Corrigendum: The dual role of low-density lipoprotein receptor–related protein 1 in atherosclerosis
- Department of Neurology, Tongji Medical College, Union Hospital, Huazhong University of Science and Technology, Wuhan, China
Low-density lipoprotein receptor–related protein-1 (LRP1) is a large endocytic and signaling receptor belonging to the LDL receptor (LDLR) gene family and that is widely expressed in several tissues. LRP1 comprises a large extracellular domain (ECD; 515 kDa, α chain) and a small intracellular domain (ICD; 85 kDa, β chain). The deletion of LRP1 leads to embryonic lethality in mice, revealing a crucial but yet undefined role in embryogenesis and development. LRP1 has been postulated to participate in numerous diverse physiological and pathological processes ranging from plasma lipoprotein homeostasis, atherosclerosis, tumor evolution, and fibrinolysis to neuronal regeneration and survival. Many studies using cultured cells and in vivo animal models have revealed the important roles of LRP1 in vascular remodeling, foam cell biology, inflammation and atherosclerosis. However, its role in atherosclerosis remains controversial. LRP1 not only participates in the removal of atherogenic lipoproteins and proatherogenic ligands in the liver but also mediates the uptake of aggregated LDL to promote the formation of macrophage- and vascular smooth muscle cell (VSMC)-derived foam cells, which causes a prothrombotic transformation of the vascular wall. The dual and opposing roles of LRP1 may also represent an interesting target for atherosclerosis therapeutics. This review highlights the influence of LRP1 during atherosclerosis development, focusing on its dual role in vascular cells and immune cells.
Introduction
Atherosclerosis (AS), in concert with its related disorders, such as coronary heart diseases, stroke, and peripheral vascular diseases, is the leading cause of morbidity and mortality worldwide (1–4). Some researchers have characterized atherosclerosis as damage to three major processes: systemic and cellular cholesterol homeostasis, inflammation and apoptosis/endocytosis (5). Atherosclerosis is initiated by endothelial dysfunction due to activation of endothelial cells by irritative stimuli, such as hyperlipidemia, high shear forces, hypertension, and proinflammatory mediators, which allow blood monocytes to permeate the endothelial cell layer and infiltrate into the intima and subintima. After entering into the intima, the monocytes differentiate into macrophages, which subsequently uptake modified low-density lipoprotein (LDL) or oxidized phospholipids to further form foam cells. The successive accumulation of apoptotic foam cells in the endothelium cannot be cleared in time, gradually leading to the formation of thrombi and inflammatory necrotic cores (6, 7). Subsequently, the release of the chemoattractant platelet-derived growth factor from macrophages, activated platelets and endothelial cells causes vascular smooth muscle cells (VSMCs) to migrate from the medium to the intima, proliferate, undergo apoptosis and senescence, and produce extracellular matrix to form fibrous caps of atherosclerotic plaques to prevent plaques rupture. However, the aberrant proliferation of VSMCs promotes plaque formation, and the balance of VSMC proliferation between migration vs. cell death and senescence determines the population of VSMCs within the atherosclerotic plaques. These processes play important roles in the formation of atherosclerosis and the stability of plaques (8, 9). Lastly, the rupture of atherosclerotic plaques promoted by cap thinning due to the death of VSMCs and the breakdown of collagen and ECM leads to thrombosis, potentially resulting in major cardiovascular episodes such as stroke and myocardial infarction (9).
LDL receptor-related protein (LRP1) is a 600-kDa type I glycosylated transmembrane protein belonging to the LDL receptor (LDLR) superfamily and is ubiquitously expressed in multiple cell types (10–13). This multifunctional transmembrane protein has been reported to regulate cholesterol homeostasis, inflammation and apoptosis/endocytosis (14–16). LRP1 is expressed in both normal and atherosclerotic arteries (17) and can recognize both lipoprotein and non-lipoprotein ligands to participate in a wide variety of biological processes, including lipid metabolism (18), blood-brain barrier (BBB) integrity (19) and macrophage migration (20). Genome-wide association studies have revealed that the LRP1 gene constitutes a susceptibility locus for abdominal aortic aneurysms, elevated plasma lipids and coronary heart disease (21–23). Many translational studies have shown that LRP1 is involved in two major physiological processes: endocytosis and signaling pathway regulation. The endocytosis of multiple extracellular ligands of LRP1, including apolipoprotein E (ApoE)- and lipoprotein lipase-enriched lipoproteins, thrombospondin, and plasminogen activators, is important in vascular biology and tumor progression. In addition, LRP1 can initiate and regulate diverse signaling pathways, including the mitogen-activated protein kinase (MAPK), insulin receptor (IR), serine/threonine protein kinase (AKT), extracellular signal-regulated kinase (ERK), and c-jun N-terminal kinase (JNK) pathways (24–29).
However, recent studies have shown that LRP1 has dual and opposing roles in regulating arteriosclerosis. Studies have indicated that LRP1 participates in the removal of atherogenic lipoproteins and other proatherogenic ligands, such as tissue-type plasminogen activator (t-PA) and urokinase-type plasminogen activator (u-PA), from the circulation in the liver (30, 31). Hepatic LRP1 plays a protective role in atherogenesis but does so independent of plasma cholesterol. In addition, several studies have revealed that LRP1 expressed in the VSMCs (14, 32–35) and macrophages (15, 35–39) protects the vasculature against the development of atherosclerosis. Moreover, another study showed that LRP1 stimulates a canonical Wnt5a signaling pathway to prevent cholesterol accumulation in fibroblasts (40). However, several studies have revealed that LRP1 efficiently internalizes aggregated LDL (aggLDL), which binds to LRP1 cluster II, into VSMCs (41–46) and macrophages (46), causing high intracellular accumulation of cholesteryl esters (CEs) in these cells. Furthermore, LRP1 also regulates cholesterol accumulation in macrophages (47), promoting their transformation into foam cells and leading to atherosclerosis. In turn, cholesterol accumulation promotes LRP1 overexpression and induces a positive feedback loop that efficiently induces the formation of VSMC- and macrophage-derived foam cells (44, 46, 48). AggLDL, a major modified form of LDL in the arterial intima, is a potent inducer of massive intracellular cholesteryl ester accumulation in both macrophages (49–51) and VSMCs (41, 44, 48). In addition, studies have shown that LRP1 mediates cholesteryl ester accumulation from lipoproteins in cardiomyocytes (52, 53), and another study showed that LRP1 controls adipogenesis and is upregulated in obese adipose tissue from humans and mice (54). Moreover, LRP1 plays a major role in controlling brain protein and lipoprotein metabolism as well as the development and regeneration in the central nervous system (55) and can also regulate the inflammatory response in the lung (56).
In this review, we mainly provide a great detailed discussion of the dual role of LRP1 in regulating atherosclerosis and its implication in antiatherosclerosis or proatherosclerosis, in order to enhance our understanding of the underlying mechanism in order to develop novel prophylactic and therapeutic strategies against cardiovascular diseases (CVDs).
Introduction to LRP1
Discovery of LRP1
In 1988, Herz et al. described a cell surface protein containing 4,544 amino acids that was abundant in the liver and had high structural and biochemical similarity with the LDL receptor. They named this protein LDL receptor-related protein (LRP) (12). Later, this receptor was identified by Ashcom et al. and Moestrup and Gliemann, who isolated and sequenced the liver receptor responsible for the catabolism of the α-2-M-proteinase complex (57, 58). Beisiegel et al. described LRP as an ApoE-binding protein that plays an important role in cholesterol metabolism by mediating the uptake of LDL from plasma into cells (59). In addition, LRP is a large multifunctional clearance receptor that has been implicated in the hepatic uptake of chylomicron remnants and the removal of both circulation and extracellular space-associated protease-inhibitor complexes (60). The LRP1 gene is located on chromosome 12q13-14 (61) and is synthesized in the endoplasmic reticulum as a transmembrane glycosylated precursor protein with an apparent molecular mass of ~600 kDa. After it enters the Golgi complex, LRP1 is cleaved to generate two subunits (62). Global knockout of the LRP1 gene in mice is embryonic lethal, demonstrating an essential role for LRP1 in embryogenesis and development (60, 63).
Structure of LRP1 and sLRP1
Structure of LRP1
LRP1, also called CD91 or α-2-M receptor, is a type I glycosylated transmembrane protein comprising a large extracellular domain (ECD) (515 kDa, α chain) and a small intracellular domain (ICD; 85 kDa, β chain), which are non-covalently associated on the cell surface. The large ECD contains four clusters of complement-like repeats and epidermal growth factor (EGF) repeats. The small ICD contains one YXxL motif, two dileucine motifs responsible for endocytosis and two NPxY motifs that function as secondary endocytosis signals and binding sites for signaling adaptor proteins (10–12, 18, 62, 64–69). The NPxY motif can be phosphorylated on several serine, threonine or tyrosine residues to regulate signal transduction (70–72). Additionally, LRP1 can undergo intramembrane proteolysis called regulated intramembrane proteolysis (RIP), which induces cleavage of the released ICD of LRP (LRP-ICD) by γ-secretase and subsequent translocation of this domain into the cell nucleus, where it represses interferon-γ promoter activity to suppress inflammation (73, 74). Like all members of the LDL receptor family, LRP1 consists of five modular structural units: cysteine-rich complement-type repeats (CRs), EGF precursor repeats, β-propeller (YWTD) domains, a transmembrane domain and a cytoplasmic domain (Figure 1) (75). The EGF precursor consists of two cysteine-rich EGF repeats, a YWTD repeat and another EGF-like repeat (76). The extracellular region contains four ligand-binding domains (clusters I-IV) consisting of 2, 8, 10, and 11 CRs, respectively, with clusters II and IV are the major ligand-binding regions (77).
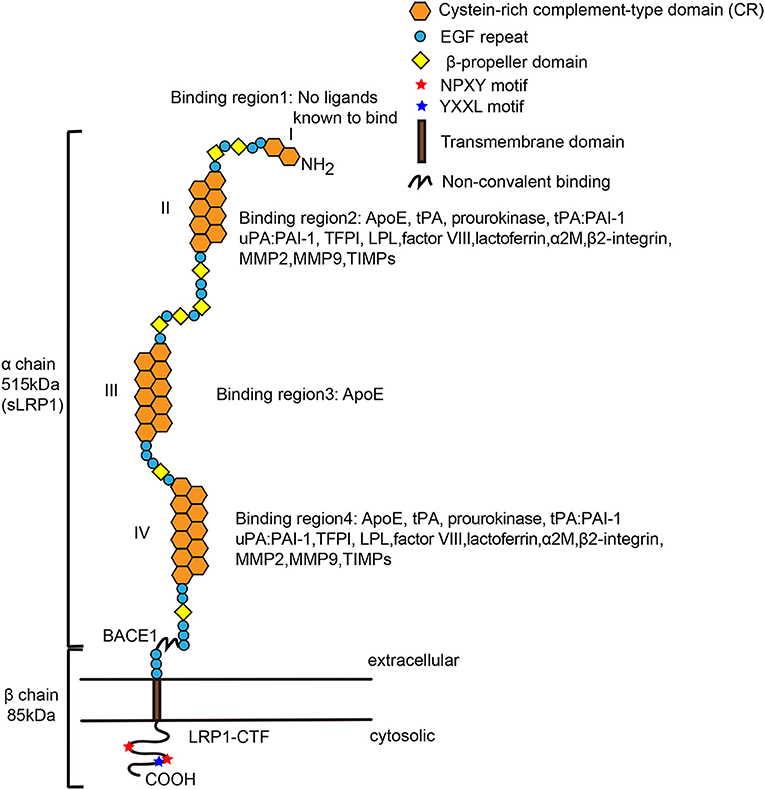
Figure 1. Structure of mature low-density lipoprotein receptor-related protein-1 (LRP-1). LRP1 is a multifunctional receptor that binds a large spectrum of extracellular and intracellular ligands. The α chain contains long modular arrays of acidic cysteine-rich complement-type repeats (CRs), along with epidermal growth factor (EGF)-like domains and β-propeller modules. Also, it consists of four ligand-binding regions (I, II, III, and VI), which are composed of 2, 8, 10, and 11 CRs, respectively. The cytoplasmic tail contains two NPxY motifs that are required for endocytosis and multiple signaling pathways. LRP1 has an YXXL motif adjacent to the second NPxY motif, enabling rapid endocytosis. The β chain also interacts with scaffolding proteins such as PSD-95, Dab-1, and FE-65. Regions II and IV bind most of the currently mapped known ligands of LRP1. β-Secretase (BACE1) cleaves the extracellular domain of LRP1 to form sLRP1 and LRP1-CTF (LRP1-C-terminal fragment). Both extra- and intracellular chains can act independently of each other when the α chain is shed as a soluble LRP1 and the β chain translocates to the nucleus and activates gene transcription and signaling cascades. LPL, lipoprotein lipase; ApoE, apolipoprotein E; COOH, carboxy terminal; EGF, epidermal growth factor; NH2, amino terminal. The image of Figure 1 was modified from the reference (75), adding details to the pictures of the ligands of LRP1.
sLRP1: The Soluble Form of LRP1
Similar to a wide variety of receptors and other plasma membrane proteins, LRP1 can be cleaved by cell surface proteases such as hepatic metalloproteinases, tPA and neuronal β-secretase protease (BACE1) to produce soluble LRP1 (sLRP1), which can be detected in plasma and cerebrospinal fluid (19, 78–84). This process can be accelerated by inflammatory mediators in cultured bone marrow macrophages, such as lipopolysaccharide (LPS) and interferon-γ (IFN-γ); sLRP1 contains the α chain and a 55-kDa fragment of the β chain, suggesting that cleavage occurs near the plasma membrane (85, 86). In addition, sLRP1 maintains the ligand-binding properties of cellular LRP1 and may therefore act as a competitive ligand uptake inhibitor that binds LRP1 on the cell surface (87). By binding extracellular ligands interacting with LRP1, sLRP1 could regulate their endocytosis or control multiple cell signaling pathways. In RAW 264.7 macrophage-like cells, sLRP1 was shown to promote tumor necrosis factor-α (TNF-α), monocyte chemoattractant protein type-1 (MCP-1)/CCL2 and IL-10 expression through activation of the MAPK and JNK cellular pathways to regulate the inflammatory response (85). These results suggest that sLRP1 may modulate regulatory cytokine expression by macrophages to regulate inflammation or monocyte chemotaxis.
