- 1Department of Coronary Heart Disease, Fuwai Hospital, State Key Laboratory of Cardiovascular Disease, National Centre for Cardiovascular Diseases, Chinese Academy of Medical Sciences and Peking Union Medical College, Beijing, China
- 2Magnetic Resonance Centre, Fuwai Hospital, State Key Laboratory of Cardiovascular Disease, National Centre for Cardiovascular Disease, Chinese Academy of Medical Sciences and Peking Union Medical College, Beijing, China
- 3Xiamen Cardiovascular Hospital, Xiamen University, Fujian, China
- 4Fuwai Hospital, Chinese Academy of Medical Sciences, Shenzhen, China
Objective: Left ventricular systolic dysfunction (LVSD) after ST-segment elevation myocardial infarction (STEMI) is associated with poor outcome. Trimethylamine N-oxide (TMAO), a gut metabolite, is linked to cardiovascular diseases but its relationship with LVSD after STEMI remains unclear. The present study therefore aimed to investigate the relationship between TMAO and LVSD at 30 days after a first anterior STEMI.
Methods: This was a sub-study from the OCTAMI (Optical Coherence Tomography Examination in Acute Myocardial Infarction) registry. Eligible patients were included in current study if they: (1) presented with a first anterior STEMI; (2) had available baseline TMAO concentration; (3) completed a cardiovascular magnetic resonance examination at 30 days after STEMI. LVSD was defined as left ventricular ejection fraction < 50%. Associations between TMAO and left ventricular ejection fraction, infarct size and left ventricular global strain were examined.
Results: In total, 78 patients were included in final analysis. Overall, TMAO was moderately associated with peak cTnI (r = 0.27, p = 0.01), age (r = 0.34, p < 0.01), and estimated glomerular filtration rate (r = −0.30, p < 0.01). At 30-day follow-up, 41 patients were in the LVSD group and 37 in the non-LVSD group. Baseline TMAO levels were not significantly different between the two groups (LVSD vs. non-LVSD: median 1.9 μM, 25−75th percentiles 1.5–3.3 μM vs. median 1.9 μM, 25−75th percentiles 1.5–2.7 μM; p = 0.46). Linear regression analyses showed that TMAO was not associated with left ventricular ejection fraction, infarct size or left ventricular global strain at 30 days (all p > 0.05).
Conclusions: TMAO was not significantly correlated with 30-day LVSD in patients with a first anterior STEMI after primary revascularization.
Clinical Trial Registration: www.ClinicalTrials.gov, identifier: NCT03593928.
Introduction
Left ventricular systolic dysfunction (LVSD) and heart failure are frequently observed after ST-segment elevation myocardial infarction (STEMI) (1–3) and patients with left anterior descending artery as the culprit vessel face even higher risks (4). Thus, it's important to optimize risk stratification after STEMI and find out novel treatment targets for preventing heart failure.
Trimethylamine N-oxide (TMAO), a gut-derived metabolite (5), has been related to long-term cardiovascular risks in various populations (6–14), including those with established chronic heart failure (7, 12, 14) or acute heart failure (9). However, TMAO was unable to predict risks of death and recurrent myocardial infarction at 6 months after acute myocardial infarction (11) or to predict cardiovascular risks in patients with type 1 diabetes mellitus (15), high risk type 2 diabetes mellitus (16), or previous myocardial infarction (17). It was also reported that TMAO was associated with advanced left ventricular diastolic dysfunction, but not with systolic dysfunction in patients with chronic systolic heart failure (8). Results from animal studies remain controversial. Organ and her colleagues reported that TMAO exacerbated pulmonary edema, cardiac enlargement and left ventricular ejection fraction (LVEF) decline in pressure-overloaded mice heart failure model (18). Further, they showed that removing dietary TMAO had a beneficial effect on the myocardium in another murine heart failure model (19). In contrast, a four- to five-fold increased plasma TMAO alleviated diastolic dysfunction in pressure-overloaded rat heart failure (20). Nevertheless, there was a negative effect of TMAO on aortic atherosclerotic lesions in ApoE knockout mice (21). Evidence to date supports detrimental effects of TMAO that include promoting inflammation (22–24), impairing cardiomyocyte (25), disrupting energy metabolism and increasing oxidative stress (26), which may play a role in heart failure after myocardial infarction.
Cardiovascular magnetic resonance (CMR) has become an intriguing imaging modality by providing a comprehensive and multifaceted evaluation of the heart (27). LVEF is widely used in clinical practice and CMR provides more accurate measurements compared to echocardiography (28). CMR allows the unique detection of infarcted size or fibrosis by late gadolinium enhancement (LGE) (29) and features of injured myocardium such as microvascular obstruction (MVO) and intramyocardial hemorrhage (30). Left ventricular strain by CMR feature tracking analysis has emerged in the past decade as a useful tool for quantitative evaluation of deformation (31) and provides additional prognostic values following myocardial infarction (32).
Therefore, we aimed to investigate the possible association between baseline TMAO and consequential development of LVSD at 30 days, as assessed by CMR, in patients after a first anterior STEMI and primary percutaneous coronary intervention.
