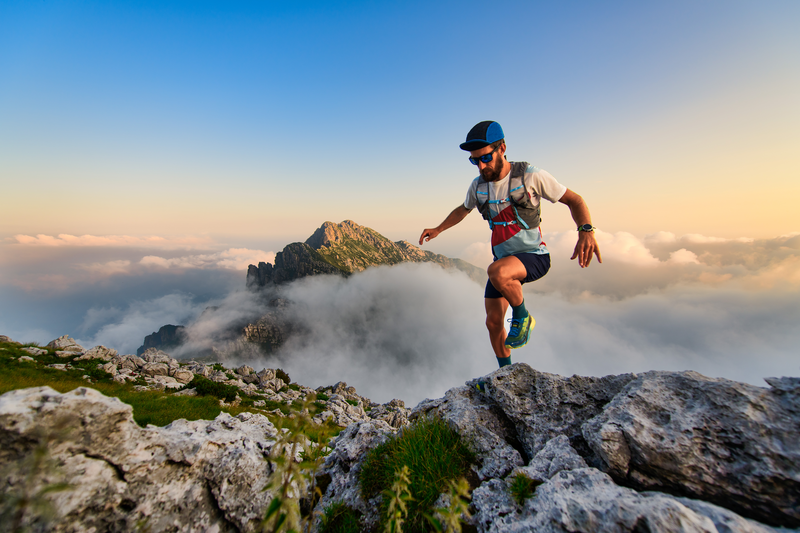
95% of researchers rate our articles as excellent or good
Learn more about the work of our research integrity team to safeguard the quality of each article we publish.
Find out more
REVIEW article
Front. Cardiovasc. Med. , 11 August 2021
Sec. Cardiovascular Therapeutics
Volume 8 - 2021 | https://doi.org/10.3389/fcvm.2021.695547
This article is part of the Research Topic Translating Artificial Intelligence into Clinical Use within Cardiology View all 19 articles
Heart failure is a major public health problem, which is associated with significant mortality, morbidity, and healthcare expenditures. A substantial amount of the morbidity is attributed to volume overload, for which loop diuretics are a mandatory treatment. However, the variability in response to diuretics and development of diuretic resistance adversely affect the clinical outcomes. Morevoer, there exists a marked intra- and inter-patient variability in response to diuretics that affects the clinical course and related adverse outcomes. In the present article, we review the mechanisms underlying the development of diuretic resistance. The role of the autonomic nervous system and chronobiology in the pathogenesis of congestive heart failure and response to therapy are also discussed. Establishing a novel model for overcoming diuretic resistance is presented based on a patient-tailored variability and chronotherapy-guided machine learning algorithm that comprises clinical, laboratory, and sensor-derived inputs, including inputs from pulmonary artery measurements. Inter- and intra-patient signatures of variabilities, alterations of biological clock, and autonomic nervous system responses are embedded into the algorithm; thus, it may enable a tailored dose regimen in a continuous manner that accommodates the highly dynamic complex system.
Heart failure (HF) is a staggering clinical and public health problem with high morbidity and mortality burden, affecting more than 6 million individuals in the United States where ~670,000 individuals are diagnosed with HF each year (1). Patients with HF are frequently hospitalized with HF exacerbation and have reduced quality of life and increased mortality rates. HF exacerbation is the leading cause of hospitalization among patients aged >65 years (2).
HF is associated with high rates of morbidity and reduced quality of life which are related to symptoms of volume overload resulting from sodium retention and volume overload. Loop diuretics are commopnly used for the teratment of volume overload resulting from HF. There is a marked inter and intra-patient variability that characterizes the response to diuretics at different stages. These variations may alter patients' clinical course and adversely affect their clinical outcomes (3, 4). A diminished response to loop diuretics is a well-recognized clinical challenge, which limits their clinical use (5, 6). Current methods for dose adjustment are somewhat arbitrary and do not take into consideration patients' resistance to diuretics (6). A continuous increase in the diuretic doses may further worsen diuretic resistance.
In the present article, we review the common mechanisms underlying the development of diuretic resistance and discuss current methods to overcome this devastating phenomenon. The role of the autonomic nervous system (ANS) and chronobiology in the pathogenesis of congestive heart failure (CHF) are discussed. The implementation of a patient-tailored variability and chronotherapy-guided algorithm for the treatment of these patients is presented as a potential strategy for alleviating drug resistance and improving their long-term beneficial effects.
The effects of loop diuretics on systemic and renal hemodynamics are driven by multiple factors, including the dose, route of administration, concomitant diseases, and medications and chronicity of their use (7). Furosemide is a potent prototypic loop diuretic that exerts its effect by binding to the translocation pocket at the extracellular surface of sodium-potassium-chloride symporters (NKCCs). It blocks ion transport directly by inhibiting NKCCs at the apical surface of the thick ascending loop of Henle (7, 8). The half-life of loop diuretics is generally shorter than typical dosing intervals of twice daily. Additionally, as they inhibit solute transport at only a single segment out of the numerous sodium-reabsorbing nephron sections, their impacts on extracellular fluid volume are multifaceted. Loop diuretics cause renal vasodilation through direct vascular dilation of the afferent arterioles and inhibition of the tubuloglomerular feedback (9).
Diuretic resistance is defined as the failure to achieve effective decongestion with low urine sodium concentration despite the use of appropriate or escalating diuretic doses (10). When diuretic resistance develops, the response to diretics is reduced before the achieving the goal of treatment, leading to poor prognosis. Resistance (or tolerance) to diuretic therapy can develop over time, making volume reduction in HF more challenging. There are two forms of diuretic tolerance, namely, short- and long-term resistance. Short-term tolerance occurs when the effect of the diuretic is weakened after the first dose. It may be prevented by reestablishing diuretic-induced loss of volume. Long-term tolerance is observed following administration of a loop diuretic for prolonged periods of time, which is associated with sodium reabsorption at the distal sites (10).
Multiple factors can explain non-responsiveness to diuretics, including inadequate doses, lack of adherence, advanced age, high sodium intake, impaired secretion into the tubule lumen, chronic kidney disease (CKD), gut edema, use of non-steroidal anti-inflammatory agents, hypoproteinemia, hypotension, nephrotic syndrome, reduced renal blood flow, and neurohormonal activation (7, 11).
Tolerance at the receptor- or post-receptor points may be associated with diuretic tolerance or resistance (11). In the circulation, loop diuretics are bound to various proteins (mainly albumin) and secreted into the tubules by the organic anion transporters (OAT1 and OAT2) located at the basolateral membrane and multidrug resistant protein-4 located at the apical membrane. As diuretics compete with chloride for binding to NKCC2, increased salt absorption in the proximal tubules limits the diuretic-sensitive transport (7).
In patients with HF, RAAS activation results in sodium retention. These patients may manifet with renal dysfunction assciated with additional activation of neurohormones. As HF progresses, persistent activation of these neurohormonal systems enhances sodium retention and contributes to the development of diuretic resistance (12–14). Worsening renal function in HF reduces the usefulness of loop diuretics by reducing their secretion into the renal tubules, a process mediated by increased organic ions competing for organic ion transporter binding (15).
Compensatory responses to diuretics may increase drug resistance. The diuretic-associated increase of the plasma renin activity promotes angiotensin II while blocking the tubuloglomerular feedback for increasing the glomerular filtration rate (16). Angiotensin II stimulates proximal tubular sodium reabsorption, thereby reducing distal sodium delivery and leading to diuretic resistance (11). Elevated plasma levels of both angiotensin II and aldosterone activate sodium transporters in the distal nephron (17). Post-diuretic sodium retaining may occur once the loop diuretic concentration drops below a certain threshold in the renal tubules. Sodium reabsorption is increased in the distal tubules and collecting ducts. This effect counters the loop diuretic effects (14). Adding of a second or third daily dose of loop diuretic may overcome this effect but may be associated with further long-term tolerance.
An additional contributing factor to diuretic resistance is the “braking phenomenon.” Chronic use of high dosages of loop diuretics leads to distal nephron remodeling, which involves hypertrophy and hyperplasia of the distal convoluted and connecting tubules and the collecting duct. This process augments the reabsorption capacity of the distal nephron and results in enhanced sodium reabsorption that negates the anticipated beneficial effects of these diuretics (18–20). This process is partially mediated by the RAAS (21) and activation of the sympathetic nervous system (SNS) (22). Adding a thiazide diuretic that blocks sodium reabsorption in the distal tubules This occurrence can overcome this effect; thus, it augments the net loss of sodium. Activation of baroreceptors in the arterial system triggers the SNS and RAAS, nephron remodeling, and extracellular volume depletion, thereby resulting in sodium retention (23). Extracellular volume contraction, which occurs with prolonged diuretic use in the setting of persistent congestion, contributes to the development of drug resistance (7).
Several pharmacologic and non-pharmacologic interventions are being used in an attempt to improve the diuretic response, including intravenous administration, increasing diuretic doses, and changing diuretic agents (7, 11). Several of these are directly or indirectly related to continuous daily administration of similar or higher dosages of diuretics. However, despite the prevalence of diuretic resistance, there remains a paucity of clinical trials to provide evidence on how to mitigate the resistance and guide therapy when patients develop this phenomenon (24).
Increasing dosages of loop diuretics may lead to a plateau in their effect, suggesting that raising doses beyond a “ceiling” will not further augment response (7). High diuretic doses, which stimulate the RAAS and SNS, are associated with worse outcomes, raising the possibility that they should be avoided in patients with severe decompensated HF (25). However, the Diuretic Optimization Strategies Evaluation study demonstrated that patients with acute decompensated HF on higher diuretic doses showed more favorable outcomes, including significant relief of dyspnea, reduction in weight, and higher net fluid loss, although they had a higher incidence of worsening renal function. Notably, the initial elevation in serum creatinine levels was associated with improved clinical outcomes (26).
Combined diuretic therapy is increasingly used to overcome high sodium retention. A common way to overcome diuretic resistance is to combine a diuretic with other medications including mineralocorticoid receptor antagonists, acetazolamide, or metolazone (6). The sequential nephron blockade by combining loop and thiazide diuretics in patients with inadequate response to optimal doses of loop diuretics was reportedly effective in some studies (27). Dopamine can improve renal perfusion and exert a diuretic effect (28). Hypertonic saline transiently increases the intravascular volume and improves sodium delivery to the tubules of the nephron (28). Additionally, metalazone (zaroxylin) in combination with loop diuretics or ultrafiltration is also being used in patients with resistance (29, 30).