Furthermore, sLRP1 has been demonstrated to be associated with inflammation (85, 88, 89), and increased levels of circulating sLRP1 have been observed shown to be increased in patients with rheumatoid arthritis (RA) or systemic lupus erythematosus (SLE) (85). Several studies have also shown that sLRP1 can mediate Aβ clearance from the brain to the bloodstream via transcytosis across the BBB (90–92), leading to the proposal that sLRP1 could also be used as a biomarker for Alzheimer's disease (93). In addition, the circulating sLRP1 concentration has been reported to be significantly higher in patients with severe hypercholesterolemia than in those with moderate hypercholesterolemia and normocholesterolemic controls. Moreover, as a robust and direct association between sLRP1 and lipid parameters, sLRP1 may be useful as a biomarker for atherosclerosis (94). Another study revealed that sLRP1 is a novel biomarker for the P2Y12 receptor expression, which can aggravate atherosclerosis, in atherosclerotic plaques (95). However, additional studies are needed to confirm the function of sLRP1 in vivo.
Distribution of LRP1
LRP1 is ubiquitously expressed by multiple cells, including VSMCs (17, 96, 97), macrophages (17, 96, 97), hepatocytes (30, 67, 97), epithelial cells (18), retinal Müller glial cells (18), neurons (90, 92, 97), astrocytes (97), fibroblasts (96, 97), adipocytes (54, 98), tumor cells (68), endothelial cells (96, 99), neutrophils (100) and T cells (101). In this review, we primarily discuss the role of LRP1 primarily in cells associated with atherosclerosis.
Ligands Binding to LRP1
Through its ECD, LRP1 can bind with high affinity and internalize more than 75 distinct, structurally and functionally unrelated ligands, such as proteins involved in lipoprotein metabolism and transport (30, 31, 59, 102–105), proteins involved in AD (59, 90, 91, 106), proteases and protease/inhibitor complexes (27, 31, 34, 57, 107–120), growth factors (121–123), extracellular matrix proteins (124–126), infection (127–130), transcription activation (131), chaperones (132–136), blood coagulation (137, 138) and others (103, 139–157) (Table 1). In addition, these ligands may compete with each other for binding, such as the receptor-associated protein (RAP), a 39-kDa molecular chaperone (77), which can tightly bind to and arrest the binding to LRP1 of some ligands, including tissue-type plasminogen activator (t-PA), thrombospondin (TSP), plasminogen activator inhibitor-1 (PAI-1), midkine (MK), β2-integrins and connective tissue growth factor (CTGF) (100, 122, 123, 133, 158, 159). LRP1 can regulate the cell surface abundance of other membrane proteins, some of which have cell signaling activity, by binding and facilitating their delivery to lysosomes for subsequent degradation (87, 160, 161). Thus, based on the broad spectrum of ligands that it recognizes, LRP1 participates in multiple physiological and pathological processes, including lipid and glucose metabolism, protein degradation, wound healing and tissue repair, cell differentiation, hepatic steatosis, kidney fibrosis, acute respiratory distress syndrome (ARDS), Alzheimer's disease, tumor growth and progression, atherosclerosis, and inflammation (10, 64, 68, 159, 162). The LRP1 cytoplasmic domain can also interact with numerous signaling adapter proteins (163–167) (Table 2), including Shc, disabled protein 1 (Dab1) and Fe65, which are involved in directing cellular trafficking and in cell signaling events.
The Dual Roles of LRP1 in Animal Models of Atherosclerosis
Numerous genetic studies have demonstrated the dual roles of LRP1 in atherosclerosis-related cells in different animal models (Table 3). LRP1 gene deletion promotes the progression of atherosclerosis for progressive plaques, while promotes the regression of atherosclerosis for established plaques. The generation and analysis of animal models with receptor gene deletion have elucidated important functions of LRP1 in lipoprotein metabolism and significantly advanced our understanding of the pathophysiological process in patients with lipid disorder.
LRP1 Gene Deletion Facilitates the Development of Atherosclerotic Lesions
The effect of LRP1 on the progression of atherosclerosis has been tested in ApoE–/– and LDLR–/– mice, with the results showing that atherosclerosis development was enhanced in ApoE–/–, LDLR–/– and ApoE/LDLR double knockout mice harboring LRP gene deletions. Hu et al. reported that macrophage-specific LRP-deficient mice in an ApoE/LDLR double-deficient background showed a 1.8-fold increase in total atherosclerotic lesion area in the aortic root that was accompanied by a 1.7-fold increase in collagen content and a 2.3-fold decrease in the number of CD3+ T cells in lesions (37). Similarly, another study showed that macrophage LRP deletion in the LDLR-deficient mouse model enhanced atherosclerosis development and increased monocyte chemoattractant protein type-1 (MCP-1), TNFα, and matrix metalloproteinase-9 (MMP-9) levels as well as proximal aorta macrophage cellularity (36). Another study showed a similar result that specific deletion of macrophage LRP1 in the ApoE deficient mice increased atherosclerosis, which is concomitant with the accumulation of apoptotic cells and proinflammatory monocytes in lesions (39). Moreover, the inactivation of LRP1 in VSMCs in LDLR–/– mice resulted in disruption of the elastic layer and marked susceptibility to atherosclerosis together with platelet-derived growth factor (PDGF) signaling pathway overactivation (14, 121). Furthermore, LRP1 deficiency in macrophages led to an increase in cell death and inflammation and abolished the antiatherosclerotic benefits of the antitumor necrosis factor-α (TNFα) inhibitor adalimumab (63, 168). A similar result was observed in adipocytes, where adipocyte-specific LRP1 knockout (adLRP1–/–) mice fed a western diet for 16 weeks exhibited a 3-fold increase in atherosclerosis and enhanced inflammation in adipose tissues compared to adLRP1+/+ mice (98).
LRP1 Gene Deletion Accelerates Atherosclerosis Regression in Mice
In 2018, Paul et al. reported that macrophages (MΦLRP1–/–) can promote atherosclerosis regression independent of plasma lipid levels, increase reverse cholesterol transport (RCT) and cause selective loss of inflammatory M1 macrophages. In this study, ApoE–/– mice were fed a high-fat diet for 12 weeks, and then reconstituted with bone marrow from apoE-producing wildtype (WT) or MΦLRP1–/– mice, then fed a chow diet for 10 weeks. The results showed that MΦLRP1–/– recipients showed 13% smaller plaques, 1.4-fold higher reverse cholesterol transport (RCT), 36% fewer M1 macrophages and 2.5-fold more CCR7+ macrophages in the plaques than those of WT recipients (169).
Molecular Mechanism of LRP1 in Atherosclerosis-Associated Cells
LRP1 in VSMCs
VSMCs are the primary cell type in the vessel wall and a major component of atherosclerotic plaques at all stages (170, 171). VSMCs can also generate extracellular matrix to form the fibrous cap and hence stabilize plaques, yet aberrant VSMCs proliferation promotes atherosclerotic plaque formation (9). The results of some studies indicated that in VSMCs, LRP1 helps to suppress atherosclerosis by inhibiting the platelet-derived growth factor (PDGF) signaling pathway (14, 121). However, the opposite result has been observed in other studies, indicating that LRP1 mediates aggLDL uptake to induce high intracellular cholesteryl ester accumulation in VSMCs, causing the formation of VSMC-derived foam cells and promoting atherosclerosis progression (44–46). Thus, in VSMCs, LRP1 may exert two different and opposing effects on atherosclerosis (Figure 2A).
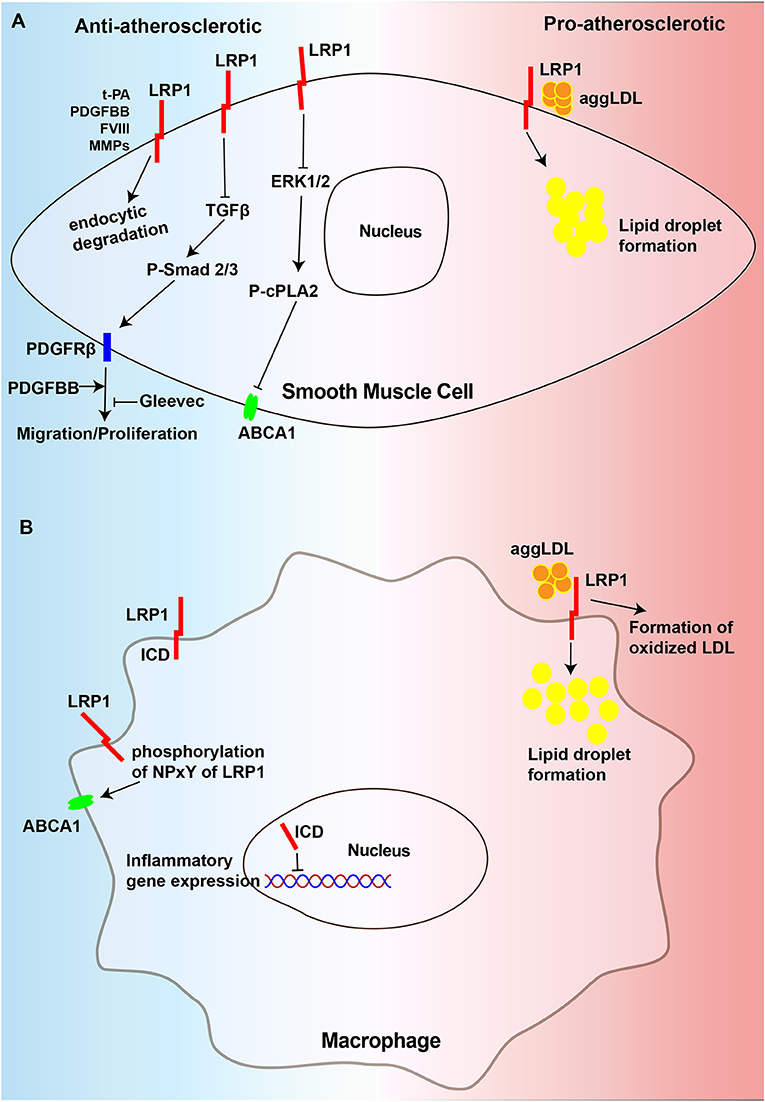
Figure 2. Diagram of the mechanism of regulating atherosclerosis of LRP1 in VSMCs and macrophages. (A) LRP1 in VSMCs could degrade extracellular PDFGBB, MMPs, t-PA, and FVIII, which are atherogenic factors, in the way of endocytosis to play the role of anti-atherosclerosis. It could inhibit migration and proliferation of VSMCs to resist atherosclerosis by preventing the activation of TGFβ/Smad2/3/PDGFRβ signaling pathway. Gleevec could also has the effect to inhibit PDGFRβ. Additionally, LRP1 inhibit the activation of ERK1/2 which could suppresses the expression of ABCA1 by promoting phosphorylation of cPLA2. In contrast, LRP1 in VSMCs accelerates the development of atherosclerosis by promoting the uptake of aggLDL and the formation of foam cells. (B) The LRP1 can also be cleaved by γ-secretase to release a 25-kDa β-chain fragment, which translocates to the nucleus to suppress inflammatory gene expression. The phosphorylation of NPxY of LRP1 ICD promotes the expression of ABCA1. Nevertheless, LRP1 in macrophages accelerates the development of atherosclerosis by promoting the uptake of aggLDL and the formation of oxidized LDL. AS, atherosclerosis, ABCA1, ATP-binding cassette transporter A1, cPLA2, cytosolic phospholipase A2.
LRP1 in VSMCs Protects Against Atherosclerosis
VSMCs are induced to proliferate and migrate from the media to the intima, contributing to the development of atherosclerosis and restenosis under pathological conditions (9, 32, 172). The binding of PDGF, a potent mitogen for fibroblasts and VSMCs, to PDGF receptor β (PDGFRβ) activates a signaling pathway that promotes VSMs growth and migration, which are crucial in atherosclerosis and neointima formation and LRP1 has been proven to suppress this process through incompletely understood mechanisms (14, 24, 33, 121, 173–175). Studies have elegantly demonstrated this effect via the generation of smLRP1–/– mice on a background of LDLR deficiency, showing that smLRP1–/–/LDLR–/– mice are not only more susceptible to cholesterol-induced atherosclerosis than LDLR–/– mice but also exhibit PDGFR overexpression and increased phosphorylation of Smad2, a downstream component of the TGFβ pathway (14, 121). Moreover, the PDGF-BB pathway has previously been described as a target of the TGFβ signaling pathway (176–178). In addition, LRP1 is identical to TGFβ receptor (V), which is a member of the TGFβ receptor superfamily and is expressed on the cell surface together with TGFβ receptors I, II, and III (179). Therefore, the TGFβ activation pathway in the absence of LRP1 could further activate the PDGF-BB signaling pathway by increasing PDGFRβ expression in the arterial wall, promoting the formation of atherosclerotic lesions. Indeed, the vascular pathology observed in smLRP1–/–/LDLR–/– mice was significantly improved by blockade of the PDGF or TGFβ receptor signaling cascades with the tyrosine kinase inhibitor Gleevec (121). Overall, the results of these studies reveal that in VSMCs, LRP1 plays a major role in maintaining the integrity of the vascular wall and reducing atherosclerosis by suppressing the PDGFRβ and TGFβ signaling pathways.
The role of the intracellular NPxYxxL motif of the LRP1-ICD in the development of atherosclerosis has attracted increasing attention. One study has thoroughly demonstrated that inactivation of the LRP1 NPxYxxL motif in LDLR–/– mice showed a significant 1.5-fold increase in the development of atherosclerosis compared to that observed in LDLR–/– control mice. MMP2 activity, which could be degraded by lysosomal proteases after being endocytically taken by LRP1 (113, 180), as well as could facilitate VSMC migration and plaque rupture by degrading the extracellular matrix (181, 182), showed a significant 2.7-fold increase in the aortas of NPxYxxL-inactivated mice. The results of this study also indicated that there was a significant 2-fold increase in the number of apoptotic cells relative to the plaque size in mice with the NPxYxxL-inactivation mice, which was caused by the secretion of the proapoptotic cytokine TNF-α. Therefore, we can conclude that the intracellular NPxYxxL motif of the LRP1-ICD is essential for the atheroprotective role of LRP1 (183). LRP1 can also protect against atherosclerosis by regulating the expression of ATP-binding cassette transporter A1 (ABCA1). The results of multiple studies have indicated that increased mitogenic signaling in the absence of LRP1 can regulate ERK1/2 activation, leading to increased cytosolic phospholipase A2 (cPLA2) phosphorylation, which in turn promotes the production of arachidonic acid (14, 184, 185), a suppressor of LXR-driven ABCA1 expression (186). This cascade reduces cholesterol efflux from VSMCs and promotes the formation of foam cells (187). In summary, LRP1 plays an antiatherosclerotic role in VSMCs, and its absence promotes atherosclerosis development (Figure 2A).
