- 1Department of Internal Medicine, Maastricht Universitair Medisch Centrum+, Maastricht, Netherlands
- 2Department of Internal Medicine, Zuyderland Medisch Centrum, Heerlen, Netherlands
- 3Department of Biochemistry–CARIM, Maastricht University, Maastricht, Netherlands
- 4Groene Hart Ziekenhuis, Gouda, Netherlands
- 5Department of Internal Medicine, Jeroen Bosch Ziekenhuis, ‘s-Hertogenbosch, Netherlands
- 6Department of Cardiology, Zuyderland Medisch Centrum, Heerlen, Netherlands
- 7Department of Cardiology, Maastricht Universitair Medisch Centrum+, Maastricht, Netherlands
Background: Patients using dual antiplatelet therapy after percutaneous coronary intervention are at risk for bleeding. It is currently unknown whether thrombin generation can be used to identify patients receiving dual antiplatelet therapy with increased bleeding risk.
Objectives: To investigate whether thrombin generation measurement in plasma provides additional insight into the assessment of bleeding risk for high clinical-risk patients using dual antiplatelet therapy.
Methods: Coagulation factors and thrombin generation in platelet-poor plasma were measured in 93 high clinical-risk frail patients using dual antiplatelet therapy after percutaneous coronary intervention. During 12-month follow-up, clinically relevant bleedings were reported. Thrombin generation at 1 and 6 months after percutaneous coronary intervention was compared between patients with and without bleeding events.
Results: One month after percutaneous coronary intervention, the parameters of thrombin generation, endogenous thrombin potential, peak height, and velocity index were significantly lower in patients with bleeding in the following months compared to patients without bleeding. At 6 months follow-up, endogenous thrombin potential, peak height, and velocity index were still (significantly) decreased in the bleeding group as compared to non-bleeders. Thrombin generation in the patients' plasma was strongly dependent on factor II, V, and VIII activity and fibrinogen.
Conclusion: High clinical-risk patients using dual antiplatelet therapy with clinically relevant bleeding during follow-up show reduced and delayed thrombin generation in platelet-poor plasma, possibly due to variation in coagulation factors. Thus, impaired thrombin-generating potential may be a “second hit” on top of dual antiplatelet therapy, increasing the bleeding risk in high clinical-risk patients. Thrombin generation has the potential to improve the identification of patients using dual antiplatelet therapy at increased risk of bleeding.
Introduction
Each year in Europe, about 3.6 million patients receive dual antiplatelet therapy (DAPT) during 6 to 12 months after a percutaneous coronary intervention (PCI) (1). This patient population has an increased risk for both bleeding and ischemic events. Risk factors for bleeding and ischemic events are multifactorial, and it remains difficult to predict the individual bleeding vs. ischemic risk. Known risk factors for the occurrence of bleeding complications are, among others, low on-treatment platelet reactivity, the use of chronic oral anticoagulation therapy and older age (2, 3). On the other hand, ischemic events after PCI are associated with initial presentation with acute coronary syndrome (ACS), the stent itself (in-stent thrombosis), older age, diabetes, and chronic kidney disease (4). Identification of patients who are at higher risk of bleeding or ischemic events could lead to better personalized treatment and reduce the burden of adverse events.
While DAPT substantially reduces the risk of ischemic events, it comes with an impairment of primary hemostasis (5, 6). However, the overall hemostatic potential is determined not only by platelet reactivity but also by other factors like endothelial cell barrier integrity and the coagulation system including multiple coagulation factors (7). Variation in coagulant activity therefore impacts the bleeding risk in patients with a platelet function impairment inflicted by DAPT. This “second hit” of coinciding coagulation impairment can be due to variation in specific plasma components, like the level of factor VIII that shows substantial variation in the population (8), or DAPT-dependent reduction in the availability of the platelet procoagulant surface for efficient assembly of coagulation factor complexes promoting the formation of thrombin (9–11). Both mechanisms can be addressed in thrombin generation (TG) measurements using the “calibrated automated thrombogram” (CAT) (12). Thus far, a number of studies have demonstrated TG as a valid tool to detect a bleeding tendency in patients with hemophilia A and B, von Willebrand disease, factor XI deficiency, platelet disorders, and vitamin K antagonist (VKA) treatment (13–17).
One would expect that a reduction of platelet activation and aggregation by antiplatelet therapy leads to a diminished TG, measured in platelet-rich plasma (PRP) or whole blood (WB). Indeed, studies confirmed that treatment with aspirin, clopidogrel, or both reduced TG parameters in PRP and WB (18–23). The relationship between TG and the risk of bleeding in case of DAPT is rather underexposed. Therefore, in the present study, we investigated the potential use of the TG assay for the assessment of bleeding risk in high clinical-risk patients using DAPT. Besides, we were interested whether bleeding risk is influenced by the level of coagulation factors (24). TG in this study was measured in PPP, since this is by far the most used and standardized method of TG measurement. Hence, we hypothesized that patients who suffer from clinically relevant bleedings during DAPT show lower TG parameters compared to patients without bleeding events.
Materials and Methods
Study Population and Design
The current study is a sub-study of a prospective cohort study in the Maastricht University Medical Center+ (MUMC+), monitoring high clinical-risk patients on dual/triple antithrombotic therapy after PCI, as previously described (25). DAPT includes a combination of low-dose aspirin with either clopidogrel, ticagrelor, or prasugrel. DAPT duration was either 6 or 12 months, dependent on the PCI indication: elective vs. acute (ACS), respectively. In this exploratory study, patients were included via the outpatient clinic of the Thrombosis Expertise Center in the MUMC+, during a period of 2 years.
