Corrigendum: Arterial Doppler Waveforms Are Independently Associated With Maximal Walking Distance in Suspected Peripheral Artery Disease Patients
- 1Vascular Medicine Unit, CHU Rennes, Rennes, France
- 2Vascular Medicine Unit, CHU Caen Normandie, Caen, France
- 3CHU Rennes, Inserm, CIC 1414 (Clinical Investigation Center), Rennes, France
- 4Univ Rennes, M2S-EA 7470, Rennes, France
- 5Univ Rennes 1, Rennes, France
Objective: Arterial Doppler waveform recordings are commonly used to assess lower extremity arterial disease (LEAD) severity. However, little is known about the relationship between arterial Doppler waveform profiles and patients' walking capacity. The purpose of this study was to assess whether arterial Doppler waveforms are independently associated with maximal walking distance (MWD) in patients experiencing exertional limb symptoms.
Materials and Methods: This cross-sectional study included suspected LEAD patients experiencing exertional limb symptoms. In both lower extremities, arterial Doppler waveforms and ankle-brachial index (ABI) values were obtained from the pedis and tibial posterior arteries. Each arterial flow measurement was ranked using the Saint-Bonnet classification system. Treadmill stress testing (3.2 km/h, 10% slope) coupled with exercise oximetry (Exercise-TcPO2) were used to determine MWD. Delta from rest oxygen pressure (DROP) was calculated. Following treadmill stress testing, post-exercise ABI values were recorded. Univariate and multivariate analyses were used to determine the clinical variables associated with MWD.
Results: 186 patients experiencing exertional limb symptoms (62 ± 12 years and 26.8 ± 4.5 kg/m2) were included between May 2016 and June 2019. Median [25th; 75th] treadmill MWD was 235 [125;500]m. Better arterial Doppler waveforms were associated with better walking distance (p = 0.0012). Whereas, median MWD was 524 [185;525]m in the group that yielded the best Doppler waveforms, it was 182 [125,305]m in the group with the poorest Doppler waveforms (p = 0.0012). MWD was significantly better (p = 0.006) in the patients with the best ABIs. However, arterial Doppler waveforms alone were significantly associated with MWD (p = 0.0009) in the multivariate model. When exercise variables (post-exercise ABI or DROP) were incorporated into the multivariate model, these were the only variables to be associated with MWD.
Conclusion: Of the various clinical parameters at rest, Doppler flow waveform profiles were associated with MWD in suspected LEAD patients. A stronger link was however found between exercise variables and MWD.
Introduction
Lower extremity arterial disease (LEAD) is a common condition that affects an estimated total of over 230 million patients worldwide (1). Half of these patients experience exertional limb symptoms resulting in a negative impact on quality of life (2).
Ankle-brachial pressure index (ABI) at rest is one means of diagnosing LEAD, whereby values equal to or <0.90 are regarded as pathological (3, 4). Lower resting ABI values are also known to be associated with more severe LEAD (5, 6). Several studies have assessed an association between ABI and walking impairment but the results remain controversial (7–11). There are three main explanations for the disparity found: (i) the small sample size of included patients ranging from 14 to 156; (ii) the lack of homogeneity among the populations of interest; and (iii) the variety of testing protocols employed. Indeed, Gardner and colleagues (8) reported correlation between ABI and maximal walking distance (MWD) when assessed according to a graded testing protocol (speed 2 mph at 0% gradient increased by 2% every 2 min) but not when a single-stage protocol (1.5 mph and 7.5% gradient) was employed (8).
Arterial Doppler waveform recordings are commonly used for the purposes of LEAD diagnosis and follow-up (12–14). Arterial Doppler waveforms are influenced by the extent of upstream arterial stenosis (13, 15). Thus, arterial Doppler waveform profiles provide useful information, especially in medial calcific sclerosis patients whose ABI is unreliable (12, 16), as they may be indicative of LEAD severity. As such, walking impairment in LEAD patients could be linked to Doppler waveform profiles. To date, no study has addressed this issue.
If clinical signs or bedside assessments were able to determine functional limitations of patients, this would be of great interest to clinicians insofar as treadmill tests and 6-min walk tests would prove unnecessary.
The aim of this study was therefore to assess the relationship between Doppler waveform profiles and maximal walking distance in: (i) patients experiencing exertional limb symptoms; and (ii) in and confirmed LEAD patients experiencing exertional limb symptoms.
Methods
Ethical Considerations
The present study was conducted from May 2016 to June 2019 and received institutional review board approval (IRB; no. 17.12). All of the participants provided written informed consent. The study protocol is consistent with 1975 Declaration of Helsinki ethical guidelines. The “Exercise PAD” study was registered with the American National Institute of Health database under reference number NCT03186391.
The data substantiating the findings from this study are available from the corresponding author upon reasonable request. The corresponding author had full access to all of the study data and takes responsibility for its integrity and for all data analysis.
Study Design
This cross-sectional single-institution study included consecutive patients referred to the vascular unit of Rennes University Hospital (France) for exertional limb symptoms suggestive of LEAD. The relationship between Doppler waveform profiles and maximal walking distance was assessed in: (i) patients experiencing exertional limb symptoms (suspected LEAD); and (ii) patients experiencing exertional limb symptoms AND at least one hemodynamic criterion for LEAD (confirmed LEAD).
Inclusion and Exclusion Criteria
Patients were included provided they met all of the following criteria:
- clinical history of exertional limb symptoms suggestive of LEAD (i.e., pain in active muscle groups occurring in the course of exercise and lasting for under 10 min);
- arterial Doppler waveform data availability for both limbs;
- ABI data availability for both limbs;
- completion of treadmill exercise testing at 3.2 km/h and 10% slope;
- written informed consent.
