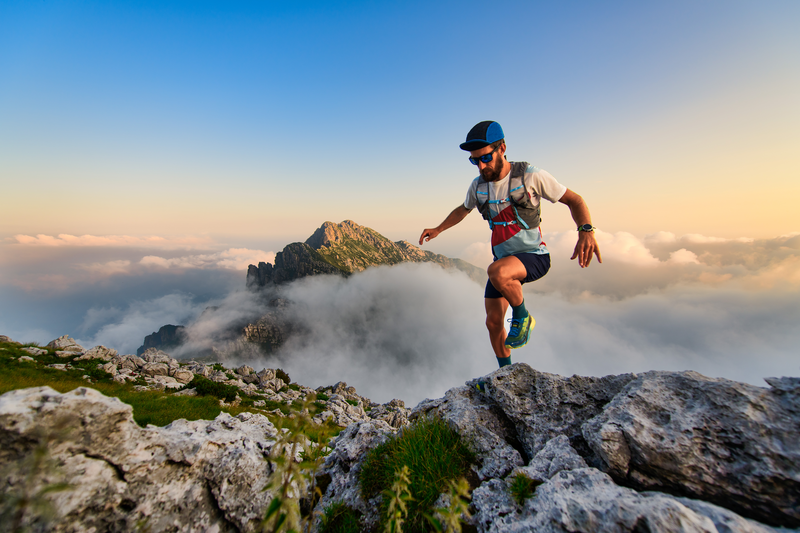
94% of researchers rate our articles as excellent or good
Learn more about the work of our research integrity team to safeguard the quality of each article we publish.
Find out more
MINI REVIEW article
Front. Cardiovasc. Med. , 23 January 2019
Sec. Atherosclerosis and Vascular Medicine
Volume 6 - 2019 | https://doi.org/10.3389/fcvm.2019.00003
This article is part of the Research Topic Cardioimmunology: Inflammation and Immunity in Cardiovascular Disease View all 22 articles
Immune checkpoint inhibitors (ICIs) have changed the treatment landscape of advanced cancers. Unfortunately, these agents can induce a wide spectrum of immune-related adverse events (irAEs) through activation of immune responses in non-target organs, including the heart. As the clinical use of ICI therapy increases rapidly, management of irAEs is becoming extremely important. The most commonly presented cardiac irAE is myocarditis. Histopathologically, T-cell (with a predominance of CD8+ cells) and macrophage infiltration in the myocardium is typically observed in ICI-associated myocarditis. Other presentations of cardiac irAEs include congestive heart failure, Takotsubo cardiomyopathy, pericardial disease, arrhythmias, and conduction disease. Although cardiac irAEs are relatively rare, they can be life-threatening. Hence, cardiologists and oncologists should be vigilant for these presentations.
Immune checkpoint inhibitors (ICIs), including monoclonal antibodies (mAbs) against cytotoxic T-lymphocyte-associated antigen 4 (CTLA-4), programmed cell death protein 1 (PD-1), and programmed cell death ligand 1 (PD-L1), are being routinely used in clinical settings and have shown unprecedented efficacy in treating multiple cancers (1–6). Unfortunately, these agents can induce a wide spectrum of immune-related adverse events (irAEs) (7–9) through activation of immune responses in non-target organs, including the heart. In recent years, several cases of cardiotoxicity have been reported in cancer patients treated with ICIs (10–17). Although its frequency is lower than that for other irAEs, cardiotoxicity can become life-threatening, making it an important consideration for cardiologists, oncologists, and immunologists.
In this review, we describe the mechanisms and summarize the reported clinical scenarios of cardiotoxicities associated with ICIs. Evidence available for diagnosis, management, and prognosis are considered.
T lymphocytes play a pivotal role as modulators and effectors of the immune system. Naïve T cells are activated after recognizing a cognate peptide presented by antigen-presenting cells (APCs) via interaction between the major histocompatibility complex (MHC) on the APCs and the T cell receptor (TCR), but further co-stimulatory signals are required for activation. CD28 is a stimulatory co-receptor expressed on T cells. Binding of CD80 (also known as B7-1) or CD86 (also known as B7-2) molecules on APCs with CD28 molecules provides an essential signal for T cell activation. However, to prevent destructive immune activation, these signals are finely regulated by immune checkpoints (e.g., CTLA-4 and PD-1) (18).