All PCI patients were screened by one dedicated cardiologist, and, after informed consent was obtained, high clinical-risk patients were included within 1 month after PCI. High clinical-risk patients in the prospective cohort study were defined as patients who had ≥3 risk factors for bleeding or ischemic events, which include female gender, hypertension, anemia at time of PCI (<13.2 g/dl for men, <11.8 g/dl for women), age >75 years, previous stroke, previous major bleeding, renal dysfunction [estimated glomerular filtration ratio (eGFR) <60 ml/min], known hepatitis or liver transplant, triple antithrombotic therapy, previous gastric ulcers, diabetes mellitus, low body weight (<60 kg), use of non-steroidal anti-inflammatory drugs or selective serotonin reuptake inhibitors, previous in-stent thrombosis, and/or high-risk stenting (multivessel PCI or main coronary artery stenting). The cohort population included patients who met the criteria named above, had been fasting for 4 h before blood withdrawal, and had a PCI in the past 1–2 months. Patients were excluded in case of non-compliance. The latter was checked during the visit by interview and by contacting the pharmacy to confirm dispensing of the drugs. According to the study protocol, TG was measured in the first group of 200 patients included in the prospective cohort study. Specific inclusion criteria for this sub-study were usage of a combination of low-dose aspirin (80–100 mg) and a P2Y12 inhibitor (clopidogrel 75 mg, prasugrel 5–10 mg, or ticagrelor 90 mg) (DAPT) for a planned duration of >6 months, and patients for whom TG measurements were available. An additional exclusion criterion for this sub-study was the use of vitamin K agonists, direct oral anticoagulants, or low-molecular-weight heparins. All patients provided written consent. Ethical approval was obtained from the medical ethical committee of the MUMC+ [(METC aZM/UM), approval number: 11-2-096].
Follow-Up and Study Endpoints
After referral, consultation at the thrombosis expertise center and study visits were planned at 1 month (T1) and 6 months (T2) after PCI (Figure 1). Twelve months after PCI (T3) patients using DAPT >6 months were contacted by phone for final follow-up. Blood was drawn at T1 and once again at T2 in case DAPT was continued for a total treatment duration of 9–12 months. During all three contact moments, a thorough history on minor and major bleeding was taken using the International Society on Thrombosis and Haemostasis (ISTH) Bleeding Assessment Tool (ISTH-AT). Using these structured questionnaires, bleeding events were recorded and categorized according to the definition of the Bleeding Academic Research Consortium (BARC), which contains unified and validated bleeding criteria (26–28). The primary endpoint of this study was clinically relevant bleeding during DAPT treatment, defined as BARC type 2, 3, or 5. Bleeding events presented at T1 include bleedings from PCI until T1, at T2 from T1 until T2, and at T3 from T2 until T3 (Figure 1). To investigate whether TG could identify patients with future clinically relevant bleedings, all bleeding events recorded at T2 and T3 were included in the analysis for TG measured at T1, and bleeding events recorded at T3 for TG measured at T2. In addition, during the follow-up visits, medical history, recurrent ischemic events, concomitant medication, intoxications, and compliance were recorded. To minimize recall bias, this information was collected in conversation with the patient and cross-checked with the hospital's electronic medical records.
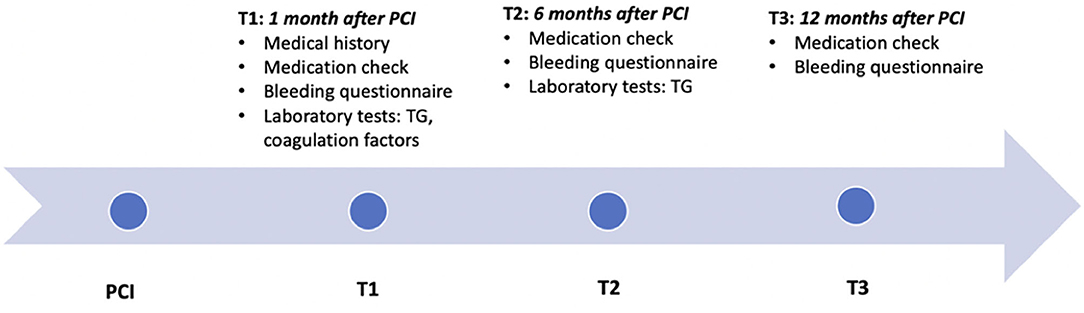
Figure 1. Timeline. Timeline of the study, showing the three contact moments: TI, T2, and T3, at respectively, 1, 6, and 12 months after PCI. The collected information and performed tests at each consultation is described. TG, Thrombin generation; PCI, percutaneous coronary intervention.
Blood Samples
During the first and second study visit, venous blood was collected through separate antecubital venipuncture in Vacuette® tubes (Greiner Bio-One, GmbH, Kremsmünster, Austria) containing sodium citrate (3.2%). PPP was prepared by two centrifugation steps at 2,000 g for 10 min and aliquots were stored at −80°C. TG was measured in all samples, simultaneously.
Reagents
Innovin (Dade-Behring, Marburg, Germany) was used as a source of recombinant tissue factor (TF). Synthetic phospholipids (PL), consisting of phosphatidylserine, phosphatidylethanolamine, and phosphatidylcholine (1:1:3, mol:mol:mol), were from Avanti Polar Lipids Inc. (Alabaster, AL, USA). Z-Gly-Gly-Arg-aminomethylcoumarine (ZGGR-AMC) was purchased from Bachem (Basel, Switzerland). The calibrator, α2-macroglobulin-thrombin complex, was prepared as described by Hemker et al. (12). Hepes buffers containing 5 mg/ml or 60 mg/ml bovine serum albumin were prepared as described previously (29).