LRP1 in VSMCs Promotes the Development of Atherosclerosis
In addition to protecting against atherosclerosis, many studies have also shown that LRP1 overexpression is associated with atherosclerosis progression in both animal (188) and human models of atherosclerosis (17, 97, 189). LRP1 can promote the formation of VSMC-derived foam cells, leading to the progression of atherosclerosis. In addition, its expression is upregulated by lipid accumulation during the progression of atherosclerotic lesions in humans, and VSMCs derived from advanced atherosclerotic plaques show higher intracellular lipid deposition than those from less-advanced plaques due to their higher LRP1 expression levels (190). In addition, the results of another study also indicated that the transient receptor potential vanilloid type-1 (TRPV1) activation-induced decrease in LRP1 expression reduces lipid uptake by VSMCs (191). Several studies have shown that LRP1 mediates the uptake of aggLDL and induces adipose differentiation-related protein (ADRP) overexpression, leading to an increase in intracellular lipid deposition in VSMCs. ADRP is localized on the surface monolayer of lipid droplets and is considered a specific marker of lipid droplet formation (41, 42, 44–46, 189, 192, 193). Moreover, aggLDL can further upregulate LRP1 expression in VSMCs in a time- and dose-dependent manner (48). This positive feedback mechanism is highly efficient in promoting the formation of VSMCs foam cells. The formation of VSMC-derived foam cells, a main proatherogenic mechanism of LRP1, is associated with atherosclerotic lesion progression. Therefore, in VSMCs, LRP1 can also promote atherosclerosis development by promoting the formation of foam cells (Figure 2A).
LRP1 in Macrophages
Macrophages are the most abundant type of immune cells in atherosclerotic lesions, playing an important role in all stages of atherosclerosis, from the formation of atherosclerotic lesions to plaque rupture (194). Lipoprotein receptors in macrophages can accelerate the progression of atherosclerosis by facilitating the uptake of atherogenic particles, such as oxidized lipoproteins, and inducing vascular inflammation (172, 195, 196). However, the role of macrophage LRP1 in atherosclerosis is controversial, as macrophage LRP1 not only protects against but also promotes atherosclerosis (Figure 2B).
LRP1 in Macrophages Protects Against the Development of Atherosclerosis
Macrophage LRP1 has been shown to exert atheroprotective effects. However, mice macrophage-specific LRP deficiency in an apoE/LDLR double-deficient background did not exhibit altered plasma lipid levels or plasma lipoprotein profiles but did exhibit an increase in the total atherosclerotic lesion area (37). Additionally, Overton et al. showed that LDLR–/– mice transplanted with bone marrow from macLRP1–/– mice were 40% greater in proximal aorta lesions than that observed in high-fat diet-fed mice transplanted with control bone marrow, and accompanied by the increase in proinflammatory factors, such as monocyte chemoattractant protein type-1 (MCP-1) and TNF-α, but en face analysis of the distal aorta showed no significant difference likely attributable to the shor-term experiments (36). Furthermore, atherosclerotic lesions in mice lacking LRP1 expression in macrophages are characterized by increased apoptosis, suggesting that LRP1 prevents atherosclerosis by promoting efferocytosis to remove apoptotic cells from plaques, which is also manifested as inhibition of the p-AKT survival pathway and the promotion of inflammation with increased IL-1, IL-6 and TNF-α expression (63). Furthermore, LDLR–/– mice that received lethal irradiation and were reconstituted with bone marrow from MΦLRP1–/– mice fed on a western-type diet for 10 weeks showed increased necrosis and apoptosis, defective efferocytosis and increased inflammation in the lesions (168). Another study showed that the specific deletion of macrophage LRP1 in ApoE–/– mice promoted atherogenesis and apoptotic cell accumulation in lesions, partially due to decreased efferocytosis and increased lesion necrosis compared to ApoE–/– mice. In this research, the authors also indicated that 88% more lipid-stainable lesion areas in the proximal aorta and a 138% increase in MOMA-2 stainable intimal macrophages in LDLR–/– mice receiving ApoE–/– macLRP1–/– bone marrow compared with those that receiving ApoE–/– marrow. Aorta en face lesions were not significantly different between ApoE–/– macLRP1–/– and ApoE–/– BM-recipient mice, which is consistent with the previous report in 2007 (39). In addition, LRP-1 can also affect macrophage polarization and promote polarization toward the anti-inflammatory M2 functional phenotype (197), leading to an increase in the number of anti-inflammatory M2 macrophages in lesions. In another study, LRP1 was proposed to inhibit cellular inflammatory responses in an adipocyte-specific LRP1-deficient mouse model (98). Another potential mechanism by which LRP1 affects inflammation in macrophages may be through the direct regulation of inflammatory gene transcription. In cultured macrophages, the LRP1-ICD can be cleaved from the plasma membrane by γ-secretase upon induction by inflammatory mediators, such as LPS and interferon-γ, and then translocate into the nucleus, where it promotes the nuclear export and proteasomal degradation of interferon regulatory factor 3, thereby limiting the expression of the proinflammatory genes in cultured fibroblasts and macrophages (73, 85). Apart from regulating inflammation, a study using a knock-in mouse model of LRP1Y63F, in which the tyrosine in the distal NPxY motif was replaced with phenylalanine to prevent NPxY phosphorylation of LRP1, revealed that LRP1 not only regulates the expression of ABCA1, the major cholesterol exporter, to maintain cholesterol efflux but also integrates cellular cholesterol homeostasis with inflammation and efferocytosis (15). In summary, macrophage LRP1 may primarily exert an atheroprotective effect mainly by decreasing inflammation, facilitating efferocytosis and promoting cholesterol efflux (Figure 2B).
LRP1 in Macrophages Promotes the Development of Atherosclerosis
Evidence from several in vitro studies shows that LRP1 has proatherogenic properties in macrophages. First, LRP1 is upregulated during macrophage foam cell formation (198). Second, macrophage LRP1 has been shown to play a vital role in the translocation of 12/15-lipoxygenase, promoting the formation of oxidized LDL (199, 200). Third, LRP1 was shown to mediate the internalization of aggLDL and apoE-rich atherogenic lipoproteins, along with LDLR, into macrophages (46, 201, 202). Through the above mechanisms, the accumulation of lipids and macrophage-derived foam cells increases, leading to the progression of atherosclerosis. In addition, the results from an animal study proved that mice with macrophages lacking LRP1 (MΦLRP1–/– mice) exhibit accelerated regression of atherosclerosis and enhanced reverse cholesterol transport (RCT), and drive macrophage egress from lesions by inducing expression of the motility receptor CCR7 (169). Therefore, macrophage LRP1 can also lead to atherosclerosis progression by promoting foam cell formation (Figure 2B).
LRP1 in Endothelial Cells
Atherosclerosis is a chronic process initiated by endothelial dysfunction and structural changes (203). Although LRP1 is highly expressed in a variety of cells, its protein expression levels in endothelial cells are low (96, 204). However, LRP1 expression is tightly regulated by various physiological conditions in endothelial cells, reflecting its crucial role in these cells. An elegant study showed that LRP1 regulates hypoxia-mediated angiogenesis by inhibiting PARP-1 activity and suppresses endothelial cell proliferation by preventing cell cycle progression in an oxygen-induced retinopathy (OIR) mouse model (205). Another study also showed that LRP1 can mediate bone morphogenetic protein-binding endothelial regulator (BMPER)-mediated bone morphogenetic protein 4 (BMP4) signaling to regulate endothelial cell migration and angiogenesis (206). Angiogenesis is the process of growing new blood vessels from the existing vascular network that occurs during embryonic development and throughout adulthood, and begins under wound healing and pathological conditions, such as retinopathy. Pathological retinal angiogenesis produces physiologically defective blood vessels, leading to exudation and hemorrhage that threatens vision (207). Moreover, a study showed that LRP1-dependent BMPER signaling is required for LPS-induced nuclear factor of activated T cells 1 (NFATc1) activation to induce acute inflammatory responses in endothelial cells (208), which may cause the initiation of atherosclerosis. Interestingly, LRP1 can also promote peroxisome proliferator-activated receptor-γ (PPARγ) activity by acting as a coactivator to regulate lipid and glucose metabolism in endothelial cells (209). Therefore, it can be used to treat pathological retinal angiogenesis induced by diabetic retinopathy and atherosclerosis by regulating the expression and function of LRP1 in endothelial cells.
LRP1 in Adipocytes
Another cell type with high LRP1 expression and associated with the development of atherosclerosis is adipocytes. An increasing number of studies have shown that adipose tissues, especially those in the perivascular area surrounding the vessel wall, such as the aorta, coronary artery, and carotid artery, also play a vital role in the pathogenesis of atherosclerosis (210, 211). Perivascular adipose tissue (PVAT) is a unique conglomerate of various cell types, including adipocytes, preadipocytes, and mesenchymal stem cells that are embedded in a matrix that is invested with microvessels, and that is important for the maintenance of the vascular structure and the regulation of vascular function and homeostasis (210). The interaction between perivascular adipocytes and vascular wall cells, such as endothelial cells and VSMCs, is essential for normal vascular function, and may be disturbed in diseases such as atherosclerosis. In the early process of hyperlipidemia, atherosclerosis prone animal models and human arteries, PVAT expansion and the production of chemokines near the adventitia of large arteries have been detected, leading to the aggravation of inflammation, which may play a fundamental role in the pathogenesis of the cardiovascular disease (CVD) (212). Adipose tissues have been shown to exhibit increased inflammation with enhanced monocyte–macrophage infiltration and inflammatory gene expression in mice with adipocyte-specific LRP1 knockout (adLRP1–/–) in PVAT, and that mice transplanted with PVAT from adLRP1–/– mice displayed a 3-fold increase in atherosclerosis compared to those transplanted with PVAT from adLRP1+/+ mice after a western diet (98). In addition, mice with LRP1 knocked out in adipocytes (adLRP1–/– mice) exhibited delayed postprandial lipid clearance, reduced fat stores, improved glucose tolerance and decreased body weight compared to wild-type mice (213). Taken together, the results of these studies have added the adipose tissue to the list of anatomic sites where LRP1 expression is important for atheroprotection. Thus, it is possible to prevent the development of atherosclerosis and relieve associated clinical symptoms by regulating LRP1 in adipocytes, including through the use of natural and synthetic LRP1 agonists.
LRP1 in Immune Cells
LRP1 in Neutrophils
LRP1 is also abundantly present in neutrophils, which are the most abundant type of white blood cell in the human circulation and the principal cell type during acute inflammatory reactions (97, 214). The number of circulating neutrophils is a predictor of future adverse cardiovascular events and positively correlates with the size of developing lesions in humans and mice, respectively (215, 216). The expression of endothelial cell adhesion molecules (e.g., E-selectin, P-selectin, and intercellular adhesion molecule-1) increases once the endothelial cell dysfunction is triggered by exposure to irritative stimuli such as hyperlipidemia and proinflammatory cytokines, which then triggers the recruitment of neutrophils. In addition, neutrophils can release chemotactic proteins and proinflammatory mediators to promote monocyte recruitment as well as vascular inflammation, promoting atherosclerosis development. Furthermore, neutrophils can degranulate large amounts of different proteases including matrix metalloproteases (MMPs), myeloperoxidase (MPO) and neutrophil elastase or form neutrophil extracellular traps, leading to a thinner fibrous cap and subsquent plaque rupture (214, 217). The results of one study indicated LRP1 blockade could prevent intravascular adherence and neutrophil recruitment within the ischemic tissue induced by PAI-1 derived from both leukocytes and non-leukocytic sources (158). Another similar study showed that LRP1 synthesized and expressed by neutrophils accounts for the r-tPA-induced migration and degranulation of neutrophils (217), which can aggravate tissue damage and inflammation. Additionally, in a hind limb ischemia model, LRP-1 was observed to act as the receptor of cytokine midkine (MK) to support neutrophil adhesion and trafficking by promoting the high-affinity conformation of β2 integrin, suggesting a role of LRP-1 in acute inflammation (218). Furthermore, another study also demonstrated that LRP1 can bind to β2 integrin complex expressed in neutrophils to regulate the firm adhesion and subsequent transmigration of neutrophils (100). Taken together, the result of these studies indicate that LRP1 in neutrophils primarily plays a role in neutrophil-associated inflammation.
LRP1 in T Cells
T lymphocytes account for another majority of immune cells in human atherosclerotic plaques obtained from endarterectomy (219). A large body of evidence from animal studies suggests that the T-cell response is proatherogenic. Apoe–/– mice crossed with mice lacking the V(D)J recombination-activating protein 1 Rag1 (Rag1–/–) or mice with a severe combined immunodeficiency (SCID) mutation (scid/scid mice) are immunodeficient owing to impaired T-cell and B-cell development and showed reduced atherosclerosis lesions when fed a chow diet (220). Poor LRP1 expression in T cells led to suppression of T cell adhesion to fibronectin and ICAM-1 as well as TCR-induced activation, subsequently suppressing accumulation at sites of inflammation (221). Nevertheless, another study showed that LRP1 inhibites the adhesion of T cells to ICAM-1 and fibronectin via JAK signaling (222). Furthermore, LRP1 on T cells interactes with membrane-associated proteinase 3 (mP3) on neutrophils resulting in inhibition of the inflammatory response (101). In summary, LRP1 on T cells may also play a major role in the inflammatory response associated with T cells.
Conclusions
LRP1 is a large, multifunctional type 1 transmembrane receptor and essential for maintaining basic cellular functions and the development of organisms. Due to its ability to mediate actions of a broad range of ligands, LRP1 participates in the development of multiple degenerative diseases, such as atherosclerosis and Alzheimer's disease. In this review, we summarized the dual and opposing roles of LRP1 in atherosclerosis in vivo and in vitro. The role of LRP1 in VSMCs and macrophages in the development of atherosclerosis is different and opposite in vivo and in vitro, which may be the reason that the complexity of cross-talks among various signal pathways and different cell types and organ systems in vivo. Probably more surprising, for established plaque, the lack of LRP1 expression in macrophages unexpectedly promotes atherosclerosis regression. As a result, the opposite effect of LRP1 involved in the regulation of atherosclerosis might depend on whether the plaque is growing or shrinking. The dual role of LRP1 in mediating the effect of TNF-α on vascular inflammation and in balancing the effects of CD47 on efferocytosis could be involved in this phenomenon (223, 224). However, in endothelial cells and adipocytes, LRP1 may promote resistance to atherosclerosis, primarily by inhibiting inflammation. These results suggest that the functionality of LRP1 is dependent on the cell type in which this receptor is expressed.