Methods
Study Population
Data were used from the OCTAMI (Optical Coherence Tomography Examination in Acute Myocardial Infarction) registry (NCT03593928), which enrolled a prospective, consecutive, observational, single-center cohort of patients with STEMI for optical coherence tomography examination at the culprit lesion (33–35). Study flow is shown in Figure 1. We approached all patients from the registry who presented with a first anterior STEMI and had available baseline TMAO level and 30-day CMR follow-up between March 2017 and July 2018. STEMI was defined as persistent symptoms suggesting myocardial ischemia, elevated troponin I levels, and ST-segment elevation in at least 2 contiguous leads or new left bundle branch block on the 18-lead electrocardiogram (36). Baseline LVEF was measured by echocardiography as part of standard routine and results were extracted from electronic medical records. Left ventricular evaluation by CMR was scheduled at 30 days after the index STEMI. This study complied with the principles of the Declaration of Helsinki and was approved by the review board at Fuwai Hospital. All patients provided written informed consent.
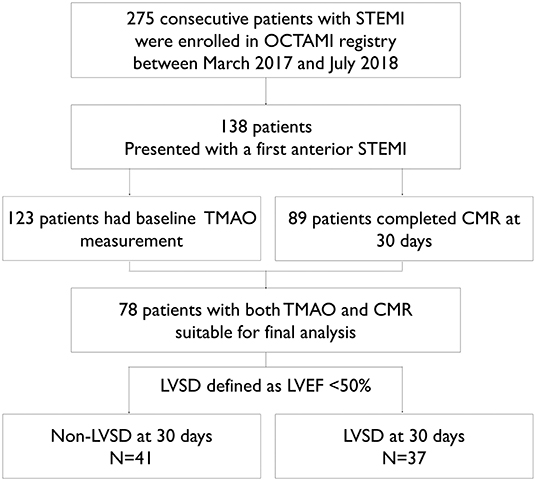
Figure 1. Study flow. CMR indicates cardiovascular magnetic resonance; LVEF, left ventricular ejection fraction; LVSD, left ventricular systolic dysfunction; OCTAMI registry, Optical Coherence Tomography Examination in Acute Myocardial Infarction registry (NCT03593928); STEMI, ST-segment elevation myocardial infarction; TMAO, trimethylamine-N-oxide.
Procedural Data
Patients received initial oral treatment of 300 mg aspirin, 180 mg ticagrelor or 600 mg clopidogrel, and intravascular infusions of 75–100 IU/kg heparin prior to coronary angiography. Percutaneous coronary intervention was performed via radial or femoral access. Infusions of glycoprotein IIb/IIIa receptor inhibitors were at the discretion of the operators. The culprit vessel was determined primarily by coronary angiography and corroborated with electrocardiogram and echocardiography.
Biomarker Analysis
Peripheral blood samples were collected before heparinization using vacutainer tubes containing ethylenediamine tetra-acetic acid, maintained at 4°C and processed within 3 h. Then, plasma samples were stored at −80°C and thawed once for analysis. Plasma levels of TMAO were quantified by stable isotope dilution high performance liquid chromatography with online tandem electrospray ionization mass spectrometry using an API 3200 triple quadrupole mass spectrometer (AB SCIEX, Framingham, MA) with a d9-(trimethyl)-labeled internal standard as described previously (37). In addition, cardiac troponin I (cTnI) and NT-proBNP were measured as routine biochemical work-up during the hospital stay. As a standard care, cTnI was measured at least 3 times within 24 h after admission and daily until normalization and then every 2 days before discharge; NT-proBNP was measured on admission, immediately after and on the second day after the index procedure and then up to physicians. Results of cTnI and NT-proBNP during hospitalization were retrieved from electronic medical records and baseline values and peak values were reported.
CMR Image Acquisition
CMR imaging was performed on a 3.0-Tesla scanner (Discovery MR750, GE Healthcare, Milwaukee, USA) with a phased-array cardiovascular coil, using electrocardiographic and respiratory gating. Cine images were acquired in three long-axis views (two-chamber, four-chamber, and left ventricular outflow tract) and short-axis views encompassing the entire left ventricle, using balanced steady state free precession sequence. Acquisition parameters were as follows: 3.3/1.7 ms repetition time/echo time; 320*320 mm field of view; 224*192 matrix; 50° flip angle; 46–60 ms temporal resolution; 8 mm slice thickness; 2 mm slice gap. LGE images were acquired 10 to 15 min after intravenous administration of gadolinium-DTPA (Magnevist, Bayer, Berlin, Germany) at a dose of 0.2 mmol/kg, using a segmented phase-sensitive inversion recovery Turbo Fast Low Angle Shot sequence at the same views as cine images in end diastole. Typical imaging parameters were: 360*360 mm field of view; 224*192 matrix, 6.0/2.8 ms TR/TE time; 25° flip angle; 8 mm slice thickness; 2 mm slice gap; 300 ms TI.
CMR Image Analysis
All CMR images were analyzed with CVI42 (Circle Cardiovascular Imaging Inc., Calgary, Canada) by radiologists with more than 5-year experience of CMR imaging. Infarct size was quantified by LGE detected as +5 SDs over the signal intensity of normal myocardium and the percentage of enhanced myocardium mass of left ventricular mass was recorded. MVO was identified as areas of hypointensity within the region of hyperenhanced infarct tissue. Both the presence of MVO and quantitative measurements as percentage of left ventricular mass were reported. Feature tracking analysis provided peak left ventricular global strain parameters, including: global radial strain (GRS), global circumferential strain (GCS) and global longitudinal strain (GLS). Briefly, endocardial and epicardial contours of the left ventricle throughout the cardiac cycle were automatically tracked by contours that were manually drawn at end diastole. Papillary muscles were assigned to the left ventricular volume. Other cardiac parameters were computed automatically based on manually traced endocardial and epicardial contours of left ventricular myocardium on short-axis cine at end diastole and end systole, respectively. Inter-observer and intra-observer variability analyses of CMR strain were determined from 10 randomly selected STEMI patients (data not shown).