Thrombin Generation
TG in PPP was measured by calibrated automated thrombogram (CAT) in triplicate, as previously described (30). In short, the reaction was initiated with 20 μl of stimulus [tissue factor (TF)], containing a final concentration of 1 pM TF and 4 μM phospholipids (PL). Next, 80 μl of plasma was added to the well. The calibration wells contained 20 μl of calibrator, with a final thrombin concentration of 100 nM. The reaction was started by adding 20 μl of FluCa (416.7 μM ZGGR-AMC and 16.7 mM CaCl2 in BSA60 buffer). To minimize variation, samples of normal pool plasma were measured on each plate. Data were analyzed using software from Thrombinoscope version 5.0 (Maastricht, the Netherlands). Low TF concentration was used to include the contribution of the intrinsic pathway factors VIII, IX, and XI (31, 32). The analyzed TG parameters for all samples were lag time, Endogenous Thrombin Potential (ETP), velocity index, peak level of thrombin formation, and time to peak.
Additional Analyses
Measurements of coagulation factors were performed using the STA-R Evolution analyzer (Stago, Asnières sur Seine, France). Factor (F)II, V, VII, VIII, IX, X, XI, and XII activity levels were assessed with clotting assays triggered by either a thromboplastin-based reagent (FII, FV, FVII, and FX) or a kaolin-based reagent (FVIII, FXI, FIX, and FXII). Fibrinogen levels were measured using the Clauss method, and both fibrinogen and von Willebrand factor (vWF) were measured with the CS2100i analyzer from Sysmex (33).
Statistical Analysis
Statistical analysis was performed using SPSS software (version 24). Patients with missing data (other than TG) were not excluded from the analysis. Normal distribution of all continuous variables was calculated using the Kolmogorov–Smirnov and Shapiro–Wilk Test of Normality combined with evaluation of the histograms. For normally distributed continuous variables, differences between groups were tested by Student's t-test and represented by means and standard deviations (SD). For non-normally distributed continuous variables, differences between groups were tested by Mann–Whitney U test and represented by medians and interquartile range (IQR) or range (when subgroups where too small for an IQR). Categorical variables were tested by Chi-square test and represented as numbers and percentages.
A multiple regression analysis was done to investigate coagulation factors as determinants of thrombin generation. For each model, the adjusted R2 and the standardized regression coefficients (beta) of the independent variables (coagulation factors) were calculated. A two-sided p ≤ 0.05 was considered statistically significant.
Results
Study Population
In total, 111 patients were eligible for this sub-study, of whom 18 patients had to be excluded for several reasons: 3 patients were not compliant, 2 patients used DAPT <1 month, and 13 patients were lost to follow-up within 6 months (Figure 2). Thus, the final study population consisted of 93 patients, of whom 13 patients with an elective PCI could finalize their DAPT at 6 months. Out of 80 patients continuing DAPT with a total treatment duration of 9–12 months, blood was drawn again at the second study visit (T2, 6 months) in 68 patients. Unfortunately, no TG could be measured in the other 12 patients due to logistical reasons. Baseline characteristics of patients lost to follow-up or with missing TG measurements at T2 did not differ from the final study population or the T2 population, respectively (Supplementary Tables 2, 3). During the treatment period, 14 patients (15.1%) suffered from clinically relevant bleeding (BARC score ≥2), of which 7 bleedings were documented at T1, 3 bleedings at T2, and 4 bleedings at T3 (Supplementary Table 1). No patient had more than one clinically relevant bleeding event during follow-up.
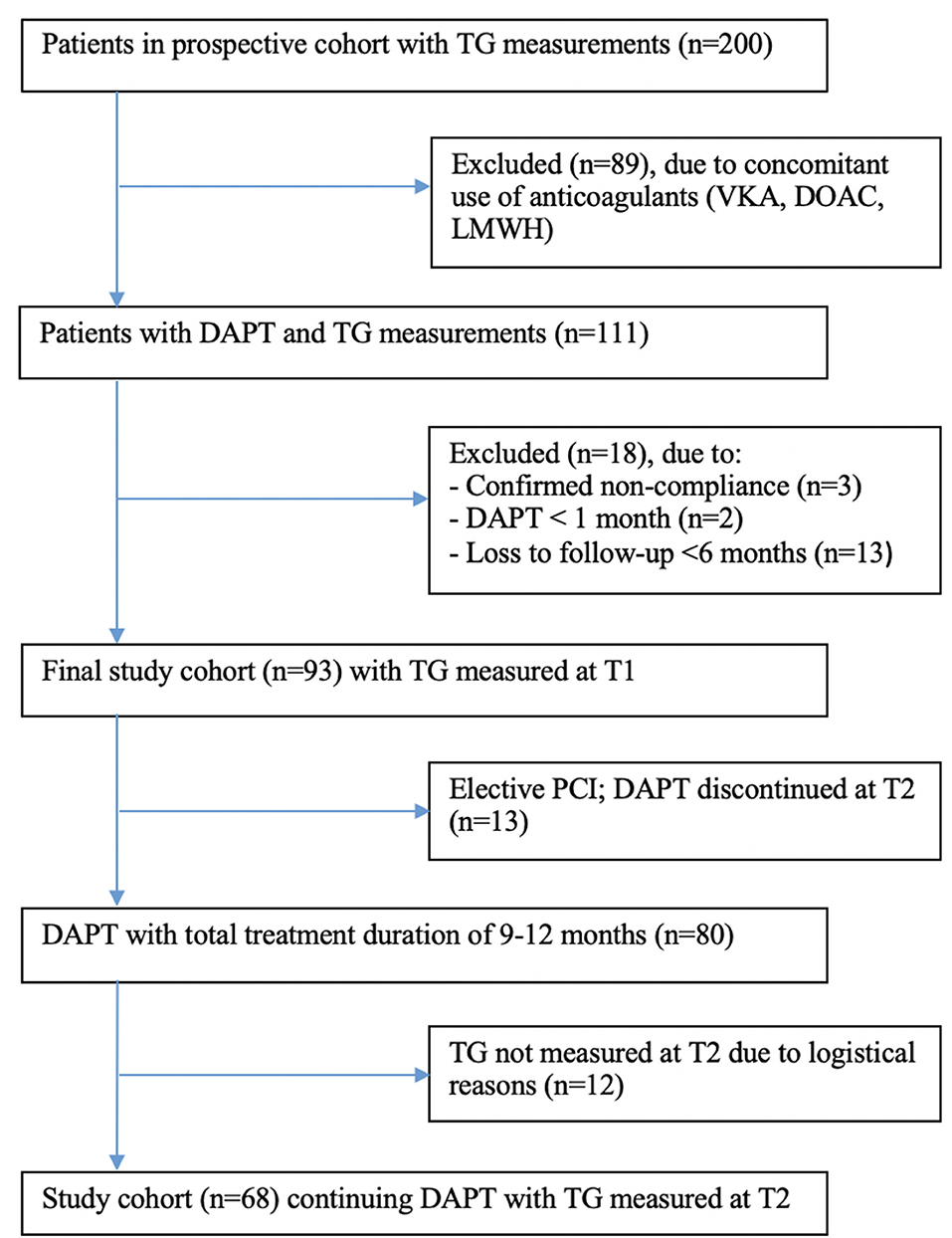
Figure 2. Flow diagram of study inclusion and follow-up. TG, thrombin generation; VKA, vitamin K antagonist; DOAC, direct-acting oral anticoagulants; LMWH, low molecular weight heparin; DAFT, dual antiplatelet therapy; PCI, percutaneous coronary intervention.
