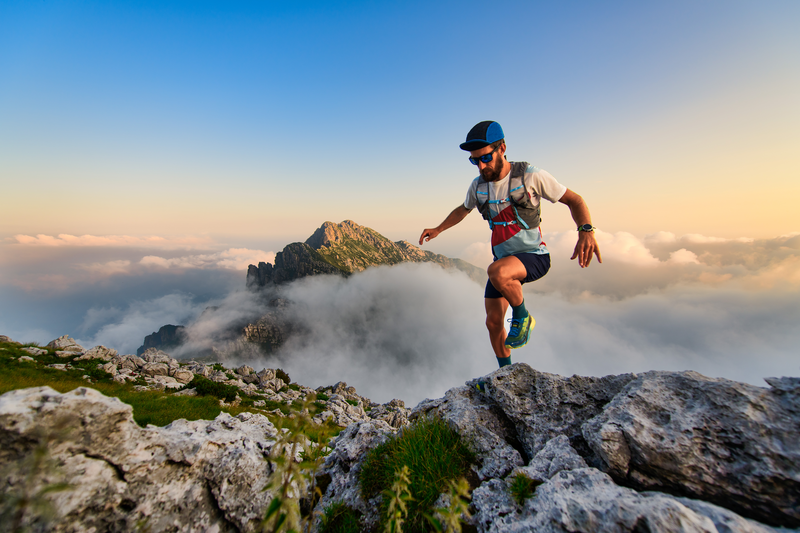
94% of researchers rate our articles as excellent or good
Learn more about the work of our research integrity team to safeguard the quality of each article we publish.
Find out more
ORIGINAL RESEARCH article
Front. Cardiovasc. Med. , 24 June 2020
Sec. Atherosclerosis and Vascular Medicine
Volume 7 - 2020 | https://doi.org/10.3389/fcvm.2020.00101
Upon activation, platelets release a host of soluble and vesicular signals, collectively termed the “platelet releasate” (PR). The contents of this PR play a significant role in haemostasis, inflammation, and pathologic sequelae. Despite this, proteomic studies investigating the PR in coronary artery disease have not been performed. Here, we undertook a comparative label-free quantitative (LFQ) proteomic profiling of the 1 U/ml thrombin-induced PR from 13 acute coronary syndrome vs. 14 stable angina pectoris patients using a tandem mass spectrometry approach. Data are available via ProteomeXchange with identifier PXD009356. 318 PR proteins were identified across both cohorts with 9 proteins found to be differentially released, including tetranectin (CLEC3B), protein disulfide-isomerase-A3 (PDIA3), coagulation factor V (F5), and fibronectin (FN1). Strikingly, these 9 differential proteins were all associated with the gene ontology cellular component term “extracellular vesicle” and reduced levels of EVs were detected in the corresponding plasma of ST-segment elevation myocardial infarction (STEMI) patients. Network analysis revealed 3 proteins either reduced (F5; FN1) or absent (CLEC3B) in the PR of STEMI patients that are strongly connected to both the clotting cascade and major druggable targets on platelets. This moderated proteomic signature may prove useful for non-invasive risk assessment of the progression of coronary artery disease. These data further contribute to the growing evidence-base of using the platelet releasate as a predictor of pathological state and disease severity.
Circulatory platelets are primary responders at the site of vascular or tissue injury and are active accomplices in vascular inflammation, atherothrombotic progression and thromboischemic diseases (1–3). With an enriched source of effector proteins stored in their granule repertoire (4, 5), they can contribute significantly to circulating levels of pro/anti-inflammatory mediators by releasing their powerful cocktail of soluble, cleaved and vesicular factors, termed the “platelet releasate” (PR), at the site of injury (4–8). This stockpile of platelet cargo, with both pathological and regenerative potential that can direct the course of thromboinflammation (9), provides a directly accessible discrete set of proteins for non-invasive biomarker analysis, possibly to predict cardiovascular outcome among patient groups in near future.
Acute coronary syndromes (ACS) can be categorized as unstable angina, non-ST segment elevation (NSTE) myocardial infarction, and ST-segment elevation myocardial infarction (STEMI). STEMI usually results from prolonged thrombotic occlusion of the coronary artery as thrombus formation ensues following rupture of an atheromatous plaque (10). Platelets circulate in a hyper-reactive state in the circulation of ACS patients. In fact, the number of platelet-derived microparticles (PMPs) is enhanced following acute myocardial infarction and associated with the extent of myocardial damage (11). Furthermore, circulatory platelets release chemokines such as CXCL12, CXCL16, levels of which are elevated in the plasma of ACS patients (12). Thus, following an ischemic episode anti-platelet therapies are recommended to keep subsequent hyper-coagulatory and thrombotic activities in check, and to prevent future ischemic events in these patients.