Statistical Analysis
Continuous variables were presented as means with standard deviations (SD) or medians with 25th and 75th percentiles and were compared between two groups with t test or Mann Whitney test. In addition, Kruskal–Wallis test was applied to comparing continuous variables among four groups. Categorical variables were presented as counts and percentages and were compared with chi-square test. LVSD was defined as LVEF <50%. Bivariate correlations were evaluated with Pearson's correlation. Univariate and multivariate linear regression analyses were performed to detect possible associations between TMAO and left ventricular parameters (LVEF, infarct size, MVO, GCS, GRS, GLS) at 30 days. Model 1 included adjustments for age and estimated glomerular filtration rate (eGFR), and Model 2 included adjustments for age, eGFR, peak cTnI and peak NT-proBNP. A 2-tailed p < 0.05 was considered statistically significant. All statistical analyses were performed using SPSS software, version 24 (IBM, Armonk, NY).
Results
Baseline Characteristics
From March 2017 to July 2018, 275 patients were enrolled in the OCTAMI registry and 138 of them presented with a first anterior STEMI. Finally, seventy-eight patients with both baseline TMAO level and 30-day CMR follow-up were qualified for the current study. Comparisons of baseline characteristics between included and excluded groups of patients with a first anterior STEMI were reported in Supplementary Table 1. Among the seventy-eight patients, the median TMAO level was 1.9 μM with 25th percentile of 1.5 μM and 75th percentile of 2.9 μM. According to LVEF at 30 days, 41 patients were in the LVSD group and 37 in the non-LVSD group. Baseline characteristics between groups are shown in Table 1. There was no significant difference of TMAO levels between the two groups. Baseline LVEF was similar between the two groups. However, the LVSD group had higher peak NT-proBNP, and higher peak cTnI. Moreover, there was a significantly higher percentage of females in the group of LVSD. No significant differences between groups were observed regarding age, body mass index, medical history, procedural data, and medications at discharge.
Associations of TMAO With Traditional Biomarkers
Bivariate relationships between TMAO and traditional biomarkers are presented in Figure 2. TMAO was not associated with peak NT-proBNP (r = 0.06, p = 0.63), but had a statistically significant and moderate association with peak cTnI (r = 0.32, p = 0.01). Besides, TMAO was also significantly correlated with age (r = 0.34, p < 0.01) and eGFR (r = −0.30, p = 0.01) in the current study cohort.
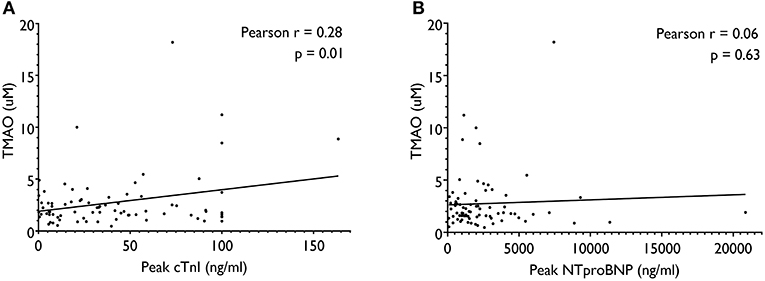
Figure 2. Pearson correlations between TMAO and traditional biomarkers, including peak cTnI (A) and peak NT-proBNP (B). cTnI indicates cardiac troponin I; NT-proBNP, N-terminal pro B-type Natriuretic Peptide; TMAO, trimethylamine-N-oxide.
CMR Parameters at 30-Day Follow-Up
Between-group levels of left ventricular CMR parameters are shown in Table 2. The LVSD group, compared with the non-LVSD group, presented significantly larger end-systolic volume, larger end-diastole volume, and larger infarct size. We observed no significant difference of MVO presence or MVO percentages between the two groups. In accordance with traditional parameters, left ventricular feature tracking strain analyses showed that GRS, GCS, and GLS were significantly worse in patients with LVSD than in those without LVSD at 30 days. In bivariate correlation analysis, GRS, GCS, and GLS were significantly associated with peak cTnI and peak NT-proBNP but not with TMAO (Table 3).
Relationship Between TMAO and LVSD
Baseline TMAO levels between patients with and without LVSD at 30 days were not significantly different (Figure 3B). Then, we re-grouped patients according to LVEF at baseline and we observed no significant differences of TMAO levels between groups (Figure 3A). We further grouped patients according to the change of LVEF from baseline to 30 days. As a result, twenty-six patients had no LVSD at baseline or at 30 days (Group a); twenty-three patients had LVSD solely at 30 days (Group b); eleven patients had LVSD solely at baseline (Group c); and eighteen patients presented persistent LVSD at both baseline and at 30 days (Group d). Nevertheless, there were no significant differences of TMAO levels among the four groups (Figure 3C). In linear regression analyses (Table 4), TMAO was not significantly correlated with LVEF, infarct size, MVO, GRS, GCS, or GLS at 30 days.