Baseline characteristics of the 93 included patients are listed in Table 1. Significant differences in prevalence of anemia and TIA/CVA in medical history were found between patients with and without clinically relevant bleeding. No significant differences were found in amount of days from PCI to T1 between both groups. Most of the patients (n = 80, 86.0%) used DAPT for 9–12 months after PCI of which 12 patients (15.0%) suffered from clinically relevant bleeding during treatment. Thirteen patients (14.0%) used DAPT for 6 months according to prescription after elective PCI, of whom three (20.0%) had a clinically relevant bleeding.
Thrombin Generation and Clinically Relevant Bleeding During Follow-Up
TG measured 1 month after PCI (T1) was significantly lower with respect to ETP (p < 0.001), peak height (p = 0.004), and velocity index (p = 0.016) in plasma from patients with bleeding during follow-up (n = 8) compared to patients without bleeding complications (n = 85) (bleedings recorded at T2 and T3) (Figure 3). Moreover, patients with bleeding had a significantly longer time-to-peak (p = 0.007) and lag time (p = 0.036) compared to patients without bleeding (Figure 3). Similarly, at 6 months after PCI (T2, n = 68), significantly lower peak level (p = 0.039), velocity index (p = 0.031), and a trend toward a lower ETP (p = 0.072) were detected in plasma from patients who experienced bleeding during follow-up (n = 4) (bleedings recorded at T3) (Table 2).
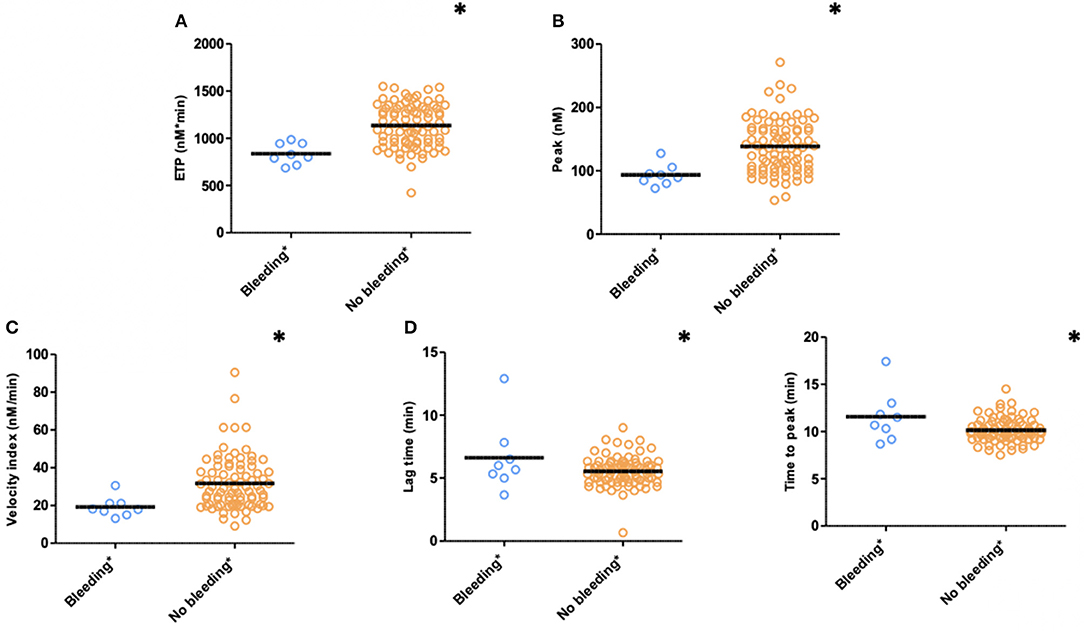
Figure 3. Thrombin generation (TG) in PPP patients with (n = 15) and without (n = 78) clinically relevant bleeding (N = 93) during follow-up (bleedings recorded at T2 and T3). (A) ETP, (B) Peak, (C) Velocity index, (D) Lag time, and (E) Time to peak. Significant lower ETP levels (p ≤ 0.001), peak height (p = 0.004), and velocity index (p = 0.016) in patients with clinically relevant bleeding during follow-up. Significant longer time to peak (p = 0.007) and lag time (p = 0.036) in patients with clinically relevant bleeding. Means are indicated by lines. *p < 0.05. ETP, Endogenous Thrombin Potential; PPP, Platelet Poor Plasma; N, number of patients.