Therapeutic Considerations
This review may provide a basis for the development of new therapeutic approaches for atherosclerosis that target LRP1 and its downstream cellular signaling pathways. For example, the C-terminal half of the cluster II CR9 domain (Gly1127–Cys1140) has been reported to be crucial for LRP1-mediated aggLDL binding and internalization in human VSMCs (hVSMCs) (45). Moreover, anti-P3 (Gly1127–Cys1140) antibodies (Abs) that specifically block the LRP1 (CR9) domain have been shown to efficiently prevent LRP1-mediated aggLDL internalization and aggLDL-induced LRP1 upregulation to prevent human macrophages and VSMCs from forming foam cell formation (45, 225). These findings indicate that this strategy could be used to prevent the occurrence and progression of atherosclerosis. In terms of established plaques, inhibiting LRP1 in macrophages with blocking antibodies could accelerate plaque regression, potentially alleviating atherosclerosis and related cardiovascular and cerebrovascular complications. Notably, LRP1 can regulate the endocytic clearance of several MMPs (113, 226), which could degrade the extracellular matrix to promote VSMC migration and thin the fibrous cap, causing plaque rupture and leading to myocardial infarction and stroke (227). Therefore, whether the inhibition of LRP1 would disrupt the signaling pathways involved in the proliferation and migration of VSMCs and proteolysis activity of MMPs will require a more comprehensive understanding.
However, some studies have shown that the natural LRP1 agonists SERPINs, such as α-2-macroglobulin (A2MG), α-1-antitrypsin (AAT), antithrombin III, and synthetic LRP1 agonists (SP16) can be used to treat myocardial ischemia-reperfusion injury by inducing cytoprotective signals in cardiomyocytes, such as the activation of Akt- and ERK1/2-dependent prosurvival as well as anti-inflammatory signaling pathways. Moreover, AAT and SP16 are under clinical development, and also there are no treatment-related serious adverse events or toxicity (228, 229). Thus, the natural and synthetic agonists of LRP1 may be useful in treating atherosclerosis by inhibiting inflammation and promoting vascular cell survival, but additional evidence is needed to elucidate the associated mechanism. Gene therapy could be used to increase LRP1 expression. For instance, it may be possible to use adeno-associated virus-2 (AAV-2) carrying the cDNA of LRP1 or its smaller fragments to increase LRP1 expression in vascular cells to resist atherosclerosis. In a mouse model of Alzheimer's disease, treatment with recombinant ligand-binding domain IV of LRP1 by using an in situ arterial brain perfusion technique for 3 months reduced brain Aβ levels (90). Therefore, gene therapy and recombinant LRP1 could be used to treat and prevent atherosclerosis.
In summary, in this review, LRP1 was shown to participate in a large number of physiological activities as a coreceptor and to interact with many adaptor proteins through its cytoplasmic domain. The understanding of the mechanisms and the further identification of LRP1 partners may open up new ways to treat metabolic diseases, such as lipid metabolism, atherosclerosis, inflammation, Alzheimer's disease and obesity. Futher investigations will most likely uncover even more functions of these receptor beyond those considered here.
Author Contributions
LM and BH designed the review. JC, YS, and SP wrote the manuscript. All authors contributed to the article and approved the submitted version.
Funding
This work was supported by the National Natural Science Foundation of China (no. 81820108010 to BH and no. 81974182 to LM), and the National Key Research and Development Program of China (no. 2018YFC1312200 to BH).
Conflict of Interest
The authors declare that the research was conducted in the absence of any commercial or financial relationships that could be construed as a potential conflict of interest.
Abbreviations
VSMCs, vascular smooth muscle cells; α-2-M, α2-macroglobulin receptor; LRP1, low-density lipoprotein receptor-related protein 1; α-SMA, α-smooth muscle actin; HFD, high-fat diet; BBB, blood-brain barrier; VLDL, very-low-density lipoprotein receptor; aggLDL, aggregated low-density lipoprotein; CEs, cholesteryl esters; ICD, intracellular domain; ECD, extracellular domain; EGF, epidermal growth factor; CRs, cysteine-rich complement-type repeats; cPLA2, cytosolic phospholipase A2; BMPER, bone morphogenetic protein-binding endothelial regulator; NFATc1, nuclear factor of activated T cells 1; MAPK, mitogen-activated protein kinase; IR, insulin receptor; AKT, serine/threonine protein kinase; ERK, extracellular signal-regulated kinase; JNK, c-jun N-terminal kinase; t-PA, tissue-type plasminogen activator; u-PA, urokinase-type plasminogen activator; MMP-9, matrix metalloproteinase-9; MCP-1, monocyte chemoattractant protein type-1; TNFα, tumor necrosis factor-α; TSP, thrombospondin.
References
1. Rosamond W, Flegal K, Furie K, Go A, Greenlund K, Haase N, et al. Heart disease and stroke statistics−2008 update: a report from the American heart association statistics committee and stroke statistics subcommittee. Circulation. (2008) 117:e25–146. doi: 10.1161/CIRCULATIONAHA.107.187998
3. Hansson GK. Inflammation, atherosclerosis, and coronary artery disease. N Engl J Med. (2005) 352:1685–95. doi: 10.1056/NEJMra043430
4. Lopez AD, Mathers CD, Ezzati M, Jamison DT, Murray CJ. Global and regional burden of disease and risk factors, 2001: systematic analysis of population health data. Lancet. (2006) 367:1747–57. doi: 10.1016/S0140-6736(06)68770-9
5. Libby P, Ridker PM, Hansson GK. Progress and challenges in translating the biology of atherosclerosis. Nature. (2011) 473:317–25. doi: 10.1038/nature10146
6. Weber C, Noels H. Atherosclerosis: current pathogenesis and therapeutic options. Nat Med. (2011) 17:1410–22. doi: 10.1038/nm.2538
7. Lim S, Park S. Role of vascular smooth muscle cell in the inflammation of atherosclerosis. BMB Rep. (2014) 47:1–7. doi: 10.5483/BMBRep.2014.47.1.285
8. Libby P, Buring JE, Badimon L, Hansson GK, Deanfield J, Bittencourt MS, et al. Atherosclerosis. Nat Rev Disease Primers. (2019) 5:56. doi: 10.1038/s41572-019-0106-z
9. Bennett MR, Sinha S, Owens GK. Vascular smooth muscle cells in atherosclerosis. Circul Res. (2016) 118:692–702. doi: 10.1161/CIRCRESAHA.115.306361
10. Wujak L, Schnieder J, Schaefer L, Wygrecka M. LRP1: A chameleon receptor of lung inflammation and repair. Matrix Biol. (2018) 68-69:366–81. doi: 10.1016/j.matbio.2017.12.007
11. May P, Herz J, Bock HH. Molecular mechanisms of lipoprotein receptor signalling. Cell Mol Life Sci. (2005) 62:2325–38. doi: 10.1007/s00018-005-5231-z
12. Herz J, Hamann U, Rogne S, Myklebost O, Gausepohl H, Stanley KK. Surface location and high affinity for calcium of a 500-kd liver membrane protein closely related to the LDL-receptor suggest a physiological role as lipoprotein receptor. EMBO J. (1988) 7:4119–27. doi: 10.1002/j.1460-2075.1988.tb03306.x
13. Krieger M, Herz J. Structures and functions of multiligand lipoprotein receptors: macrophage scavenger receptors and LDL receptor-related protein (LRP). Annu Rev Biochem. (1994) 63:601–37. doi: 10.1146/annurev.bi.63.070194.003125
14. Boucher P, Gotthardt M, Li WP, Anderson RG, Herz J. LRP: role in vascular wall integrity and protection from atherosclerosis. Science. (2003) 300:329–32. doi: 10.1126/science.1082095
15. Xian X, Ding Y, Dieckmann M, Zhou L, Plattner F, Liu M, et al. LRP1 integrates murine macrophage cholesterol homeostasis and inflammatory responses in atherosclerosis. eLife. (2017) 6:19. doi: 10.7554/eLife.29292.019
16. Boucher P, Liu P, Gotthardt M, Hiesberger T, Anderson RG, Herz J. Platelet-derived growth factor mediates tyrosine phosphorylation of the cytoplasmic domain of the low Density lipoprotein receptor-related protein in caveolae. J Biol Chem. (2002) 277:15507–13. doi: 10.1074/jbc.M200428200
17. Luoma J, Hiltunen T, Särkioja T, Moestrup SK, Gliemann J, Kodama T, et al. Expression of alpha 2-macroglobulin receptor/low density lipoprotein receptor-related protein and scavenger receptor in human atherosclerotic lesions. J Clin Investig. (1994) 93:2014–21. doi: 10.1172/JCI117195
18. Actis Dato V, Chiabrando GA. The role of low-density lipoprotein receptor-related protein 1 in lipid metabolism, glucose homeostasis and inflammation. Int J Mol Sci. (2018) 19:1780. doi: 10.3390/ijms19061780
19. Polavarapu R, Gongora MC, Yi H, Ranganthan S, Lawrence DA, Strickland D, et al. Tissue-type plasminogen activator-mediated shedding of astrocytic low-density lipoprotein receptor-related protein increases the permeability of the neurovascular unit. Blood. (2007) 109:3270–8. doi: 10.1182/blood-2006-08-043125
20. Cao C, Lawrence DA, Li Y, Von Arnim CA, Herz J, Su EJ, et al. Endocytic receptor LRP together with tPA and PAI-1 coordinates Mac-1-dependent macrophage migration. EMBO J. (2006) 25:1860–70. doi: 10.1038/sj.emboj.7601082
21. Bown MJ, Jones GT, Harrison SC, Wright BJ, Bumpstead S, Baas AF, et al. Abdominal aortic aneurysm is associated with a variant in low-density lipoprotein receptor-related protein 1. Am J Hum Gene. (2011) 89:619–27. doi: 10.1016/j.ajhg.2011.10.002
22. Teslovich TM, Musunuru K, Smith AV, Edmondson AC, Stylianou IM, Koseki M, et al. Biological, clinical and population relevance of 95 loci for blood lipids. Nature. (2010) 466:707–13. doi: 10.1038/nature09270
23. McCarthy JJ, Parker A, Salem R, Moliterno DJ, Wang Q, Plow EF, et al. Large scale association analysis for identification of genes underlying premature coronary heart disease: cumulative perspective from analysis of 111 candidate genes. J Med Gene. (2004) 41:334–41. doi: 10.1136/jmg.2003.016584
24. Muratoglu SC, Mikhailenko I, Newton C, Migliorini M, Strickland DK. Low density lipoprotein receptor-related protein 1 (LRP1) forms a signaling complex with platelet-derived growth factor receptor-beta in endosomes and regulates activation of the MAPK pathway. J Biol Chem. (2010) 285:14308–17. doi: 10.1074/jbc.M109.046672
25. Kang HS, Kim J, Lee HJ, Kwon BM, Lee DK, Hong SH. LRP1-dependent pepsin clearance induced by 2'-hydroxycinnamaldehyde attenuates breast cancer cell invasion. Int J Biochem Cell Biol. (2014) 53:15–23. doi: 10.1016/j.biocel.2014.04.021
26. Mantuano E, Lam MS, Gonias SL. LRP1 assembles unique co-receptor systems to initiate cell signaling in response to tissue-type plasminogen activator and myelin-associated glycoprotein. J Biol Chem. (2013) 288:34009–18. doi: 10.1074/jbc.M113.509133
27. Kasza A, Petersen HH, Heegaard CW, Oka K, Christensen A, Dubin A, et al. Specificity of serine proteinase/serpin complex binding to very-low-density lipoprotein receptor and alpha2-macroglobulin receptor/low-density-lipoprotein-receptor-related protein. Eur J Biochem. (1997) 248:270–81. doi: 10.1111/j.1432-1033.1997.00270.x
28. Roura S, Cal R, Gálvez-Montón C, Revuelta-Lopez E, Nasarre L, Badimon L, et al. Inverse relationship between raft LRP1 localization and non-raft ERK1,2/MMP9 activation in idiopathic dilated cardiomyopathy: potential impact in ventricular remodeling. Int J Cardiol. (2014) 176:805–14. doi: 10.1016/j.ijcard.2014.07.270
29. Fuentealba RA, Liu Q, Kanekiyo T, Zhang J, Bu G. Low density lipoprotein receptor-related protein 1 promotes anti-apoptotic signaling in neurons by activating Akt survival pathway. J Biol Chem. (2009) 284:34045–53. doi: 10.1074/jbc.M109.021030
30. Rohlmann A, Gotthardt M, Hammer RE, Herz J. Inducible inactivation of hepatic LRP gene by cre-mediated recombination confirms role of LRP in clearance of chylomicron remnants. J Clin Investig. (1998) 101:689–95. doi: 10.1172/JCI1240
31. Espirito Santo SM, Pires NM, Boesten LS, Gerritsen G, Bovenschen N, van Dijk KW, et al. Hepatic low-density lipoprotein receptor-related protein deficiency in mice increases atherosclerosis independent of plasma cholesterol. Blood. (2004) 103:3777–82. doi: 10.1182/blood-2003-11-4051
