy on cardiac function in scleroderma associated pulmonary arterial hypertension patients: cardiac magnetic resonance feature tracking study. Pulm Circ. (2018) 8:2045893217748307. doi: 10.1177/2045893217748307
36. Kempny A, Diller GP, Kaleschke G, Orwat S, Funke A, Schmidt R, et al. Impact of transcatheter aortic valve implantation or surgical aortic valve replacement on right ventricular function. Heart. (2012) 98:1299–304. doi: 10.1136/heartjnl-2011-301203
37. Balderas-Muñoz K, Rodríguez-Zanella H, Fritche-Salazar JF, Ávila-Vanzzini N, Juárez Orozco LE, Arias-Godínez JA, et al. Improving risk assessment for post-surgical low cardiac output syndrome in patients without severely reduced ejection fraction undergoing open aortic valve replacement. The role of global longitudinal strain and right ventricular free wall strain. Int J Cardiovasc Imaging. (2017) 33:1483–9. doi: 10.1007/s10554-017-1139-6
38. Posada-Martinez EL, Fritche-Salazar JF, Arias-Godinez JA, Ortiz-Leon XA, Balderas-Muñoz K, Ruiz-Esparza ME, et al. Right ventricular longitudinal strain predicts low-cardiac- output syndrome after surgical aortic valve replacement in patients with preserved and mid-range ejection fraction. J Cardiothorac Vasc Anesth. (2020). 35(6):1638–1645. doi: 10.1053/j.jvca.2020.12.008
39. Vizzardi E, Gavazzoni M, Sciatti E, Dallapellegrina L, Bernardi N, Raddino R, et al. Right ventricular deformation and right ventricular-arterial coupling in patients with heart failure due to severe aortic stenosis undergoing TAVI: long-term results. Am J Cardiovasc Dis. (2020) 10:150–63.
40. Orde SR, Chung SY, Pulido JN, Suri RM, Stulak JM, Oh JK, et al. Changes in right ventricle function after mitral valve repair surgery. Heart Lung Circ. (2020) 29:785–792. doi: 10.1016/j.hlc.2019.06.724
41. Chang WT, Wu NC, Shih JY, Hsu CH, Chen ZC, Cheng BC. Right ventricular reserve post mitral valve repair is associated with heart failure hospitalization. Pulm Circ. (2020) 10:2045894020943858. doi: 10.1177/2045894020943858
42. Elgharably H, Javadikasgari H, Koprivanac M, Lowry AM, Sato K, Blackstone EH, et al. Right versus left heart reverse remodelling after treating ischaemic mitral and tricuspid regurgitation. Eur J Cardiothorac Surg. (2020) ezaa326. doi: 10.1093/ejcts/ezaa326
43. Bannehr M, Kahn U, Liebchen J, Okamoto M, Hähnel V, Georgi C, et al. Right ventricular longitudinal strain predicts survival in patients with functional tricuspid regurgitation. Can J Cardiol. (2021). doi: 10.1016/j.cjca.2021.01.006
44. Romano S, Dell'atti D, Judd RM, Kim RJ, Weinsaft JW, Kim J, et al. Prognostic value of feature-tracking right ventricular longitudinal strain in severe functional tricuspid regurgitation: a multicenter study. J Am Coll Cardiol Img. (2021). doi: 10.1016/j.jcmg.2021.02.009
45. Cameli M, Righini FM, Lisi M, Bennati E, Navarri R, Lunghetti S, et al. Comparison of right versus left ventricular strain analysis as a predictor of outcome in patients with systolic heart failure referred for heart transplantation. Am J Cardiol. (2013) 112:1778–84. doi: 10.1016/j.amjcard.2013.07.046
46. Hamada-Harimura Y, Seo Y, Ishizu T, Nishi I, Machino-Ohtsuka T, Yamamoto M, et al. Incremental prognostic value of right ventricular strain in patients with acute decompensated heart failure. Circ Cardiovasc Imaging. (2018) 11:e007249. doi: 10.1161/CIRCIMAGING.117.007249
47. Vizzardi E, D'Aloia A, Caretta G, Bordonali T, Bonadei I, Rovetta R, et al. Long-term prognostic value of longitudinal strain of right ventricle in patients with moderate heart failure. Hellenic J Cardiol. (2014) 55:150–5.