Patients were excluded if they met at least one the following criteria:
- treadmill exercise testing discontinued on account of symptoms other than ischemia-induced lower-limb pain (e.g., dyspnea, angina, rheumatic pain)
- incomplete resting ABI and arterial Doppler waveform data.
Characteristics of Patients
Collected variables included age, sex, body mass index, smoking status, comorbidities, and medication (statins, anti-hypertensive treatment, antiplatelets, oral antidiabetic treatment or insulin). Patients were classified as confirmed LEAD if they fulfilled at least one of the following criteria: ABI ≤ 0.90 or Doppler waveform profile other than N or A under the simplified Saint-Bonnet classification system (see below); or a decrease in post-exercise ABI ≥18.5% (see below) or delta from rest oxygen pressure (DROP) ≤-15 mmHg (see below).
ABI Measurement
ABIs were measured by a trained vascular medicine physician (4) consistent with American Heart Association guidelines using a hand-held Doppler probe (8 MHz; Basic Atys Medical, Soucieu en Jarrest, France), with the exception of brachial blood pressure that was measured using an automated oscillometric blood pressure monitor (Carescape Dinamap V100; GE Healthcare) (17). Measurements were taken after a 10-min supine rest period, head and heels supported, in a temperature-controlled room (21°C) (18). The following counterclockwise sequence was used: right brachial artery, right posterior tibial artery, right dorsalis pedis artery, left posterior tibial artery, left dorsalis pedis artery, left brachial artery, and right brachial artery. The ABI was calculated by dividing the highest lower limb pressure (dorsalis pedis or posterior tibial pressures) by the highest arm pressure as recommended (4, 18). The symptomatic lower limb ABI as reported via case history was used for the purposes of analysis.
Arterial Doppler Waveform Recordings
Arterial Doppler waveforms were acquired in both lower extremities from the pedis and tibial posterior arteries before ABI measurement. Each arterial flow measurement was ranked using the Saint-Bonnet classification system as recommended in France by professors from the French College of Vascular Medicine (CEMV) (13, 19). In brief, the simplified version of this classification system portrays types according to arterial lesion severity (Saint-Bonnet N, Saint-Bonnet A, Saint-Bonnet B, Saint-Bonnet CD, Saint-Bonnet E, Saint-Bonnet 0 [i.e., no flow]) (13, 19). The Saint-Bonnet classification system is outlined in Figure 1. Saint-Bonnet N and A are regarded as normal whereas any other type of flow is regarded as symptomatic of LEAD. Only the Doppler waveforms from the pedis and tibial posterior arteries of the symptomatic limb reported via case history were used for analysis. Doppler flow waveforms from the pedis and tibial posterior arteries were ranked in accordance with the Saint-Bonnet classification system. The waveforms ranked closest to N were labeled the “best waveforms” and those ranked closest to E or 0 were labeled the “worst waveforms.” Each limb was thus characterized by two flows: a best flow and a worst flow.
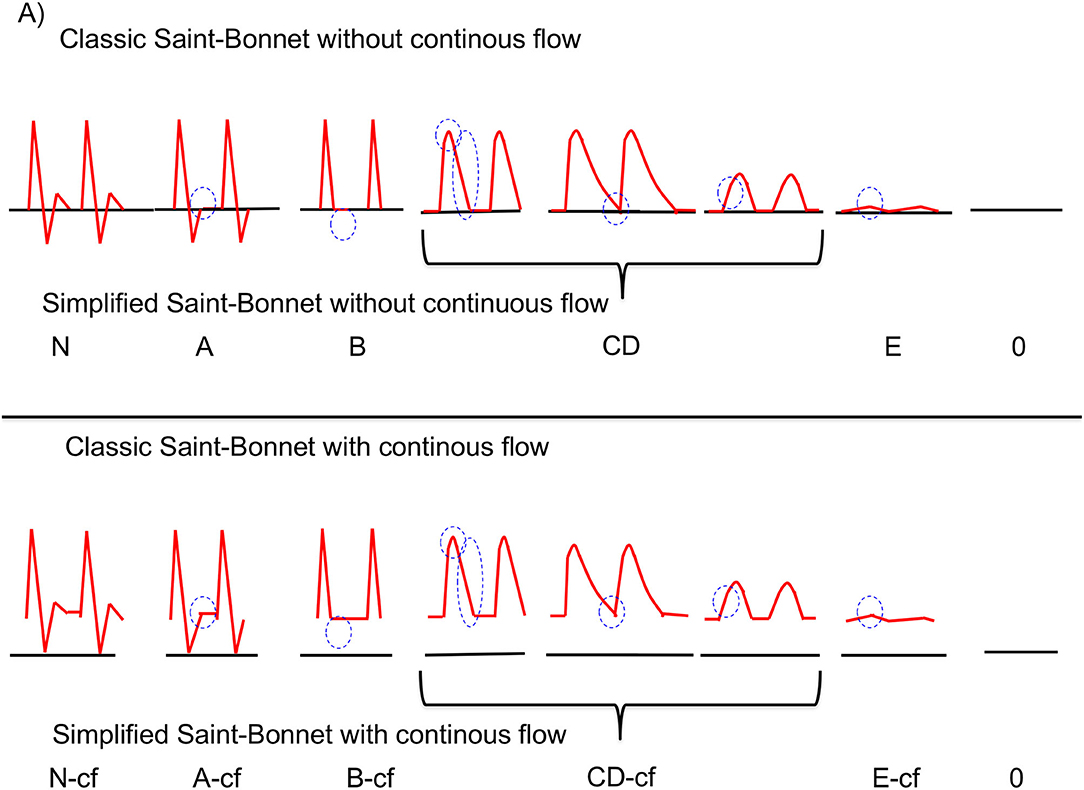
Figure 1. Simplified Saint-Bonnet Classification. Saint-Bonnet classification according to arterial lesion severity (from type N to 0). cf, continuous flow. The blue circles represent the key elements that vary from type to type. Of note: continuous flow may be horizontal or slightly descending. This diagram shows stable continuous flow and is therefore depicted horizontally. For more information please refer to initial Vasa publication (13).