Despite previously recognized importance of platelets and platelet derived factors in CAD (13–15), proteomic studies investigating the PR have not been performed. We recently established that the PR is highly reproducible across 32 healthy adults, with low variation in secretion levels for individual PR proteins (16). As there is accumulating evidence that circulating platelets may sense or be “educated” by their environment (17, 18); we hypothesized that the PR may alter between stable angina pectoris (SAP) and STEMI and that such alterations may be relevant to disease progression. To address this, we performed comparative label-free quantitative (LFQ) proteomic profiling of the platelet releasate (PR), from STEMI vs. SAP patients using a tandem mass spectrometry (MS) approach.
Blood samples were collected during PCI from the from the TUEPIC clinical registry of coronary artery disease (CAD) patients. All subjects gave written informed consent in accordance with the Declaration of Helsinki. Patients were admitted and treated at the department of Cardiology and Cardiovascular Diseases at the University Clinic of Tübingen, Germany. We included consecutive patients with symptomatic CAD (stable angina pectoris-SAP n = 14, ST-elevation myocardial infarction -STEMI n = 13). The study was approved by the institutional ethics committee (270/2011BO1) and complies with the declaration of Helsinki and the good clinical practice guidelines (19). Baseline characteristics of patients are tabulated in Table 1. All STEMI patients were directly transferred to the catheterization laboratory for direct percutaneous coronary intervention (PCI). Patients with STEMI were treated with 500 mg aspirin and 5,000 U of non-fractionated heparin at time of diagnosis of STEMI. For platelet analysis whole blood was taken at the beginning of the catherization procedure from the inserted 6 french arterial sheet before additional antithrombotic therapy was given (ACT-adjusted heparin and ticagrelor). Further, in patients with SAP whole blood was collected from the arterial access site before planned PCI after administration of 500 mg aspirin and 5,000 U of non-fractionated heparin. Thereafter, dual antiplatelet therapy was initiated with a loading dose of clopidogrel (20).
Table 1. Patient demographics, cardiovascular risk factors, medication on admission and lipid profile parameters in those with STEMI and SAP.
Platelet isolation was performed as we described previously using a series of well-documented serial centrifugations (7, 16, 21–23). In brief, platelet rich plasma was isolated from whole blood by centrifugation (200 × g for 10 min at room temperature [RT]). Platelet rich plasma was then supplemented with 1 μM prostaglandin E1 and remaining erythrocyte contamination was minimized by further centrifugation (150 × g for 7 min at RT). Platelets were then isolated from plasma by centrifugation (600 × g for 10 min at RT) and subsequently washed using a modified Tyrodes buffer followed again by centrifugation (600 × g for 10 min at RT). Platelets were resuspended at 1 x 109 platelets/ml, left to rest for 30 min at RT and then activated with 1 U/ml thrombin (Roche, Basel, Switzerland) under constant stirring for 5 min at 37°C using a Chronolog-700 platelet aggregometer (Chronolog Cor, Manchester, UK). A minimum measured aggregation threshold of 80% was set for patient inclusion in proteomic analysis. Platelet activation was terminated by immediately placing the tube on ice and with the addition of 1 μM prostaglandin E1. Intact platelets and the platelet clot were carefully removed by centrifuging sequentially x3 at 1000 × g for 10 min at 4°C (in the presence of 2% protease and 2% phosphatase inhibitors; Roche) and harvesting the supernatant each time. The final spin yielded the activated PR as before (7, 16, 21, 22), which was stored at −80 °C until further use.
PR samples were prepared for MS as we described previously (16). In brief, samples were individually solubilised in RIPA buffer and proteins precipitated overnight with 95 % acetone (4:1 acetone: sample volume) at −20°C. Dried protein pellets were resuspended in 8 M urea/24 mM Tris-HCL, pH 8.2, at 37°C for 1 h. Disulphide bonds were reduced with 5 mM DTT and protected with 15 mM iodoacetamide. PR samples were digested with Lys-C (1:100; Promega, Madison, WI) followed by digestion with trypsin (1:100; Promega). Peptides were purified using ZipTipC18 pipette tips (Millipore, Billerica, MA, USA) and resuspended in 1 % formic acid. Each biological sample was analyzed using a Thermo-Scientific Q-Exactive mass spectrometer connected to a Dionex Ultimate 3,000 (RSLCnano) liquid chromatography (LC) system as described (23). In brief, each biological sample was individually loaded onto a fused silica emitter (75 μm ID), pulled using a laser puller (Sutter Instruments P2000, Novato, CA, USA), packed with Reprocil Pur (Dr. Maisch, Ammerbuch-Entringen, Germany) C18 (1.9 μm; 12 cm in length) reverse-phase media and separated by an increasing acetonitrile gradient over 47 min (flow rate = 250 nL/min) direct into a Q-Exactive MS. The MS was operated in positive ion mode with a capillary temperature of 320°C, and with a potential of 2,300 V applied to the frit. All data was acquired while operating in automatic data-dependent switching mode. A high resolution (70,000) MS scan (300–1,600 m/z) was performed using the Q-Exactive to select the 12 most intense ions prior to MS/MS analysis using high-energy collision dissociation (HCD).