The CardioMEMS HF System was designed for monitoring and measuring pulmonary artery (PA) pressure in patients with CHF. It transmits daily sensor readings from patients to their healthcare providers, allowing for personalized diuretic management in order to reduce the likelihood of hospitalization. In a retrospective analysis, PA pressure-guided management reduced HF hospitalization by 43% and mortality by 57% (31–33). The CHAMPION trial was a controlled, single-blind study of 550 patients with New York Heart Association class III HF and an HF hospitalization in the previous year. After 6 months of follow-up, the PA sensor-actively monitored patients who experienced an increased frequency of medication adjustments, higher dosages of diuretics, and diuretics intensification (34). Compared with the standard of care, its use was cost-effective, with an incremental cost-effectiveness ratio per quality-adjusted life year (31). However, dose adjusting is marginally arbitrary and does not consider patients developing resistance to diuretics.
Taken together, similar mechanisms are responsible for both the resistance and adaptation to diuretics. Several measures taken for overcoming diuretic resistance may further enhance the vicious cycle of inducing resistance and jeopardizing the clinical condition. Continuous administration on a regular basis of the same or higher dosages of diuretics is associated with a vicious cycle of actually augmenting the resistance.
Chronobiology describes the control of multiple biological functions by the circadian rhythm. Many cellular and physiological processes exhibit a circadian rhythm, oscillating approximately once in 24 h. These endogenous cycles enable the organism to optimally arrange its patterns of behavior in synchronization with the predictable changes in environmental conditions (35, 36). The central pacemaker is loated in the suprachiasmatic nuclei (SCN) and is synchronized with multiple peripheral clocks in various cells (37). In the cellular level, four genes—circadian locomotor output cycle kaput (clock), brain and muscle ARNT-like 1 (bmal1), period 1 and 2 (Per1,2), and Cryptochrome (Cry)—form a transcriptions-translation feedback loop that cycles approximately every 24 h, providing the periodicity of the cellular circadian rhythm.
The heart and blood vessels are characterized by marked daily oscillations in gene expression, metabolism, growth, and remodeling. The circadian clocks within the cardiomyocytes are linked to the regulation of myocardial function and metabolism (38). A proper response of the heart to its diurnal environment is mandatory for survival. It involves response to changes in workload, nutrients, neurohumoral stimuli, and metabolic alterations. Blood pressure (BP), heart rate (HR), coagulation activity, and endothelial function manifest in a day/night pattern (39).
Chronodisruption is an alteration of circadian rhythms associated with diverse diseases, including cardiovascular diseases and malignancy. Night-shift workers, who are exposed to artificial light disrupting the endogenous circadian rhythm, have a higher risk of all-cause and cardiovascular mortality (40). Obesity, high triglycerides, and low high-density lipoprotein cholesterol levels are more common in night-shift workers (41). Brachial artery endothelial function is determined as the reaction to reactive hyperemia (flow-mediated dilation [FMD]) and was irregular in residents following a 24-h shift, including night duty. A marked decrease in FMD was noted in physicians with a history of night-shift duty and in those reporting fewer sleeping hours during the shift (42).
Chronodisruption was described with acute and chronic cardiovascular events. Intramyocellular circadian clock and diurnal variations in fatty acid responsiveness were noted in the rat cardiomyocytes. Reversal of the 12-h/12-h light/dark (L/D) cycle led to re-entrainment of the circadian clock in the heart. Disruption of the circadian clock within the heart via the expression of a dominant negative CLOCK mutant lowered the promotion of myocardial fatty acid-responsive genes during fasting (43). Under pathologic conditions, the endogenous circadian phase corresponds to 10 a.m., the peak time for adverse cardiac events. Diurnal rhythm disruption after myocardial infarction (MI) hinders cardiac healing and exacerbates pathological cardiac remodeling. In a mouse model of MI, a short-term diurnal disruption adversely affected metabolism while altering innate immune responses shown by differences in cytokines, cardiac myeloperoxidase, and macrophage and neutrophil infiltration. Clock mutant mice showed changed infiltration after MI, which is linked to the innate immune responses required for scar formation and associated with reduced blood vessel formation in the infarct region, increased left ventricular dilation, and infarct expansion (44).
In an animal study, the likelihood of ventricular fibrillation (VF) was assessed by the time of the day during which acute coronary failure occurred. A coronary failure between 15.30 and 18.00 led to irreversible VF and death while modeling a similar condition from 11.00 to 15.00 was not associated with VF (45). In a study of 268 consecutive healthy subjects, FMD was diminished in early compared to late morning post-waking hours and predicted long-term cardiovascular events in healthy subjects with no known heart disease (46). FMD of the brachial artery analyzed three times a day (6:30 a.m., 11:30 a.m., and 9 p.m.) in patients with idiopathic dilated cardiomyopathy was lower during the day, manifesting reduction of the normal circadian variation in endothelial function (47).
A meta-analysis encompassing more than 2 million people, described a moderately increased risk for MI and ischemic stroke among shift workers (48). Another chronobiological characteristic of cardiovascular diseases is exhibited in the varying prevalence of adverse cardiovascular events throughout the day. MI, ischemic strokes, and sudden cardiac death (SCD) have higher incidences in the morning hours, corresponding with an increase in the sympathetic tone, peripheral arteries resistance, platelet aggregability, decreased parasympathetic tone, and endothelial function (39).
Disruption of the circadian rhythm contributes to the pathogenesis of HF (49). In HF, the dynamics are associated with an increased parameter of the scaling exponent of the inter-beat interval. In these patients, the peak in the scaling exponent at the circadian phase corresponds to the time of increased heart vulnerability. The endogenous circadian-mediated effects on cardiac regulation are linked to a day/night pattern of adverse cardiac events (50). The heart rate variability (HRV) and mean arterial pressure (MAP) variability were decreased in rats with HF; this decrease was accompanied by disturbances in the normal circadian pattern of HR and BP (51). Differences were demonstrated in the circadian outcome of angiotensin converting enzyme inhibitors (ACEis) on BP in rats with HF (52). The circadian clock contributes to regulation of the mitochondrial metabolism and maintaining the cardiac function. Ablation of the Bmal1circadian clock gene is associated with mitochondrial defects in the heart including morphological changes and reduced enzymatic activities within the respiratory complex, reduced expression of cardiac genes associated with the tricarboxylic acid cycle, fatty acid oxidative pathway, and mitochondrial respiratory chain. These changes were associated with the development of HF. Similar changes were noted in mice exposed to the chronic reversal of the L/D cycle and disrupted circadian rhythmicity (53). In a study of 1,401 asymptomatic subjects in the Cardiovascular Health Study with interpretable 24-h baseline Holter recordings, irregular HRV parameters were related with CHF. Combined with higher incidence of PVCs, HRV enhanced the predictive power of the Health ABC score (54). Autonomic dysfunction quantified HRV parameters characterized subjects with no benefits from cardiac resynchronization treatment, suggesting that pre-implant HRV study helps in improving qualifications for this treatment. In a study of 719 subjects with normal sinus rhythm enrolled in MADIT-CRT (Multicenter Automatic Defibrillator Implantation Trial-Cardiac Resynchronization Therapy), followed for over 3 years, 124 patients reached the primary end point of heart failure or death and 47 died. In multivariate analysis, low SDNN or low VLF was related to a significantly increased risk of HF and mortality (55).
A blunt BP circadian rhythm in HF was documented in humans and linked to the disappearance of circadian variation in atrial natriuretic peptide (56) and in normotensive patients, with the degree of left ventricle function impairment (57). A narrower decrease in the nocturnal systolic BP correlated with a lower ejection fraction. Respiratory function also varies between the light and dark periods, and these L/D variations were shown in HF. In an animal model, HF was associated with blunted L/D differences in resting and chemoreflex breathing, suggesting that the HF disrupts cardiovascular and respiratory circadian rhythms. Cyclic variation of heart rate (CVHR) is linked with sleep-disordered breathing reflecting cardiac autonomic responses to apneic/hypoxic stress. Blunted CVHR in a night-time Holter ECG predicts increased mortality risk in patients with post-MI, end-stage renal disease, and HF. In a study of 717 patients after MI, decreased cyclic variation amplitude was a predictor of mortality (58). Diurnal variations in respiration and arterial BP were abolished in HF with reduced ejection fraction (HFrEF) (59). Circadian oscillations in calcineurin-dependent activities in the left ventricle of normal mice showed that calcineurin-dependent transcript levels and nuclear occupancy of the nuclear factor of activated T-cells fluctuate over the course of a day, peaking in the morning when mice enter a period of rest (60).
SCD, a prominent cause of death in patients with HF, exhibits diurnal variation and is linked to irregularities in the duration (short or long QT syndromes and HF) or pattern (Brugada's syndrome) of myocardial repolarization. Cardiac ion-channel expression and QT-interval duration, which reflects myocardial repolarization, manifest endogenous circadian rhythmicity controlled by a clock-dependent oscillator, Krüppel-like factor 15 (Klf15). Klf15 transcriptionally regulates a rhythmic expression of the Kv channel-interacting protein 2, which is necessary for the transient outward potassium current (61).
Overall, these data suggest a possible association between HF and alteration in the normal circadian rhythms, implying that novel treatment strategies for HF must take into consideration the timing of treatment administration, adjusting it to the individualized circadian rhythm of patients.
The ANS underlie some mechanisms associated with CHF. The sympathetic nerve fibers travel alongside the coronary arteries and terminate in the sub-epicardium. Its activation results in accelerated HR, augmented contractility, and increased afterload. In contrast, the parasympathetic system, which branches from the vagal nerve to the sub-endocardium, slows the HR (62). Chronic sympathetic overactivation was described in HF and diuretic resistance and is related to components of the metabolic syndrome, such as obesity, dyslipidemia, BP elevation, and reduced fasting glucose with hyperinsulinemia (63, 64).
Figure 1 describes the interfaces between the circadian rhythm and the autonomic nervous system, contributing to the pathogenesis of congestive heart failure.
Figure 1. The interfaces between the circadian rhythm and the autonomic nervous system, contributing to the pathogenesis of congestive heart failure.
Dietary sodium affects the circadian oscillators downstream of the master light-dark-adjusted pacemaker in the SCN. The chronobiology of renin activity (RA), BP, and fractional excretion of renal sodium (UFENa) and potassium (UFEK) handling in relation to meal timing was studied in dogs. Data showed that RA, UFENa, UFEK, diastolic and systolic BP fluctuate with a circadian periodicity in dogs fed at 07:00 h with a regular diet. Modeling using a fixed 24-h period reflected the variations of UFEK, RA, and BP, and cyclic changes in UFENa suggested a postprandial sodium excretion and a monotonous decay. A delay in the feeding schedule by 6- or 12-h was associated with a shift of comparable magnitude in the rhythm of these biomarkers (65).