CTLA-4 is an inhibitory co-receptor expressed on activated T cells. CTLA-4 inhibits T cell functions by competing with CD28 for binding with B7 ligands, CD80, and CD86. CTLA-4 is homologous to CD28 but has much higher binding affinity and avidity for B7 ligands. In resting naïve T cells, unlike CD28, which is constitutively expressed on the cell surface, CTLA-4 is localized primarily in intracellular vesicles (19). CTLA-4 is upregulated on the cell surface in response to TCR activation and the signal is enhanced by co-stimulation through CD28 and/or interleukin-2 (20). Of note, the stronger the TCR signal, the greater the CTLA-4 translocation to the cell surface, thereby preventing harmful T cell activation (19–21).
PD-1 is another inhibitory receptor and plays a pivotal role in regulating the effector phase of T cell responses through binding with its ligands PD-L1 and programmed death ligand 2 (PD-L2) (21). PD-L1 is expressed constitutively on hematopoietic cells and a wide range of non-hematopoietic cells, including hepatocytes, astrocytes, epithelial cells, muscle cells including cardiomyocytes, vascular endothelial cells, and pancreatic cells (22, 23). PD-L1 is also expressed on numerous tumors, and its expression is reported to be associated with poor prognosis in several cancers (24–26). In contrast to PD-L1, PD-L2 is expressed primarily on APCs and certain B cell lymphomas (20, 21). Similar to CTLA-4 signaling, PD-1 signaling abrogates T cell proliferation and cytokine production and reduces T cell survival (23). PD-1 exhibits minimal expression on resting immune cells. However, upon activation, PD-1 expression is induced on the surface of T cells, B cells, natural killer cells, natural killer T cells, dendritic cells, and macrophages (23).
To date, six ICIs have been approved by the United States Food and Drug Administration: ipilimumab (anti-CTLA-4 mAb); nivolumab and pembrolizumab (anti-PD-1 mAbs); and atezolizumab, avelumab, and durvalumab (anti-PD-L1 mAbs) (Table 1). Antibody therapies against the CTLA-4 and PD-1/PD-L1 axes have revolutionized the treatment of cancer (Figure 1).
Figure 1. Distinct roles of CTLA-4 and PD-1/PD-L1 in the regulation of antitumor immune responses. (A) Interactions between CTLA-4/B7 and PD-1/PD-L1 inhibit T cell-mediated tumor cell killing. (B) Blockade of CTLA-4, PD-1, and PD-L1 results in T cell activation and proliferation, which reactivates T cell-mediated tumor cell killing.
Cancer is characterized by genetic mutations that can lead to the expression of various tumor-associated antigens. APCs present these antigens via MHC molecules expressed on their surface, which interact with TCRs. Thus, T cells can recognize tumor-associated antigens as “non-self” and attack tumor cells expressing these antigens (27). However, CTLA-4 inhibits T cell activation and clonal expansion. In the tumor immunotherapy setting, CTLA-4-targeting mAbs support the activation and proliferation of effector T cells, resulting in broad activation of immune responses against tumor cells (28). In contrast, CTLA-4 blockade inhibits regulatory T cell-mediated immunosuppression (28). These are thought to be the main mechanisms of action of CTLA-4 blockade.
PD-1 is expressed on tumor-infiltrating lymphocytes in many cancers. Chronic or high exposure to tumor antigens can induce persistent PD-1 expression, which leads to a state of exhaustion or anergy (lack of response). PD-1 blockade may reverse anergy of tumor-specific T cells. PD-1 is upregulated on the cell surface of many different tumor types. Tumor PD-L1 expression indicates an active tumor immune microenvironment and is strongly associated with efficacious responses to PD-1- and PD-L1-targeting mAbs (29). It is commonly accepted that PD-L1 expression on tumors and immune cells can inhibit the T-cell antitumor response and facilitate cancer development. However, the role of PD-L2 in antitumor immunity remains controversial (30, 31).
Due to the central role played by immune checkpoints in the maintenance of self-tolerance, immune checkpoint blockade can induce a spectrum of adverse events, called irAEs (32). Remarkably, irAEs can affect almost any organ system (Figure 2) and have been reported at a substantially high frequency. irAEs occur in up to 90% of patients (10–15% grade 3/4) treated with ipilimumab (1) and 79% of patients (13% grade 3/4) treated with pembrolizumab (3). In a meta-analysis, the incidence was reported to be 75% in patients treated with CTLA-4-targeting mAbs and 30% for PD-1- and/or PD-L1-blocking mAbs (33). The frequency of grade 3/4 irAEs was substantially higher in patients treated with a combination of ipilimumab and nivolumab (54%) than in those receiving ipilimumab monotherapy (20%) (34).