32. Niu X, Pi SL, Baral S, Xia YP, He QW, Li YN, et al. P2Y12 promotes migration of vascular smooth muscle cells through cofilin dephosphorylation during atherogenesis. Arterioscler Thromb Vasc Biol. (2017) 37:515–24. doi: 10.1161/ATVBAHA.116.308725
33. Basford JE, Moore ZW, Zhou L, Herz J, Hui DY. Smooth muscle LDL receptor-related protein-1 inactivation reduces vascular reactivity and promotes injury-induced neointima formation. Arteriosclerosis Thrombosis Vasc Biol. (2009) 29:1772–8. doi: 10.1161/ATVBAHA.109.194357
34. Muratoglu SC, Belgrave S, Hampton B, Migliorini M, Coksaygan T, Chen L, et al. LRP1 protects the vasculature by regulating levels of connective tissue growth factor and HtrA1. Arteriosclerosis Thrombosis Vasc Biol. (2013) 33:2137–46. doi: 10.1161/ATVBAHA.113.301893
35. Boucher P, Herz J. Signaling through LRP1: protection from atherosclerosis and beyond. Biochem Pharmacol. (2011) 81:1–5. doi: 10.1016/j.bcp.2010.09.018
36. Overton CD, Yancey PG, Major AS, Linton MF, Fazio S. Deletion of macrophage LDL receptor-related protein increases atherogenesis in the mouse. Circul Res. (2007) 100:670–7. doi: 10.1161/01.RES.0000260204.40510.aa
37. Hu L, Boesten LS, May P, Herz J, Bovenschen N, Huisman MV, et al. Macrophage low-density lipoprotein receptor-related protein deficiency enhances atherosclerosis in ApoE/LDLR double knockout mice. Arteriosclerosis Thrombosis Vasc Biol. (2006) 26:2710–5. doi: 10.1161/01.ATV.0000249641.96896.e6
38. Muratoglu SC, Belgrave S, Lillis AP, Migliorini M, Robinson S, Smith E, et al. Macrophage LRP1 suppresses neo-intima formation during vascular remodeling by modulating the TGF-beta signaling pathway. PLoS ONE. (2011) 6:e28846. doi: 10.1371/journal.pone.0028846
39. Yancey PG, Ding Y, Fan D, Blakemore JL, Zhang Y, Ding L, et al. Low-density lipoprotein receptor-related protein 1 prevents early atherosclerosis by limiting lesional apoptosis and inflammatory Ly-6Chigh monocytosis: evidence that the effects are not apolipoprotein E dependent. Circulation. (2011) 124:454–64. doi: 10.1161/CIRCULATIONAHA.111.032268
40. Terrand J, Bruban V, Zhou L, Gong W, El Asmar Z, May P, et al. LRP1 controls intracellular cholesterol storage and fatty acid synthesis through modulation of Wnt signaling. J Biol Chem. (2009) 284:381–8. doi: 10.1074/jbc.M806538200
41. Llorente-Cortés V, Martínez-González J, Badimon L. LDL receptor-related protein mediates uptake of aggregated LDL in human vascular smooth muscle cells. Arteriosclerosis Thrombosis Vasc Biol. (2000) 20:1572–9. doi: 10.1161/01.ATV.20.6.1572
42. Llorente-Cortés V, Otero-Viñas M, Badimon L. Differential role of heparan sulfate proteoglycans on aggregated LDL uptake in human vascular smooth muscle cells and mouse embryonic fibroblasts. Arteriosclerosis Thrombosis Vasc Biol. (2002) 22:1905–11. doi: 10.1161/01.ATV.0000035391.46201.9A
43. Llorente-Cortes V, Otero-Vinas M, Hurt-Camejo E, Martinez-Gonzalez J, Badimon L. Human coronary smooth muscle cells internalize versican-modified LDL through LDL receptor-related protein and LDL receptors. Arterioscler Thromb Vasc Biol. (2002) 22:387–93. doi: 10.1161/hq0302.105367
44. Llorente-Cortés V, Otero-Viñas M, Camino-López S, Costales P, Badimon L. Cholesteryl esters of aggregated LDL are internalized by selective uptake in human vascular smooth muscle cells. Arteriosclerosis Thrombosis Vasc Biol. (2006) 26:117–23. doi: 10.1161/01.ATV.0000193618.32611.8b
45. Costales P, Fuentes-Prior P, Castellano J, Revuelta-Lopez E, Corral-Rodriguez MA, Nasarre L, et al. K Domain CR9 of Low Density Lipoprotein (LDL) Receptor-related Protein 1 (LRP1) is critical for aggregated LDL-induced foam cell formation from human vascular smooth muscle cells. J Biol Chem. (2015) 290:14852–65. doi: 10.1074/jbc.M115.638361
46. Llorente-Cortés V, Royo T, Juan-Babot O, Badimon L. Adipocyte differentiation-related protein is induced by LRP1-mediated aggregated LDL internalization in human vascular smooth muscle cells and macrophages. J Lipid Res. (2007) 48:2133–40. doi: 10.1194/jlr.M700039-JLR200
47. Lillis AP, Muratoglu SC, Au DT, Migliorini M, Lee MJ, Fried SK, et al. LDL Receptor-Related Protein-1 (LRP1) regulates cholesterol accumulation in macrophages. PLoS ONE. (2015) 10:e0128903. doi: 10.1371/journal.pone.0128903
48. Llorente-Cortés V, Otero-Viñas M, Sánchez S, Rodríguez C, Badimon L. Low-density lipoprotein upregulates low-density lipoprotein receptor-related protein expression in vascular smooth muscle cells: possible involvement of sterol regulatory element binding protein-2-dependent mechanism. Circulation. (2002) 106:3104–10. doi: 10.1161/01.CIR.0000041434.28573.0B
49. Khoo JC, Miller E, McLoughlin P, Steinberg D. Enhanced macrophage uptake of low density lipoprotein after self-aggregation. Arteriosclerosis. (1988) 8:348–58. doi: 10.1161/01.ATV.8.4.348
50. Zhang WY, Ishii I, Kruth HS. Plasmin-mediated macrophage reversal of low density lipoprotein aggregation. J Biol Chem. (2000) 275:33176–83. doi: 10.1074/jbc.M908714199
51. Kruth HS. Sequestration of aggregated low-density lipoproteins by macrophages. Curr Opin Lipidol. (2002) 13:483–8. doi: 10.1097/00041433-200210000-00003
52. Cal R, Juan-Babot O, Brossa V, Roura S, Galvez-Monton C, Portoles M, et al. Low density lipoprotein receptor-related protein 1 expression correlates with cholesteryl ester accumulation in the myocardium of ischemic cardiomyopathy patients. J Transl Med. (2012) 10:160. doi: 10.1186/1479-5876-10-160
53. Cal R, Castellano J, Revuelta-Lopez E, Aledo R, Barriga M, Farre J, et al. Low-density lipoprotein receptor-related protein 1 mediates hypoxia-induced very low density lipoprotein-cholesteryl ester uptake and accumulation in cardiomyocytes. Cardiovasc Res. (2012) 94:469–79. doi: 10.1093/cvr/cvs136
54. Masson O, Chavey C, Dray C, Meulle A, Daviaud D, Quilliot D, et al. LRP1 receptor controls adipogenesis and is up-regulated in human and mouse obese adipose tissue. PLoS ONE. (2009) 4:e7422. doi: 10.1371/journal.pone.0007422
55. Bu G, Maksymovitch EA, Nerbonne JM, Schwartz AL. Expression and function of the low density lipoprotein receptor-related protein (LRP) in mammalian central neurons. J Biol Chem. (1994) 269:18521–8. doi: 10.1016/S0021-9258(17)32340-2
56. Gardai SJ, Xiao YQ, Dickinson M, Nick JA, Voelker DR, Greene KE, et al. By binding SIRPalpha or calreticulin/CD91, lung collectins act as dual function surveillance molecules to suppress or enhance inflammation. Cell. (2003) 115:13–23. doi: 10.1016/S0092-8674(03)00758-X
57. Ashcom JD, Tiller SE, Dickerson K, Cravens JL, Argraves WS, Strickland DK. The human alpha 2-macroglobulin receptor: identification of a 420-kD cell surface glycoprotein specific for the activated conformation of alpha 2-macroglobulin. J Cell Biol. (1990) 110:1041–8. doi: 10.1083/jcb.110.4.1041
58. Moestrup SK, Gliemann J. Purification of the rat hepatic alpha 2-macroglobulin receptor as an approximately 440-kDa single chain protein. J Biol Chem. (1989) 264:15574–7. doi: 10.1016/S0021-9258(19)84870-6
59. Beisiegel U, Weber W, Ihrke G, Herz J, Stanley KK. The LDL-receptor-related protein, LRP, is an apolipoprotein E-binding protein. Nature. (1989) 341:162–4. doi: 10.1038/341162a0
60. Herz J, Clouthier DE, Hammer RE. LDL receptor-related protein internalizes and degrades uPA-PAI-1 complexes and is essential for embryo implantation. Cell. (1992) 71:411–21. doi: 10.1016/0092-8674(92)90511-A
61. Franchini M, Montagnana M. Low-density lipoprotein receptor-related protein 1: new functions for an old molecule. Clin Chem Lab Med. (2011) 49:967–70. doi: 10.1515/CCLM.2011.154
62. Herz J, Kowal RC, Goldstein JL, Brown MS. Proteolytic processing of the 600 kd low density lipoprotein receptor-related protein (LRP) occurs in a trans-Golgi compartment. EMBO J. (1990) 9:1769–76. doi: 10.1002/j.1460-2075.1990.tb08301.x
63. Yancey PG, Blakemore J, Ding L, Fan D, Overton CD, Zhang Y, et al. Macrophage LRP-1 controls plaque cellularity by regulating efferocytosis and Akt activation. Arteriosclerosis Thrombosis Vasc Biol. (2010) 30:787–95. doi: 10.1161/ATVBAHA.109.202051
64. Schnieder J, Mamazhakypov A, Birnhuber A, Wilhelm J, Kwapiszewska G, Ruppert C, et al. Loss of LRP1 promotes acquisition of contractile-myofibroblast phenotype and release of active TGF-β1 from ECM stores. Matrix Biol. (2020) 88:69–88. doi: 10.1016/j.matbio.2019.12.001
65. Bres EE, Faissner A. Low density receptor-related protein 1 interactions with the extracellular matrix: more than meets the eye. Front Cell Dev Biol. (2019) 7:31. doi: 10.3389/fcell.2019.00031
66. Lillis AP, Greenlee MC, Mikhailenko I, Pizzo SV, Tenner AJ, Strickland DK, et al. Murine low-density lipoprotein receptor-related protein 1 (LRP) is required for phagocytosis of targets bearing LRP ligands but is not required for C1q-triggered enhancement of phagocytosis. J Immunol. (2008) 181:364–73. doi: 10.4049/jimmunol.181.1.364
67. Moon JH, Kim HJ, Kim HM, Choi SH, Lim S, Park YJ, et al. Decreased expression of hepatic low-density lipoprotein receptor-related protein 1 in hypothyroidism: a novel mechanism of atherogenic dyslipidemia in hypothyroidism. Thyroid. (2013) 23:1057–65. doi: 10.1089/thy.2012.0457
68. Gonias SL, Campana WM. LDL receptor-related protein-1: a regulator of inflammation in atherosclerosis, cancer, and injury to the nervous system. Am J Pathol. (2014) 184:18–27. doi: 10.1016/j.ajpath.2013.08.029
69. Willnow TE, Moehring JM, Inocencio NM, Moehring TJ, Herz J. The low-density-lipoprotein receptor-related protein (LRP) is processed by furin in vivo and in vitro. Biochem J. (1996) 313:71–6. doi: 10.1042/bj3130071
70. Bu G, Sun Y, Schwartz AL, Holtzman DM. Nerve growth factor induces rapid increases in functional cell surface low density lipoprotein receptor-related protein. J Biol Chem. (1998) 273:13359–65. doi: 10.1074/jbc.273.21.13359
71. Li Y, Lu W, Marzolo MP, Bu G. Differential functions of members of the low density lipoprotein receptor family suggested by their distinct endocytosis rates. The Journal of biological chemistry. (2001) 276:18000–6. doi: 10.1074/jbc.M101589200
72. van der Geer P. Phosphorylation of LRP1: regulation of transport and signal transduction. Trends Cardiovasc Med. (2002) 12:160–5. doi: 10.1016/S1050-1738(02)00154-8
73. Zurhove K, Nakajima C, Herz J, Bock HH, May P. Gamma-secretase limits the inflammatory response through the processing of LRP1. Sci Signal. (2008) 1:ra15. doi: 10.1126/scisignal.1164263
74. May P, Reddy YK, Herz J. Proteolytic processing of low density lipoprotein receptor-related protein mediates regulated release of its intracellular domain. J Biol Chem. (2002) 277:18736–43. doi: 10.1074/jbc.M201979200
75. Emonard H, Théret L, Bennasroune AH, Dedieu S. Regulation of LRP-1 expression: make the point. Pathol Biol (Paris). (2014) 62:84–90. doi: 10.1016/j.patbio.2014.02.002
76. Lillis AP, Van Duyn LB, Murphy-Ullrich JE, Strickland DK. LDL receptor-related protein 1: unique tissue-specific functions revealed by selective gene knockout studies. Physiol Rev. (2008) 88:887–918. doi: 10.1152/physrev.00033.2007
77. Herz J, Strickland DK. LRP: a multifunctional scavenger and signaling receptor. J Clin Investig. (2001) 108:779–84. doi: 10.1172/JCI200113992
78. Selvais C, D'Auria L, Tyteca D, Perrot G, Lemoine P, Troeberg L, et al. Cell cholesterol modulates metalloproteinase-dependent shedding of low-density lipoprotein receptor-related protein-1 (LRP-1) and clearance function. FASEB J. (2011) 25:2770–81. doi: 10.1096/fj.10-169508
79. von Einem B, Schwanzar D, Rehn F, Beyer AS, Weber P, Wagner M, et al. The role of low-density receptor-related protein 1 (LRP1) as a competitive substrate of the amyloid precursor protein (APP) for BACE1. Experi Neurol. (2010) 225:85–93. doi: 10.1016/j.expneurol.2010.05.017
80. Zlokovic BV, Deane R, Sagare AP, Bell RD, Winkler EA. Low-density lipoprotein receptor-related protein-1: a serial clearance homeostatic mechanism controlling Alzheimer's amyloid β-peptide elimination from the brain. J Neurochem. (2010) 115:1077–89. doi: 10.1111/j.1471-4159.2010.07002.x