48. Carluccio E, Biagioli P, Alunni G, Murrone A, Zuchi C, Coiro S, et al. Prognostic value of right ventricular dysfunction in heart failure with reduced ejection fraction: superiority of longitudinal strain over tricuspid annular plane systolic excursion. Circ Cardiovasc Imaging. (2018) 11:e006894. doi: 10.1161/CIRCIMAGING.117.006894
49. Kusunose K, Seno H, Yamada H, Nishio S, Torii Y, Hirata Y, et al. Right ventricular function and beneficial effects of cardiac rehabilitation in patients with systolic chronic heart failure. Can J Cardiol. (2018) 34:1307–15. doi: 10.1016/j.cjca.2018.06.003
50. Houard L, Benaets MB, de Meester de Ravenstein C, Rousseau MF, Ahn SA, Amzulescu MS, et al. Additional Prognostic Value of 2D right ventricular speckle-tracking strain for prediction of survival in heart failure and reduced ejection fraction: a comparative study with cardiac magnetic resonance. JACC Cardiovasc Imaging. (2019) 12:2373–85. doi: 10.1016/j.jcmg.2018.11.028
51. Carluccio E, Biagioli P, Lauciello R, Zuchi C, Mengoni A, Bardelli G, et al. Superior prognostic value of right ventricular free wall compared to global longitudinal strain in patients with heart failure. J Am Soc Echocardiogr. (2019) 32:836–44. doi: 10.1016/j.echo.2019.02.011
52. Lisi M, Cameli M, Righini FM, Malandrino A, Tacchini D, Focardi M, et al. RV Longitudinal deformation correlates with myocardial fibrosis in patients with end-stage heart failure. JACC Cardiovasc Imaging. (2015) 8:514–22. doi: 10.1016/j.jcmg.2014.12.026
53. Park JH, Park JJ, Park JB, Cho GY. Prognostic value of biventricular strain in risk stratifying in patients with acute heart failure. J Am Heart Assoc. (2018) 7:e009331. doi: 10.1161/JAHA.118.009331
54. Bosch L, Lam CSP, Gong L, Chan SP, Sim D, Yeo D, et al. Right ventricular dysfunction in left-sided heart failure with preserved versus reduced ejection fraction. Eur J Heart Fail. (2017) 19:1664–71. doi: 10.1002/ejhf.873
55. Meyer P, Filippatos GS, Ahmed MI, Iskandrian AE, Bittner V, Perry GJ, et al. Effects of right ventricular ejection fraction on outcomes in chronic systolic heart failure. Circulation. (2010) 121:252–8. doi: 10.1161/CIRCULATIONAHA.109.887570
56. Ghio S, Recusani F, Klersy C, Sebastiani R, Laudisa ML, Campana C, et al. Prognostic usefulness of the tricuspid annular plane systolic excursion in patients with congestive heart failure secondary to idiopathic or ischemic dilated cardiomyopathy. Am J Cardiol. (2000) 85:837–42. doi: 10.1016/S0002-9149(99)00877-2
57. Dini FL, Carluccio E, Simioniuc A, Biagioli P, Reboldi G, Galeotti GG, et al. Right ventricular recovery during follow-up is associated with improved survival in patients with chronic heart failure with reduced ejection fraction. Eur J Heart Fail. (2016) 18:1462–71. doi: 10.1002/ejhf.639
58. Anavekar NS, Skali H, Bourgoun M, Ghali JK, Kober L, Maggioni AP, et al. Usefulness of right ventricular fractional area change to predict death, heart failure, and stroke following myocardial infarction (from the VALIANT ECHO Study). Am J Cardiol. (2008) 101:607–12. doi: 10.1016/j.amjcard.2007.09.115
59. Shin HW, Kim H, Son J, Yoon HJ, Park HS, Cho YK, et al. Tissue Doppler imaging as a prognostic marker for cardiovascular events in heart failure with preserved ejection fraction and atrial fibrillation. J Am Soc Echocardiogr. (2010) 23:755–61. doi: 10.1016/j.echo.2010.05.003
60. Iacoviello M, Citarelli G, Antoncecchi V, Romito R, Monitillo F, Leone M, et al. Right ventricular longitudinal strain measures independently predict chronic heart failure mortality. Echocardiography. (2016) 33:992–1000. doi: 10.1111/echo.13199