Raw MS files were analyzed by MaxQuant (MQ) version 1.5.0.30. MS/MS spectra were searched by the Andromeda search engine against a human FASTA (August 2016) obtained from UniProt. Raw MS data has been deposited to the ProteomeXchange Consortium via the PRIDE partner repository with the dataset identifier PXD009356. MQ analysis included an initial search with a precursor mass tolerance of 20 ppm the results of which were used for mass recalibration. In the main Andromeda search precursor mass and fragment mass had an initial mass tolerance of 6 and 20 ppm, respectively. The search included fixed modification of carbamidomethyl cysteine. Minimal peptide length was set to 7 amino acids and a maximum of 2 miscleavages was allowed. The false discovery rate (FDR) was set to 0.01 for peptide/protein identification. For quantitative comparison between samples we used label-free quantification (LFQ) with a minimum of two ratio counts to determine the normalized protein intensity. LFQ intensities were assigned to identified proteins by comparing the area under the curve of the signal intensity for any given peptide as we performed previously (23).
Data was processed in the Perseus open framework (http://www.perseus-framework.org). Protein IDs were filtered to eliminate identifications from the reverse database, proteins only identified by site, and common contaminants. LFQ intensity values were Log2 transformed. A protein was included if it was identified in at least 50% of samples in at least one (SAP or STEMI) patient cohort. Panther (v13; released 2017-11-12; http://pantherdb.org/) was used to assign gene ontology (GO) with Bonferroni correction against the 2017_04 release of ReferenceProteome dataset. STRING (http://string-db.org/; v10.5) was used to allocate high confidence (minimum required interaction score of 0.700) protein interactions utilizing experimental and database sources.
A methodology flow chart can be found in Figure 1.
Here, we used LFQ-proteomic profiling to compare the PR proteome of SAP and STEMI (Figure 1). In brief, 1 U/ml thrombin-activated PR was isolated from 13 STEMI and from 14 SAP patients and analyzed by LC-MS/MS (Figure 1). Utilizing statistical algorithms within the Perseus open framework, we examined the biological reproducibility of the LFQ-proteomic analysis between patients in each group using Pearson correlation coefficient analysis (r) (Figure 2A). Strong inter-patient reproducibility was observed across our PR samples, averaging at r = 0.938 ± 0.023 for SAP patients (Figure 2Ai; Supplementary Table 1) and r = 0.878 ± 0.088 for STEMI patients (Figure 2Aii; Supplementary Table 2).
Figure 2. The PR is different between STEMI and SAP. (A) Strong inter-patient correlations (r > 0.88) were found in the 13 STEMI and 14 SAP platelet releasate (PR) samples. (B) 318 PR proteins were identified (in at least 50% of samples in at least one patient cohort), including one PR protein unique to STEMI (red) and two PR proteins unique to SAP (blue). (C) Volcano plot of STEMI vs. SAP PR proteomes (x-axis representing the difference between the mean log2 LFQ values of STEMI to SAP PR; y-axis, the negative log transformed p-value) representing the proteins significantly altered in each condition. The black hyperbolic curved lines show the threshold for statistical significance, where a false discovery rate of 0.05 and an S0 (minimal difference between the mean log2 LFQ values) of 0.1 was used. Of the 14 proteins used for statistical comparison (difference between the mean log2 LFQ values >1/1), our analysis revealed that the secretion levels of 4 proteins were significantly increased in STEMI PR (red dots) whereas 2 proteins were significantly decreased in STEMI PR (blue dots). The secretion levels of the remaining 8 proteins were unchanged (gray dots). (D) The STRING database (http://string-db.org/; version 10.5) was used to allocate protein interactions utilizing experimental and database sources. An interaction network of high confidence (>/= 0.700 denoted by line thickness) consisting of three proteins (F5; FN1; CLEC3B) was revealed.