The diurnal rhythm in the standard deviation of the averages of normal R-R intervals (SDANN) and LF/HIF ratio is disrupted in MI. L/D differences in the LF/HIF ratio change from negative to positive values along with a decrease in SDANN, HR, LF/HIF ratio in the dark phase, and elevated plasma norepinephrine levels (66). These data suggest that the timing of the disturbance of diurnal rhythm in SDANN and the LF/HIF ratio are different from those in HR and in the plasma norepinephrine levels (67). In patients with HF, arterial underfilling caused by decreased cardiac output or peripheral arterial vasodilation activates the SNS, RAAS, and non-osmotic vasopressin release (68). Excess activation of the SNS, which aims to maintain cardiac output, has deleterious effects on the heart in the long run. Activation of the SNS in HF results from interactions between the appropriate compensatory reflexes to pathologic excitatory stimuli associated with the depressed systolic function and additional comorbidities. These interactions elicit adrenergic activation in excess to homeostatic requirements (62). To counteract the increased SNS activation, vagal nerve stimulation was suggested as a novel strategy. However, although profound anti-arrhythmic effects were exerted and cardiac function in HF models was improved (69), this method failed to reduce mortality and disease progression in HFrEF patients in the INOVATE-HF trail (70).
The circadian pattern of HR and BP, which are affected by the ANS, is disrupted in patients with HF. In an animal model of MI, the association between vagal nerve activity and stellate ganglion nerve activity was documented. A circadian variation following MI reached a peak at a time when sympathetic activation was the uppermost and vagal activity was the lowest (71). The circadian and short-term regulation of BP and HR were shown to be preserved in young, non-failing beta1-transgenic mice, suggesting that the loss of blood pressure and HRV in HF cannot be attributed to over activity of the sympathetic system. However, it reveals loss of adrenergic responsiveness to changes in the activity of the ANS (72). Angiotensin II participates in abnormal autonomic cardiovascular control, which occurs during HF progression. In a model of post-MI, HR increased with the severity of HF, loss of circadian HR, MAP, and BRS rhythms were noted, along with an upregulation in the central angiotensin II type 1 receptors (AT1R) and gp91 proteins in the brainstem. Losartan reduced AT1R expression in daytime but failed to restore circadian variability (73). HF is associated with increased LF constituents of diastolic BP variability, an index of sympathetic tone, during the awakening period compared to during the sleeping period. Amiodarone suppressed this transient increase in LF power during the awakening period (74). Circadian changes in ANS function were also observed at the molecular level. Rats with coronary artery ligation-induced HF showed a shift in the adrenoreceptor beta 1:beta 2 ratio toward beta 2 and decreased beta 1 adrenergic stimulation by adenylyl cyclase. These findings were in association with disturbed circadian patterns in BP and HR (75).
HRV is a method to visualize the link between ANS and circadian dysfunction to the development of HF. It measures irregularity of intervals between adjacent heartbeats, representing a neurocardiac function and ANS regulation state (76). Normal HRV presents a circadian pattern with parasympathetic parameters peaking at night-time and shows sleep-stage dependence (77, 78). HRV alteration is associated with numerous disease states and correlates with cardiovascular and all-cause mortality, mainly in patients with established cardiovascular disease (79). A decrease in the HRV was associated with increased mortality and was a better predictor of death than conventional clinical management (80). HRV parameters are altered in both animal models of HF and in humans. In a study determining autonomic parameters 3 and 7 weeks after left coronary artery ligation in rats, LF and HF parameters of HRV were reduced in CHF 3 weeks after infarction, in addition to the prolonged loss of baroreflex sensitivity (BRS). Correlation between HRV and MAP variability in the LF domain was reduced in HF (81). HRV and BRS are severely affected in patients with ADHF and improve with clinical stabilization (77–80, 82). In addition, HRV parameters are abnormal in stable patients with HF. HRV parameters were linked to the incidence of CHF in 1,401 asymptomatic, older adults (54). Increased sympathetic activity in CHF is associated with obstructive and central sleep apnea (SA). In a study of patients with CHF and SA, a lowered cardiac autonomic modulation across the 24-h period was documented. The RR variance, LF, HIF parameters of HRV, and spontaneous BRS were reduced in subjects with SA. The HIF power, a marker of vagal activity, elevated during sleep in patients without SA, whereas it did not alter across the 24-h period in subjects with SA. In a study of 167 patients with CHF with central sleep apnea (CSA) and obstructive sleep apnea (OSA), morning premature ventricular contractions and non-sustained VT were more frequent in CSA. CSA was linked to the occurrence of VT irrespective of sleep/wake status (83–85).
Patients with CHF manifested with lower RR interval complexity and loss of its circadian rhythm, along with reduced frequency-domain RR interval variability and its irregular circadian rhythm (86). The circadian variability of RR and QT intervals is altered in CHF due to neurohumoral activation and functional and structural remodeling of the heart. In a study of 121 patients with HF in the sinus rhythm, all subjects showed marked circadian rhythms in the QT, RR, and QTc intervals and the QT/RR slope by cosine-curve fitting. The increased HR was associated with longer QT interval, and steeper QT/RR slope, lowered circadian variability of QT interval and later maximum RR interval were related to increased cardiac mortality (87).
As sympathetic activation underlies the pathogenesis of HF and diuretic resistance, the selective reduction of the renal afferent and sympathetic efferent nerves was proposed to improve diuretic resistance, CHF, and cardio renal disorders (63). Treatments directed against neurohormonal compensatory mechanisms, such as losartan, spironolactone, and beta-blockers, resulted in an improved HRV profile (88, 89). The favorable effect of spironolactone on the ANS showed a circadian pattern and was limited to the morning hours. Patients with decompensated HF on beta-blockers had a higher power in all HRV frequency bands but were significantly associated with low circulating norepinephrine levels (90). Altering effect of these drugs on the circadian pattern of HRV is known in ischemic heart disease (91).
Implantation of a left ventricular assist device (LVAD) can increase the reduced HRV, which correlates with the degree of ventricular dysfunction. HRV was lower in LVAD patients early after device implantation, suggesting that cardiac dysautonomia is still evident in the first 2 months (92). The algorithm provided by LVADs shows circadian variation. In a study of 18 patients with fixed rotation speed continuous-flow LVAD support, the motor current was lower during the night and higher during the day (93).
Despite these supportive data, other studies failed to support circadian variation in patients with HF. Analysis of data from the PRAISE trial showed that SCD in HF did not show an a.m. peak, signifying that circadian sympathetic activation did not affect these events (94). A study of eight patients with mild-to-moderate HF showed that circadian variation of the cardiac autonomic nervous activity (CANA) was preserved. The CANA was affected by patient position and the time of day. Circadian variation was noted in the recumbent position. The HIF power was lower and LF/HIF was higher in the morning than at night (95).
Overall, the currently available data support the role of the ANS in regulating the heart function under circadian rhythm in health and disease. As many of the effective treatments in HF aim to antagonize the neurohormonal compensatory mechanisms, a personalized approach based on understanding the ANS characteristics and circadian rhythm changes may confer clinical benefits.
Chronobiology impacts the effectiveness and toxicity of drugs and is linked to the pharmacodynamics and pharmacokinetics of medications. Chronotherapy is based on linking the absorption, metabolism, and elimination of medications to the circadian patterns (96) and involves the use of a specific drug at the most optimal time, pattern, and dose possible, with the goal of maximizing efficacy and minimizing toxicity. The ideal timing is based on circadian-rhythm-dependent biological systems, which alter drugs bioavailability, pharmacokinetics, and pharmacodynamics (97, 98). Identification of a rhythmic marker for selecting the dosing time has been suggested to improve the response to drugs (99).
Table 1 summarizes examples of the linkage between administration time and effect in cardiovascular drugs.
Chronotherapeutic benefits of antihypertensive medications have been demonstrated (107). A study of 19,084 hypertensive patients treated with BP-lowering drugs at bedtime, as opposed to upon wakening, reported improved BP control and cardiovascular outcomes, including MI, HF, and cardiovascular death (101). Low-dose aspirin administered at bedtime compared with during the awakening hours can improve platelet inhibition during the critical morning hours (102). Several studies have supported the chronotherapeutic attributes of loop diuretics. Administration of furosemide to rats at 10 a.m. showed greater urine volume and urinary excretion of sodium and furosemide than that at 10 p.m. A correlation between the urinary output of furosemide and urinary volume of sodium, representing the known mechanism of furosemide, has been described (104).
Pretreatment with clorgyline, a monoamine-oxidase inhibitor that alters circadian variations in the SNS, diminished the variability in furosemide effect (108). Pretreatment with propranolol also caused a loss of variability, while atenolol did not cause the same effect, suggesting the involvement of the beta2-adrenergic receptor (109). In two additional studies, continuous infusion of norepinephrine and denervation of the kidneys caused the same influence of diminished variability (110, 111). In humans, the efficacy of furosemide was improved by bedtime dosing as compared to dosing upon awakening. The fraction of patients with controlled ambulatory BP following therapy was also increased after bedtime treatment (105).
Chronotherapy can control the RAAS overactivation in HF. The efficacy of ACEi in HF depends on the time of administration. ACEis were more effective in preventing heart remodeling when administered during sleep (103). Dosing of ACEi in the evening, at an inactive period, was associated with an improved protective effect against heart hypertrophy in hypertensive rats and also proposed to reduce the severity of the drug-related dry cough of hypertensive subjects treated in the morning (100). Bedtime administration of valsartan normalizes the circadian rhythm and protects the kidneys and heart in patients with CKD (106). An integrated pharmacokinetic-pharmacodynamics model was designed based on the kinetics of the drug and time-varying changes of RAAS, showing that the optimal efficacy of ACEis is achieved with bedtime dosing (112).
Melatonin determines the circadian physiology, and its circulating levels vary on a daily cycle, allowing the regulation of circadian rhythms of multiple biological systems. It was shown to exert cardioprotective effects impacting the clinical course of HF. In a study of 32 patients with CHF, nocturnal melatonin secretion negatively associated with N-terminal pro-brain natriuretic peptide (NT-proBNP). Higher melatonin levels were noted at 02:00 than at 07:00 (113).