Treadmill Stress Testing Coupled With Exercise Transcutaneous Oxygen Pressure Measurement (Exercise-TcPO2)
A treadmill stress test (3.2 km/h, 10% slope) was used to determine MWD (17). The patients were asked to inform the physician of pain onset (17). Exercise was discontinued when patients reached their thresholds or lasted for a maximal distance of 525 m (over a period of 10 min) (17).
Exercise-TcPO2 was measured during the treadmill stress test as previously documented using calibrated TcPO2 electrodes (TCOM/TcPO2; PF 6000TcPO2/CO2 Unit; Perimed® Jarfalla, Sweden) (20–23). Delta from rest oxygen pressure (DROP) was recorded in each lower extremity in real time by in-house validated Oxymonitor (version 2019.01.05) free software (https://imagemed.univ-rennes1.fr/en/oxymonitor/download) as previously documented (17, 24). As defined in previous studies, DROP ≤-15 mmHg was used to diagnose LEAD (17, 20, 22).
Post-exercise ABI
Readings were taken by two vascular physicians: one reading from the brachial artery using an automatic blood pressure device (Carescape Dinamap V100; GE Healthcare) and one from the lower limb with a handheld Doppler device. Post-exercise brachial pressure was taken from the same artery that was used for ABI measurement at rest. While resting ABI was being measured, a black pen was used to mark the skin area in which the highest lower limb pressure had been recorded by a hand-held Doppler device (25, 26) in order to streamline the post-exercise measurement procedure. As defined in a previous study, a post-exercise decrease in ABI ≥18.5% was used to diagnose LEAD (17).
Statistical Analysis
Data Analysis
Continuous variables were expressed as means ± standard deviation (sd) regarding normal distribution and as median and interquartile range (IQR) values regarding non-normal distribution, and categorical variables were expressed as counts (percentages). With respect to quantitative variables, the Student's t-test or U Mann-Whitney test were used to compare baseline characteristics where two groups were involved and the one-way Anova and Kruskal-Wallis tests where more than two groups were involved; the chi-squared test or Fisher's exact test were used for qualitative variables, subject to distribution. Analysis was performed per patient, rather than per limb and only the symptomatic lower limb reported via case history was included in the analysis. Where both lower limbs were symptomatic, the symptomatic lower limb was selected randomly. In our experience of analyzing of Doppler waveforms, the prevalence of E and 0 Doppler flow waveforms as per Saint-Bonnet classification is low. Thus, Doppler flow waveforms categorized as CD, E or 0 were placed in the same category, in effect reducing the number of flow waveform categories to 4 (N, A, B and CDE0). First and foremost, univariate logistic regression was conducted to define the variables representing the characteristics of patients and at-rest clinical variables (ABI and Doppler waveforms) associated with MWD in the suspected LEAD population in the first instance and in the confirmed LEAD population in the second. Then variables whose p-value was <0.20 were included in multivariate analysis. A backward stepwise procedure was used. A significance threshold of 0.05 was used for all statistical testing. Finally, we incorporated exercise variables (post-exercise ABI and DROP) into the model to assess whether there was a stronger correlation between these variables and MWD in both populations. Statistical analysis was performed using SAS 9.4 software (SAS Institute, Cary, NC, USA) (https://www.sas.com/en_us/home.html).
Results
Population of Interest
Of the 369 consecutive patients who attended the clinic for standard care, 186 patients with exertional limb symptoms were included (Figure 2). The reasons for exclusion and the corresponding proportion of potential participants meeting each criterion were as follows: protocol other than Strandness with a 10% slope and 3.2 km/h (n = 16; 9%); etiologies other than LEAD atherosclerosis (n = 3; 2%); missing ABI or arterial Doppler waveforms data (n = 93; 51%); walking hampered by a condition other than LEAD (n = 41; 22%); and multiple tests (n = 30; 16%).
Characteristics of patients are listed in Table 1. Mean age and body mass index were 62 ± 12 years and 26.8 ± 4.5 kg/m2, respectively. Median treadmill MWD was 235 [133–500]m. Eighteen patients fully completed the treadmill stress test. Patient totals in each Saint-Bonnet category were 21 (11%), 67 (36%), 44 (24%), 52 (28%), 2 (1%), and 0 (0%) pertaining to Saint-Bonnet N, Saint-Bonnet A, Saint-Bonnet B, Saint-Bonnet CD, Saint-Bonnet E, and Saint-Bonnet 0 categories, respectively.
Relationship Between MWD and Arterial Doppler Waveforms
Median MWD from Doppler flow waveform categories are shown in Figure 3. In the suspected LEAD population, better arterial Doppler waveforms (i.e., closer to rank N) were associated with better walking distance (p = 0.0012). The relationship tended to be more marked with respect to the best arterial Doppler waveforms (p = 0.0012) compared with the worst arterial Doppler waveforms (p = 0.0088). A similar trend was found in confirmed LEAD patients yet no statistical significance was observed (best arterial waveform: p = 0.084; worst arterial waveform: p = 0.252).