318 PR proteins were identified (in at least 50% of samples in at least one patient cohort) including 2 proteins tetranectin (CLEC3B) and heat shock protein 90-alpha (HSP90AA1) uniquely released from SAP platelets and 1 protein fumarylacetoacetase (FAH) found released only from STEMI platelets (Figure 2B). Supplementary Table 3 displays the log2 transformed LFQ intensity values for the 318 proteins in each sample. Using Perseus software, we determined if there were differences in the secretion levels of the 315 shared proteins. The secretion levels of 301/315 (96%) proteins were deemed unchanged (difference between the mean log2 LFQ values −1/1) and thus these proteins were excluded from further statistical analysis (24). The alteration in the secretion levels of the remaining 14 proteins (difference between the mean log2 LFQ values >1/1) were evaluated for statistical significance (p < 0.05) utilizing Student's t-test analysis with an FDR value of 0.05 and an S0 correction factor of 0.1, denoted by the black hyperbolic lines (Figure 2C) (24). We found that the secretion levels of 4 proteins (red dots; Figure 2C) were significantly increased in STEMI patients in comparison to SAP patients namely glucose-6-phosphate isomerase (GPI), protein disulfide-isomerase A3 (PDIA3), serum amyloid A-1 protein (SAA1) and superoxide dismutase (SOD1). Moreover, the secretion levels of a further 2 proteins (blue dots; Figure 2C) were significantly reduced in STEMI patients when compared to SAP PR, specifically coagulation factor V (F5) and fibronectin (FN1). The secretion levels of the remaining 8 proteins were not significantly changed (gray dots).
GO biological pathway analysis (Supplementary Table 4) was performed on the 9 differential proteins (For a list see Table 2) and revealed a significant enrichment of functional terms including “platelet degranulation” (GO:0002576) (4 proteins: SOD1; FN1; CLEC3B; F5) and “regulated exocytosis” (GO:0045055) (6 proteins: HSP90AA1; SOD1; GPI; FN1; CLEC3B; F5). Strikingly, all 9 differential proteins (HSP90AA1; SAA1; SOD1; GPI; PDIA3; FN1; CLEC3B; F5; FAH) were associated with “vesicle” and “extracellular vesicle” when GO cellular component was analyzed (Supplementary Table 5). Using nanoparticle tracking analysis (NTA), we detected particles in the exosomal-vesicle size range (30–120 nm in size) in the available PR and corresponding plasma (25) from our two patient groups (Supplementary Methods; Supplementary Figures 1A,B). While it is important to note that NTA cannot distinguish between small EVs and lipoproteins, total particle counts (0–200 nm) did reveal a significant decrease in circulating smaller particles (<200 nm) in the corresponding plasma of STEMI vs. SAP patients (p = 0.048) (Supplementary Figure 1B).
These 9 differentially secreted proteins were also subjected to a network analysis using the STRING database (v10.5) (Figure 2D; Supplementary Figure 1C). This revealed an interaction network of high confidence of three α-granular proteins coagulation factor V (F5), fibronectin (FN1) (26) and tetranectin (CLEC3B) (Figure 2D) (27). Interestingly, the secretion levels of the three proteins in this network were either significantly reduced (F5; FN1) or absent (CLEC3B) in the PR of our STEMI patients (Table 2). Secondary protein interactions of these differential proteins highlighted the close connection with the clotting cascade (F2; F10) as well as the major platelet integrins β3 (ITGB3) and αV (ITGAV) (Supplementary Figure 1C).
A wealth of information has been gleaned on the PR from platelet proteomic studies, especially over the last few years with dramatic improvements in MS-based proteomics technology (5, 7, 16, 28, 29). Here, we utilized recent advances in label-free quantitation (30) together with more sensitive MS instrumentation to demonstrate the exclusive presence and differential abundance of specific proteomic signatures in the PR from SAP and STEMI patients. Three hundred and eighteen PR proteins were identified, with 9 proteins differentially released. Strikingly, all 9 differential proteins were associated with the GO cellular component term “extracellular vesicle” and we detected reduced levels of smaller particles, which include small EVs (<200 nm), in plasma of STEMI patients. We did not measure larger EVs in our samples, which was an oversight, however these smaller EVs (exosomes) can provide a delivery vehicle for intercellular communication. Whether these smaller EVs mediate protective or pathogenic effects in cardiovascular disease has however yet to be determined (31).
Five proteins were observed to be either exclusively found [fumarylacetoacetase (FAH)] or have increased expression in [glucose-6-phosphate isomerase (GPI), protein disulfide-isomerase A3 (PDIA3), serum amyloid A-1 protein (SAA1) and superoxide dismutase (SOD1)] the PR of STEMI patients on admission in comparison to SAP. FAH is an enzyme involved in the tyrosine catabolism pathway whilst GPI functions in glycolysis. While the pathophysiological significance of increased circulating FAH is unknown, GPI can function extracellularly as a tumor-secreted cytokine or as an angiogenic factor that stimulates endothelial cell motility (32). Although PDIA3 is involved in protein folding in the endoplasmic reticulum, platelets have been shown to release PDIA3 in response to vascular injury, where it can drive platelet activation and subsequent thrombus formation. In fact, PDIA3 is incorporated into the developing thrombus and inhibition can reduce arterial thrombus formation (33, 34). SAA1 is a major acute phase protein that is highly expressed in response to inflammation and tissue injury. Our observation of increased SAA1 in STEMI is consistent with previous reports (35) where high levels of SAA1 can be predictive of coronary artery disease and cardiovascular outcome (36). Therefore, enhanced secretion of GPI, PDIA3 and SAA1 in the PR from STEMI patients with acute thromboischemic events is in accordance with their assigned functions. However, while higher concentrations of circulating SOD1 (one of two isozymes responsible for destroying free superoxide radicals) have been found in STEMI when compared to healthy controls, there is conflicting evidence as to whether levels may vary significantly in comparison to SAP (37).