Rev-erb alpha, which is the nuclear receptor 1D1, is a circadian rhythm regulator that controls inflammation and glucose and lipid metabolism. Administration of SR9009, a Rev-erb, in a mouse model of MI and HF improved the survival rates and reduced the left ventricular function. These effects were associated with reduced BNP levels and reduced expression of inflammatory-related molecules, such as matrix metallopeptidase 9, Ly6g, Cd11b, IL-6, Mcp1, and phosphorylated NF-kappaB p65, phosphorylated extracellular signal-regulated ligand, and phosphorylated p38. This treatment also reduced neutrophil and pro-inflammatory macrophage infiltration (114). SR9009 improved the long-term cardiac repair post-myocardial ischemia-reperfusion injury in animals. A single therapy decreased cardiac NLRP3 inflammasome, fibroblast, and immunocyte infiltration, in turn augmenting the MI healing process (115).
Taken together, these studies suggest that chronotherapy, including the targeting of ANS activation, exerts a beneficial effect on HF and diuretic resistance-related parameters.
Variability is inherent to biological systems and has been proposed to be part of the normal function of cells and organs (116–119). Variability at the genome and cellular level (118, 120), HRV (121), respiratory variability (122, 123), and gate variability (124) are some examples. The loss or alteration in the physiologic variability is associated with disease states and poor prognoses (125–127). At the cellular level, the cell is packed with a non-uniform spreading of macromolecules that interact specifically and nonspecifically with a drug. This leads to a high degree of variability between individual cells in the response to drugs (128). Many of these variabilities do not follow specific patterns or rules and are characterized by marked inherent intra- and inter-patients unpredictability.
Variability in the heart function was the basis for the development of the heart-slowing medication ivabradine. The sinus node is the central cardiac pacemaker, and its function is associated with several ionic transporter circuits that can produce a rhythm. If one apparatus fails, another one can take over the task. Eliminating a transporter that could carry as much as 80% of the ionic current required for producing the rhythm alters the incidence by only around 10–15%, and this is due to a substitution mechanism. This supports the concept of a high degree of molecular-level variations as a protective mechanism (129, 130).
High degrees of both inter- and intra-patient variability are described for the response to many drugs. There are marked intra- and inter-patient variabilities in drug pharmacodynamics associated with the loss of an effective response to drugs (119, 131–136). For example, high tacrolimus intra-patient variability was associated with graft rejection (134), and intra-patient variability in many antiepileptic circulatory levels in stable patients was described with observed differences of tens of percentages in the serum levels (137).
Regular dosing regimens for diuretics are not compatible with physiological variability and may further increase drug resistance. Taking a constant dose of diuretics on a regular basis, or continuously increasing the dose, may increase the serum drug levels reaching a peak level and its subsequent gradual decrease. This process is observed to repeat on a daily basis. It is a monotonic cyclic pattern associated with adaptation to the effect and results in the partial or complete loss of the response to the diuretic (12–14). Part of the loss of the effect is associated with augmentation of the compensatory responses to diuretics as a result of a continous increase in doses, which further reduces their effect. For several drugs, a regular dosing regimen or continuous increase of a dose was proposed to contribute to drug resistance, compared with the irregular consumption of the same dose or with altering the daily dose (117, 138).
A model for heartbeat was developed built on a noise titration assay, which provides a time-resolved and quantitative degree of the chaos. It uses the HIF and LF parameters of HRV for determining whether they reflect stochastic or chaotic phenomenon (139). Noise titration of the running short-segment Holter tachograms from healthy people showed circadian sleep/wake-dependent heartbeat chaos that linked with the HF parameter (respiratory sinus arrhythmia). In contrast, in patients with HF, a near-regular heartbeat was non-chaotic and resulted in transient chaotic rhythms. HRV alterations in HF were accompanied by little changes in approximate entropy, a measure of signal irregularity, which reflects an autonomic, cardiac, respiratory, and circadian/sleep-wake changes (139).
A model using time irreversibility analysis was developed for the analysis of the short heart period sequences derived from 24 h Holter recordings. Irreversible dynamics over short time scales were noted, while the irreversibility of longer time scales was marginal. In healthy subjects, the percentage of irreversible dynamics was higher during the daytime than during the night-time, suggesting augmented non-linear dynamics during the daytime. In healthy subjects, the non-linear behavior reflected that bradycardic ones run shorter than the tachycardic ones during the daytime. In contrast, patients with HF demonstrated an increased percentage of irreversible series along with a reverse pattern, showing the tachycardic ones run shorter than the bradycardic ones (140).
Using treatment regimens based on aperiodic intervals and at irregular strengths has been suggested to improve response to diuretics. For maximum benefits, irregular, pulsed, or multiple intervals-based administration of a chronic drug at continually changing dosage strengths may improve the overall effect, thereby reducing the likelihood of drug resistance (117, 119, 141). Manipulating the conditions of living organs using rhythmic administration of altered feeding schedules or several drugs appears successful (99, 142).
Establishing a novel model for overcoming diuretic resistance was proposed based on a closed loop system, which comprises clinical, laboratory, and sensors-derived inputs, including PA pressure sensors. Alteration of rhythmicity may adversely affect homeostatic regulation and lead to deleterious effects. These changes in therapeutic regimens should take into account the inter- and intra-patient variability, alterations of the biological clock, and ANS responses.
This model has been developed at four levels. In the first step, random alterations in the dosing and timing of administration are introduced into the regimens. The implementation of irregular regimens is expected to improve the response to diuretics by lowering the harmful effects of compensatory mechanisms (117, 138, 143, 144). The physician is asked to register the patient and to enter into the systme the ranges for the dosages and for the times of administration. Patients receive daily reminders for taking the drug. The algorithm randomly alters the dosages and times of administration on a daily basis (143, 144).
In the second step, an increase in the efficiency of diuretics is achieved by administering drugs that are at times the best tolerated by synchronizing medication concentrations to rhythms in disease activity. Technologies for drug delivery precisely in a time-modulated mode by bedside or ambulatory pumps can improve both the safety and efficacy of the chronic use of diuretics (99).
In the third stage, machine learning is introduced to regulate the variability and chronotherapy-based regimens using closed loop systems that control the dosing and timing in an individualized manner. This enables the overcoming of intra- and inter-patient variability, which prevents the “single bullet for all” concept from being applicable to the majority of patients. This concept is being introduced for overcoming drug resistance in several chronic diseases (142, 143, 145–156). In the last step, signatures based on parameters relevant to the pathogenesis of HF, mechanisms of action, and the cardio renal axis are introduced in a personalized approach for tailoring the appropriate dosing in a continuous and consistent manner (143, 144) (Figure 2).
Figure 2. A suggested scheme of the closed loop machine learning system for improving the response to diurectis in patients with HF. Inputs from various sensors are being incorporated in a dynamic manner for determining the timings of administration and dosages in a personalized approach. In a dynamic changing system with multiple alternating parameters, a continuously changing dosing regimen is generated based on the individualized-variability pattern that comprises data from the ANS (e.g., HRV), heart, and kidney. Cardiac, renal, and ANS, along with numerous other physiological and biological processes, exhibit intrinsic randomness. These variations in their activity are also contributed from the generalized circadian rhythm and from intrinsic biological rhythms. Randomized treatment administration within the preset limits augmented with personalized signature from the clinical status, echocardiographic, HRV, pulmonary artery pressure monitoring, biomarkers, and more can improve outcomes of the diuretic treatment. In addition, randomization might prevent renal adaptivity involved in the development of diuretic resistance. ANS, autonomic nervous system; HRV, heart rate variability.
In summary, CHF remains a major clinical problem with an enormous morbidity and mortality burden. Diuretic resistance is a major obastacle for the effective long-term treatment of HF with considerable inter- and intra-patient variability, in addition to the implication of both the ANS and chronobiology in the pathogenesis and progression of HF. A personalized machine learing algorithm, which comprises continously changing parameters derived from clinical, laboratory, and sensors-derived inputs, including inputs from PA measurements, is suggested as an effective tool for improving the resposne to duretics. Ongoing trials will determine if these platforms are efficacious in reducing the adverse clinical outcomes and improving long-term prognoses.
All authors listed have made a substantial, direct and intellectual contribution to the work, and approved it for publication.
YI was the founder of Oberon Sciences.
The remaining authors declare that the research was conducted in the absence of any commercial or financial relationships that could be construed as a potential conflict of interest.
All claims expressed in this article are solely those of the authors and do not necessarily represent those of their affiliated organizations, or those of the publisher, the editors and the reviewers. Any product that may be evaluated in this article, or claim that may be made by its manufacturer, is not guaranteed or endorsed by the publisher.
CHF, congestive heart failure; HF, heart failure; ANS, autonomic nervous system; NKCC, sodium-potassium-chloride symporter; RASS, renin-angiotensin-aldosterone system; CKD, chronic kidney disease; OAT, organic anion transporter; SNS, sympathetic nervous system; ADHF, acute decompensated heart failure; PA, pulmonary artery; QALY, quality-adjusted life years; SCN, suprachiasmatic nucleus; CLOCK, circadian locomotor output cycle kaput; BMAL1, brain and muscle ARNT-like 1; Per, period; Cry, cryptochrome; BP, blood pressure; HR, heart rate; L/D, light/dark; HFrEF, heart failure with reduced ejection fraction; MI, myocardial infarction; SCD, sudden cardiac death; HRV, heart rate variability; MAP, mean arterial pressure; ACEi, angiotensin converting enzyme inhibitors; Klf15, kruppel-like factor 15; SNS, sympathetic nervous system; LF, low frequency; HIF, high frequency; BRS, baroreflex sensitivity; OSA, obstructive sleep apnea; CVHR, cyclic variation of heart rate; FMD, flow-mediated dilation; SA, sleep apnea; LVAD, left ventricular assist device; SDANN, standard deviation of averages of normal R-R intervals; AT1R, angiotensin II type 1 receptor; RA, renin activity; Una, fe, fractional excretion of sodium in the urine; UK, fe, fractional excretion of potassium in the urine; CANA, cardiac autonomous nervous activity; CSA, central sleep apnea.