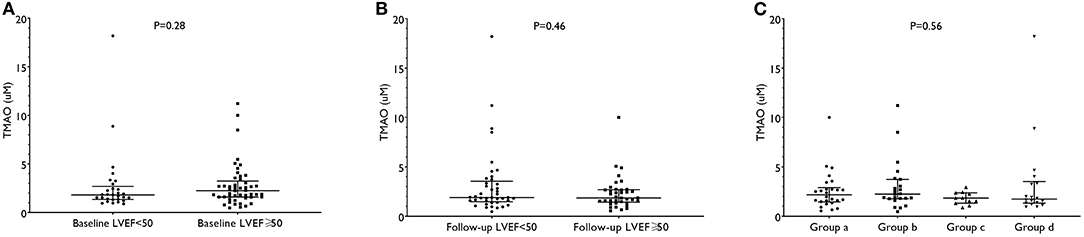
Figure 3. TMAO levels grouped by LVSD at baseline (A), at 30-day follow-up (B) and both (C). In (C), groups are defined as follows: Group a includes patients not presenting LVSD at both baseline and 30 days; Group b includes patients presenting with LVSD solely at 30 days; Group c includes patients presenting with LVSD solely at baseline; Group d includes patients presenting persistent LVSD at both baseline and 30-days. LVEF indicates left ventricular ejection fraction; LVSD, left ventricular systolic dysfunction; TMAO, trimethylamine-N-oxide.
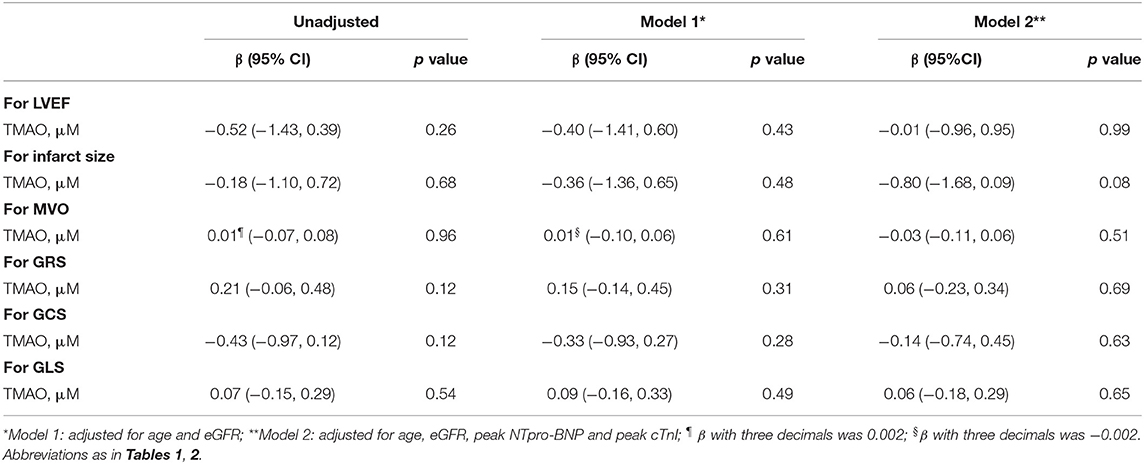
Table 4. Linear regression analyses of associations between TMAO and left ventricular parameters at 30 days.
Discussion
The present study showed that TMAO levels were not significantly different between patients with or without LVSD at 30 days after a first anterior STEMI. Although TMAO was significantly and moderately associated with peak cTnI, it had no statistically significant association with LVEF, infarct size, MVO or left ventricular global strain at 30 days in the current study cohort.
TMAO is a metabolite derived from gut flora (5) and is related to cardiovascular diseases (6–11, 14). However, its prognostic utility remains controversial, due to different follow-up intervals (11), study populations (16, 17, 38), geographical regions (10, 39), and ethnicities (40, 41). In addition, it has been reported that the core intestinal microbiota in patients with heart failure were usually altered (42), resulting in significantly increased circulating TMAO levels (43). It is intriguing that Tang et al. reported that in chronic heart failure, TMAO was associated with advanced left ventricular diastolic dysfunction, but not systolic dysfunction or inflammatory biomarkers (8). Consistently, the present study showed no significant relationship between baseline TMAO level and 30-day LVSD in patients with a first anterior STEMI. To note, the average level of TMAO in current study was relatively lower compared with other studies except for that reported by Liu et al. (44), which might result from ethnic differences and regional diet characteristics (45). Moreover, the predictive value of TMAO for cardiovascular events was concentration-dependent, and probably with a threshold-type association, as Salzano et al. reported that TMAO as a continuous variate was not associated with a composite outcome of mortality and hospitalization for heart failure but TMAO level exceeding a cut-off of 5 mmol/L was significantly associated with fourfold increased event rates (46). Similarly, another study (10) included two independent large cohort, the Cleveland Cohort and the Swiss Cohort, and results showed that patients in the third quartile of TMAO level in the former cohort but not those in the latter cohort presented significant higher cardiovascular risks, which might be due to a higher TMAO level in the former cohort than in the latter one (the third quartile in the former cohort: 4.28–7.89 uM; the third quartile in the latter cohort: 2.87–4.84 uM). Although previous studies reported TMAO as a prognostic biomarker in patients with heart failure, only a few studies (15–17, 38) evaluated TMAO in patients without established heart failure and surprisingly, these studies all reported non-significant prognostic value of TMAO for cardiovascular outcomes after adjustments for traditional parameters. To our knowledge, our study is the first to investigate the role of TMAO in LVSD after a first anterior STEMI and we did not observe a significant relationship between the two. Further, a recent bidirectional mendelian randomization analysis between TMAO and cardiometabolic diseases supported that type 2 diabetes mellitus and chronic kidney diseases increased TMAO and that observational evidence for TMAO's role in cardiovascular diseases probably resulted from confounders or reverse casualty (47).