A further 4 proteins were found to be either absent from [tetranectin (CLEC3B); heat shock protein 90-alpha (HSP90AA1)], or statistically reduced in [coagulation factor V (F5) and fibronectin (FN1)], the PR of STEMI patients when compared to SAP. Tetranectin (CLEC3B), a member of the C-type lectin domain family with importance in platelet biology (38), has already emerged as a marker of CAD; where levels were reduced in SAP patients in comparison to healthy controls (35, 39). In fact, an incremental reduction in serum levels and concomitant expression in atherosclerotic lesions correlated with disease progression (39). Moreover, CLEC3B induces plasminogen activation (40), which is an important fibrinolytic pathway that may be deficient in the ACS cohort. HSP90AA1 is a molecular chaperone, known to be involved in thrombin-induced platelet activation (41). Extracellularly, HSP90AA1 has been shown to mediate immune-regulatory and tissue remodeling effects (42) and increased HSP90 levels are associated with atherosclerotic plaque instability (43). As CAD severity has also been inversely associated with circulating HSP levels (44), the absence of CLEC3B and HSP90AA1 from the PR of STEMI patients may reflect circulating activated platelets and increased plaque uptake; however, the pathophysiological significance of these particular PR proteins in the peripheral circulation of CAD patients remains to be explored.
The levels of F5 and FN1 were also found decreased in the PR of STEMI patients. Platelet-derived Factor V (F5) was found to be a critical mediator of arterial thrombosis in mice (45) and CAD patients show significantly higher levels of plasma FN1 as compared to healthy subjects, with platelet FN1 levels correlating with severity of disease (46, 47). Decreased F5 and FN1 levels in PR of STEMI patients as compared to SAP patients may reflect possible consumption of these proteins during acute MI. Together with CLEC3B, F5, and FN1 emerged as a modified network in the PR of STEMI patients. This triad is strongly connected to both the clotting cascade (association with F2; F10) and major integrin receptors on platelets (association with ITGB3 and ITGAV) that have emerged in previous proteomics studies of platelets in ACS (48), highlighting these proteins as relevant players in the acute event.
In conclusion, our comprehensive analysis of the PR proteome in STEMI provides a moderated protein network which may be useful for non-invasive risk assessment of the onset and progression of coronary artery disease.
Raw MS data has been deposited to the ProteomeXchange Consortium via the PRIDE partner repository with the dataset identifier PXD009356.
The studies involving human participants were reviewed and approved by Ethik-Kommission an der Medizinischen Fakultät der Eberhard-Karls-Universität und am Universitätsklinikum Tübingen. The patients/participants provided their written informed consent to participate in this study.
PBM, MP, PS, MZ, PM, MC, KW, FN, and MG designed research. MP, PS, MZ, PM, KW, and MH performed the experiments. PBM, MP, PS, MZ, PM, MC, DR, FN, and MG analyzed the data. PBM, PS, MC, DR, SC, FN, and MG wrote the paper.
This research was funded by PI Award 10/IN.1/B3012 grant from Science Foundation Ireland (to PBM). PS was supported by Irish Research Council award GOIPG/2014/575. This project was supported by the Deutsche Forschungsgemeinschaft (Klinische Forschungsgruppe-KFO-274: Platelets-Molecular Mechanisms and Translational Implications) and by DFG CRC/TR 240 Platelets—Molecular, cellular, and systemic functions in health and disease.
The authors declare that the research was conducted in the absence of any commercial or financial relationships that could be construed as a potential conflict of interest.
The Supplementary Material for this article can be found online at: https://www.frontiersin.org/articles/10.3389/fcvm.2020.00101/full#supplementary-material
ACS, Acute coronary syndrome; SAP, stable angina pectoris; STEMI, ST-segment elevation myocardial infarction; PR, platelet releasate; LFQ, label-free quantification; LC-MS/MS, liquid chromatography tandem mass spectrometry; MQ, MaxQuant; CV, coefficient of variation; GO, Gene Ontology.