81. Quinn KA, Grimsley PG, Dai YP, Tapner M, Chesterman CN, Owensby DA. Soluble low density lipoprotein receptor-related protein (LRP) circulates in human plasma. J Biol Chem. (1997) 272:23946–51. doi: 10.1074/jbc.272.38.23946
82. Liu Q, Zhang J, Tran H, Verbeek MM, Reiss K, Estus S, et al. LRP1 shedding in human brain: roles of ADAM10 and ADAM17. Mol Neurodegen. (2009) 4:17. doi: 10.1186/1750-1326-4-17
83. von Arnim CA, Kinoshita A, Peltan ID, Tangredi MM, Herl L, Lee BM, et al. The low density lipoprotein receptor-related protein (LRP) is a novel beta-secretase (BACE1) substrate. J Biol Chem. (2005) 280:17777–85. doi: 10.1074/jbc.M414248200
84. Selvais C, Gaide Chevronnay HP, Lemoine P, Dedieu S, Henriet P, Courtoy PJ, et al. Metalloproteinase-dependent shedding of low-density lipoprotein receptor-related protein-1 ectodomain decreases endocytic clearance of endometrial matrix metalloproteinase-2 and−9 at menstruation. Endocrinology. (2009) 150:3792–9. doi: 10.1210/en.2009-0015
85. Gorovoy M, Gaultier A, Campana WM, Firestein GS, Gonias SL. Inflammatory mediators promote production of shed LRP1/CD91, which regulates cell signaling and cytokine expression by macrophages. J Leukocyte Biol. (2010) 88:769–78. doi: 10.1189/jlb.0410220
86. Quinn KA, Pye VJ, Dai YP, Chesterman CN, Owensby DA. Characterization of the soluble form of the low density lipoprotein receptor-related protein (LRP). Experi Cell Res. (1999) 251:433–41. doi: 10.1006/excr.1999.4590
87. May P, Woldt E, Matz RL, Boucher P. The LDL receptor-related protein (LRP) family: an old family of proteins with new physiological functions. Ann Med. (2007) 39:219–28. doi: 10.1080/07853890701214881
88. Brifault C, Gilder AS, Laudati E, Banki M, Gonias SL. Shedding of membrane-associated LDL receptor-related protein-1 from microglia amplifies and sustains neuroinflammation. J Biol Chem. (2017) 292:18699–712. doi: 10.1074/jbc.M117.798413
89. Wygrecka M, Wilhelm J, Jablonska E, Zakrzewicz D, Preissner KT, Seeger W, et al. Shedding of low-density lipoprotein receptor–related protein-1 in acute respiratory distress syndrome. Am J Respir Crit Care Med. (2011) 184:438–48. doi: 10.1164/rccm.201009-1422OC
90. Sagare A, Deane R, Bell RD, Johnson B, Hamm K, Pendu R, et al. Clearance of amyloid-beta by circulating lipoprotein receptors. Nat Med. (2007) 13:1029–31. doi: 10.1038/nm1635
91. Sagare AP, Deane R, Zetterberg H, Wallin A, Blennow K, Zlokovic BV. Impaired lipoprotein receptor-mediated peripheral binding of plasma amyloid-β is an early biomarker for mild cognitive impairment preceding Alzheimer's disease. J Alzheimer's Dis. (2011) 24:25–34. doi: 10.3233/JAD-2010-101248
92. Sagare AP, Deane R, Zlokovic BV. Low-density lipoprotein receptor-related protein 1: a physiological Aβ homeostatic mechanism with multiple therapeutic opportunities. Pharmacol Therap. (2012) 136:94–105. doi: 10.1016/j.pharmthera.2012.07.008
93. Ouwens DM, van Duinkerken E, Schoonenboom SN, Herzfeld de Wiza D, Klein M, van Golen L, et al. Cerebrospinal fluid levels of Alzheimer's disease biomarkers in middle-aged patients with type 1 diabetes. Diabetologia. (2014) 57:2208–14. doi: 10.1007/s00125-014-3333-6
94. de Gonzalo-Calvo D, Cenarro A, Martínez-Bujidos M, Badimon L, Bayes-Genis A, Ordonez-Llanos J, et al. Circulating soluble low-density lipoprotein receptor-related protein 1 (sLRP1) concentration is associated with hypercholesterolemia: a new potential biomarker for atherosclerosis. Int J Cardiol. (2015) 201:20–9. doi: 10.1016/j.ijcard.2015.07.085
95. Chen J, Pi S, Yu C, Shi H, Liu Y, Guo X, et al. sLRP1 (Soluble Low-Density Lipoprotein Receptor-Related Protein 1): A novel biomarker for P2Y12 (P2Y Purinoceptor 12) receptor expression in atherosclerotic plaques. Arteriosclerosis Thrombosis Vasc Biol. (2020) 40:e166–79. doi: 10.1161/ATVBAHA.120.314350
96. Strickland DK, Au DT, Cunfer P, Muratoglu SC. Low-density lipoprotein receptor-related protein-1: role in the regulation of vascular integrity. Arteriosclerosis Thrombosis Vasc Biol. (2014) 34:487–98. doi: 10.1161/ATVBAHA.113.301924
97. Moestrup SK, Gliemann J, Pallesen G. Distribution of the alpha 2-macroglobulin receptor/low density lipoprotein receptor-related protein in human tissues. Cell Tissue Res. (1992) 269:375–82. doi: 10.1007/BF00353892
98. Konaniah ES, Kuhel DG, Basford JE, Weintraub NL, Hui DY. Deficiency of LRP1 in mature adipocytes promotes diet-induced inflammation and atherosclerosis-brief report. Arteriosclerosis Thrombosis Vasc Biol. (2017) 37:1046–9. doi: 10.1161/ATVBAHA.117.309414
99. de Gonzalo-Calvo D, Colom C, Vilades D, Rivas-Urbina A, Moustafa AH, Pérez-Cuellar M, et al. Soluble LRP1 is an independent biomarker of epicardial fat volume in patients with type 1 diabetes mellitus. Sci Rep. (2018) 8:1054. doi: 10.1038/s41598-018-19230-3
100. Spijkers PP, da Costa Martins P, Westein E, Gahmberg CG, Zwaginga JJ, Lenting PJ. LDL-receptor-related protein regulates beta2-integrin-mediated leukocyte adhesion. Blood. (2005) 105:170–7. doi: 10.1182/blood-2004-02-0498
101. Yang TH, St John LS, Garber HR, Kerros C, Ruisaard KE, Clise-Dwyer K, et al. Membrane-associated proteinase 3 on granulocytes and acute myeloid leukemia inhibits T cell proliferation. J Immunol. (2018) 201:1389–99. doi: 10.4049/jimmunol.1800324
102. Beisiegel U, Weber W, Bengtsson-Olivecrona G. Lipoprotein lipase enhances the binding of chylomicrons to low density lipoprotein receptor-related protein. Proc Natl Acad Sci USA. (1991) 88:8342–6. doi: 10.1073/pnas.88.19.8342
103. Hiesberger T, Hüttler S, Rohlmann A, Schneider W, Sandhoff K, Herz J. Cellular uptake of saposin (SAP) precursor and lysosomal delivery by the low density lipoprotein receptor-related protein (LRP). EMBO J. (1998) 17:4617–25. doi: 10.1093/emboj/17.16.4617
104. Kounnas MZ, Chappell DA, Wong H, Argraves WS, Strickland DK. The cellular internalization and degradation of hepatic lipase is mediated by low density lipoprotein receptor-related protein and requires cell surface proteoglycans. J Biol Chem. (1995) 270:9307–12. doi: 10.1074/jbc.270.16.9307
105. Foley EM, Gordts P, Stanford KI, Gonzales JC, Lawrence R, Stoddard N, et al. Hepatic remnant lipoprotein clearance by heparan sulfate proteoglycans and low-density lipoprotein receptors depend on dietary conditions in mice. Arteriosclerosis Thrombosis Vasc Biol. (2013) 33:2065–74. doi: 10.1161/ATVBAHA.113.301637
106. Ulery PG, Beers J, Mikhailenko I, Tanzi RE, Rebeck GW, Hyman BT, et al. Modulation of beta-amyloid precursor protein processing by the low density lipoprotein receptor-related protein (LRP). Evidence that LRP contributes to the pathogenesis of Alzheimer's disease. J Biol Chem. (2000) 275:7410–5. doi: 10.1074/jbc.275.10.7410
107. Hussain MM, Strickland DK, Bakillah A. The mammalian low-density lipoprotein receptor family. Ann Rev Nutr. (1999) 19:141–72. doi: 10.1146/annurev.nutr.19.1.141
108. Kounnas MZ, Church FC, Argraves WS, Strickland DK. Cellular internalization and degradation of antithrombin III-thrombin, heparin cofactor II-thrombin, and alpha 1-antitrypsin-trypsin complexes is mediated by the low density lipoprotein receptor-related protein. J Biol Chem. (1996) 271:6523–9. doi: 10.1074/jbc.271.11.6523
109. Makarova A, Mikhailenko I, Bugge TH, List K, Lawrence DA, Strickland DK. The low density lipoprotein receptor-related protein modulates protease activity in the brain by mediating the cellular internalization of both neuroserpin and neuroserpin-tissue-type plasminogen activator complexes. J Biol Chem. (2003) 278:50250–8. doi: 10.1074/jbc.M309150200
110. Conese M, Olson D, Blasi F. Protease nexin-1-urokinase complexes are internalized and degraded through a mechanism that requires both urokinase receptor and alpha 2-macroglobulin receptor. J Biol Chem. (1994) 269:17886–92. doi: 10.1016/S0021-9258(17)32392-X
111. Knauer MF, Kridel SJ, Hawley SB, Knauer DJ. The efficient catabolism of thrombin-protease nexin 1 complexes is a synergistic mechanism that requires both the LDL receptor-related protein and cell surface heparins. J Biol Chem. (1997) 272:29039–45. doi: 10.1074/jbc.272.46.29039
112. Stefansson S, Lawrence DA, Argraves WS. Plasminogen activator inhibitor-1 and vitronectin promote the cellular clearance of thrombin by low density lipoprotein receptor-related proteins 1 and 2. J Biol Chem. (1996) 271:8215–20. doi: 10.1074/jbc.271.14.8215
113. Etique N, Verzeaux L, Dedieu S, Emonard H. LRP-1: a checkpoint for the extracellular matrix proteolysis. BioMed Res Int. (2013) 2013:152163. doi: 10.1155/2013/152163
114. Poller W, Willnow TE, Hilpert J, Herz J. Differential recognition of alpha 1-antitrypsin-elastase and alpha 1-antichymotrypsin-cathepsin G complexes by the low density lipoprotein receptor-related protein. J Biol Chem. (1995) 270:2841–5. doi: 10.1074/jbc.270.6.2841
115. Crisp RJ, Knauer DJ, Knauer MF. Roles of the heparin and low density lipid receptor-related protein-binding sites of protease nexin 1 (PN1) in urokinase-PN1 complex catabolism. The PN1 heparin-binding site mediates complex retention and degradation but not cell surface binding or internalization. J Biol Chem. (2000) 275:19628–37. doi: 10.1074/jbc.M909172199
116. Kounnas MZ, Henkin J, Argraves WS, Strickland DK. Low density lipoprotein receptor-related protein/alpha 2-macroglobulin receptor mediates cellular uptake of pro-urokinase. J Biol Chem. (1993) 268:21862–7. doi: 10.1016/S0021-9258(20)80620-6
117. Ho G, Toomey JR, Broze GJ Jr, Schwartz AL. Receptor-mediated endocytosis of coagulation factor Xa requires cell surface-bound tissue factor pathway inhibitor. J Biol Chem. (1996) 271:9497–502. doi: 10.1074/jbc.271.16.9497
118. Knauer DJ, Majumdar D, Fong PC, Knauer MF. SERPIN regulation of factor XIa. The novel observation that protease nexin 1 in the presence of heparin is a more potent inhibitor of factor XIa than C1 inhibitor. J Biol Chem. (2000) 275:37340–6. doi: 10.1074/jbc.M003909200
119. Derocq D, Prébois C, Beaujouin M, Laurent-Matha V, Pattingre S, Smith GK, et al. Cathepsin D is partly endocytosed by the LRP1 receptor and inhibits LRP1-regulated intramembrane proteolysis. Oncogene. (2012) 31:3202–12. doi: 10.1038/onc.2011.501
120. Sánchez MC, Chiabrando GA, Vides MA. Pregnancy zone protein-tissue-type plasminogen activator complexes bind to low-density lipoprotein receptor-related protein (LRP). Arch Biochem Biophys. (2001) 389:218–22. doi: 10.1006/abbi.2001.2329
121. Boucher P, Li WP, Matz RL, Takayama Y, Auwerx J, Anderson RG, et al. LRP1 functions as an atheroprotective integrator of TGFbeta and PDFG signals in the vascular wall: implications for Marfan syndrome. PLoS ONE. (2007) 2:e448. doi: 10.1371/journal.pone.0000448
122. Segarini PR, Nesbitt JE, Li D, Hays LG, Yates JR III, Carmichael DF. The low density lipoprotein receptor-related protein/alpha2-macroglobulin receptor is a receptor for connective tissue growth factor. J Biol Chem. (2001) 276:40659–67. doi: 10.1074/jbc.M105180200
123. Muramatsu H, Zou K, Sakaguchi N, Ikematsu S, Sakuma S, Muramatsu T. LDL receptor-related protein as a component of the midkine receptor. Biochem Biophys Res Commun. (2000) 270:936–41. doi: 10.1006/bbrc.2000.2549
124. Godyna S, Liau G, Popa I, Stefansson S, Argraves WS. Identification of the low density lipoprotein receptor-related protein (LRP) as an endocytic receptor for thrombospondin-1. J Biol Chem. (1995) 129:1403–10. doi: 10.1083/jcb.129.5.1403
125. Meng H, Zhang X, Lee SJ, Strickland DK, Lawrence DA, Wang MM. Low density lipoprotein receptor-related protein-1 (LRP1) regulates thrombospondin-2 (TSP2) enhancement of Notch3 signaling. J Biol Chem. (2010) 285:23047–55. doi: 10.1074/jbc.M110.144634
126. Salicioni AM, Mizelle KS, Loukinova E, Mikhailenko I, Strickland DK, Gonias SL. The low density lipoprotein receptor-related protein mediates fibronectin catabolism and inhibits fibronectin accumulation on cell surfaces. J Biol Chem. (2002) 277:16160–6. doi: 10.1074/jbc.M201401200