Figure 2. The clinical spectrum of irAEs associated with immune checkpoint inhibitors. irAEs, immune-related adverse events.
irAEs are generally managed with corticosteroids, and less commonly, with other immunomodulatory agents (35). There are no prospective studies that have investigated management strategies for irAEs. According to the various organs involved, grade 1–2 events mainly affect the gut and skin, whereas grade 3–4 events are mainly restricted to the digestive tract. Cardiac, neurologic, renal, ocular, and hematologic irAEs are uncommon (35).
During the establishment of central tolerance in the thymus, most autoreactive T cells are deleted; however, some autoreactive T cells are released into the periphery (36, 37). In healthy individuals, peripheral tolerance mechanisms regulate the numbers of these cells. CTLA-4 competes with CD28 to downregulate T cell activation, resulting in immunotolerance and prevention of pathologic immune responses to cardiac antigens (38). Interactions between PD-1 and its ligands also maintain cardiac-reactive T cells in an anergic state (38) (Figure 3).
Figure 3. The role of immune checkpoints in establishing peripheral tolerance to the heart. During the establishment of central tolerance in the thymus, most autoreactive T cells are deleted; however, some autoreactive T cells are released into the periphery. In health, peripheral tolerance mechanisms keep these cells in check. CTLA-4 competes with CD28 to downregulate T-cell activation, resulting in immunotolerance and prevention of pathologic immune responses to cardiac-antigens. PD-1-PD-1 ligand interactions also maintain the cardiac-reactive T cells in an anergic state. Antibodies against CTLA-4, PD-1, or PD-L1 may activate cardiac antigen-reactive T cells that escape central tolerance. These T cells can clonally expand and attack the heart.
Mice deficient in CTLA-4 develop severe myocarditis with massive T cell infiltration (39). The cardiac presentation of PD-1-deficient mice is dependent on their background; mice on the BALB/c background develop autoimmune dilated cardiomyopathy (40), but PD-1-deficient autoimmune-prone MRL mice show lymphocytic myocarditis with massive infiltration of CD4+ and CD8+ T cells (41). PD-L1 is significantly upregulated on the surface of cardiac endothelial cells during myocarditis. PD-L1 deficiency in MRL mice induces similar severe myocarditis (42).
There are contrasting reports on the rates of cardiac irAEs associated with ICI therapies. For example, no incidence of myocarditis was identified after a pooled analysis of 448 patients treated with nivolumab and ipilimumab combination therapy (43). In contrast, a pharmacovigilance study identified myocarditis in 18 of 20,594 patients (0.09%) treated with nivolumab alone or in combination with ipilimumab (13), and a cohort study of 964 patients from a multicenter registry reported a prevalence of 1.14%, which increased to as high as 2.4% for combination therapy with anti-PD-1/anti-CTLA-4 (44). According to the latter report, ICI-associated myocarditis can no longer be considered a “rare” adverse effect. So far, ICI-associated myocarditis appears to be a class effect, and the incidence seems to be higher when patients are treated with a combination of ICIs (13, 44).
The most common cardiac irAE is myocarditis (45). Histopathologically, T cell (with a predominance of CD8+ cells) and macrophage infiltration in the myocardium are typically observed in ICI-related myocarditis (10, 12–15, 46). This myocarditis sometimes involves the cardiac conduction system, leading to conduction block. Other presentations of cardiac irAEs include congestive heart failure, Takotsubo cardiomyopathy, pericardial disease, arrhythmias, and conduction disease (47).
The time to onset of myocarditis is variable. An analysis of VigiBase, the World Health Organization's database of individual case safety reports that includes 101 cases of severe myocarditis, revealed that myocarditis was diagnosed at a median of 27 days (range, 5–155 days) after the initiation of ICI therapy, with 76% of the cases occurring in the first 6 weeks of treatment (48). A medical record review of 30 ICI-related cardiotoxicity patients from two cardio-oncology units revealed that the median onset of cardiotoxicity was 65 days (range, 2–454 days) and it occurred after a median of three (range, 1–33) infusions (49). In a multicenter cohort including 35 myocarditis patients, the median time to onset was 34 days and 81% of the patients developed cardiac irAE within 3 months (44). Notably, fatal myocarditis can develop after only a single treatment with an ICI (11, 13). Unfortunately, there is insufficient information regarding the time of onset of cardiac irAEs relating to specific treatment regimes. In general, most irAEs were reported to occur within 3–6 months of the initiation of anti-CTLA-4- or anti-PD-1-targeting therapy (47, 50). While the risk of severe irAEs appears to be dose-dependent with anti-CTLA-4 antibodies, toxicities with anti-PD-1/anti-PD-L1 antibodies are reported to be dose-independent (47, 50).