2. Gawaz M, Langer H, May AE. Platelets in inflammation and atherogenesis. J Clin Invest. (2005) 115:3378–84. doi: 10.1172/JCI27196
3. Koenen RR, Weber C. Platelet-derived chemokines in vascular remodeling and atherosclerosis. Semin Thromb Hemost. (2010) 36:163–9. doi: 10.1055/s-0030-1251500
4. Heijnen H, van der Sluijs P. Platelet secretory behaviour: as diverse as the granules. Or not? J Thromb Haemost. (2015) 13:2141–51. doi: 10.1111/jth.13147
5. Pagel O, Walter E, Jurk K, Zahedi RP. Taking the stock of granule cargo: platelet releasate proteomics. Platelets. (2017) 28:119–28. doi: 10.1080/09537104.2016.1254762
6. Heijnen HF, Schiel AE, Fijnheer R, Geuze HJ, Sixma JJ. Activated platelets release two types of membrane vesicles: microvesicles by surface shedding and exosomes derived from exocytosis of multivesicular bodies and alpha-granules. Blood. (1999) 94:3791–9. doi: 10.1182/blood.V94.11.3791
7. Coppinger JA, Cagney G, Toomey S, Kislinger T, Belton O, McRedmond JP, et al. Characterization of the proteins released from activated platelets leads to localization of novel platelet proteins in human atherosclerotic lesions. Blood. (2004) 103:2096–104. doi: 10.1182/blood-2003-08-2804
8. Fong KP, Barry C, Tran AN, Traxler EA, Wannemacher KM, Tang H-Y, et al. Deciphering the human platelet sheddome. Blood. (2011) 117:e15–26. doi: 10.1182/blood-2010-05-283838
9. Ekdahl KN, Teramura Y, Asif S, Jonsson N, Magnusson PU, Nilsson B. Thromboinflammation in therapeutic medicine. Adv Exp Med Biol. (2015) 865:3–17. doi: 10.1007/978-3-319-18603-0_1
10. Grech ED, Ramsdale DR. Acute coronary syndrome: ST segment elevation myocardial infarction. BMJ. (2003) 326:1379–81. doi: 10.1136/bmj.326.7403.1379
11. Hartopo AB, Puspitawati I, Gharini PP, Setianto BY. Platelet microparticle number is associated with the extent of myocardial damage in acute myocardial infarction. Arch. Med Sci. (2016) 12:529–37. doi: 10.5114/aoms.2016.59926
12. Chatterjee M, Geisler T. Inflammatory contribution of platelets revisited: new players in the arena of inflammation. Semin Thromb Hemost. (2016) 42:205–14. doi: 10.1055/s-0035-1570081
13. Mateos-Caceres PJ, Garcia-Mendez A, Lopez Farre A, Macaya C, Nunez A, Gomez J, et al. Proteomic analysis of plasma from patients during an acute coronary syndrome. J Am Coll Cardiol. (2004) 44:1578–83. doi: 10.1016/j.jacc.2004.06.073
14. Alonso-Orgaz S, Moreno-Luna R, Lopez JA, Gil-Dones F, Padial LR, Moreu J, et al. Proteomic characterization of human coronary thrombus in patients with st-segment elevation acute myocardial infarction. J Proteomics. (2014) 109:368–81. doi: 10.1016/j.jprot.2014.07.016
15. Velez P, Ocaranza-Sanchez R, Lopez-Otero D, Grigorian-Shamagian L, Rosa I, Bravo SB, et al. 2d-dige-based proteomic analysis of intracoronary versus peripheral arterial blood platelets from acute myocardial infarction patients: upregulation of platelet activation biomarkers at the culprit site. Proteomics. Clin Appl. (2016). 10:851–8. doi: 10.1002/prca.201500120
16. Parsons MEM, Szklanna PB, Guerrero JA, Wynne K, Dervin F, O'Connell K, et al. Platelet releasate proteome profiling reveals a core set of proteins with low variance between healthy adults. Proteomics. (2018) 18:e1800219. doi: 10.1002/pmic.201800219
17. Best MG, Sol N, Kooi I, Tannous J, Westerman BA, Rustenburg F, et al. Rna-seq of tumor-educated platelets enables blood-based pan-cancer, multiclass, and molecular pathway cancer diagnostics. Cancer Cell. (2015) 28:666–76. doi: 10.1016/j.ccell.2015.09.018
18. Klement GL, Yip TT, Cassiola F, Kikuchi L, Cervi D, Podust V, et al. Platelets actively sequester angiogenesis regulators. Blood. (2009) 113:2835–42. doi: 10.1182/blood-2008-06-159541
19. Thygesen K, Alpert JS, Jaffe AS, Simoons ML, Chaitman BR, White HD, et al. Third universal definition of myocardial infarction. Eur Heart J. (2012) 33:2551–67. doi: 10.1093/eurheartj/ehs184
20. Chatterjee M, Rath D, Schlotterbeck J, Rheinlaender J, Walker-Allgaier B, Alnaggar N, et al. Regulation of oxidized platelet lipidome: implications for coronary artery disease. Eur Heart J. (2017) 38:1993–2005. doi: 10.1093/eurheartj/ehx146