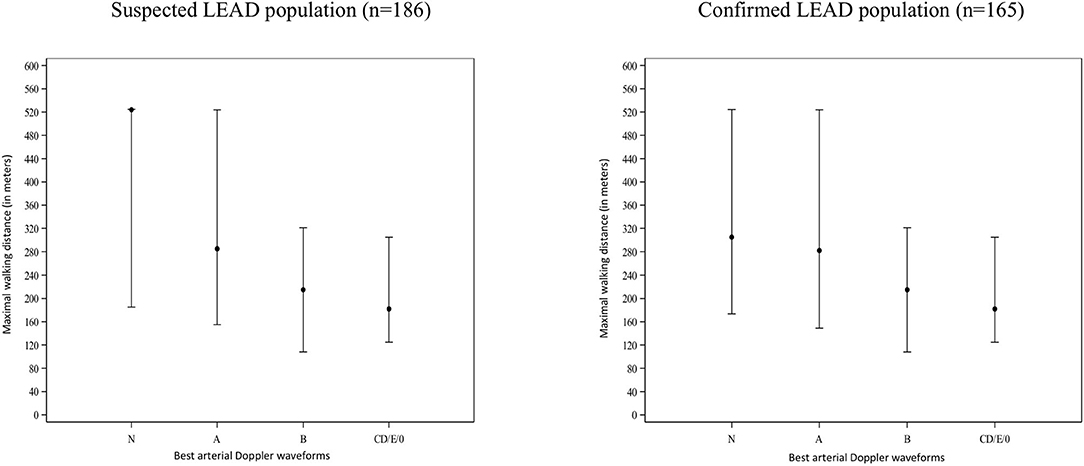
Figure 3. Maximal walking distance and arterial Doppler waveforms in patients suspected of LEAD and in confirmed LEAD. Results shown as medians [Q1 First quartile-Q3 Third quartile]. Simplified Saint-Bonnet classification sorts types according to severity of arterial lesions (Saint-Bonnet N, Saint-Bonnet A, Saint-Bonnet B, Saint-Bonnet CD, Saint-Bonnet E, Saint-Bonnet 0 [i.e., no flow]. Saint-Bonnet N and A are qualified as normal whereas, other types of flow are consistent with LEAD.
Relationship Between MWD and ABI
Median MWD in terms of ABI quartiles are shown in Table 2 for both populations. In the suspected LEAD population, MWD was significantly better in patients from the highest ABI quartile than in patients from the lowest (486 [206–524]m vs. 235 [118–335]m; p = 0.0006). Results of similar statistical significance were found in the confirmed LEAD population (354 [169;524]m vs. 235 [118;335]m; p = 0.048].
Relationship Between ABI and Arterial Doppler Waveforms
In the suspected LEAD population, arterial Doppler waveform distribution in terms of ABI quartiles are shown in Figure 4. Abnormal arterial Doppler waveforms were found in 9 out of 48 patients (19%) with ABI ≥0.99 (regarded as normal ABI) whereas, normal arterial Doppler waveforms were observed in 21 (23%) patients with ABI <0.84 (Table 2). Patients from the highest ABI quartile had higher prevalence of Saint-Bonnet N and A lower limb extremity arterial waveforms than those from the lowest ABI quartile (p < 0.0001). A higher proportion of Saint-Bonnet CDE0 arterial Doppler waveforms were found in the lowest ABI quartile group compared with the highest ABI quartile group (p < 0.0001).
Multivariate Analysis With Regard to Clinical Characteristics and Clinical Readings at Rest
Findings from univariate and multivariate analysis pertaining to patient characteristics and resting clinical readings (ABI and Doppler waveforms) are outlined in Table 3. In the multivariate model, arterial Doppler waveforms alone were associated with MWD (p = 0.0009) in the suspected LEAD population. Analysis of confirmed LEAD patients demonstrated a similar trend regarding arterial Doppler waveforms although no statistical significance was found (p = 0.083). There was no statistical association between ABI and MWD in the LEAD population (p = 0.323).
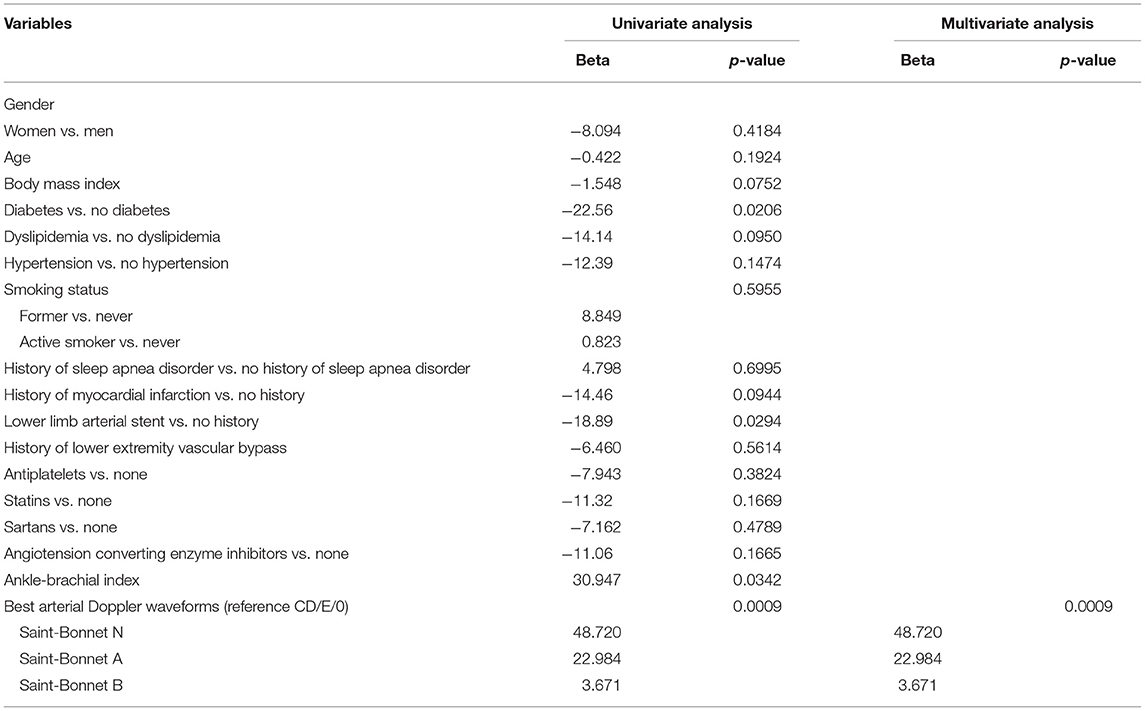
Table 3. Univariate and multivariate analysis findings including patient characteristics and clinical readings at rest (ABI and Doppler waveforms) in suspected LEAD population (n = 186).