1. Tran HA, Lin F, Greenberg BH. Potential new drug treatments for congestive heart failure. Expert Opin Investig Drugs. (2016) 25:811–26. doi: 10.1080/13543784.2016.1181749
2. Chioncel O, Lainscak M, Seferovic PM, Anker SD, Crespo-Leiro MG, Harjola VP, et al. Epidemiology and one-year outcomes in patients with chronic heart failure and preserved, mid-range and reduced ejection fraction: an analysis of the ESC heart failure long-term registry. Eur J Heart Fail. (2017) 19:1574–85. doi: 10.1002/ejhf.813
3. Zepeda P, Rain C, Sepulveda P. Continuous infusion or bolus injection of loop diuretics for congestive heart failure? Medwave. (2016) 16(Suppl. 2):e6426. doi: 10.5867/medwave.2016.6426
4. Graziani G, Pini D, Oldani S, Cucchiari D, Podesta MA, Badalamenti S. Renal dysfunction in acute congestive heart failure: a common problem for cardiologists and nephrologists. Heart Fail Rev. (2014) 19:699–708. doi: 10.1007/s10741-013-9416-5
5. Wilcox CS, Testani JM, Pitt B. Pathophysiology of diuretic resistance and its implications for the management of chronic heart failure. Hypertension. (2020) 76:1045–54. doi: 10.1161/HYPERTENSIONAHA.120.15205
6. Felker GM, Ellison DH, Mullens W, Cox ZL, Testani JM. Diuretic therapy for patients with heart failure: JACC state-of-the-art review. J Am Coll Cardiol. (2020) 75:1178–95. doi: 10.1016/j.jacc.2019.12.059
7. Ellison DH, Felker GM. Diuretic treatment in heart failure. N Engl J Med. (2017) 377:1964–75. doi: 10.1056/NEJMra1703100
8. Somasekharan S, Tanis J, Forbush B. Loop diuretic and ion-binding residues revealed by scanning mutagenesis of transmembrane helix 3 (TM3) of Na-K-Cl cotransporter (NKCC1). J Biol Chem. (2012) 287:17308–17. doi: 10.1074/jbc.M112.356014
9. Oppermann M, Hansen PB, Castrop H, Schnermann J. Vasodilatation of afferent arterioles and paradoxical increase of renal vascular resistance by furosemide in mice. Am J Physiol Renal Physiol. (2007) 293:F279–87. doi: 10.1152/ajprenal.00073.2007
10. Jardim SI, Ramos dos santos L, Araujo I, Marques F, Branco P, Gaspar A, Fonseca C. A 2018 overview of diuretic resistance in heart failure. Rev Port Cardiol. (2018) 37:935–45. doi: 10.1016/j.repc.2018.03.014
11. Hoorn EJ, Ellison DH. Diuretic resistance. Am J Kidney Dis. (2017) 69:136–42. doi: 10.1053/j.ajkd.2016.08.027
12. Robinette B. Strategies to overcome diuretic resistance in congestive HF. Cardiology Today (2015).
13. Ter Maaten JM, Rao VS, Hanberg JS, Perry Wilson F, Bellumkonda L, Assefa M, et al. Renal tubular resistance is the primary driver for loop diuretic resistance in acute heart failure. Eur J Heart Fail. (2017) 19:1014–22. doi: 10.1002/ejhf.757
14. Shah N, Madanieh R, Alkan M, Dogar MU, Kosmas CE, Vittorio TJ. A perspective on diuretic resistance in chronic congestive heart failure. Ther Adv Cardiovasc Dis. (2017) 11:271–8. doi: 10.1177/1753944717718717
15. Oh SW, Han SY. Loop diuretics in clinical practice. Electrolyte Blood Press. (2015) 13:17–21. doi: 10.5049/EBP.2015.13.1.17
16. Harrison-Bernard LM. The renal renin-angiotensin system. Adv Physiol Educ. (2009) 33:270–4. doi: 10.1152/advan.00049.2009
17. Seva Pessoa B, Van der Lubbe N, Verdonk K, Roks AJ, Hoorn EJ, Danser AH. Key developments in renin-angiotensin-aldosterone system inhibition. Nat Rev Nephrol. (2013) 9:26–36. doi: 10.1038/nrneph.2012.249
18. De Bruyne LK. Mechanisms and management of diuretic resistance in congestive heart failure. Postgrad Med J. (2003) 79:268–71. doi: 10.1136/pmj.79.931.268
20. Kaissling B, Bachmann S, Kriz W. Structural adaptation of the distal convoluted tubule to prolonged furosemide treatment. Am J Physiol. (1985) 248:F374–81. doi: 10.1152/ajprenal.1985.248.3.F374
21. Abdallah JG, Schrier RW, Edelstein C, Jennings SD, Wyse B, Ellison DH. Loop diuretic infusion increases thiazide-sensitive na(+)/Cl(-)-cotransporter abundance: role of aldosterone. J Am Soc Nephrol. (2001) 12:1335–41. doi: 10.1681/ASN.V1271335
22. Ellison DH. The physiologic basis of diuretic synergism: its role in treating diuretic resistance. Ann Intern Med. (1991) 114:886–94. doi: 10.7326/0003-4819-114-10-886
23. Gordan R, Gwathmey JK, Xie LH. Autonomic and endocrine control of cardiovascular function. World J Cardiol. (2015) 7:204–14. doi: 10.4330/wjc.v7.i4.204
24. Bowman BN, Nawarskas JJ, Anderson JR. Treating diuretic resistance: an overview. Cardiol Rev. (2016) 24:256–60. doi: 10.1097/CRD.0000000000000116
25. Felker GM, O'Connor CM, Braunwald E, Heart failure clinical research network Investigators. Loop diuretics in acute decompensated heart failure: necessary? Evil? A necessary evil? Circ Heart Fail. (2009) 2:56–62. doi: 10.1161/CIRCHEARTFAILURE.108.821785
26. Brisco MA, Zile MR, Hanberg JS, Wilson FP, Parikh CR, Coca SG, et al. Relevance of changes in serum creatinine during a heart failure trial of decongestive strategies: insights from the DOSE trial. J Card Fail. (2016) 22:753–60. doi: 10.1016/j.cardfail.2016.06.423
27. Jentzer JC, DeWald TA, Hernandez AF. Combination of loop diuretics with thiazide-type diuretics in heart failure. J Am Coll Cardiol. (2010) 56:1527–34. doi: 10.1016/j.jacc.2010.06.034
28. Verbrugge FH. Editor's choice-diuretic resistance in acute heart failure. Eur Heart J Acute Cardiovasc Care. (2018) 7:379–89. doi: 10.1177/2048872618768488
29. Rosenberg J, Gustafsson F, Galatius S, Hildebrandt PR. Combination therapy with metolazone and loop diuretics in outpatients with refractory heart failure: an observational study and review of the literature. Cardiovasc Drugs Ther. (2005) 19:301–6. doi: 10.1007/s10557-005-3350-2
30. Shi X, Bao J, Zhang H, Wang H, Li L, Zhang Y. Patients with high-dose diuretics should get ultrafiltration in the management of decompensated heart failure: a meta-analysis. Heart Fail Rev. (2019) 24:927–40. doi: 10.1007/s10741-019-09812-2
31. Schmier JK, Ong KL, Fonarow GC. Cost-effectiveness of remote cardiac monitoring with the cardioMEMS heart failure system. Clin Cardiol. (2017) 40:430–6. doi: 10.1002/clc.22696
32. Adamson PB, Abraham WT, Stevenson LW, Desai AS, Lindenfeld J, Bourge RC, et al. Pulmonary artery pressure-Guided heart failure management reduces 30-day readmissions. Circ Heart Fail. (2016) 9:e002600. doi: 10.1161/CIRCHEARTFAILURE.115.002600
33. Benza RL, Raina A, Abraham WT, Adamson PB, Lindenfeld J, Miller AB, et al. Pulmonary hypertension related to left heart disease: insight from a wireless implantable hemodynamic monitor. J Heart Lung Transplant. (2015) 34:329–7. doi: 10.1016/j.healun.2014.04.014
34. Costanzo MR, Stevenson LW, Adamson PB, Desai AS, Heywood JT, Bourge RC, et al. Interventions linked to decreased heart failure hospitalizations during ambulatory pulmonary artery pressure monitoring. JACC Heart Fail. (2016) 4:333–44. doi: 10.1016/j.jchf.2015.11.011
35. Morris CJ, Aeschbach D, Scheer FA. Circadian system, sleep and endocrinology. Mol Cell Endocrinol. (2012) 349:91–104. doi: 10.1016/j.mce.2011.09.003
36. Panda S. Circadian physiology of metabolism. Science. (2016) 354:1008–15. doi: 10.1126/science.aah4967
37. Honma S. The mammalian circadian system: a hierarchical multi-oscillator structure for generating circadian rhythm. J Physiol Sci. (2018) 68:207–19. doi: 10.1007/s12576-018-0597-5
38. Durgan DJ, Young ME. Linking the cardiomyocyte circadian clock to myocardial metabolism. Cardiovasc Drugs Ther. (2008) 22:115–24. doi: 10.1007/s10557-008-6086-y
39. Thosar SS, Butler MP, Shea SA. Role of the circadian system in cardiovascular disease. J Clin Invest. (2018) 128:2157–67. doi: 10.1172/JCI80590
40. Gu F, Han J, Laden F, Pan A, Caporaso NE, Stampfer MJ, et al. Total and cause-specific mortality of U.S. nurses working rotating night shifts. Am J Prev Med. (2015) 48:241–52. doi: 10.1016/j.amepre.2014.10.018
41. Karlsson B, Knutsson A, Lindahl B. Is there an association between shift work and having a metabolic syndrome? Results from a population based study of 27,485 people. Occup Environ Med. (2001) 58:747–52. doi: 10.1136/oem.58.11.747
42. Amir O, Alroy S, Schliamser JE, Asmir I, Shiran A, Flugelman MY, et al. Brachial artery endothelial function in residents and fellows working night shifts. Am J Cardiol. (2004) 93:947–9. doi: 10.1016/j.amjcard.2003.12.032
43. Durgan DJ, Trexler NA, Egbejimi O, McElfresh TA, Suk HY, Petterson LE, et al. The circadian clock within the cardiomyocyte is essential for responsiveness of the heart to fatty acids. J Biol Chem. (2006) 281:24254–69. doi: 10.1074/jbc.M601704200
44. Alibhai FJ, Tsimakouridze EV, Chinnappareddy N, Wright DC, Billia F, O'Sullivan ML, et al. Short-term disruption of diurnal rhythms after murine myocardial infarction adversely affects long-term myocardial structure and function. Circ Res. (2014) 114:1713–22. doi: 10.1161/CIRCRESAHA.114.302995
45. Blagonravov ML, Azova MM, Frolov VA. Chronobiology of cardiac ventricular fibrillation development in experimental acute coronary failure. Bull Exp Biol Med. (2010) 149:559–61. doi: 10.1007/s10517-010-0992-2
46. Hirsch L, Shechter A, Feinberg MS, Koren-Morag N, Shechter M. The impact of early compared to late morning hours on brachial endothelial function and long-term cardiovascular events in healthy subjects with no apparent coronary heart disease. Int J Cardiol. (2011) 151:342–7. doi: 10.1016/j.ijcard.2010.08.069
47. Maruo T, Nakatani S, Kanzaki H, Kakuchi H, Yamagishi M, Kitakaze M, et al. Circadian variation of endothelial function in idiopathic dilated cardiomyopathy. Am J Cardiol. (2006) 97:699–702. doi: 10.1016/j.amjcard.2005.09.118
48. Vyas MV, Garg AX, Iansavichus AV, Costella J, Donner A, Laugsand LE, et al. Shift work and vascular events: systematic review and meta-analysis. BMJ. (2012) 345:e4800. doi: 10.1136/bmj.e4800