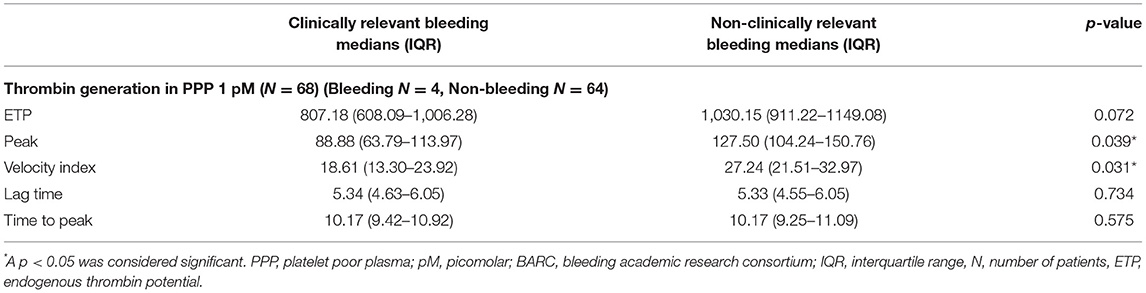
Table 2. Thrombin generation 6 months after PCI in PPP for patients with and without clinically relevant bleeding during follow-up (recorded at T3).
Coagulation Factors as Determinants of TG in PPP
At T1, ETP was significantly determined by FII activity level [Beta 8.675 (95% CI 1.336–16.014), p = 0.021], as expected, while the peak level was significantly determined by the FV activity level [Beta −0.663 (95% CI −1.244 to −0.083), p = 0.026]. ETP, peak level, and velocity index were all significantly affected by FVIII activity [Beta, respectively, 2.128 (95% CI 0.045–4.210), 0.516 (95% CI 0.156–0.877), and 0.171 (95% CI 0.052–0.291) (p-value, respectively, 0.045, 0.006, and 0.006)]. Lag time and time-to-peak were mainly determined by the plasma fibrinogen concentration [Beta, respectively, 0.688 (95% CI 0.119–1.258) and 0.788 (95% CI 0.270) (p-value, respectively, 0.019 and 0.004)] (Table 3).
Discussion
The main aim of our study was to investigate whether TG might have potential to identify patients with an increased bleeding risk during the following DAPT treatment period of 6–12 months, by comparing the TG parameters between “bleeders” and “non-bleeders.” TG parameters measured in PPP, at 1 and 6 months after PCI, showed (significant) lower ETP, peak, and velocity index levels in patients with a clinically relevant bleeding episode compared to patients without bleeding during follow-up.
Since thrombin generation was performed in the absence of platelets, the observed differences between patients with and without bleeding might be explained by variation in coagulation factors. Following this theory, impaired thrombin generation resulting from one or several coagulation factor levels in the low-normal range, on top of DAPT, could ultimately contribute to an increased risk of bleeding. Mean values of all coagulation factors measured in this study were within normal range. We showed in this study that ETP was mostly determined by prothrombin levels, and peak height by FV level, as confirmed by previous studies (24, 35–37). ETP, peak height, and velocity index were all significantly determined by the level of factor VIII. This positive relation can be explained by the strong feedback loop between thrombin and factor VIII (37, 38). Higher ETP in case of higher factor II (prothrombin) levels was expected, since factor II is the precursor of thrombin and has been confirmed in previous research (24, 36, 39). We found a negative association between FV activity and peak height. Interestingly, this inverse relationship was also found in healthy individuals in a previous publication by Dielis et al. (36). This negative relation might be attributed to the fact that factor V has both procoagulant and anticoagulant potential; activated FV functions in the procoagulant pathways, but in its inactivated form, FV acts as a cofactor for activated protein C (APC) in the regulation of FVIIIa (40). Increased lag time and time-to-peak were both associated with higher levels of fibrinogen. Based on other studies investigating TG in normal and defibrinated plasma, this can be explained by the ability of fibrinogen/fibrin to bind thrombin and subsequently inhibit thrombin-mediated FVIII activation (24, 36, 41, 42). In the presence of DAPT, such effect may obviously increase the risk of bleeding too. Besides coagulation factors, circulating cell-derived microparticles also play a role in thrombogenesis (43). However, in this study we did not focus on the contribution of these microparticles to TG potential. In this study, we have shown that TG potential is dependent on multiple coagulation factors and that lower activity of various coagulation factors can lead to impaired TG. This indicates that TG could help in the identification of patients with a high bleeding risk, along with the assessment of other risk factors that are associated with bleeding such as presented in Table 1.
A key strength of the present study is the use of state-of-the-art TG in a real-life setting with prospective documentation of bleeding complications, according to internationally accepted criteria (BARC 2 and 3 bleedings), an important patient-centric outcome with impact on the patients' quality of life. Moreover, we studied high-risk patients receiving DAPT, representing a challenging and complex patient group in whom both bleeding complications and recurrent ischemic events frequently occur. However, several limitations to this study also need to be acknowledged. First, the study population was small, especially in certain subgroups. Nevertheless, the sample size was large enough to find clinically relevant and significant differences between groups. Another limitation is the lost to follow-up of 13 patients within 6 months and missing TG data of 12 patients at T2. However, selection bias is considered unlikely since no relevant differences in baseline characteristics were found between these patients and the included study population (Supplementary Tables 2, 3) and no patients were lost to follow-up because of bleeding or death (as checked with their general practitioner and the hospital's electronic medical records). Next, baseline characteristics of the study population showed (significant) differences in prevalence of anemia, TIA/CVA, and malignancy between patients with and without clinically relevant bleeding. Unfortunately, subgroups were too small to perform a multivariate analysis to adjust for these possible confounders. Although anemia at the time of PCI was found more frequently in the bleeding group, hemoglobin levels at the time of TG measurements were comparable between groups (12.9 and 13.4 g/dl for bleeders and non-bleeders, respectively, p = 0.245). Therefore, it seems unlikely that the hemoglobin levels have affected the differences in TG parameters between groups. TIA/CVA and malignancy are, among others, well-known risk factors for increased bleeding risk after PCI, as was also stated in the recent Academic Research Consortium for High Bleeding Risk (ARC-HBR) consensus document (44). Thus, differences between bleeders and non-bleeders in incidence of these factors were to be expected. Previous studies have shown both negative and positive associations between TIA/CVA and TG parameters, making it difficult to speculate about the possible impact on TG parameters of baseline differences in previous stroke (45, 46). Lastly, it would have been interesting to investigate the effect of DAPT on TG in PRP or whole blood as well. Nevertheless, we have deliberately measured TG in PPP, since this is by far the most used and standardized method of TG measurement and offers the opportunity to rule out the possible role of platelets in this setting.