Multivariate Analysis in Terms of Clinical Characteristics, Resting Clinical Readings and Exercise Data
When exercise variables (post-exercise ABI or DROP) were added to the multivariate model, exercise variables alone were associated with MWD in the suspected LEAD population (p < 0.001 for post-exercise ABI and p < 0.001 for DROP) and in the confirmed LEAD population (p = 0.016 for post-exercise; p = 0.021 for DROP).
Discussion
Clinical tests, such as ABI and arterial Doppler waveforms analysis are commonly used for LEAD diagnosis and follow-up. The results from the present study suggest that (i) arterial Doppler waveforms are independently associated with MWD in patients suspected of LEAD with exertional limb symptoms; and (ii) arterial Doppler waveforms tended to be associated with MWD in confirmed LEAD patients with exertional limb symptoms.
Clinicians are keen to have at their disposal the clinical wherewithal to determine functional impairment in patients with exertional limb symptoms so as to avoid squandering time on objective treadmill stress testing and make savings from a health policy perspective. To this end, several tools have been made available, such as questionnaires (27, 28), clinical tests (7, 9, 10), and regression equations based on clinical measurement (8, 29).
As previously mentioned, there has been relatively little consensus on any relationship between ABI and MWD in LEAD patients (7–11). Our study findings suggest that there is indeed a relationship between ABI and MWD in patients suspected of LEAD with exertional limb symptoms even when a single stage protocol is used to assess MWD (Strandness 10%; 3.2 km/h) but that any such relationship was not observed in confirmed LEAD patients. It is true that other studies in which a relationship between resting ABI and MWD was established used incremental protocols (8, 10). In our laboratory, a single stage protocol was employed because exercise TcPO2 was measured as part of the treadmill stress test and DROP has only been endorsed in relation to single stage protocols (17, 20, 22, 24).
As shown in Table 2, ABI is apparently a good predictor of walking impairment depending on whether it is normal or otherwise. When ABI is pathological, however, no correlation is observed between ABI and MWD (p > 0.05 regarding the bottom three quartiles). This likely stems from the fact that: (i) ABI can be affected by increased arterial stiffness in patients with diabetes or renal insufficiency (our study included 38 patients with diabetes and 10 with renal insufficiency for instance) (30–32); and (ii) resting ABI is by definition measured at rest and therefore does not reflect exercise hemodynamics.
According to the results of the present study, Doppler flow waveform analysis and categorization as per the Saint-Bonnet classification system is robust, exhibiting a trend that persists among waveforms found in the confirmed LEAD population (n = 165). However, altered arterial Doppler waveforms are not necessarily indicative of low MWD. As demonstrated (Table 2), even in the group with Saint-Bonnet B or CDE0, the third quartiles were high with MWD ranging from 524 to 308 m, respectively. ABI findings yielded a similar result. Poor ABI does not point to low MWD. Such lack of accuracy in resting clinical tests designed to predict MWD may have a physiological explanation. Collateral vessels are believed to enlarge during exercise in animals and humans (33).
Another point of interest arising from this study is that the relationship between Doppler flow waveforms and MWD appears stronger when the healthier symptomatic lower limb extremity artery is investigated (p = 0.0009 for the healthier artery vs. p = 0.008 for the more unhealthy artery). This suggests that MWD could primarily be restricted by the best lower limb arterial flow. There could be several reasons for this: (i) endothelial function in the less damaged artery may be better preserved, meaning that the endothelium-dependent vasodilation mechanisms that operate during exercise could still be present (34); (ii) there may be a more substantial increase in exercise-induced shear stress; and (iii) a collateral arterial network is more likely to arise from a less severely damaged artery. These reasons should incite physicians to consistently assess all limb arteries when evaluating LEAD patients.
As MWD was only assessed via a single stage protocol (10% slope, 3.2 km/h) in the present study, we cannot ascertain that similar results will be found using other protocols (35). However, it would be of particular benefit to assess the relationship between arterial Doppler waveforms and real-life MWD as assessed by GPS or other activity monitors (36, 37) given that real-life MWD and protocol MWD have been shown to differ significantly.
Furthermore, when exercise variables (post-exercise ABI and DROP) were incorporated into the model, these were the only variables that remained significant in both populations. Although not surprising in that post-exercise ABI and DROP reflect hemodynamic changes linked to exercise, this result emphasizes the strength of the relationship between Doppler flow waveform profiles and MWD in as much as only exercise variables seem to yield stronger association.
The heterogeneity of Doppler flow waveform characterization could explain why the prospect of a relationship between arterial Doppler waveforms and MWD has never been previously investigated. Classification systems are a very useful tool in improving the homogeneity of Doppler flow waveform characterization (38–40). The simplified Saint-Bonnet classification system was used in the present study as recommended by professors of the French College of Vascular Medicine (13, 19) the SFMV/SCVE consensus (14), and a study has shown that it yields superior categorization rates by comparison with other classification systems (41).