21. Szklanna PB, Parsons ME, Wynne K, O'Connor H, Egan K, Allen S, et al. The platelet releasate is altered in human pregnancy. Proteomics Clin Appl. (2019) 13:e1800162. doi: 10.1002/prca.201800162
22. Coppinger JA, O'Connor R, Wynne K, Flanagan M, Sullivan M, Maguire PB, et al. Moderation of the platelet releasate response by aspirin. Blood. (2007) 109:4786–92. doi: 10.1182/blood-2006-07-038539
23. Szklanna PB, Foy M, Wynne K, Byrne D, Maguire PB. Analysis of the proteins associated with platelet detergent resistant membranes. Proteomics. (2016) 16:2345–50. doi: 10.1002/pmic.201500309
24. McCarthy DJ, Smyth GK. Testing significance relative to a fold-change threshold is a treat. Bioinformatics. (2009) 25:765–71. doi: 10.1093/bioinformatics/btp053
25. Parsons MEM, McParland D, Szklanna PB, Guang MHZ, O'Connell K, O'Connor HD, et al. A protocol for improved precision and increased confidence in nanoparticle tracking analysis concentration measurements between 50 and 120 nm in biological fluids. Front Cardiovasc Med. (2017) 4:68. doi: 10.3389/fcvm.2017.00068
26. Rendu F, Brohard-Bohn B. The platelet release reaction: granules' constituents, secretion and functions. Platelets. (2001) 12:261–73. doi: 10.1080/09537100120068170
27. Kluft C, Los P, Clemmensen I. Calcium-dependent binding of tetranectin to fibrin. Thromb Res. (1989) 55:233–8. doi: 10.1016/0049-3848(89)90440-4
28. Burkhart JM, Vaudel M, Gambaryan S, Radau S, Walter U, Martens L, et al. The first comprehensive and quantitative analysis of human platelet protein composition allows the comparative analysis of structural and functional pathways. Blood. (2012) 120:e73–82. doi: 10.1182/blood-2012-04-416594
29. Wijten P, van Holten T, Woo LL, Bleijerveld OB, Roest M, Heck AJ, et al. High precision platelet releasate definition by quantitative reversed protein profiling–brief report. Arterioscler Thromb Vasc Biol. (2013) 33:1635–8. doi: 10.1161/ATVBAHA.113.301147
30. Cox J, Hein MY, Luber CA, Paron I, Nagaraj N, Mann M. Accurate proteome-wide label-free quantification by delayed normalization and maximal peptide ratio extraction, termed maxlfq. Mol Cell Proteomics. (2014) 13:2513–26. doi: 10.1074/mcp.M113.031591
31. Anouar H, Daskalopoulou SS. Extracellular vesicles characteristics and emerging roles in atherosclerotic cardiovascular disease. Metabolism. (2018) 85:213–22. doi: 10.1016/j.metabol.2018.04.008
32. Haga A, Niinaka Y, Raz A. Phosphohexose isomerase/autocrine motility factor/neuroleukin/maturation factor is a multifunctional phosphoprotein. Biochim Biophys Acta. (2000) 1480:235–44. doi: 10.1016/S0167-4838(00)00075-3
33. Holbrook LM, Sasikumar P, Stanley RG, Simmonds AD, Bicknell AB, Gibbins JM. The platelet-surface thiol isomerase enzyme erp57 modulates platelet function. J Thromb Haemost. (2012) 10:278–88. doi: 10.1111/j.1538-7836.2011.04593.x
34. Wang L, Wu Y, Zhou J, Ahmad SS, Mutus B, Garbi N, et al. Platelet-derived erp57 mediates platelet incorporation into a growing thrombus by regulation of the alphaiibbeta3 integrin. Blood. (2013) 122:3642–50. doi: 10.1182/blood-2013-06-506691
35. Darde VM, de la Cuesta F, Dones FG, Alvarez-Llamas G, Barderas MG, Vivanco F. Analysis of the plasma proteome associated with acute coronary syndrome: does a permanent protein signature exist in the plasma of acs patients? J Proteome Res. (2010) 9:4420–32. doi: 10.1021/pr1002017
36. Johnson BD, Kip KE, Marroquin OC, Ridker PM, Kelsey SF, Shaw LJ, et al. Serum amyloid a as a predictor of coronary artery disease and cardiovascular outcome in women: the national heart, lung, and blood institute-sponsored women's ischemia syndrome evaluation (wise). Circulation. (2004) 109:726–32. doi: 10.1161/01.CIR.0000115516.54550.B1
37. Horiuchi M, Tsutsui M, Tasaki H, Morishita T, Suda O, Nakata S, et al. Upregulation of vascular extracellular superoxide dismutase in patients with acute coronary syndromes. Arterioscler Thromb Vasc Biol. (2004) 24:106–11. doi: 10.1161/01.ATV.0000104240.56460.AB
38. Suzuki-Inoue K, Osada M, Ozaki Y. Physiologic and pathophysiologic roles of interaction between c-type lectin-like receptor 2 and podoplanin: partners from in utero to adulthood. J Thromb Haemost. (2017) 15:219–29. doi: 10.1111/jth.13590