TMAO is also linked to the pathogenesis of atherosclerosis by promoting macrophage cholesterol accumulation and altering sterol metabolism in mice models (48). However, we did not observe correlations between TMAO and baseline cholesterol levels. Besides, we observed significant correlations between TMAO level with age, eGFR and peak cTnI. As we previously reported, higher TMAO level was related with higher prevalence of plaque rupture (34) and heavier atherosclerotic burden (33), which were reported to increase the risk of impaired myocardial reflow (49, 50). On the other hand, there was no significant association between peak NT-proBNP and TMAO in the present study. However, Tang et al. reported a significant correlation between TMAO and B-type natriuretic peptide measured from fasting blood samples in chronic heart failure patients (7). This difference might result from different disease scenarios and distinct sampling times.
Several limitations warrant mention. First, this was not a pre-specified sub-analysis and results should be considered as hypothesis generating and be interpreted with caution. Second, we enrolled a limited number of patients in the current study. However, all patients were from a continuously screened and prospectively collected STEMI cohort, which could minimize selection bias. Third, CMR was limited to 30-day follow-up, and not performed during hospitalization. Thus, we collected baseline LVEF from echocardiography for grouping patients but not in further correlation analysis or regression analysis. It was also reported that in patients with anterior STEMI, LVEF measurements from echocardiography and CMR were within acceptable limits of agreement (51). Finally, the intrinsic nature of observational studies was insufficient to draw or deny a causal relationship between TMAO and short term LVSD. Future studies with larger sample size and possible interventions on TMAO levels are needed.
Conclusion
In conclusion, baseline TMAO levels were not significantly associated with LVEF, infarct size, or left ventricular global strain at 30-day follow-up in patients with first ever anterior STEMI after primary percutaneous coronary interventions. Although TMAO was moderately correlated with peak cTnI, it was not associated with left ventricular systolic dysfunction at 30 days follow-up.
Data Availability Statement
The original contributions presented in the study are included in the article/Supplementary Materials, further inquiries can be directed to the corresponding author/s.
Ethics Statement
The studies involving human participants were reviewed and approved by the Review Board of Fuwai Hospital. The patients/participants provided their written informed consent to participate in this study.
Author Contributions
JZ analyzed and interpreted the complete data, and was a major contributor in writing the manuscript. SY analyzed and interpreted the patient data regarding cardiovascular magnetic resonance imaging results, and was a major contributor in writing the manuscript. YT contributed to the analysis of baseline TMAO concentration. PZ and CL played a leading role in patient enrolment and conducting the registry study. ZS, JL, and RC collected and analyzed the patient data regarding clinical characteristics. HY and SZ supervised the study and were responsible in funding support. All authors contributed to the article and approved the submitted version.
Funding
This work was supported by the Chinese Academy of Medical Sciences Innovation Fund for Medical Sciences (2016-I2M-1-009) and grants from National Natural Science Foundation of China (Nos. 81970308, 81930044, and 81620108015).
Conflict of Interest
The authors declare that the research was conducted in the absence of any commercial or financial relationships that could be construed as a potential conflict of interest.
Supplementary Material
The Supplementary Material for this article can be found online at: https://www.frontiersin.org/articles/10.3389/fcvm.2020.613684/full#supplementary-material
References
1. McManus DD, Chinali M, Saczynski JS, Gore JM, Yarzebski J, Spencer FA, et al. 30-year trends in heart failure in patients hospitalized with acute myocardial infarction. Am J Cardiol. (2011) 107:353–9. doi: 10.1016/j.amjcard.2010.09.026
2. Desta L, Jernberg T, Lofman I, Hofman-Bang C, Hagerman I, Spaak J, et al. Incidence, temporal trends, and prognostic impact of heart failure complicating acute myocardial infarction. The SWEDEHEART Registry (Swedish web-system for enhancement and development of evidence-based care in heart disease evaluated according to recommended therapies): a study of 199,851 patients admitted with index acute myocardial infarctions, 1996 to 2008. JACC Heart Fail. (2015) 3:234–42. doi: 10.1016/j.jchf.2014.10.007
3. Harjola VP, Parissis J, Bauersachs J, Brunner-La Rocca HP, Bueno H, Celutkiene J, et al. Acute coronary syndromes and acute heart failure: a diagnostic dilemma and high-risk combination. A statement from the Acute Heart Failure Committee of the Heart Failure Association of the European Society of Cardiology. Eur J Heart Fail. (2020) 22:1298–314. doi: 10.1002/ejhf.1831
4. Gho J, Postema PG, Conijn M, Bruinsma N, de Jong J, Bezzina CR, et al. Heart failure following STEMI: a contemporary cohort study of incidence and prognostic factors. Open Heart. (2017) 4:e000551. doi: 10.1136/openhrt-2016-000551