Limitations
This is not a single-center study, and since our vascular laboratory physicians are highly trained to measure ABI with intra-observer coefficient of variation (CV) for resting ABI equal to 9.4% (typical estimation error is 0.06), and are familiar with the Saint-Bonnet system (19, 42), our results cannot be extrapolated to other operators. Furthermore, our patients' characteristics differ from other studies that have investigated the relationship between ABI and MWD with notably lower mean age and a smaller proportion of patients suffering from diabetes (7–11). Furthermore, our walking test protocol differs from other study protocols. Hence there is likely to be a discrepancy between the results obtained herein and those derived from other walking tests. A further limitation lies in the number of patients included in this study: several patients were excluded for lack of data and only 186 patients were reviewed. Even though this constitutes the one of the largest samples as compared to previous studies addressing the relationship between ABI and MWD, it is nonetheless a small sample (7–11). Moreover, analysis was conducted on two categories of patients: those suspected of LEAD based on treadmill stress test symptoms (i.e., claudication) and so-called “confirmed LEAD” patients based on treadmill stress test symptoms and at least one hemodynamic criterion for LEAD (either ABI or Doppler waveforms, or DROP or post-exercise ABI). It was important to analyze both categories since if only patients with LEAD based on ABI ≤ 0.90 had been investigated, patients with deceptively normal ABI readings would potentially have been excluded. Finally, on the subject of Doppler flow classification, the simplified Saint-Bonnet classification system is a relatively recent system with which many vascular physicians are unfamiliar. In the absence of E and 0 waveforms even greater simplification was required, rendering extrapolation of our results to those categories impossible.
Conclusion
The results of the present study suggest that of the various resting clinical parameters used, Doppler flow waveforms categorized via simplified Saint-Bonnet classification are associated with MWD in patients suspected of LEAD. There is however, a stronger association between exercise variables (post-exercise ABI and DROP) and MWD.
Data Availability Statement
The raw data supporting the conclusions of this article will be made available by the authors, without undue reservation.
Ethics Statement
The studies involving human participants were reviewed and approved by institutional review board (IRB; no. 17.12). The patients/participants provided their written informed consent to participate in this study.
Author Contributions
AL and GM: protocol conception and design. AMi, QT, AG, AC, AMé, and GM: data acquisition. AMi, AL, DL, GM, and EL: data analysis and writing of paper. AMi and GM: data interpretation and drafting of paper. Each author revised the report and approved the submitted version of the manuscript. Each author has agreed both to be personally accountable for the his/her own contribution and to ensure that questions related to the accuracy or integrity of any part of the work, even those in which the author was not personally involved, are appropriately investigated, resolved, and the resolved outcome documented in the literature. All authors contributed to the article and approved the submitted version.
Conflict of Interest
The authors declare that the research was conducted in the absence of any commercial or financial relationships that could be construed as a potential conflict of interest.
Acknowledgments
Several parts of this paper's methods section are similar to method sections described in our previous studies (17, 21, 22).
References
1. Song P, Rudan D, Zhu Y, Fowkes FJI, Rahimi K, Fowkes FGR, et al. Global, regional, and national prevalence and risk factors for peripheral artery disease in 2015: an updated systematic review and analysis. Lancet Glob Health. (2019) 7:e1020–30. doi: 10.1016/S2214-109X(19)30255-4
2. Hirsch AT, Haskal ZJ, Hertzer NR, Bakal CW, Creager MA, Halperin JL, et al. ACC/AHA 2005 Practice Guidelines for the management of patients with peripheral arterial disease (lower extremity, renal, mesenteric, and abdominal aortic): a collaborative report from the American Association for Vascular Surgery/Society for Vascular Surgery, Society for Cardiovascular Angiography and Interventions, Society for Vascular Medicine and Biology, Society of Interventional Radiology, and the ACC/AHA Task Force on Practice Guidelines (Writing Committee to Develop Guidelines for the Management of Patients With Peripheral Arterial Disease): endorsed by the American Association of Cardiovascular and Pulmonary Rehabilitation; National Heart, Lung, and Blood Institute; Society for Vascular Nursing; TransAtlantic Inter-Society Consensus; and Vascular Disease Foundation. Circulation. (2006) 113:e463–654. doi: 10.1161/CIRCULATIONAHA.106.174526
3. Gerhard-Herman MD, Gornik HL, Barrett C, Barshes NR, Corriere MA, Drachman DE, et al. 2016 AHA/ACC guideline on the management of patients with lower extremity peripheral artery disease: a report of the American College of Cardiology/American Heart Association Task Force on clinical practice guidelines. Circulation. (2017) 135:e726–79. doi: 10.1161/CIR.0000000000000470
4. Aboyans V, Criqui MH, Abraham P, Allison MA, Creager MA, Diehm C, et al. Measurement and interpretation of the ankle-brachial index: a scientific statement from the American Heart Association. Circulation. (2012) 126:2890–09. doi: 10.1161/CIR.0b013e318276fbcb
5. Yao ST, Hobbs JT, Irvine WT. Ankle systolic pressure measurements in arterial disease affecting the lower extremities. Br J Surg. (1969) 56:676–9. doi: 10.1002/bjs.1800560910
6. Hiatt WR, Armstrong EJ, Larson CJ, Brass EP. Pathogenesis of the limb manifestations and exercise limitations in peripheral artery disease. Circ Res. (2015) 116:1527–39. doi: 10.1161/CIRCRESAHA.116.303566
7. Regensteiner JG, Hargarten ME, Rutherford RB, Hiatt WR. Functional benefits of peripheral vascular bypass surgery for patients with intermittent claudication. Angiology. (1993) 44:1–10. doi: 10.1177/000331979304400101
8. Gardner AW, Skinner JS, Cantwell BW, Smith LK. Prediction of claudication pain from clinical measurements obtained at rest. Med Sci Sports Exerc. (1992) 24:163–70. doi: 10.1249/00005768-199202000-00002
9. Szuba A, Oka RK, Harada R, Cooke JP. Limb hemodynamics are not predictive of functional capacity in patients with PAD. Vasc Med. (2006) 11:155–63. doi: 10.1177/1358863x06074828
10. McDermott MM, Ferrucci L, Guralnik JM, Dyer AR, Kiang Liu, Pearce WH, et al. The ankle-brachial index is associated with the magnitude of impaired walking endurance among men and women with peripheral arterial disease. Vasc Med. (2010) 15:251–7. doi: 10.1177/1358863X10365181
11. Parr B, Noakes TD, Derman EW. Factors predicting walking intolerance in patients with peripheral arterial disease and intermittent claudication. S Afr Med J. (2008) 98:958–62.