Conclusion
High clinical-risk patients with DAPT with clinically relevant bleeding during follow-up have (significantly) lower ETP levels, peak height, and velocity index in PPP compared to patients without bleeding, both at 1 and at 6 months after PCI. We have shown that this might at least be partly explained by variation in coagulation factors. Relatively low thrombin generation in these patients acts as a “second hit,” on top of DAPT, thus increasing the bleeding risk. TG performed in PPP may have the potential to aid in the identification of patients with an increased bleeding risk during DAPT. However, more research, with a larger sample size, would be needed to determine cutoff values for TG to be able to adequately identify these patient groups. Furthermore, future research could investigate the capability of other TG-based assays to identify patients with increased bleeding risk during DAPT, e.g., the potential benefit of PRP and/or additional measurement of microparticles.
Data Availability Statement
The raw data supporting the conclusions of this article will be made available by the authors, without undue reservation.
Ethics Statement
The studies involving human participants were reviewed and approved by METC aZM/UM approval number: 11-2-096. The patients/participants provided their written informed consent to participate in this study.
Author Contributions
CB and SZ designed and conceptualized the study. CB wrote the concept of the article, on direct supervision of SZ, HC, and RHO. MV and PM commented on the draft version of the article. CB coordinated all aspects of this research. RGO and YH directed the multiple laboratory assessments. MV, SZ, RHO, HC, CB, LV, and AH were involved in inclusion of patients. All authors contributed to the article and approved the submitted version.
Funding
This work was supported Maastricht University Medical Center+ (MUMC+) and the Cardiovascular Research Institute Maastricht (CARIM).
Conflict of Interest
The authors declare that the research was conducted in the absence of any commercial or financial relationships that could be construed as a potential conflict of interest.
Acknowledgments
The authors would like to thank the laboratory staff of the MUMC+ and the CARIM for their excellent technical assistance.
Supplementary Material
The Supplementary Material for this article can be found online at: https://www.frontiersin.org/articles/10.3389/fcvm.2021.679934/full#supplementary-material
References
1. Valgimigli M, Bueno H, Byrne RA, Collet JP, Costa F, Jeppsson A, et al. 2017 ESC focused update on dual antiplatelet therapy in coronary artery disease developed in collaboration with EACTS: the task force for dual antiplatelet therapy in coronary artery disease of the European society of cardiology (ESC) and of the European association for cardio-thoracic surgery (EACTS). Eur Heart J. (2018) 39:213–60. doi: 10.1093/eurheartj/ehx419
2. Genereux P, Giustino G, Witzenbichler B, Weisz G, Stuckey TD, Rinaldi MJ, et al. Incidence, predictors, and impact of post-discharge bleeding after percutaneous coronary intervention. J Am Coll Cardiol. (2015) 66:1036–45. doi: 10.1016/j.jacc.2015.06.1323
3. Vries MJ, van der Meijden PE, Henskens YM, ten Cate-Hoek AJ, ten Cate H. Assessment of bleeding risk in patients with coronary artery disease on dual antiplatelet therapy. A systematic review. Thromb Haemost. (2016) 115:7–24. doi: 10.1160/TH15-04-0355
4. Redfors B, Ben-Yehuda O, Lin SH, Furer A, Kirtane AJ, Witzenbichler B, et al. Quantifying ischemic risk after percutaneous coronary intervention attributable to high platelet reactivity on clopidogrel (from the assessment of dual antiplatelet therapy with drug-eluting stents study). Am J Cardiol. (2017) 120:917–23. doi: 10.1016/j.amjcard.2017.06.019
5. Degrauwe S, Pilgrim T, Aminian A, Noble S, Meier P, Iglesias JF. Dual antiplatelet therapy for secondary prevention of coronary artery disease. Open Heart. (2017) 4:e000651. doi: 10.1136/openhrt-2017-000651
6. Alfredsson J, Neely B, Neely ML, Bhatt DL, Goodman SG, Tricoci P, et al. Predicting the risk of bleeding during dual antiplatelet therapy after acute coronary syndromes. Heart. (2017) 103:1168–76. doi: 10.1136/heartjnl-2016-310090
7. Wang M, Hao H, Leeper NJ, Zhu L, Early Career C. Thrombotic regulation from the endothelial cell perspectives. Arterioscler Thromb Vasc Biol. (2018) 38:e90–5. doi: 10.1161/ATVBAHA.118.310367
8. Souto JC, Almasy L, Muniz-Diaz E, Soria JM, Borrell M, Bayen L, et al. Functional effects of the ABO locus polymorphism on plasma levels of von Willebrand factor, factor VIII, and activated partial thromboplastin time. Arterioscler Thromb Vasc Biol. (2000) 20:2024–8. doi: 10.1161/01.ATV.20.8.2024
9. Al Dieri R, de Laat B, Hemker HC. Thrombin generation: what have we learned? Blood Rev. (2012) 26:197–203. doi: 10.1016/j.blre.2012.06.001