5. Wang Z, Klipfell E, Bennett BJ, Koeth R, Levison BS, Dugar B, et al. Gut flora metabolism of phosphatidylcholine promotes cardiovascular disease. Nature. (2011) 472:57–63. doi: 10.1038/nature09922
6. Tang WH, Wang Z, Levison BS, Koeth RA, Britt EB, Fu X, et al. Intestinal microbial metabolism of phosphatidylcholine and cardiovascular risk. N Engl J Med. (2013) 368:1575–84. doi: 10.1056/NEJMoa1109400
7. Tang WHW, Wang Z, Fan Y, Levison B, Hazen JE, Donahue LM, et al. Prognostic value of elevated levels of intestinal microbe-generated metabolite trimethylamine-N-oxide in patients with heart failure. J Am Coll Cardiol. (2014) 64:1908–14. doi: 10.1016/j.jacc.2014.02.617
8. Tang WH, Wang Z, Shrestha K, Borowski AG, Wu Y, Troughton RW, et al. Intestinal microbiota-dependent phosphatidylcholine metabolites, diastolic dysfunction, and adverse clinical outcomes in chronic systolic heart failure. J Card Fail. (2015) 21:91–6. doi: 10.1016/j.cardfail.2014.11.006
9. Suzuki T, Heaney LM, Bhandari SS, Jones DJL, Ng LL. Trimethylamine N-oxide and prognosis in acute heart failure. Heart. (2016) 102:841–8. doi: 10.1136/heartjnl-2015-308826
10. Li XS, Obeid S, Klingenberg R, Gencer B, Mach F, Raber L, et al. Gut microbiota-dependent trimethylamine N-oxide in acute coronary syndromes: a prognostic marker for incident cardiovascular events beyond traditional risk factors. Eur Heart J. (2017) 38:814–24. doi: 10.1093/eurheartj/ehw582
11. Suzuki T, Heaney LM, Jones DJ, Ng LL. Trimethylamine N-oxide and risk stratification after acute myocardial infarction. Clin Chem. (2017) 63:420–8. doi: 10.1373/clinchem.2016.264853
12. Suzuki T, Yazaki Y, Voors AA, Jones DJL, Chan DCS, Anker SD, et al. Association with outcomes and response to treatment of trimethylamine N-oxide in heart failure (from BIOSTAT-CHF). Eur J Heart Fail. (2018) 21:877–86. doi: 10.1002/ejhf.1338
13. Heianza Y, Ma W, DiDonato JA, Sun Q, Rimm EB, Hu FB, et al. Long-term changes in gut microbial metabolite trimethylamine N-oxide and coronary heart disease risk. J Am Coll Cardiol. (2020) 75:763–72. doi: 10.1016/j.jacc.2019.11.060
14. Zhou X, Jin M, Liu L, Yu Z, Lu X, Zhang H. Trimethylamine N-oxide and cardiovascular outcomes in patients with chronic heart failure after myocardial infarction. ESC Heart Fail. (2020) 7:189–94. doi: 10.1002/ehf2.12552
15. Winther SA, Ollgaard JC, Tofte N, Tarnow L, Wang Z, Ahluwalia TS, et al. Utility of plasma concentration of trimethylamine N-oxide in predicting cardiovascular and renal complications in individuals with type 1 diabetes. Diabetes Care. (2019) 42:1512–20. doi: 10.2337/dc19-0048
16. Cardona A, O'Brien A, Bernier MC, Somogyi A, Wysocki VH, Smart S, et al. Trimethylamine N-oxide and incident atherosclerotic events in high-risk individuals with diabetes: an ACCORD trial post hoc analysis. BMJ Open Diabetes Res Care. (2019) 7:e000718. doi: 10.1136/bmjdrc-2019-000718
17. Gencer B, Li XS, Gurmu Y, Bonaca MP, Morrow DA, Cohen M, et al. Gut microbiota-dependent trimethylamine N-oxide and cardiovascular outcomes in patients with prior myocardial infarction: a nested case control study from the PEGASUS-TIMI 54 trial. J Am Heart Assoc. (2020) 9:e015331. doi: 10.1161/JAHA.119.015331
18. Organ CL, Otsuka H, Bhushan S, Wang Z, Bradley J, Trivedi R, et al. Choline diet and its gut microbe–derived metabolite, trimethylamine N-oxide, exacerbate pressure overload–induced heart failure. Circ Heart Fail. (2015) 9:e002314. doi: 10.1161/CIRCHEARTFAILURE.115.002314
19. Organ CL, Li Z, Thomas E, Sharp I, Polhemus DJ, Gupta N, et al. Nonlethal inhibition of gut microbial trimethylamine N-oxide production improves cardiac function and remodeling in a murine model of heart failure. J Am Heart Assoc. (2020) 9:e016223. doi: 10.1161/JAHA.119.016223
20. Huc T, Drapala A, Gawrys M, Konop M, Bielinska K, Zaorska E, et al. Chronic, low-dose TMAO treatment reduces diastolic dysfunction and heart fibrosis in hypertensive rats. Am J Physiol Heart Circ Physiol. (2018) 315:H1805–20. doi: 10.1152/ajpheart.00536.2018
21. Collins HL, Drazul-Schrader D, Sulpizio AC, Koster PD, Williamson Y, Adelman SJ, et al. L-Carnitine intake and high trimethylamine N-oxide plasma levels correlate with low aortic lesions in ApoE(-/-) transgenic mice expressing CETP. Atherosclerosis. (2016) 244:29–37. doi: 10.1016/j.atherosclerosis.2015.10.108
22. Seldin MM, Meng Y, Qi H, Zhu W, Wang Z, Hazen SL, et al. Trimethylamine N-oxide promotes vascular inflammation through signaling of mitogen-activated protein kinase and nuclear factor-kappaB. J Am Heart Assoc. (2016) 5:e002767. doi: 10.1161/JAHA.115.002767
23. Sun X, Jiao X, Ma Y, Liu Y, Zhang L, He Y, et al. Trimethylamine N-oxide induces inflammation and endothelial dysfunction in human umbilical vein endothelial cells via activating ROS-TXNIP-NLRP3 inflammasome. Biochem Biophys Res Commun. (2016) 481:63–70. doi: 10.1016/j.bbrc.2016.11.017
24. Chen M, Zhu X, Ran L, Lang H, Yi L, Mi M. Trimethylamine-N-oxide induces vascular inflammation by activating the NLRP3 inflammasome through the SIRT3-SOD2-mtROS signaling pathway. J Am Heart Assoc. (2017) 6:e006347. doi: 10.1161/JAHA.117.006347
25. Savi M, Bocchi L, Bresciani L, Falco A, Quaini F, Mena P, et al. Trimethylamine-N-oxide (TMAO)-induced impairment of cardiomyocyte function and the protective role of urolithin B-glucuronide. Molecules. (2018) 23:549. doi: 10.3390/molecules23030549