49. Sole MJ, Martino TA. Diurnal physiology: core principles with application to the pathogenesis, diagnosis, prevention, and treatment of myocardial hypertrophy and failure. J Appl Physiol 1985. (2009) 107:1318–27. doi: 10.1152/japplphysiol.00426.2009
50. Hu K, Ivanov P, Hilton MF, Chen Z, Ayers RT, Stanley HE, et al. Endogenous circadian rhythm in an index of cardiac vulnerability independent of changes in behavior. Proc Natl Acad Sci USA. (2004) 101:18223–7. doi: 10.1073/pnas.0408243101
51. Teerlink JR, Clozel JP. Hemodynamic variability and circadian rhythm in rats with heart failure: role of locomotor activity. Am J Physiol. (1993) 264:H2111–8. doi: 10.1152/ajpheart.1993.264.6.H2111
52. Giles TD, Roffidal L, Quiroz A, Sander G, Tresznewsky O. Circadian variation in blood pressure and heart rate in nonhypertensive congestive heart failure. J Cardiovasc Pharmacol. (1996) 28:733–40. doi: 10.1097/00005344-199612000-00001
53. Kohsaka A, Das P, Hashimoto I, Nakao T, Deguchi Y, Gouraud SS, et al. The circadian clock maintains cardiac function by regulating mitochondrial metabolism in mice. PLoS ONE. (2014) 9:e112811. doi: 10.1371/journal.pone.0112811
54. Patel VN, Pierce BR, Bodapati RK, Brown DL, Ives DG, Stein PK. Association of holter-derived heart rate variability parameters with the development of congestive heart failure in the cardiovascular health study. JACC Heart Fail. (2017) 5:423–31. doi: 10.1016/j.jchf.2016.12.015
55. Sherazi S, Kutyifa V, McNitt S, Aktas MK, Couderc JP, Peterson B, et al. Prognostic significance of heart rate variability among patients treated with cardiac resynchronization therapy: MADIT-CRT (Multicenter automatic defibrillator implantation trial-cardiac resynchronization therapy). JACC Clin Electrophysiol. (2015) 1:74–80. doi: 10.1016/j.jacep.2015.03.004
56. Yoshino F, Sakuma N, Date T, Unoki T, Fukagawa K, Miyamoto T, et al. Diurnal change of plasma atrial natriuretic peptide concentrations in patients with congestive heart failure. Am Heart J. (1989) 117:1316–9. doi: 10.1016/0002-8703(89)90412-2
57. Caruana MP, Lahiri A, Cashman PM, Altman DG, Raftery EB. Effects of chronic congestive heart failure secondary to coronary artery disease on the circadian rhythm of blood pressure and heart rate. Am J Cardiol. (1988) 62:755–9. doi: 10.1016/0002-9149(88)91217-9
58. Hayano J, Yasuma F, Watanabe E, Carney RM, Stein PK, Blumenthal JA, et al. Blunted cyclic variation of heart rate predicts mortality risk in post-myocardial infarction, end-stage renal disease, and chronic heart failure patients. Europace. (2017) 19:1392–400. doi: 10.1093/europace/euw222
59. Lewis R, Hackfort BT, Schultz HD. Chronic heart failure abolishes circadian rhythms in resting and chemoreflex breathing. Adv Exp Med Biol. (2018) 1071:129–36. doi: 10.1007/978-3-319-91137-3_16
60. Sachan N, Dey A, Rotter D, Grinsfelder DB, Battiprolu PK, Sikder D, et al. Sustained hemodynamic stress disrupts normal circadian rhythms in calcineurin-dependent signaling and protein phosphorylation in the heart. Circ Res. (2011) 108:437–45. doi: 10.1161/CIRCRESAHA.110.235309
61. Jeyaraj D, Haldar SM, Wan X, McCauley MD, Ripperger JA, Hu K, et al. Circadian rhythms govern cardiac repolarization and arrhythmogenesis. Nature. (2012) 483:96–9. doi: 10.1038/nature10852
62. Kishi T. Heart failure as an autonomic nervous system dysfunction. J Cardiol. (2012) 59:117–22. doi: 10.1016/j.jjcc.2011.12.006
63. Sobotka PA, Mahfoud F, Schlaich MP, Hoppe UC, Bohm M, Krum H. Sympatho-renal axis in chronic disease. Clin Res Cardiol. (2011) 100:1049–57. doi: 10.1007/s00392-011-0335-y
64. Dillier R, Zuber M, Arand P, Erne S, Erne P. Assessment of systolic and diastolic function in heart failure using ambulatory monitoring with acoustic cardiography. Ann Med. (2011) 43:403–11. doi: 10.3109/07853890.2010.550309
65. Mochel JP, Fink M, Bon C, Peyrou M, Bieth B, Desevaux C, et al. Influence of feeding schedules on the chronobiology of renin activity, urinary electrolytes and blood pressure in dogs. Chronobiol Int. (2014) 31:715–30. doi: 10.3109/07420528.2014.897711
66. Shaffer F, Ginsberg JP. An overview of heart rate variability metrics and norms. Front Public Health. (2017) 5:258. doi: 10.3389/fpubh.2017.00258
67. Lee HW, Han TH, Yi KJ, Choi MC, Lee SY, Ryu PD. Time course of diurnal rhythm disturbances in autonomic function of rats with myocardial infarction. Auton Neurosci. (2013) 179:28–36. doi: 10.1016/j.autneu.2013.06.007
68. Schrier RW, Gurevich AK, Cadnapaphornchai MA. Pathogenesis and management of sodium and water retention in cardiac failure and cirrhosis. Semin Nephrol. (2001) 21:157–72. doi: 10.1053/snep.2001.20933
69. De Ferrari GM. Vagal stimulation in heart failure. J Cardiovasc Transl Res. (2014) 7:310–20. doi: 10.1007/s12265-014-9540-1
70. Gold MR, Van Veldhuisen DJ, Hauptman PJ, Borggrefe M, Kubo SH, Lieberman RA, et al. Vagus nerve stimulation for the treatment of heart failure: the INOVATE-HF trial. J Am College Cardiol. (2016) 68:149–58. doi: 10.1016/j.jacc.2016.03.525
71. Piccirillo G, Moscucci F, D'Alessandro G, Pascucci M, Rossi P, Han S, et al. Myocardial repolarization dispersion and autonomic nerve activity in a canine experimental acute myocardial infarction model. Heart Rhythm. (2014) 11:110–8. doi: 10.1016/j.hrthm.2013.10.022
72. Witte K, Engelhardt S, Janssen BJ, Lohse M, Lemmer B. Circadian and short-term regulation of blood pressure and heart rate in transgenic mice with cardiac overexpression of the beta1-adrenoceptor. Chronobiol Int. (2004) 21:205–16. doi: 10.1081/CBI-120037801
73. Mousa TM, Schiller AM, Zucker IH. Disruption of cardiovascular circadian rhythms in mice post myocardial infarction: relationship with central angiotensin II receptor expression. Physiol Rep. (2014) 2: doi: 10.14814/phy2.12210
74. Ohori T, Hirai T, Joho S, Kameyama T, Nozawa T, Asanoi H, et al. Circadian changes in autonomic function in conscious rats with heart failure: effects of amiodarone on sympathetic surge. Auton Neurosci. (2011) 159:20–5. doi: 10.1016/j.autneu.2010.07.001
75. Witte K, Hu K, Swiatek J, Müssig C, Ertl G, Lemmer B. Experimental heart failure in rats: effects on cardiovascular circadian rhythms and on myocardial beta-adrenergic signaling. Cardiovasc Res. (2000) 47:350–58. doi: 10.1016/S0008-6363(00)00099-7
76. Shaffer F, McCraty R, Zerr CL. A healthy heart is not a metronome: an integrative review of the heart's anatomy and heart rate variability. Front Psychol. (2014) 5:1040. doi: 10.3389/fpsyg.2014.01040
77. Bonnemeier H, Richardt G, Potratz J, Wiegand UK, Brandes A, Kluge N, et al. Circadian profile of cardiac autonomic nervous modulation in healthy subjects: differing effects of aging and gender on heart rate variability. J Cardiovasc Electrophysiol. (2003) 14:791–9. doi: 10.1046/j.1540-8167.2003.03078.x
78. Boudreau P, Yeh WH, Dumont GA, Boivin DB. Circadian variation of heart rate variability across sleep stages. Sleep. (2013) 36:1919–28. doi: 10.5665/sleep.3230
79. Gerritsen J, Dekker JM, TenVoorde BJ, Kostense PJ, Heine RJ, Bouter LM, et al. Impaired autonomic function is associated with increased mortality, especially in subjects with diabetes, hypertension, or a history of cardiovascular disease: the Hoorn study. Diabetes Care. (2001) 24:1793–8. doi: 10.2337/diacare.24.10.1793
80. Nolan J, Batin PD, Andrews R, Lindsay SJ, Brooksby P, Mullen M, et al. Prospective study of heart rate variability and mortality in chronic heart failure: results of the United Kingdom heart failure evaluation and assessment of risk trial (UK-heart). Circulation. (1998) 98:1510–6. doi: 10.1161/01.CIR.98.15.1510
81. Henze M, Hart D, Samarel A, Barakat J, Eckert L, Scrogin K. Persistent alterations in heart rate variability, baroreflex sensitivity, and anxiety-like behaviors during development of heart failure in the rat. Am J Physiol Heart Circ Physiol. (2008) 295:H29–38. doi: 10.1152/ajpheart.01373.2007
82. Rydlewska A, Jankowska EA, Ponikowska B, Borodulin-Nadzieja L, Banasiak W, Ponikowski P. Changes in autonomic balance in patients with decompensated chronic heart failure. Clin Auton Res. (2011) 21:47–54. doi: 10.1007/s10286-010-0089-z
83. Omran H, Bitter T, Horstkotte D, Oldenburg O, Fox H. Characteristics and circadian distribution of cardiac arrhythmias in patients with heart failure and sleep-disordered breathing. Clin Res Cardiol. (2018) 107:965–74. doi: 10.1007/s00392-018-1269-4
84. Beckers F, Verheyden B, Couckuyt K, Aubert AE. Fractal dimension in health and heart failure. Biomed Technik. (2006) 51:194–7. doi: 10.1515/BMT.2006.035
85. Ueno LM, Drager LF, Rodrigues ACT, Rondon MUPB, Mathias W Jr, Krieger EM, et al. Day-night pattern of autonomic nervous system modulation in patients with heart failure with and without sleep apnea. Int J Cardiol. (2011) 148:53–8. doi: 10.1016/j.ijcard.2009.10.023
86. Kim SG, Yum MK. Decreased RR interval complexity and loss of circadian rhythm in patients with congestive heart failure. Jpn Circ J. (2000) 64:39–45. doi: 10.1253/jcj.64.39
87. Watanabe E, Arakawa T, Uchiyama T, Tong M, Yasui K, Takeuchi H, et al. Prognostic significance of circadian variability of RR and GT intervals and GT dynamicity in patients with chronic heart failure. Heart Rhythm. (2007) 4:999–1005. doi: 10.1016/j.hrthm.2007.04.019
88. Jandackova VK, Scholes S, Britton A, Steptoe A. Are changes in heart rate variability in middle-Aged and older people normative or caused by pathological conditions? Findings from a large population-based longitudinal cohort study. J Am Heart Assoc. (2016) 5:e002365. doi: 10.1161/JAHA.115.002365
89. Shehab A, Elnour AA, Struthers AD. A randomised, controlled, double-blind, cross-over pilot study assessing the effects of spironolactone, losartan and their combination on heart rate variability and GT dispersion in patients with chronic heart failure. Cardiovasc J Afr. (2008) 19:292–6.