12. Criqui MH, Vargas V, Denenberg JO, Ho E, Allison M, Langer RD, et al. Ethnicity and peripheral arterial disease: the San Diego population study. Circulation. (2005) 112:2703–7. doi: 10.1161/CIRCULATIONAHA.105.546507
13. Mahé G, Boulon C, Desormais I, Lacroix P, Bressollette L, Guilmot J-L, et al. Statement for Doppler waveforms analysis. VASA. (2017) 46:1–9. doi: 10.1024/0301-1526/a000638
14. Mahé G, Boge G, Bura-Rivière A, Chakfé N, Constans J, Goueffic Y, et al. Disparities between international guidelines (AHA/ESC/ESVS/ESVM/SVS) concerning lower extremity arterial disease: consensus of the french society of vascular medicine (SFMV) and the French Society for vascular and endovascular surgery (SCVE). Ann Vasc Surg. (2020). doi: 10.1016/j.avsg.2020.11.011. [Epub ahead of print].
15. Spronk S, den Hoed PT, de Jonge LCW, van Dijk LC, Pattynama PMT. Value of the duplex waveform at the common femoral artery for diagnosing obstructive aortoiliac disease. J Vasc Surg. (2005) 42:236–42. doi: 10.1016/j.jvs.2005.04.048
16. Azzopardi YM, Gatt A, Chockalingam N, Formosa C. Agreement of clinical tests for the diagnosis of peripheral arterial disease. Prim Care Diabetes. (2018) 13:82–6. doi: 10.1016/j.pcd.2018.08.005
17. Stivalet O, Paisant A, Belabbas D, Omarjee L, Le Faucheur A, Landreau P, et al. Exercise testing criteria to diagnose lower extremity peripheral artery disease assessed by computed-tomography angiography. PLoS ONE. (2019) 14:e0219082. doi: 10.1371/journal.pone.0219082
18. Chaudru S, de Müllenheim P-Y, Le Faucheur A, Kaladji A, Jaquinandi V, Mahé G. Training to perform ankle-brachial index: systematic review and perspectives to improve teaching and learning. Eur J Vasc Endovasc Surg. (2016) 51:240–7. doi: 10.1016/j.ejvs.2015.09.005
19. Mahé G, Boulon C, Désormais I, Lacroix P, Bressollette L, Guilmot JL, et al. [College of the French Vascular Medicine Teachers (CEMV) statement: Arterial Doppler waveforms analysis (simplified Saint-Bonnet classification)]. J Med Vasc. (2018) 43:255–61. doi: 10.1016/j.jdmv.2018.05.002
20. Abraham P, Picquet J, Vielle B, Sigaudo-Roussel D, Paisant-Thouveny F, Enon B, et al. Transcutaneous oxygen pressure measurements on the buttocks during exercise to detect proximal arterial ischemia: comparison with arteriography. Circulation. (2003) 107:1896–900. doi: 10.1161/01.CIR.0000060500.60646.E0
21. Mahe G, Kalra M, Abraham P, Liedl DA, Wennberg PW. Application of exercise transcutaneous oxygen pressure measurements for detection of proximal lower extremity arterial disease: a case report. Vasc Med. (2015) 20:251–5. doi: 10.1177/1358863X14567030
22. Koch C, Chauve E, Chaudru S, Le Faucheur A, Jaquinandi V, Mahé G. Exercise transcutaneous oxygen pressure measurement has good sensitivity and specificity to detect lower extremity arterial stenosis assessed by computed tomography angiography. Medicine (Baltimore). (2016) 95:e4522. doi: 10.1097/MD.0000000000004522
23. Mahé G, Kaladji A, Le Faucheur A, Jaquinandi V. Internal iliac artery stenosis: diagnosis and how to manage it in 2015. Front Cardiovasc Med. (2015) 2:33. doi: 10.3389/fcvm.2015.00033
24. Poulin A, Guilcher A, Omarjee L, Jaquinandi V, Gandon Y, Mahe G, Stivalet O. Validation of a software to perform exercise oximetry to diagnose arterial stenosis of the lower limbs. Atherosclerosis. (2018) 278:325–7. doi: 10.1016/j.atherosclerosis.2018.08.025
25. Stivalet O, Laneelle D, Omarjee L, Mahe G. Post-exercise criteria to diagnose lower extremity peripheral artery disease: which one should I use in my practice? Vasc Med. (2019) 24:76–7. doi: 10.1177/1358863X18811932
26. Mahé G, Jaquinandi V. [Diagnosis of lower limb peripheral artery disease]. Presse Med. (2017) 47:47–55. doi: 10.1016/j.lpm.2017.09.021
27. Regensteiner JG, Steiner JF, Panzer RJ, Hiatt WR. Evaluation of walking impairment by questionnaire in patients with peripheral arterial disease. J Vasc Med Biol. (1990) 2:142–52.