127. Storm D, Herz J, Trinder P, Loos M. C1 inhibitor-C1s complexes are internalized and degraded by the low density lipoprotein receptor-related protein. J Biol Chem. (1997) 272:31043–50. doi: 10.1074/jbc.272.49.31043
128. Spijkers PP, Denis CV, Blom AM, Lenting PJ. Cellular uptake of C4b-binding protein is mediated by heparan sulfate proteoglycans and CD91/LDL receptor-related protein. Eur J Immunol. (2008) 38:809–17. doi: 10.1002/eji.200737722
129. Moestrup SK, Cui S, Vorum H, Bregengård C, Bjørn SE, Norris K, et al. Evidence that epithelial glycoprotein 330/megalin mediates uptake of polybasic drugs. J Clin Investig. (1995) 96:1404–13. doi: 10.1172/JCI118176
130. Meilinger M, Gschwentner C, Burger I, Haumer M, Wahrmann M, Szollar L, et al. Metabolism of activated complement component C3 is mediated by the low density lipoprotein receptor-related protein/alpha(2)-macroglobulin receptor. J Biol Chem. (1999) 274:38091–6. doi: 10.1074/jbc.274.53.38091
131. Liu Y, Jones M, Hingtgen CM, Bu G, Laribee N, Tanzi RE, et al. Uptake of HIV-1 tat protein mediated by low-density lipoprotein receptor-related protein disrupts the neuronal metabolic balance of the receptor ligands. Nat Med. (2000) 6:1380–7. doi: 10.1038/82199
132. Basu S, Binder RJ, Ramalingam T, Srivastava PK. CD91 is a common receptor for heat shock proteins gp96, hsp90, hsp70, and calreticulin. Immunity. (2001) 14:303–13. doi: 10.1016/S1074-7613(01)00111-X
133. Bu G, Geuze HJ, Strous GJ, Schwartz AL. 39 kDa receptor-associated protein is an ER resident protein and molecular chaperone for LDL receptor-related protein. EMBO J. (1995) 14:2269–80. doi: 10.1002/j.1460-2075.1995.tb07221.x
134. Williams SE, Ashcom JD, Argraves WS, Strickland DK. A novel mechanism for controlling the activity of alpha 2-macroglobulin receptor/low density lipoprotein receptor-related protein. Multiple regulatory sites for 39-kDa receptor-associated protein. J Biol Chem. (1992) 267:9035–40. doi: 10.1016/S0021-9258(19)50384-2
135. Herz J, Goldstein JL, Strickland DK, Ho YK, Brown MS. 39-kDa protein modulates binding of ligands to low density lipoprotein receptor-related protein/alpha 2-macroglobulin receptor. J Biol Chem. (1991) 266:21232–8. doi: 10.1016/S0021-9258(18)54845-6
136. Gardai SJ, McPhillips KA, Frasch SC, Janssen WJ, Starefeldt A, Murphy-Ullrich JE, et al. Cell-surface calreticulin initiates clearance of viable or apoptotic cells through trans-activation of LRP on the phagocyte. Cell. (2005) 123:321–34. doi: 10.1016/j.cell.2005.08.032
137. Lenting PJ, Neels JG, van den Berg BM, Clijsters PP, Meijerman DW, Pannekoek H, et al. The light chain of factor VIII comprises a binding site for low density lipoprotein receptor-related protein. J Biol Chem. (1999) 274:23734–9. doi: 10.1074/jbc.274.34.23734
138. Rastegarlari G, Pegon JN, Casari C, Odouard S, Navarrete AM, Saint-Lu N, et al. Macrophage LRP1 contributes to the clearance of von Willebrand factor. Blood. (2012) 119:2126–34. doi: 10.1182/blood-2011-08-373605
139. Ling TY, Chen CL, Huang YH, Liu IH, Huang SS, Huang JS. Identification and characterization of the acidic pH binding sites for growth regulatory ligands of low density lipoprotein receptor-related protein-1. J Biol Chem. (2004) 279:38736–48. doi: 10.1074/jbc.M310537200
140. Juric V, Chen CC, Lau LF. TNFα-induced apoptosis enabled by CCN1/CYR61: pathways of reactive oxygen species generation and cytochrome c release. PLoS ONE. (2012) 7:e31303. doi: 10.1371/journal.pone.0031303
141. Brandan E, Retamal C, Cabello-Verrugio C, Marzolo MP. The low density lipoprotein receptor-related protein functions as an endocytic receptor for decorin. J Biol Chem. (2006) 281:31562–71. doi: 10.1016/S0021-9258(19)84070-X
142. Capurro MI, Shi W, Filmus J. LRP1 mediates Hedgehog-induced endocytosis of the GPC3-Hedgehog complex. J Cell Sci. (2012) 125:3380–9. doi: 10.1242/jcs.098889
143. Tsen F, Bhatia A, O'Brien K, Cheng CF, Chen M, Hay N, et al. Extracellular heat shock protein 90 signals through subdomain II and the NPVY motif of LRP-1 receptor to Akt1 and Akt2: a circuit essential for promoting skin cell migration in vitro and wound healing in vivo. Mol Cell Biol. (2013) 33:4947–59. doi: 10.1128/MCB.00559-13
144. Bilodeau N, Fiset A, Boulanger MC, Bhardwaj S, Winstall E, Lavoie JN, et al. Proteomic analysis of Src family kinases signaling complexes in Golgi/endosomal fractions using a site-selective anti-phosphotyrosine antibody: identification of LRP1-insulin receptor complexes. J Proteome Res. (2010) 9:708–17. doi: 10.1021/pr900481b
145. Huang SS, Ling TY, Tseng WF, Huang YH, Tang FM, Leal SM, et al. Cellular growth inhibition by IGFBP-3 and TGF-beta1 requires LRP-1. FASEB J. (2003) 17:2068–81. doi: 10.1096/fj.03-0256com
146. Meilinger M, Haumer M, Szakmary KA, Steinböck F, Scheiber B, Goldenberg H, et al. Removal of lactoferrin from plasma is mediated by binding to low density lipoprotein receptor-related protein/alpha 2-macroglobulin receptor and transport to endosomes. FEBS Lett. (1995) 360:70–4. doi: 10.1016/0014-5793(95)00082-K
147. Liu Q, Zhang J, Zerbinatti C, Zhan Y, Kolber BJ, Herz J, et al. Lipoprotein receptor LRP1 regulates leptin signaling and energy homeostasis in the adult central nervous system. PLoS Biol. (2011) 9:e1000575. doi: 10.1371/journal.pbio.1000575
148. Shakibaei M, Frevert U. Dual interaction of the malaria circumsporozoite protein with the low density lipoprotein receptor-related protein (LRP) and heparan sulfate proteoglycans. J Experi Med. (1996) 184:1699–711. doi: 10.1084/jem.184.5.1699
149. Landowski LM, Pavez M, Brown LS, Gasperini R, Taylor BV, West AK, et al. Low-density lipoprotein receptor-related proteins in a novel mechanism of axon guidance and peripheral nerve regeneration. J Biol Chem. (2016) 291:1092–102. doi: 10.1074/jbc.M115.668996
150. Hofer F, Gruenberger M, Kowalski H, Machat H, Huettinger M, Kuechler E, et al. Members of the low density lipoprotein receptor family mediate cell entry of a minor-group common cold virus. Proc Natl Acad Sci USA. (1994) 91:1839–42. doi: 10.1073/pnas.91.5.1839
151. Stiles TL, Dickendesher TL, Gaultier A, Fernandez-Castaneda A, Mantuano E, Giger RJ, et al. LDL receptor-related protein-1 is a sialic-acid-independent receptor for myelin-associated glycoprotein that functions in neurite outgrowth inhibition by MAG and CNS myelin. J Cell Sci. (2013) 126:209–20. doi: 10.1242/jcs.113191
152. Gaultier A, Wu X, Le Moan N, Takimoto S, Mukandala G, Akassoglou K, et al. Low-density lipoprotein receptor-related protein 1 is an essential receptor for myelin phagocytosis. J Cell Sci. (2009) 122:1155–62. doi: 10.1242/jcs.040717
153. Taylor DR, Hooper NM. The low-density lipoprotein receptor-related protein 1 (LRP1) mediates the endocytosis of the cellular prion protein. Biochem J. (2007) 402:17–23. doi: 10.1042/BJ20061736
154. Kounnas MZ, Morris RE, Thompson MR, FitzGerald DJ, Strickland DK, Saelinger CB. The alpha 2-macroglobulin receptor/low density lipoprotein receptor-related protein binds and internalizes Pseudomonas exotoxin A. J Biol Chem. (1992) 267:12420–3. doi: 10.1016/S0021-9258(18)42291-0
155. Cavallaro U, Nykjaer A, Nielsen M, Soria MR. Alpha 2-macroglobulin receptor mediates binding and cytotoxicity of plant ribosome-inactivating proteins. Eur J Biochem. (1995) 232:165–71. doi: 10.1111/j.1432-1033.1995.tb20795.x
156. Cavallaro U, del Vecchio A, Lappi DA, Soria MR. A conjugate between human urokinase and saporin, a type-1 ribosome-inactivating protein, is selectively cytotoxic to urokinase receptor-expressing cells. J Biol Chem. (1993) 268:23186–90. doi: 10.1016/S0021-9258(19)49445-3
157. Schorch B, Song S, van Diemen FR, Bock HH, May P, Herz J, et al. LRP1 is a receptor for Clostridium perfringens TpeL toxin indicating a two-receptor model of clostridial glycosylating toxins. Proc Natl Acad Sci USA. (2014) 111:6431–6. doi: 10.1073/pnas.1323790111
158. Praetner M, Zuchtriegel G, Holzer M, Uhl B, Schaubächer J, Mittmann L, et al. Plasminogen activator inhibitor-1 promotes neutrophil infiltration and tissue injury on ischemia-reperfusion. Arteriosclerosis Thrombosis Vasc Biol. (2018) 38:829–42. doi: 10.1161/ATVBAHA.117.309760
159. Mikhailenko I, Kounnas MZ, Strickland DK. Low density lipoprotein receptor-related protein/alpha 2-macroglobulin receptor mediates the cellular internalization and degradation of thrombospondin. A process facilitated by cell-surface proteoglycans. J Biol Chem. (1995) 270:9543–9. doi: 10.1074/jbc.270.16.9543
160. Gonias SL, Wu L, Salicioni AM. Low density lipoprotein receptor-related protein: regulation of the plasma membrane proteome. Thrombosis Haemostasis. (2004) 91:1056–64. doi: 10.1160/TH04-01-0023
161. Gaultier A, Arandjelovic S, Niessen S, Overton CD, Linton MF, Fazio S, et al. Regulation of tumor necrosis factor receptor-1 and the IKK-NF-kappaB pathway by LDL receptor-related protein explains the antiinflammatory activity of this receptor. Blood. (2008) 111:5316–25. doi: 10.1182/blood-2007-12-127613
162. Boulagnon-Rombi C, Schneider C, Leandri C, Jeanne A, Grybek V, Bressenot AM, et al. LRP1 expression in colon cancer predicts clinical outcome. Oncotarget. (2018) 9:8849–69. doi: 10.18632/oncotarget.24225
163. Trommsdorff M, Borg JP, Margolis B, Herz J. Interaction of cytosolic adaptor proteins with neuronal apolipoprotein E receptors and the amyloid precursor protein. J Biol Chem. (1998) 273:33556–60. doi: 10.1074/jbc.273.50.33556
164. Barnes H, Larsen B, Tyers M, van Der Geer P. Tyrosine-phosphorylated low density lipoprotein receptor-related protein 1 (Lrp1) associates with the adaptor protein SHC in SRC-transformed cells. J Biol Chem. (2001) 276:19119–25. doi: 10.1074/jbc.M011437200
165. Gotthardt M, Trommsdorff M, Nevitt MF, Shelton J, Richardson JA, Stockinger W, et al. Interactions of the low density lipoprotein receptor gene family with cytosolic adaptor and scaffold proteins suggest diverse biological functions in cellular communication and signal transduction. J Biol Chem. (2000) 275:25616–24. doi: 10.1074/jbc.M000955200
166. Su HP, Nakada-Tsukui K, Tosello-Trampont AC, Li Y, Bu G, Henson PM, et al. Interaction of CED-6/GULP, an adapter protein involved in engulfment of apoptotic cells with CED-1 and CD91/low density lipoprotein receptor-related protein (LRP). J Biol Chem. (2002) 277:11772–9. doi: 10.1074/jbc.M109336200
167. Takayama Y, May P, Anderson RG, Herz J. Low density lipoprotein receptor-related protein 1 (LRP1) controls endocytosis and c-CBL-mediated ubiquitination of the platelet-derived growth factor receptor beta (PDGFR beta). J Biol Chem. (2005) 280:18504–10. doi: 10.1074/jbc.M410265200
168. Zhu L, Giunzioni I, Tavori H, Covarrubias R, Ding L, Zhang Y, et al. Loss of macrophage low-density lipoprotein receptor-related protein 1 confers resistance to the antiatherogenic effects of tumor necrosis factor-α inhibition. Arteriosclerosis Thrombosis Vasc Biol. (2016) 36:1483–95. doi: 10.1161/ATVBAHA.116.307736
169. Mueller PA, Zhu L, Tavori H, Huynh K, Giunzioni I, Stafford JM, et al. Deletion of macrophage low-density lipoprotein receptor-related protein 1 (LRP1) accelerates atherosclerosis regression and increases C-C chemokine receptor type 7 (CCR7) expression in plaque macrophages. Circulation. (2018) 138:1850–63. doi: 10.1161/CIRCULATIONAHA.117.031702
170. Rudijanto A. The role of vascular smooth muscle cells on the pathogenesis of atherosclerosis. Acta Med Indonesiana. (2007) 39:86–93.
171. Chistiakov DA, Orekhov AN, Bobryshev YV. Vascular smooth muscle cell in atherosclerosis. Acta Physiol. (2015) 214:33–50. doi: 10.1111/apha.12466
172. Linton MF, Yancey PG, Davies SS, Jerome WG, Linton EF, Song WL, et al. The role of lipids and lipoproteins in atherosclerosis. In: Feingold KR, Anawalt B, Boyce A, Chrousos G, Dungan K, Grossman A, et al., editors. Endotext. South Dartmouth MA: MDText.com, Inc. (2000).