90. Aronson D, Burger AJ. Effect of beta-blockade on autonomic modulation of heart rate and neurohormonal profile in decompensated heart failure. Ann Noninvas Electrocardiol. (2001) 6:98–106. doi: 10.1111/j.1542-474X.2001.tb00093.x
91. Burger AJ, Kamalesh M. Effect of beta-adrenergic blocker therapy on the circadian rhythm of heart rate variability in patients with chronic stable angina pectoris. Am J Cardiol. (1999) 83:596–A8. doi: 10.1016/S0002-9149(98)00921-7
92. Compostella L, Russo N, Setzu T, Tursi V, Bottio T, Tarzia V, et al. Cardiac autonomic dysfunction in the early phase after left ventricular assist device implant: implications for surgery and follow-up. Int J Artif Organs. (2013) 36:410–8. doi: 10.5301/ijao.5000210
93. Suzuki K, Nishinaka T, Miyamoto T, Ichihara Y, Yamazaki K. Circadian variation of motor current observed in fixed rotation speed continuous-flow left ventricular assist device support. J Artif Organs. (2014) 17:157–61. doi: 10.1007/s10047-014-0762-4
94. Carson PA, O'Connor CM, Miller AB, Anderson S, Belkin R, Neuberg GW, et al. Circadian rhythm and sudden death in heart failure: results from prospective randomized amlodipine survival trial. J Am Coll Cardiol. (2000) 36:541–6. doi: 10.1016/S0735-1097(00)00728-2
95. Miyamoto S, Fujita M, Tambara K, Sekiguchi H, Eiho S, Hasegawa K, et al. Circadian variation of cardiac autonomic nervous activity is well preserved in patients with mild to moderate chronic heart failure: effect of patient position. Int J Cardiol. (2004) 93:247–52. doi: 10.1016/S0167-5273(03)00190-6
96. Dallmann R, Brown SA, Gachon F. Chronopharmacology: new insights and therapeutic implications. Annu Rev Pharmacol Toxicol. (2014) 54:339–61. doi: 10.1146/annurev-pharmtox-011613-135923
97. Buttgereit F, Smolen JS, Coogan AN, Cajochen C. Clocking in: chronobiology in rheumatoid arthritis. Nat Rev Rheumatol. (2015) 11:349–56. doi: 10.1038/nrrheum.2015.31
98. Smolensky MH, Peppas NA. Chronobiology, drug delivery, and chronotherapeutics. Adv Drug Deliv Rev. (2007) 59:828–51. doi: 10.1016/j.addr.2007.07.001
99. Ohdo S. Chronotherapeutic strategy: rhythm monitoring, manipulation and disruption. Adv Drug Deliv Rev. (2010) 62:859–75. doi: 10.1016/j.addr.2010.01.006
100. Ohmori M, Fujimura A. ACE inhibitors and chronotherapy. Clin Exp Hypertens. (2005) 27:179–85. doi: 10.1081/CEH-200048762
101. Hermida RC, Crespo JJ, Domínguez-Sardiña M, Otero A, Moyá A, Ríos MT, et al. Bedtime hypertension treatment improves cardiovascular risk reduction: the hygia chronotherapy trial. Eur Heart J. (2019) 41:4565–76. doi: 10.1093/eurheartj/ehz754
102. Buurma M, Van diemen JJK, Thijs A, Numans ME, Bonten TN. Circadian rhythm of cardiovascular disease: the potential of chronotherapy with aspirin. Front Cardiovasc Med. (2019) 6:84. doi: 10.3389/fcvm.2019.00084
103. Martino TA, Tata N, Simpson JA, Vanderlaan R, Dawood F, Kabir MG, et al. The primary benefits of angiotensin-converting enzyme inhibition on cardiac remodeling occur during sleep time in murine pressure overload hypertrophy. J Am Coll Cardiol. (2011) 57:2020–8. doi: 10.1016/j.jacc.2010.11.022
104. Fujimura A, Ebihara A. Chronopharmacological study of furosemide in rats. Life Sci. (1986) 38:1215–20. doi: 10.1016/0024-3205(86)90176-1
105. Calvo C, Hermida RC, Ayala DE, Lopez JE, Rodriguez M, Chayan L, et al. [Chronotherapy with torasemide in hypertensive patients: increased efficacy and therapeutic coverage with bedtime administration]. Med Clin. (2006) 127:721–9. doi: 10.1157/13095521
106. Wang C, Zhang J, Liu X, Li CC, Ye ZC, Peng H, et al. Effect of valsartan with bedtime dosing on chronic kidney disease patients with nondipping blood pressure pattern. J Clin Hypertens. (2013) 15:48–54. doi: 10.1111/jch.12021
107. Tsimakouridze EV, Alibhai FJ, Martino TA. Therapeutic applications of circadian rhythms for the cardiovascular system. Front Pharmacol. (2015) 6:77. doi: 10.3389/fphar.2015.00077
108. Fujimura A, Sudoh T, Shiga T, Ohashi K, Ebihara A. Influence of clorgyline treatment on chronopharmacology of furosemide in rats. Life Sci. (1993) 52:819–24. doi: 10.1016/0024-3205(93)90080-M
109. Fujimura A, Ebihara A. Chronopharmacological study of furosemide in rats: (II). Influence of beta-adrenoceptor blockade. Life Sci. (1988) 42:1431–7. doi: 10.1016/0024-3205(88)90053-7
110. Fujimura A, Ohashi K, Ebihara A. Chronopharmacological study of furosemide; (IX). Influence of continuous norepinephrine infusion. Life Sci. (1992) 50:449–55. doi: 10.1016/0024-3205(92)90380-8
111. Fujimura A, Shiga T, Sudoh T, Ohashi K, Ebihara A. Influence of renal denervation on chronopharmacology of furosemide in rats. Life Sci. (1992) 51:1811–6. doi: 10.1016/0024-3205(92)90052-Q
112. Mochel JP, Danhof M. Chronobiology and pharmacologic modulation of the renin-angiotensin-aldosterone system in dogs: what have we learned? Rev Physiol Biochem Pharmacol. (2015) 169:43–69. doi: 10.1007/112_2015_27
113. Dzida G, Prystupa A, Lachowska-Kotowska P, Kadas T, Kamienski P, Kimak E, et al. Alteration in diurnal and nocturnal melatonin serum level in patients with chronic heart failure. Ann Agric Environ Med. (2013) 20:745–8.