39. Chen Y, Han H, Yan X, Ding F, Su X, Wang H, et al. Tetranectin as a potential biomarker for stable coronary artery disease. Sci Rep. (2015) 5:17632. doi: 10.1038/srep17632
40. Clemmensen I, Petersen LC, Kluft C. Purification and characterization of a novel, oligomeric, plasminogen kringle 4 binding protein from human plasma: tetranectin. Eur J Biochem. (1986) 156:327–33. doi: 10.1111/j.1432-1033.1986.tb09586.x
41. Suttitanamongkol S, Polanowska-Grabowska R, Gear AR. Heat-shock protein 90 complexes in resting and thrombin-activated platelets. Biochem Biophys Res Commun. (2002) 297:129–33. doi: 10.1016/S0006-291X(02)02138-1
42. Thuringer D, Hammann A, Benikhlef N, Fourmaux E, Bouchot A, Wettstein G, et al. Transactivation of the epidermal growth factor receptor by heat shock protein 90 via toll-like receptor 4 contributes to the migration of glioblastoma cells. J Biol Chem. (2011) 286:3418–28. doi: 10.1074/jbc.M110.154823
43. Madrigal-Matute J, Lopez-Franco O, Blanco-Colio LM, Munoz-Garcia B, Ramos-Mozo P, Ortega L, et al. Heat shock protein 90 inhibitors attenuate inflammatory responses in atherosclerosis. Cardiovasc Res. (2010) 86:330–7. doi: 10.1093/cvr/cvq046
44. Zhu J, Quyyumi AA, Wu H, Csako G, Rott D, Zalles-Ganley A, et al. Increased serum levels of heat shock protein 70 are associated with low risk of coronary artery disease. Arterioscler Thromb Vasc Biol. (2003) 23:1055–9. doi: 10.1161/01.ATV.0000074899.60898.FD
45. Ren M, Li R, Chen N, Pang N, Li Y, Deng X, et al. Platelet-derived factor v is a critical mediator of arterial thrombosis. J Am Heart Assoc. (2017) 6:006345. doi: 10.1161/JAHA.117.006345
46. Ekmekci H, Isler I, Sonmez H, Gurel C, Ciftci O, Ulutin T, et al. Comparison of platelet fibronectin, adp-induced platelet aggregation and serum total nitric oxide (nox) levels in angiographically determined coronary artery disease. Thromb Res. (2006) 117:249–54. doi: 10.1016/j.thromres.2005.03.006
47. Lemanska-Perek A, Krzyzanowska-Golab D, Pupek M, Klimeczek P, Witkiewicz W, Katnik-Prastowska I. Analysis of soluble molecular fibronectin-fibrin complexes and eda-fibronectin concentration in plasma of patients with atherosclerosis. Inflammation. (2016) 39:1059–68. doi: 10.1007/s10753-016-0336-0
Keywords: platelets, platelet releasate, proteomics, mass spectrometry, acute coronary syndrome, stable coronary artery disease
Citation: Maguire PB, Parsons ME, Szklanna PB, Zdanyte M, Münzer P, Chatterjee M, Wynne K, Rath D, Comer SP, Hayden M, Ní Áinle F and Gawaz M (2020) Comparative Platelet Releasate Proteomic Profiling of Acute Coronary Syndrome versus Stable Coronary Artery Disease. Front. Cardiovasc. Med. 7:101. doi: 10.3389/fcvm.2020.00101
Received: 26 March 2020; Accepted: 12 May 2020;
Published: 24 June 2020.
Edited by:
Rory R. Koenen, Maastricht University, NetherlandsReviewed by:
Marie Lordkipanidzé, Université de Montréal, CanadaCopyright © 2020 Maguire, Parsons, Szklanna, Zdanyte, Münzer, Chatterjee, Wynne, Rath, Comer, Hayden, Ní Áinle and Gawaz. This is an open-access article distributed under the terms of the Creative Commons Attribution License (CC BY). The use, distribution or reproduction in other forums is permitted, provided the original author(s) and the copyright owner(s) are credited and that the original publication in this journal is cited, in accordance with accepted academic practice. No use, distribution or reproduction is permitted which does not comply with these terms.
*Correspondence: Patricia B. Maguire, cGF0cmljaWEubWFndWlyZUB1Y2QuaWU=
Disclaimer: All claims expressed in this article are solely those of the authors and do not necessarily represent those of their affiliated organizations, or those of the publisher, the editors and the reviewers. Any product that may be evaluated in this article or claim that may be made by its manufacturer is not guaranteed or endorsed by the publisher.
Research integrity at Frontiers
Learn more about the work of our research integrity team to safeguard the quality of each article we publish.