10. Lisman T, Weeterings C, de Groot PG. Platelet aggregation: involvement of thrombin and fibrin(ogen). Front Biosci. (2005) 10:2504–17. doi: 10.2741/1715
11. Blair P, Flaumenhaft R. Platelet alpha-granules: basic biology and clinical correlates. Blood Rev. (2009) 23:177–89. doi: 10.1016/j.blre.2009.04.001
12. Hemker HC, Giesen P, Al Dieri R, Regnault V, de Smedt E, Wagenvoord R, et al. Calibrated automated thrombin generation measurement in clotting plasma. Pathophysiol Haemost Thromb. (2003) 33:4–15. doi: 10.1159/000071636
13. Dargaud Y, Lienhart A, Meunier S, Hequet O, Chavanne H, Chamouard V, et al. Major surgery in a severe haemophilia A patient with high titre inhibitor: use of the thrombin generation test in the therapeutic decision. Haemophilia. (2005) 11:552–8. doi: 10.1111/j.1365-2516.2005.01141.x
14. Reverter JC, Beguin S, Kessels H, Kumar R, Hemker HC, Coller BS. Inhibition of platelet-mediated, tissue factor-induced thrombin generation by the mouse/human chimeric 7E3 antibody. Potential implications for the effect of c7E3 Fab treatment on acute thrombosis and “clinical restenosis”. J Clin Invest. (1996) 98:863–74. doi: 10.1172/JCI118859
15. Rugeri L, Quelin F, Chatard B, De Mazancourt P, Negrier C, Dargaud Y. Thrombin generation in patients with factor XI deficiency and clinical bleeding risk. Haemophilia. (2010) 16:771–7. doi: 10.1111/j.1365-2516.2010.02246.x
16. Bloemen S, Zwaveling S, Ten Cate H, Ten Cate-Hoek A, de Laat B. Prediction of bleeding risk in patients taking vitamin K antagonists using thrombin generation testing. PLoS ONE. (2017) 12:e0176967. doi: 10.1371/journal.pone.0176967
17. Rugeri L, Beguin S, Hemker C, Bordet JC, Fleury R, Chatard B, et al. Thrombin-generating capacity in patients with von Willebrand's disease. Haematologica. (2007) 92:1639–46. doi: 10.3324/haematol.11460
18. Berezovskaya G, Smirnova O, Malev E, Khromov-Borisov N, Klokova E, Karpenko M, et al. Thrombin generation test for evaluation of antiplatelet treatment in patients with coronary artery disease after percutaneous coronary intervention. Platelets. (2018) 29:185–91. doi: 10.1080/09537104.2017.1294680
19. Wallen NH, Ladjevardi M. Influence of low- and high-dose aspirin treatment on thrombin generation in whole blood. Thromb Res. (1998) 92:189–94. doi: 10.1016/S0049-3848(98)00126-1
20. Herault JP, Dol F, Gaich C, Bernat A, Herbert JM. Effect of clopidogrel on thrombin generation in platelet-rich plasma in the rat. Thromb Haemost. (1999) 81:957–60. doi: 10.1055/s-0037-1614606
21. Kessels H, Beguin S, Andree H, Hemker HC. Measurement of thrombin generation in whole blood–the effect of heparin and aspirin. Thromb Haemost. (1994) 72:78–83. doi: 10.1055/s-0038-1648815
22. Gremmel T, Panzer S, Steiner S, Seidinger D, Koppensteiner R, Pabinger I, et al. Response to antiplatelet therapy is independent of endogenous thrombin generation potential. Thromb Res. (2013) 132:e24–30. doi: 10.1016/j.thromres.2013.04.008
23. Tobin WO, Kinsella JA, Kavanagh GF, O'Donnell JS, McGrath RA, Collins DR, et al. Longitudinal assessment of thrombin generation potential in response to alteration of antiplatelet therapy after TIA or ischaemic stroke. J Neurol. (2013) 260:590–6. doi: 10.1007/s00415-012-6684-2
24. Duchemin J, Pan-Petesch B, Arnaud B, Blouch MT, Abgrall JF. Influence of coagulation factors and tissue factor concentration on the thrombin generation test in plasma. Thromb Haemost. (2008) 99:767–73. doi: 10.1160/TH07-09-0581
25. Vries MJ, Bouman HJ, Olie RH, Veenstra LF, Zwaveling S, Verhezen PW, et al. Determinants of agreement between proposed therapeutic windows of platelet function tests in vulnerable patients. Eur Heart J Cardiovasc Pharmacother. (2017) 3:11–7. doi: 10.1093/ehjcvp/pvw026
26. Mehran R, Rao SV, Bhatt DL, Gibson CM, Caixeta A, Eikelboom J, et al. Standardized bleeding definitions for cardiovascular clinical trials: a consensus report from the bleeding academic research consortium. Circulation. (2011) 123:2736–47. doi: 10.1161/CIRCULATIONAHA.110.009449
27. Ndrepepa G, Schuster T, Hadamitzky M, Byrne RA, Mehilli J, Neumann FJ, et al. Validation of the bleeding academic research consortium definition of bleeding in patients with coronary artery disease undergoing percutaneous coronary intervention. Circulation. (2012) 125:1424–31. doi: 10.1161/CIRCULATIONAHA.111.060871
28. Rodeghiero F, Tosetto A, Abshire T, Arnold DM, Coller B, James P, et al. ISTH/SSC bleeding assessment tool: a standardized questionnaire and a proposal for a new bleeding score for inherited bleeding disorders. J Thromb Haemost. (2010) 8:2063–5. doi: 10.1111/j.1538-7836.2010.03975.x