114. Stujanna EN, Murakoshi N, Tajiri K, Xu D, Kimura T, Qin R, et al. Rev-erb agonist improves adverse cardiac remodeling and survival in myocardial infarction through an anti-inflammatory mechanism. PLoS ONE. (2017) 12:e0189330. doi: 10.1371/journal.pone.0189330
115. Reitz CJ, Alibhai FJ, Khatua TN, Rasouli M, Bridle BW, Burris TP, et al. SR9009 administered for one day after myocardial ischemia-reperfusion prevents heart failure in mice by targeting the cardiac inflammasome. Commun Biol. (2019) 2:353. doi: 10.1038/s42003-019-0595-z
116. Goldberger AL. Non-linear dynamics for clinicians: chaos theory, fractals, and complexity at the bedside. Lancet. (1996) 347:1312–4. doi: 10.1016/S0140-6736(96)90948-4
117. Ilan Y. Advanced tailored randomness: a novel approach for improving the efficacy of biological systems. J Comput Biol. (2019) 27:20–29. doi: 10.1089/cmb.2019.0231
118. Ilan Y. Randomness in microtubule dynamics: an error that requires correction or an inherent plasticity required for normal cellular function? Cell Biol Int. (2019) 43:739–48. doi: 10.1002/cbin.11157
119. Ilan Y. Generating randomness: making the most out of disordering a false order into a real one. J Transl Med. (2019) 17:49. doi: 10.1186/s12967-019-1798-2
120. Finn EH, Misteli T. Molecular basis and biological function of variability in spatial genome organization. Science. (2019) 365:eaaw9498. doi: 10.1126/science.aaw9498
121. Singh N, Moneghetti KJ, Christle JW, Hadley D, Plews D, Froelicher V. Heart rate variability: an old metric with new meaning in the era of using mHealth technologies for health and exercise training guidance. Part One: Physiology and Methods. Arrhythm Electrophysiol Rev. (2018) 7:193–8. doi: 10.15420/aer.2018.27.2
122. Shields RW Jr. Heart rate variability with deep breathing as a clinical test of cardiovagal function. Cleve Clin J Med. (2009) 76(Suppl 2):S37–40. doi: 10.3949/ccjm.76.s2.08
123. Kox M, Pompe JC, Van der hoeven JG, Hoedemaekers CW, Pickkers P. Influence of different breathing patterns on heart rate variability indices and reproducibility during experimental endotoxaemia in human subjects. Clin Sci. (2011) 121:215–22. doi: 10.1042/CS20110027
124. Konig N, Singh NB, Baumann CR, Taylor WR. Can gait signatures provide quantitative measures for aiding clinical decision-making? A systematic meta-analysis of gait variability behavior in patients with Parkinson's disease. Front Hum Neurosci. (2016) 10:319. doi: 10.3389/fnhum.2016.00319
125. Nayyar S, Hasan MA, Roberts-Thomson KC, Sullivan T, Baumert M. Effect of loss of heart rate variability on T-wave heterogeneity and QT variability in heart failure patients: implications in ventricular arrhythmogenesis. Cardiovasc Eng Technol. (2017) 8:219–228. doi: 10.1007/s13239-017-0299-9
126. Avolio A. Heart rate variability and stroke: strange attractors with loss of complexity. J Hypertens. (2013) 31:1529–31. doi: 10.1097/HJH.0b013e328362ff5e
127. Moon Y, Sung J, An R, Hernandez ME, Sosnoff JJ. Gait variability in people with neurological disorders: a systematic review and meta-analysis. Hum Mov Sci. (2016) 47:197–208. doi: 10.1016/j.humov.2016.03.010
128. Elgart V, Lin JR, Loscalzo J. Determinants of drug-target interactions at the single cell level. PLoS Comput Biol. (2018) 14:e1006601. doi: 10.1371/journal.pcbi.1006601
129. Noble D. Evolution viewed from physics, physiology and medicine. Interface Focus. (2017) 7:20160159. doi: 10.1098/rsfs.2016.0159
130. DiFrancesco D, Camm JA. Heart rate lowering by specific and selective i(f) current inhibition with ivabradine: a new therapeutic perspective in cardiovascular disease. Drugs. (2004) 64:1757–65. doi: 10.2165/00003495-200464160-00003
131. Leino AD, King EC, Jiang W, Vinks AA, Klawitter J, Christians U, et al. Assessment of tacrolimus intrapatient variability in stable adherent transplant recipients: establishing baseline values. Am J Transplant. (2018) 19:1410–20. doi: 10.1111/ajt.15199
132. Gueta I, Markovits N, Yarden-Bilavsky H, Raichlin E, Freimark D, Lavee J, et al. High tacrolimus trough level variability is associated with rejections after heart transplant. Am J Transplant. (2018) 18:2571–78. doi: 10.1111/ajt.15016
133. Gueta I, Markovits N, Yarden-Bilavsky H, Raichlin E, Freimark D, Lavee J, et al. Intrapatient variability in tacrolimus trough levels after solid organ transplantation varies at different postoperative time periods. Am J Transplant. (2018) 19:611. doi: 10.1111/ajt.15134
134. Del Bello, Congy-Jolivet N, Danjoux M, Muscari F, Lavayssiere L, Esposito L, et al. High tacrolimus intra-patient variability is associated with graft rejection, and de novo donor-specific antibodies occurrence after liver transplantation. World J Gastroenterol. (2018) 24:1795–802. doi: 10.3748/wjg.v24.i16.1795
135. Ilan Y. Why targeting the microbiome is not so successful: can randomness overcome the adaptation that occurs following gut manipulation? Clin Exp Gastroenterol. (2019) 12:209–17. doi: 10.2147/CEG.S203823
136. Ilan Y. Beta-glycosphingolipids as mediators of both inflammation and immune tolerance: a manifestation of randomness in biological systems. Front Immunol. (2019) 10:1143. doi: 10.3389/fimmu.2019.01143
137. Contin M, Alberghini L, Candela C, Benini G, Riva R. Intrapatient variation in antiepileptic drug plasma concentration after generic substitution vs stable brand-name drug regimens. Epilepsy Res. (2016) 122:79–83. doi: 10.1016/j.eplepsyres.2016.02.012
138. Strik AB, Mould S, Mathôt D, Ponsioen R, van den Brande C, Jansen J, et al. Dashboard driven vs. conventional dosing of infliximab in inflammatory bowel disease patients: the PRECISION trial. J Crohns Colitis. (2019) 13:S063. doi: 10.1093/ecco-jcc/jjy222.090
139. Wu GQ, Arzeno NM, Shen LL, Tang DK, Zheng DA, Zhao NQ, et al. Chaotic signatures of heart rate variability and its power spectrum in health, aging and heart failure. PLoS ONE. (2009) 4:e4323. doi: 10.1371/journal.pone.0004323
140. Porta A, D'Addio G, Corbi G, Maestri R, Pinna GD. Circadian variations of short-term heart period irreversibility in healthy and chronic heart failure patients. Conf Proc IEEE Eng Med Biol Soc. (2008) 2008:2116–9. doi: 10.1109/IEMBS.2008.4649611
141. Kyriazis M. Practical applications of chaos theory to the modulation of human ageing: nature prefers chaos to regularity. Biogerontology. (2003) 4:75–90. doi: 10.1023/A:1023306419861
142. Ilan Y. Overcoming compensatory mechanisms toward chronic drug administration to ensure long-term, sustainable beneficial effects. Mol Ther Methods Clin Dev. (2020) 18:335–44. doi: 10.1016/j.omtm.2020.06.006
143. Ilan Y. Improving global healthcare and reducing costs using second-generation artificial intelligence-based digital pills: a market disruptor. Int J Environ Res Public Health. (2021) 18:811. doi: 10.3390/ijerph18020811
144. Ilan Y. Second-generation digital health platforms: placing the patient at the center and focusing on clinically meaningful endpoints title: second-generation artificial intelligence algorithms. Front Digit Health. (2020) 2:569178. doi: 10.3389/fdgth.2020.569178
145. Kenig A, Ilan Y. A personalized signature and chronotherapy-Based platform for improving the efficacy of sepsis treatment. Front Physiol. (2019) 10:1542. doi: 10.3389/fphys.2019.01542
146. Khoury T, Ilan Y. Introducing patterns of variability for overcoming compensatory adaptation of the immune system to immunomodulatory agents: a Novel method for improving clinical response to anti-TNF therapies. Front Immunol. (2019) 10:2726. doi: 10.3389/fimmu.2019.02726
147. El-Haj M, Kanovitch D, Ilan Y. Personalized inherent randomness of the immune system is manifested by an individualized response to immune triggers and immunomodulatory therapies: a novel platform for designing personalized immunotherapies. Immunol Res. (2019) 67:337–47. doi: 10.1007/s12026-019-09101-y
148. Ilan Y. Advanced tailored randomness: a novel approach for improving the efficacy of biological systems. J Comput Biol. (2020) 27:20–9.
149. Khoury T, Ilan Y. Platform introducing individually tailored variability in nerve stimulations and dietary regimen to prevent weight regain following weight loss in patients with obesity. Obes Res Clin Pract. (2021) 15:114–23. doi: 10.1016/j.orcp.2021.02.003
150. Kolben Y, Weksler-Zangen S, Ilan Y. Adropin as a potential mediator of the metabolic system-autonomic nervous system-chronobiology axis: implementing a personalized signature-based platform for chronotherapy. Obes Rev. (2021) 22:e13108. doi: 10.1111/obr.13108
151. Ilan Y, Spigelman Z. Establishing patient-tailored variability-based paradigms for anti-cancer therapy: using the inherent trajectories which underlie cancer for overcoming drug resistance. Cancer Treat Res Commun. (2020) 25:100240. doi: 10.1016/j.ctarc.2020.100240
152. Kessler A, Weksler-Zangen S, Ilan Y. Role of the immune system and the circadian rhythm in the pathogenesis of chrnic pancreatitis: establishing a personalized signature for improving the effect of immunotherapies for chronic pancreatitis. Pancreas. (2020) 49:1024–32. doi: 10.1097/MPA.0000000000001626
153. Potruch A, Khoury ST, Ilan Y. The role of chronobiology in drug-resistance epilepsy: the potential use of a variability and chronotherapy-based individualized platform for improving the response to anti-seizure drugs. Seizure. (2020) 80:201–11. doi: 10.1016/j.seizure.2020.06.032
154. Forkosh E, Kenig A, Ilan Y. Introducing variability in targeting the microtubules: review of current mechanisms and future directions in colchicine therapy. Pharmacol Res Perspect. (2020) 8:e00616. doi: 10.1002/prp2.616
155. Gelman R, Bayatra A, Kessler A, Schwartz A, Ilan Y. Targeting SARS-CoV-2 receptors as a means for reducing infectivity and improving antiviral and immune response: an algorithm-based method for overcoming resistance to antiviral agents. Emerg Microbes Infect. (2020) 9:1397–406. doi: 10.1080/22221751.2020.1776161
Keywords: heart failure, diuretic resistance, chronobiology, variability, digital systems
Citation: Kenig A, Kolben Y, Asleh R, Amir O and Ilan Y (2021) Improving Diuretic Response in Heart Failure by Implementing a Patient-Tailored Variability and Chronotherapy-Guided Algorithm. Front. Cardiovasc. Med. 8:695547. doi: 10.3389/fcvm.2021.695547
Received: 15 April 2021; Accepted: 21 July 2021;
Published: 11 August 2021.
Edited by:
Paul Leeson, University of Oxford, United KingdomReviewed by:
Julio Nunez, Hospital Clínico Universitario de Valencia, SpainCopyright © 2021 Kenig, Kolben, Asleh, Amir and Ilan. This is an open-access article distributed under the terms of the Creative Commons Attribution License (CC BY). The use, distribution or reproduction in other forums is permitted, provided the original author(s) and the copyright owner(s) are credited and that the original publication in this journal is cited, in accordance with accepted academic practice. No use, distribution or reproduction is permitted which does not comply with these terms.
*Correspondence: Yaron Ilan, aWxhbkBoYWRhc3NhaC5vcmcuaWw=
†These authors share first authorship
Disclaimer: All claims expressed in this article are solely those of the authors and do not necessarily represent those of their affiliated organizations, or those of the publisher, the editors and the reviewers. Any product that may be evaluated in this article or claim that may be made by its manufacturer is not guaranteed or endorsed by the publisher.
Research integrity at Frontiers
Learn more about the work of our research integrity team to safeguard the quality of each article we publish.