61. Di Franco A, Kim J, Rodriguez-Diego S, Khalique O, Siden JY, Goldburg SR, et al. Multiplanar strain quantification for assessment of right ventricular dysfunction and non-ischemic fibrosis among patients with ischemic mitral regurgitation. PLoS ONE. (2017) 12:e0185657. doi: 10.1371/journal.pone.0185657
62. Tadic M, Cuspidi C, Pencic B, Ivanovic B, Grassi G, Kocijancic V, et al. The impact of arterial hypertension on left ventricular strain in patients with aortic stenosis and preserved ejection fraction. J Hypertens. (2019) 37:747–53. doi: 10.1097/HJH.0000000000001963
63. Dahou A, Clavel MA, Capoulade R, Bartko PE, Magne J, Mundigler G, et al. Right ventricular longitudinal strain for risk stratification in low-flow, low-gradient aortic stenosis with low ejection fraction. Heart. (2016) 102:548–54. doi: 10.1136/heartjnl-2015-308309
64. Bootsma IT, de Lange F, Koopmans M, Haenen J, Boonstra PW, Symersky T. Right ventricular function after cardiac surgery is a strong independent predictor for long-term mortality. J Cardiothorac Vasc Anesth. (2017) 31:1656–62. doi: 10.1053/j.jvca.2017.02.008
65. Van Orman JR, Connelly K, Albinmousa Z, Tousignant C. Early recovery of tricuspid annular isovolumic acceleration after mitral valve surgery - an observational study. Can J Anaesth. (2016) 63:920–7. doi: 10.1007/s12630-016-0651-9
66. Lurz P, Serpytis R, Blazek S, Seeburger J, Mangner N, Noack T, et al. Assessment of acute changes in ventricular volumes, function, and strain after interventional edge-to-edge repair of mitral regurgitation using cardiac magnetic resonance imaging. Eur Heart J Cardiovasc Imaging. (2015) 16:1399–404. doi: 10.1093/ehjci/jev115
67. Hirasawa K, van Rosendael PJ, Dietz MF, Ajmone Marsan N, Delgado V, Bax JJ. Comparison of the usefulness of strain imaging by echocardiography versus computed tomography to detect right ventricular systolic dysfunction in patients with significant secondary tricuspid regurgitation. Am J Cardiol. (2020) 134:116–22. doi: 10.1016/j.amjcard.2020.07.063
Keywords: right ventricle, longitudinal strain, outcome, pulmonary hypertension, heart failure, aortic stenosis, mitral regurgitation, tricuspid regurgitation
Citation: Tadic M, Nita N, Schneider L, Kersten J, Buckert D, Gonska B, Scharnbeck D, Reichart C, Belyavskiy E, Cuspidi C and Rottbauer W (2021) The Predictive Value of Right Ventricular Longitudinal Strain in Pulmonary Hypertension, Heart Failure, and Valvular Diseases. Front. Cardiovasc. Med. 8:698158. doi: 10.3389/fcvm.2021.698158
Received: 20 April 2021; Accepted: 24 May 2021;
Published: 17 June 2021.
Edited by:
Carla Sousa, São João University Hospital Center, PortugalReviewed by:
Mariana Paiva, Centro Hospitalar Universitário de São João (CHUSJ), PortugalHelena Nascimento, Centro Hospitalar de Entre o Douro e Vouga E.P.E., Portugal
Copyright © 2021 Tadic, Nita, Schneider, Kersten, Buckert, Gonska, Scharnbeck, Reichart, Belyavskiy, Cuspidi and Rottbauer. This is an open-access article distributed under the terms of the Creative Commons Attribution License (CC BY). The use, distribution or reproduction in other forums is permitted, provided the original author(s) and the copyright owner(s) are credited and that the original publication in this journal is cited, in accordance with accepted academic practice. No use, distribution or reproduction is permitted which does not comply with these terms.
*Correspondence: Marijana Tadic, bWFyaWphbmFfdGFkaWMmI3gwMDA0MDtob3RtYWlsLmNvbQ==