173. Zhou L, Takayama Y, Boucher P, Tallquist MD, Herz J. LRP1 regulates architecture of the vascular wall by controlling PDGFRbeta-dependent phosphatidylinositol 3-kinase activation. PLoS ONE. (2009) 4:e6922. doi: 10.1371/journal.pone.0006922
174. Newton CS, Loukinova E, Mikhailenko I, Ranganathan S, Gao Y, Haudenschild C, et al. Platelet-derived growth factor receptor-beta (PDGFR-beta) activation promotes its association with the low density lipoprotein receptor-related protein (LRP). Evidence for co-receptor function. J Biol Chem. (2005) 280:27872–8. doi: 10.1074/jbc.M505410200
175. Loukinova E, Ranganathan S, Kuznetsov S, Gorlatova N, Migliorini MM, Loukinov D, et al. Platelet-derived growth factor (PDGF)-induced tyrosine phosphorylation of the low density lipoprotein receptor-related protein (LRP). Evidence for integrated co-receptor function betwenn LRP and the PDGF. J Biol Chem. (2002) 277:15499–506. doi: 10.1074/jbc.M200427200
176. Battegay EJ, Raines EW, Seifert RA, Bowen-Pope DF, Ross R. TGF-beta induces bimodal proliferation of connective tissue cells via complex control of an autocrine PDGF loop. Cell. (1990) 63:515–24. doi: 10.1016/0092-8674(90)90448-N
177. Ihn H. Pathogenesis of fibrosis: role of TGF-beta and CTGF. Curr Opin Rheumatol. (2002) 14:681–5. doi: 10.1097/00002281-200211000-00009
178. Taylor LM, Khachigian LM. Induction of platelet-derived growth factor B-chain expression by transforming growth factor-beta involves transactivation by Smads. J Biol Chem. (2000) 275:16709–16. doi: 10.1074/jbc.275.22.16709
179. Huang SS, Leal SM, Chen CL, Liu IH, Huang JS. Identification of insulin receptor substrate proteins as key molecules for the TbetaR-V/LRP-1-mediated growth inhibitory signaling cascade in epithelial and myeloid cells. FASEB J. (2004) 18:1719–21. doi: 10.1096/fj.04-1872fje
180. Henriet P, Emonard H. Matrix metalloproteinase-2: not (just) a “hero” of the past. Biochimie. (2019) 166:223–32. doi: 10.1016/j.biochi.2019.07.019
181. Newby AC. Metalloproteinase expression in monocytes and macrophages and its relationship to atherosclerotic plaque instability. Arteriosclerosis Thrombosis Vasc Biol. (2008) 28:2108–14. doi: 10.1161/ATVBAHA.108.173898
182. Wang M, Kim SH, Monticone RE, Lakatta EG. Matrix metalloproteinases promote arterial remodeling in aging, hypertension, and atherosclerosis. Hypertension. (2015) 65:698–703. doi: 10.1161/HYPERTENSIONAHA.114.03618
183. Gordts PL, Reekmans S, Lauwers A, Van Dongen A, Verbeek L, Roebroek AJ. Inactivation of the LRP1 intracellular NPxYxxL motif in LDLR-deficient mice enhances postprandial dyslipidemia and atherosclerosis. Arteriosclerosis Thrombosis Vasc Biol. (2009) 29:1258–64. doi: 10.1161/ATVBAHA.109.192211
184. Graves LM, Bornfeldt KE, Sidhu JS, Argast GM, Raines EW, Ross R, et al. Platelet-derived growth factor stimulates protein kinase A through a mitogen-activated protein kinase-dependent pathway in human arterial smooth muscle cells. J Biol Chem. (1996) 271:505–11. doi: 10.1074/jbc.271.1.505
185. Lin LL, Lin AY, Knopf JL. Cytosolic phospholipase A2 is coupled to hormonally regulated release of arachidonic acid. Proc Natl Acad Sci USA. (1992) 89:6147–51. doi: 10.1073/pnas.89.13.6147
186. DeBose-Boyd RA, Ou J, Goldstein JL, Brown MS. Expression of sterol regulatory element-binding protein 1c (SREBP-1c) mRNA in rat hepatoma cells requires endogenous LXR ligands. Proc Natl Acad Sci USA. (2001) 98:1477–82. doi: 10.1073/pnas.98.4.1477
187. Zhou L, Choi HY, Li WP, Xu F, Herz J. LRP1 controls cPLA2 phosphorylation, ABCA1 expression and cellular cholesterol export. PLoS ONE. (2009) 4:e6853. doi: 10.1371/journal.pone.0006853
188. Hiltunen TP, Luoma JS, Nikkari T, Ylä-Herttuala S. Expression of LDL receptor, VLDL receptor, LDL receptor-related protein, and scavenger receptor in rabbit atherosclerotic lesions: marked induction of scavenger receptor and VLDL receptor expression during lesion development. Circulation. (1998) 97:1079–86. doi: 10.1161/01.CIR.97.11.1079
189. Llorente-Cortés V, Otero-Viñas M, Berrozpe M, Badimon L. Intracellular lipid accumulation, low-density lipoprotein receptor-related protein expression, and cell survival in vascular smooth muscle cells derived from normal and atherosclerotic human coronaries. Eur J Clin Investig. (2004) 34:182–90. doi: 10.1111/j.1365-2362.2004.01316.x
190. Llorente-Cortés V, Badimon L. LDL receptor-related protein and the vascular wall: implications for atherothrombosis. Arteriosclerosis Thrombosis Vasc Biol. (2005) 25:497–504. doi: 10.1161/01.ATV.0000154280.62072.fd
191. Ma L, Zhong J, Zhao Z, Luo Z, Ma S, Sun J, et al. Activation of TRPV1 reduces vascular lipid accumulation and attenuates atherosclerosis. Cardiovasc Res. (2011) 92:504–13. doi: 10.1093/cvr/cvr245
192. Cal R, García-Arguinzonis M, Revuelta-López E, Castellano J, Padró T, Badimon L, et al. Aggregated low-density lipoprotein induces LRP1 stabilization through E3 ubiquitin ligase CHFR downregulation in human vascular smooth muscle cells. Arteriosclerosis Thrombosis Vasc Biol. (2013) 33:369–77. doi: 10.1161/ATVBAHA.112.300748
193. Castellano J, Aledo R, Sendra J, Costales P, Juan-Babot O, Badimon L, et al. Hypoxia stimulates low-density lipoprotein receptor-related protein-1 expression through hypoxia-inducible factor-1α in human vascular smooth muscle cells. Arteriosclerosis Thrombosis Vasc Biol. (2011) 31:1411–20. doi: 10.1161/ATVBAHA.111.225490
194. Tong Y, Cai L, Yang S, Liu S, Wang Z, Cheng J. The research progress of vascular macrophages and atherosclerosis. Oxidat Med Cell Longevity. (2020) 2020:7308736. doi: 10.1155/2020/7308736
195. Yan ZQ, Hansson GK. Innate immunity, macrophage activation, and atherosclerosis. Immunol Rev. (2007) 219:187–203. doi: 10.1111/j.1600-065X.2007.00554.x
196. Linton MF, Babaev VR, Gleaves LA, Fazio S. A direct role for the macrophage low density lipoprotein receptor in atherosclerotic lesion formation. J Biol Chem. (1999) 274:19204–10. doi: 10.1074/jbc.274.27.19204
197. May P, Bock HH, Nofer JR. Low density receptor-related protein 1 (LRP1) promotes anti-inflammatory phenotype in murine macrophages. Cell Tissue Res. (2013) 354:887–9. doi: 10.1007/s00441-013-1699-2
198. Watanabe Y, Inaba T, Shimano H, Gotoda T, Yamamoto K, Mokuno H, et al. Induction of LDL receptor-related protein during the differentiation of monocyte-macrophages. Possible involvement in the atherosclerotic process. Arteriosclerosis Thrombosis. (1994) 14:1000–6. doi: 10.1161/01.ATV.14.6.1000
199. Zhu H, Takahashi Y, Xu W, Kawajiri H, Murakami T, Yamamoto M, et al. Low density lipoprotein receptor-related protein-mediated membrane translocation of 12/15-lipoxygenase is required for oxidation of low density lipoprotein by macrophages. J Biol Chem. (2003) 278:13350–5. doi: 10.1074/jbc.M212104200
200. Xu W, Takahashi Y, Sakashita T, Iwasaki T, Hattori H, Yoshimoto T. Low density lipoprotein receptor-related protein is required for macrophage-mediated oxidation of low density lipoprotein by 12/15-lipoxygenase. J Biol Chem. (2001) 276:36454–9. doi: 10.1074/jbc.M105093200
201. Harrach-Ruprecht B, Küchenhoff A, Robenek H. Interaction of apo E-containing lipoproteins with the LDL-receptor-related protein, LRP. Zeitschrift Gastroenterol. (1996) 34(Suppl. 3):122–3.
202. Fujioka Y, Cooper AD, Fong LG. Multiple processes are involved in the uptake of chylomicron remnants by mouse peritoneal macrophages. J Lipid Res. (1998) 39:2339–49. doi: 10.1016/S0022-2275(20)33313-7
203. Kwon GP, Schroeder JL, Amar MJ, Remaley AT, Balaban RS. Contribution of macromolecular structure to the retention of low-density lipoprotein at arterial branch points. Circulation. (2008) 117:2919–27. doi: 10.1161/CIRCULATIONAHA.107.754614
204. Strickland DK, Muratoglu SC. LRP in endothelial cells: a little goes a long way. Arteriosclerosis Thrombosis Vasc Biol. (2016) 36:213–6. doi: 10.1161/ATVBAHA.115.306895
205. Mao H, Lockyer P, Townley-Tilson WH, Xie L, Pi X. LRP1 regulates retinal angiogenesis by inhibiting PARP-1 activity and endothelial cell proliferation. Arteriosclerosis Thrombosis Vasc Biol. (2016) 36:350–60. doi: 10.1161/ATVBAHA.115.306713
206. Pi X, Schmitt CE, Xie L, Portbury AL, Wu Y, Lockyer P, et al. LRP1-dependent endocytic mechanism governs the signaling output of the bmp system in endothelial cells and in angiogenesis. Circul Res. (2012) 111:564–74. doi: 10.1161/CIRCRESAHA.112.274597
207. Gariano RF, Gardner TW. Retinal angiogenesis in development and disease. Nature. (2005) 438:960–6. doi: 10.1038/nature04482
208. Lockyer P, Mao H, Fan Q, Li L, Yu-Lee LY, Eissa NT, et al. LRP1-dependent BMPER signaling regulates lipopolysaccharide-induced vascular inflammation. Arteriosclerosis Thrombosis Vasc Biol. (2017) 37:1524–35. doi: 10.1161/ATVBAHA.117.309521
209. Mao H, Lockyer P, Li L, Ballantyne CM, Patterson C, Xie L, et al. Endothelial LRP1 regulates metabolic responses by acting as a co-activator of PPARγ. Nat Commun. (2017) 8:14960. doi: 10.1038/ncomms14960
210. Hu H, Garcia-Barrio M, Jiang ZS, Chen YE, Chang L. Roles of perivascular adipose tissue in hypertension and atherosclerosis. Antioxidants Redox Signal. (2020) 34:736–49. doi: 10.1089/ars.2020.8103
211. Omar A, Chatterjee TK, Tang Y, Hui DY, Weintraub NL. Proinflammatory phenotype of perivascular adipocytes. Arteriosclerosis Thrombosis Vasc Biol. (2014) 34:1631–6. doi: 10.1161/ATVBAHA.114.303030
212. Verhagen SN, Visseren FL. Perivascular adipose tissue as a cause of atherosclerosis. Atherosclerosis. (2011) 214:3–10. doi: 10.1016/j.atherosclerosis.2010.05.034
213. Hofmann SM, Zhou L, Perez-Tilve D, Greer T, Grant E, Wancata L, et al. Adipocyte LDL receptor-related protein-1 expression modulates postprandial lipid transport and glucose homeostasis in mice. J Clin Investig. (2007) 117:3271–82. doi: 10.1172/JCI31929
214. Silvestre-Roig C, Braster Q, Ortega-Gomez A, Soehnlein O. Neutrophils as regulators of cardiovascular inflammation. Nat Rev Cardiol. (2020) 17:327–40. doi: 10.1038/s41569-019-0326-7
215. Guasti L, Dentali F, Castiglioni L, Maroni L, Marino F, Squizzato A, et al. Neutrophils and clinical outcomes in patients with acute coronary syndromes and/or cardiac revascularisation. A systematic review on more than 34,000 subjects. Thrombosis Haemostasis. (2011) 106:591–9. doi: 10.1160/TH11-02-0096
216. Drechsler M, Megens RT, van Zandvoort M, Weber C, Soehnlein O. Hyperlipidemia-triggered neutrophilia promotes early atherosclerosis. Circulation. (2010) 122:1837–45. doi: 10.1161/CIRCULATIONAHA.110.961714
217. Liberale L, Bertolotto M, Minetti S, Contini P, Verzola D, Ameri P, et al. Recombinant tissue plasminogen activator (r-tPA) induces in-vitro human neutrophil migration via low density lipoprotein receptor-related protein 1 (LRP-1). Int J Mol Sci. (2020) 21:7014. doi: 10.3390/ijms21197014
218. Weckbach LT, Gola A, Winkelmann M, Jakob SM, Groesser L, Borgolte J, et al. The cytokine midkine supports neutrophil trafficking during acute inflammation by promoting adhesion via β2 integrins (CD11/CD18). Blood. (2014) 123:1887–96. doi: 10.1182/blood-2013-06-510875
219. Fernandez DM, Rahman AH, Fernandez NF, Chudnovskiy A, Amir ED, Amadori L, et al. Single-cell immune landscape of human atherosclerotic plaques. Nat Med. (2019) 25:1576–88. doi: 10.1038/s41591-019-0590-4
220. Zhou X, Nicoletti A, Elhage R, Hansson GK. Transfer of CD4(+) T cells aggravates atherosclerosis in immunodeficient apolipoprotein E knockout mice. Circulation. (2000) 102:2919–22. doi: 10.1161/01.CIR.102.24.2919
221. Panezai J, Bergdahl E, Sundqvist KG. T-cell regulation through a basic suppressive mechanism targeting low-density lipoprotein receptor-related protein 1. Immunology. (2017) 152:308–27. doi: 10.1111/imm.12770
222. Bergström SE, Bergdahl E, Sundqvist KG. A cytokine-controlled mechanism for integrated regulation of T-lymphocyte motility, adhesion and activation. Immunology. (2013) 140:441–55. doi: 10.1111/imm.12154
223. Kojima Y, Downing K, Kundu R, Miller C, Dewey F, Lancero H, et al. Cyclin-dependent kinase inhibitor 2B regulates efferocytosis and atherosclerosis. J Clin Investig. (2014) 124:1083–97. doi: 10.1172/JCI70391
224. Kojima Y, Volkmer JP, McKenna K, Civelek M, Lusis AJ, Miller CL, et al. CD47-blocking antibodies restore phagocytosis and prevent atherosclerosis. Nature. (2016) 536:86–90. doi: 10.1038/nature18935
225. Bornachea O, Benitez-Amaro A, Vea A, Nasarre L, de Gonzalo-Calvo D, Escola-Gil JC, et al. Immunization with the Gly (1127) -Cys (1140) amino acid sequence of the LRP1 receptor reduces atherosclerosis in rabbits. Molecular, immunohistochemical and nuclear imaging studies. Theranostics. (2020) 10:3263–80. doi: 10.7150/thno.37305
226. Emonard H, Bellon G, de Diesbach P, Mettlen M, Hornebeck W, Courtoy PJ. Regulation of matrix metalloproteinase (MMP) activity by the low-density lipoprotein receptor-related protein (LRP). A new function for an “old friend”. Biochimie. (2005) 87:369–76. doi: 10.1016/j.biochi.2004.11.013
227. Schäfers M, Schober O, Hermann S. Matrix-metalloproteinases as imaging targets for inflammatory activity in atherosclerotic plaques. J Nucl Med. (2010) 51:663–6. doi: 10.2967/jnumed.109.065698
228. Potere N, Del Buono MG, Niccoli G, Crea F, Toldo S, Abbate A. Developing LRP1 agonists into a therapeutic strategy in acute myocardial infarction. Int J Mol Sci. (2019) 20:544. doi: 10.3390/ijms20030544
Keywords: LRP1, atherosclerosis, smooth muscle cells, macrophages, endothelial cells, adipocytes, immune cells
Citation: Chen J, Su Y, Pi S, Hu B and Mao L (2021) The Dual Role of Low-Density Lipoprotein Receptor-Related Protein 1 in Atherosclerosis. Front. Cardiovasc. Med. 8:682389. doi: 10.3389/fcvm.2021.682389
Received: 18 March 2021; Accepted: 05 May 2021;
Published: 28 May 2021.
Edited by:
Klaus T. Preissner, University of Giessen, GermanyReviewed by:
Dudley Strickland, University of Maryland, Baltimore, United StatesMalgorzata Wygrecka, University of Giessen, Germany
William Boisvert, University of Hawaii at Manoa, United States
Copyright © 2021 Chen, Su, Pi, Hu and Mao. This is an open-access article distributed under the terms of the Creative Commons Attribution License (CC BY). The use, distribution or reproduction in other forums is permitted, provided the original author(s) and the copyright owner(s) are credited and that the original publication in this journal is cited, in accordance with accepted academic practice. No use, distribution or reproduction is permitted which does not comply with these terms.
*Correspondence: Ling Mao, maoling@hust.edu.cn; Bo Hu, hubo@hust.edu.cn
†These authors have contributed equally to this work and share first authorship