29. Coen Hemker H, Hemker PW, Al Dieri R. The technique of measuring thrombin generation with fluorescent substrates: 4. The H-transform, a mathematical procedure to obtain thrombin concentrations without external calibration. Thromb Haemost. (2009) 101:171–7. doi: 10.1160/TH08-09-0562
30. Hemker HC, Kremers R. Data management in thrombin generation. Thromb Res. (2013) 131:3–11. doi: 10.1016/j.thromres.2012.10.011
31. Ninivaggi M, Apitz-Castro R, Dargaud Y, de Laat B, Hemker HC, Lindhout T. Whole-blood thrombin generation monitored with a calibrated automated thrombogram-based assay. Clin Chem. (2012) 58:1252–9. doi: 10.1373/clinchem.2012.184077
32. Duarte RCF, Ferreira CN, Rios DRA, Reis HJD, Carvalho MDG. Thrombin generation assays for global evaluation of the hemostatic system: perspectives and limitations. Rev Bras Hematol Hemoter. (2017) 39:259–65. doi: 10.1016/j.bjhh.2017.03.009
33. Clauss A. Rapid physiological coagulation method in determination of fibrinogen. Acta Haematol. (1957) 17:237–46. doi: 10.1159/000205234
34. Pisters R, Lane DA, Nieuwlaat R, de Vos CB, Crijns HJ, Lip GY. A novel user-friendly score (HAS-BLED) to assess 1-year risk of major bleeding in patients with atrial fibrillation: the euro heart survey. Chest. (2010) 138:1093–100. doi: 10.1378/chest.10-0134
35. Al Dieri R, Peyvandi F, Santagostino E, Giansily M, Mannucci PM, Schved JF, et al. The thrombogram in rare inherited coagulation disorders: its relation to clinical bleeding. Thromb Haemost. (2002) 88:576–82. doi: 10.1055/s-0037-1613258
36. Dielis AW, Castoldi E, Spronk HM, van Oerle R, Hamulyak K, Ten Cate H, et al. Coagulation factors and the protein C system as determinants of thrombin generation in a normal population. J Thromb Haemost. (2008) 6:125–31. doi: 10.1111/j.1538-7836.2007.02824.x
37. Dargaud Y, Beguin S, Lienhart A, Al Dieri R, Trzeciak C, Bordet JC, et al. Evaluation of thrombin generating capacity in plasma from patients with haemophilia A and B. Thromb Haemost. (2005) 93:475–80. doi: 10.1160/TH04-10-0706
38. Jesty J, Beltrami E. Positive feedbacks of coagulation: their role in threshold regulation. Arterioscler Thromb Vasc Biol. (2005) 25:2463–9. doi: 10.1161/01.ATV.0000187463.91403.b2
39. Krishnaswamy S. The transition of prothrombin to thrombin. J Thromb Haemost. (2013) 11 (Suppl. 1):265–76. doi: 10.1111/jth.12217
40. Nicolaes GA, Dahlback B. Factor V and thrombotic disease: description of a janus-faced protein. Arterioscler Thromb Vasc Biol. (2002) 22:530–8. doi: 10.1161/01.ATV.0000012665.51263.B7
41. Mosesson MW. Update on antithrombin I (fibrin). Thromb Haemost. (2007) 98:105–8. doi: 10.1160/TH07-04-0274
42. Hemker HC, Al Dieri R, De Smedt E, Beguin S. Thrombin generation, a function test of the haemostatic-thrombotic system. Thromb Haemost. (2006) 96:553–61. doi: 10.1160/TH06-07-0408
43. Bidot L, Jy W, Bidot C Jr, Jimenez JJ, Fontana V, Horstman LL, et al. Microparticle-mediated thrombin generation assay: increased activity in patients with recurrent thrombosis. J Thromb Haemost. (2008) 6:913–9. doi: 10.1111/j.1538-7836.2008.02963.x
44. Urban P, Mehran R, Colleran R, Angiolillo DJ, Byrne RA, Capodanno D, et al. Defining high bleeding risk in patients undergoing percutaneous coronary intervention. Circulation. (2019) 140:240–61. doi: 10.1161/CIRCULATIONAHA.119.040167
45. Loeffen R, Winckers K, Ford I, Jukema JW, Robertson M, Stott DJ, et al. Associations between thrombin generation and the risk of cardiovascular disease in elderly patients: results from the PROSPER study. J Gerontol A Biol Sci Med Sci. (2015) 70:982–8. doi: 10.1093/gerona/glu228
Keywords: thrombin generation, dual antiplatelet therapy, bleeding risk, percutaneous coronary intervention, coagulation factors
Citation: de Breet CPDM, Zwaveling S, Vries MJA, van Oerle RG, Henskens YMC, van't Hof AWJ, van der Meijden PEJ, Veenstra L, ten Cate H and Olie RH (2021) Thrombin Generation as a Method to Identify the Risk of Bleeding in High Clinical-Risk Patients Using Dual Antiplatelet Therapy. Front. Cardiovasc. Med. 8:679934. doi: 10.3389/fcvm.2021.679934
Received: 12 March 2021; Accepted: 04 May 2021;
Published: 10 June 2021.
Edited by:
Jonathan Douxfils, University of Namur, BelgiumReviewed by:
Patricia B. Maguire, University College Dublin, IrelandErich Vinicius De Paula, State University of Campinas, Brazil
Copyright © 2021 de Breet, Zwaveling, Vries, van Oerle, Henskens, van't Hof, van der Meijden, Veenstra, ten Cate and Olie. This is an open-access article distributed under the terms of the Creative Commons Attribution License (CC BY). The use, distribution or reproduction in other forums is permitted, provided the original author(s) and the copyright owner(s) are credited and that the original publication in this journal is cited, in accordance with accepted academic practice. No use, distribution or reproduction is permitted which does not comply with these terms.
*Correspondence: R. H. Olie, renske.olie@mumc.nl