28. Fouasson-Chailloux A, Abraham P, Vielle B, Laporte I, Omarjee L, Ouedraogo N. The correlation of the “Walking Estimated-Limitation Calculated by History” (WELCH) questionnaire with treadmill maximal walking time is not impaired by age, in patients with claudication. Qual Life Res. (2015) 24:1857–64. doi: 10.1007/s11136-015-0915-9
29. Gardner AW, Ricci MA, Case TD, Pilcher DB. Practical equations to predict claudication pain distances from a graded treadmill test. Vasc Med. (1996) 1:91–6. doi: 10.1177/1358863X9600100201
30. Suominen V, Uurto I, Saarinen J, Venermo M, Salenius J. PAD as a risk factor for mortality among patients with elevated ABI–a clinical study. Eur J Vasc Endovasc Surg. (2010) 39:316–22. doi: 10.1016/j.ejvs.2009.12.003
31. Emanuele MA, Buchanan BJ, Abraira C. Elevated leg systolic pressures and arterial calcification in diabetic occlusive vascular disease. Diabetes Care. (1981) 4:289–92. doi: 10.2337/diacare.4.2.289
32. Arroyo D, Betriu A, Valls J, Gorriz JL, Pallares V, Abajo M, et al. Factors influencing pathological ankle-brachial index values along the chronic kidney disease spectrum: the NEFRONA study. Nephrol Dial Transplant. (2016) 32:gfw039. doi: 10.1093/ndt/gfw039
33. Prior BM, Lloyd PG, Ren J, Li H, Yang HT, Laughlin MH, et al. Time course of changes in collateral blood flow and isolated vessel size and gene expression after femoral artery occlusion in rats. Am J Physiol Heart Circ Physiol. (2004) 287:H2434–47. doi: 10.1152/ajpheart.00398.2004
35. Nicolaï SPA, Viechtbauer W, Kruidenier LM, Candel MJJM, Prins MH, Teijink JAW. Reliability of treadmill testing in peripheral arterial disease: a meta-regression analysis. J Vasc Surg. (2009) 50:322–9. doi: 10.1016/j.jvs.2009.01.042
36. Le Faucheur A, Abraham P, Jaquinandi V, Bouyé P, Saumet JL, Noury-Desvaux B. Measurement of walking distance and speed in patients with peripheral arterial disease: a novel method using a global positioning system. Circulation. (2008) 117:897–04. doi: 10.1161/CIRCULATIONAHA.107.725994
37. de Müllenheim P-Y, Chaudru S, Mahé G, Prioux J, Le Faucheur A. Clinical interest of ambulatory assessment of physical activity and walking capacity in peripheral artery disease: physical activity and walking capacity in PAD. Scand J Med Sci Sports. (2016) 26:716–30. doi: 10.1111/sms.12512
38. Scissons R. Characterizing triphasic, biphasic, and monophasix Doppler waveforms: Should a simple task be so difficult? J Diagn Med Sonogr. (2008) 24:269–76. doi: 10.1177/8756479308323128
39. Omarjee L, Stivalet O, Hoffmann C, Scissons R, Bressollette L, Mahé G, et al. Heterogeneity of Doppler waveforms description is decreased with the use of a dedicated classification. VASA Zeitschrift fur Gefasskrankheiten. (2018) 47:471–4. doi: 10.1024/0301-1526/a000724
40. Wen C, Gao M, Fu Y, Zhao R, Tong Y, Scissons R, et al. A high variability of arterial Doppler waveform descriptions exists in China. Vasc Med. (2020) 25:221–2. doi: 10.1177/1358863X20903808
41. Guilcher A, Lanéelle D, Hoffmann C, Guillaumat J, Constans J, Bressollette L, et al. Comparison of the use of arterial doppler waveform classifications in clinical routine to describe lower limb flow. J Clin Med. (2021) 10:464. doi: 10.3390/jcm10030464
Keywords: Doppler waveforms, peripheral artery disease, maximal walking distance, ankle-, brachial index, peripheral artery (occlusive) disease
Citation: Miossec A, Tollenaere Q, Lanéelle D, Guilcher A, Métairie A, Le Pabic E, Carel A, Le Faucheur A and Mahé G (2021) Arterial Doppler Waveforms Are Independently Associated With Maximal Walking Distance in Suspected Peripheral Artery Disease Patients. Front. Cardiovasc. Med. 8:608008. doi: 10.3389/fcvm.2021.608008
Received: 18 September 2020; Accepted: 16 March 2021;
Published: 20 April 2021.
Edited by:
Marianne Brodmann, Medical University of Graz, AustriaReviewed by:
Christine Espinola-Klein, Johannes Gutenberg University Mainz, GermanyKara Annette Thompson, University of Texas MD Anderson Cancer Center, United States
Copyright © 2021 Miossec, Tollenaere, Lanéelle, Guilcher, Métairie, Le Pabic, Carel, Le Faucheur and Mahé. This is an open-access article distributed under the terms of the Creative Commons Attribution License (CC BY). The use, distribution or reproduction in other forums is permitted, provided the original author(s) and the copyright owner(s) are credited and that the original publication in this journal is cited, in accordance with accepted academic practice. No use, distribution or reproduction is permitted which does not comply with these terms.
*Correspondence: Guillaume Mahé, maheguillaume@yahoo.fr