26. Makrecka-Kuka M, Volska K, Antone U, Vilskersts R, Grinberga S, Bandere D, et al. Trimethylamine N-oxide impairs pyruvate and fatty acid oxidation in cardiac mitochondria. Toxicol Lett. (2017) 267:32–8. doi: 10.1016/j.toxlet.2016.12.017
27. Bulluck H, Dharmakumar R, Arai AE, Berry C, Hausenloy DJ. Cardiovascular magnetic resonance in acute ST-segment-elevation myocardial infarction: recent advances, controversies, and future directions. Circulation. (2018) 137:1949–64. doi: 10.1161/CIRCULATIONAHA.117.030693
28. Bellenger NG, Burgess MI, Ray SG, Lahiri A, Coats AJ, Cleland JG, et al. Comparison of left ventricular ejection fraction and volumes in heart failure by echocardiography, radionuclide ventriculography and cardiovascular magnetic resonance; are they interchangeable? Eur Heart J. (2000) 21:1387–96. doi: 10.1053/euhj.2000.2011
29. Mewton N, Liu CY, Croisille P, Bluemke D, Lima JAC. Assessment of myocardial fibrosis with cardiovascular magnetic resonance. J Am Coll Cardiol. (2011) 57:891–903. doi: 10.1016/j.jacc.2010.11.013
30. Hamirani YS, Wong A, Kramer CM, Salerno M. Effect of microvascular obstruction and intramyocardial hemorrhage by CMR on LV remodeling and outcomes after myocardial infarction: a systematic review and meta-analysis. J Am Coll Cardiol Img. (2014) 7:940–52. doi: 10.1016/j.jcmg.2014.06.012
31. Schuster A, Hor KN, Kowallick JT, Beerbaum P, Kutty S. Cardiovascular magnetic resonance myocardial feature tracking: concepts and clinical applications. Circ Cardiovasc Imaging. (2016) 9:e004077. doi: 10.1161/CIRCIMAGING.115.004077
32. Eitel I, Stiermaier T, Lange T, Rommel K-P, Koschalka A, Kowallick JT, et al. Cardiac magnetic resonance myocardial feature tracking for optimized prediction of cardiovascular events following myocardial infarction. J Am Coll Cardiol Imaging. (2018) 11:1433–44. doi: 10.1016/j.jcmg.2017.11.034
33. Sheng Z, Tan Y, Liu C, Zhou P, Li J, Zhou J, et al. Relation of circulating trimethylamine N-oxide with coronary atherosclerotic burden in patients with ST-segment elevation myocardial infarction. Am J Cardiol. (2018) 123:894–8. doi: 10.1016/j.amjcard.2018.12.018
34. Tan Y, Sheng Z, Zhou P, Liu C, Zhao H, Song L, et al. Plasma trimethylamine N-oxide as a novel biomarker for plaque rupture in patients with ST-segment-elevation myocardial infarction. Circ Cardiovasc Interv. (2019) 12:e007281. doi: 10.1161/CIRCINTERVENTIONS.118.007281
35. Zhou J, Sheng Z, Liu C, Zhou P, Li J, Chen R, et al. Association between admission hyperglycemia and culprit lesion characteristics in nondiabetic patients with acute myocardial infarction: an intravascular optical coherence tomography study. J Diabetes Res. (2020) 2020:1763567. doi: 10.1155/2020/1763567
36. Ibanez B, James S, Agewall S, Antunes MJ, Bucciarelli-Ducci C, Bueno H, et al. 2017 ESC Guidelines for the management of acute myocardial infarction in patients presenting with ST-segment elevation: the task force for the management of acute myocardial infarction in patients presenting with ST-segment elevation of the European Society of Cardiology (ESC). Eur Heart J. (2018) 39:119–77. doi: 10.1093/eurheartj/ehx393
37. Wang Z, Levison BS, Hazen JE, Donahue L, Li XM, Hazen SL. Measurement of trimethylamine-N-oxide by stable isotope dilution liquid chromatography tandem mass spectrometry. Anal Biochem. (2014) 455:35–40. doi: 10.1016/j.ab.2014.03.016
38. Bjornestad EO, Olset H, Dhar I, Loland K, Pedersen EKR, Svingen GFT, et al. Circulating trimethyllysine and risk of acute myocardial infarction in patients with suspected stable coronary heart disease. J Intern Med. (2020) 288:446–56. doi: 10.1111/joim.13067
39. Yazaki Y, Salzano A, Nelson CP, Voors AA, Stefan DA, Cleland JG, et al. Geographical location affects the levels and association of trimethylamine N-oxide with heart failure mortality in BIOSTAT-CHF: a post-hoc analysis. Eur J Heart Fail. (2019) 21:1291–4. doi: 10.1002/ejhf.1550
40. Shafi T, Powe NR, Meyer TW, Hwang S, Hai X, Melamed ML, et al. Trimethylamine N-oxide and cardiovascular events in hemodialysis patients. J Am Soc Nephrol. (2017) 28:321–31. doi: 10.1681/ASN.2016030374
41. Yazaki Y, Aizawa K, Israr MZ, Negishi K, Salzano A, Saitoh Y, et al. Ethnic differences in association of outcomes with trimethylamine N-oxide in acute heart failure patients. ESC Heart Fail. (2020) 7:2373–8. doi: 10.1002/ehf2.12777
42. Luedde M, Winkler T, Heinsen FA, Ruhlemann MC, Spehlmann ME, Bajrovic A, et al. Heart failure is associated with depletion of core intestinal microbiota. ESC Heart Fail. (2017) 4:282–90. doi: 10.1002/ehf2.12155
43. Hayashi T, Yamashita T, Watanabe H, Kami K, Yoshida N, Tabata T, et al. Gut microbiome and plasma microbiome-related metabolites in patients with decompensated and compensated heart failure. Circ J. (2018) 83:182–92. doi: 10.1253/circj.CJ-18-0468
44. Liu X, Xie Z, Sun M, Wang X, Li J, Cui J, et al. Plasma trimethylamine N-oxide is associated with vulnerable plaque characteristics in CAD patients as assessed by optical coherence tomography. Int J Cardiol. (2018) 265:18–23. doi: 10.1016/j.ijcard.2018.04.126
45. Huang L, Wang Z, Wang H, Zhao L, Jiang H, Zhang B, et al. Nutrition transition and related health challenges over decades in China. Eur J Clin Nutr. (2020). doi: 10.1038/s41430-020-0674-8. [Epub ahead of print].
46. Salzano A, Israr MZ, Yazaki Y, Heaney LM, Kanagala P, Singh A, et al. Combined use of trimethylamine N-oxide with BNP for risk stratification in heart failure with preserved ejection fraction: findings from the DIAMONDHFpEF study. Eur J Prev Cardiol. (2020) 27:2159–62. doi: 10.1177/2047487319870355
47. Jia J, Dou P, Gao M, Kong X, Li C, Liu Z, et al. Assessment of causal direction between gut microbiota-dependent metabolites and cardiometabolic health: a bidirectional mendelian randomization analysis. Diabetes. (2019) 68:1747–55. doi: 10.2337/db19-0153
48. Koeth RA, Wang Z, Levison BS, Buffa JA, Org E, Sheehy BT, et al. Intestinal microbiota metabolism of L-carnitine, a nutrient in red meat, promotes atherosclerosis. Nat Med. (2013) 19:576–85. doi: 10.1038/nm.3145
49. Hu S, Zhu Y, Zhang Y, Dai J, Li L, Dauerman H, et al. Management and outcome of patients with acute coronary syndrome caused by plaque rupture versus plaque erosion: an intravascular optical coherence tomography study. J Am Heart Assoc. (2017) 6:e004730. doi: 10.1161/JAHA.116.004730
50. Soeda T, Higuma T, Abe N, Yamada M, Yokoyama H, Shibutani S, et al. Morphological predictors for no reflow phenomenon after primary percutaneous coronary intervention in patients with ST-segment elevation myocardial infarction caused by plaque rupture. Eur Heart J Cardiovasc Imaging. (2017) 18:103–10. doi: 10.1093/ehjci/jev341
51. Schwaiger JP, Reinstadler SJ, Tiller C, Holzknecht M, Reindl M, Mayr A, et al. Baseline LV ejection fraction by cardiac magnetic resonance and 2D echocardiography after ST-elevation myocardial infarction - influence of infarct location and prognostic impact. Eur Radiol. (2020) 30:663–71. doi: 10.1007/s00330-019-06316-3
Keywords: trimethylamine N-oxide, ST-segment elevation myocardial infarction, left ventricular systolic dysfunction, gut metabolite, cardiovascular magnetic resonance
Citation: Zhou J, Yu S, Tan Y, Zhou P, Liu C, Sheng Z, Li J, Chen R, Zhao S and Yan H (2020) Trimethylamine N-Oxide Was Not Associated With 30-Day Left Ventricular Systolic Dysfunction in Patients With a First Anterior ST-Segment Elevation Myocardial Infarction After Primary Revascularization: A Sub-analysis From an Optical Coherence Tomography Registry. Front. Cardiovasc. Med. 7:613684. doi: 10.3389/fcvm.2020.613684
Received: 03 October 2020; Accepted: 30 November 2020;
Published: 23 December 2020.
Edited by:
Dennis W. T. Nilsen, Stavanger University Hospital, NorwayReviewed by:
Hyung Joon Joo, Korea University Anam Hospital, South KoreaNanhu Quan, Jilin University, China
Gard Frodahl Tveitevaag Svingen, Haukeland University Hospital, Norway
Copyright © 2020 Zhou, Yu, Tan, Zhou, Liu, Sheng, Li, Chen, Zhao and Yan. This is an open-access article distributed under the terms of the Creative Commons Attribution License (CC BY). The use, distribution or reproduction in other forums is permitted, provided the original author(s) and the copyright owner(s) are credited and that the original publication in this journal is cited, in accordance with accepted academic practice. No use, distribution or reproduction is permitted which does not comply with these terms.
*Correspondence: Shihua Zhao, Y2pyemhhb3NoaWh1YTIwMDlAMTYzLmNvbQ==; Hongbing Yan, aGJ5YW5mdXdhaTIwMThAMTYzLmNvbQ==
†These authors have contributed equally to this work