Cardiac signs and symptoms of ICI-related cardiotoxicity vary from asymptomatic to sudden death (10–17, 51) and lack specificity. Sometimes cardiac irAE is accompanied by other organ irAEs, especially those in skeletal muscles (11, 13).
Currently, patients who are likely to develop cardiac irAEs cannot be identified before ICI therapy. Therefore, early detection of ICI-related myocarditis is thought to be important for improved management. Mahmood et al. (44) showed that measuring troponin levels at baseline and at each cycle of ICI treatment may be useful for surveillance because this parameter was abnormal in 94% of ICI-myocarditis patients at clinical presentation. In contrast, Sarocchi et al. (52) measured troponin levels at each nivolumab administration in 59 patients and found an elevation in only six patients, none of whom developed overt cardiac irAEs. These researchers mentioned possible reasons for a “false positive” elevation of troponin, including it being a consequence of myocardial oxygen demand-supply mismatch due to aggravation of the clinical status or the presence of subclinical ICI-induced myocarditis. An elevation of troponin indicates the presence of, but not the underlying reason for, myocardial injury. Therefore, myocarditis or other myocardial damage should be considered in cases presenting with elevated troponin, and these patients should be referred immediately to cardiologists/onco-cardiologists for further evaluation (44). Mahmood et al. (44) also reported abnormal electrocardiograms in 89% of ICI-related myocarditis patients. In contrast, NT-ProBNP was abnormal in 66%, and the left ventricular ejection fraction (LVEF) was abnormal in only 49% of these patients. Thus, NT-proBNP or LVEF may be less useful for early diagnosis of cardiac irAEs than troponin or ECG. Information on the utility of hsCRP as an early biomarker of cardiac irAEs is lacking.
Pharmacovigilance data show that the mortality of ICI-associated myocarditis exceeded 60% in patients receiving ipilimumab-nivolumab combination therapy (13). Mahmood et al. (44) reported that nearly half of all myocarditis cases developed a major adverse cardiac event (a combination of cardiovascular death, cardiac arrest, cardiogenic shock, and hemodynamically significant complete heart block). Escudier et al. (49) reported after a medical record review of 30 ICI-related cardiotoxicity patients that eight (27%) died of cardiovascular complications. They also reported that cardiovascular mortality was significantly associated with conduction abnormalities and ipilimumab-nivolumab combination therapy (49).
Currently, there are no guidelines for the treatment of cardiac irAEs. Steroids have been used to treat cardiac irAEs in most cases (11, 13–16, 44, 45). For steroid non-responders, other immunosuppressive agents, high-dose intravenous immunoglobulin therapy, plasmapheresis, and immunoadsorption therapy have been used (45, 53).
ICI therapies have changed the treatment landscape of advanced cancer. As the clinical use of ICI therapy rapidly increases, management of irAEs is extremely important. Cardiac irAEs are relatively rare but can be life-threatening. Large scale, prospective, and longitudinal cohort studies are needed to clarify predisposing risk factors and long-term consequences of ICI-induced cardiac irAEs. In addition to clinical studies, basic studies are crucially needed to provide insights into underlying mechanisms and to find biomarkers to identify high risk patients and minimize the risk of ICI-associated cardiac irAEs.
KT has written the main manuscript text and prepared the figures. MI participated to the redaction of the manuscript and its extensive revision.
This study was supported in part by Grant-in-Aid for Scientific Research (Japan Society for the Promotion of Science KAKENHI Grant Number 17K09567) to KT and a grant from Japan Cardiovascular Research Foundation (The Bayer Scholarship for Cardiovascular Research) to KT.
The authors declare that the research was conducted in the absence of any commercial or financial relationships that could be construed as a potential conflict of interest.
We would like to thank Editage (www.editage.jp) for English language editing.
α-MHC, myosin heavy chain α isoform; APC, antigen-presenting cells; CTLA-4, cytotoxic T-lymphocyte-associated antigen 4; ICI, immune checkpoint inhibitor; irAE, immune-related adverse event; mAb, monoclonal antibody; PD-1, programmed cell death protein 1; PD-L1, programmed cell death ligand 1; TCR, T cell receptor.
1. Hodi FS, O'Day SJ, McDermott DF, Weber RW, Sosman JA, Haanen JB, et al. Improved survival with ipilimumab in patients with metastatic melanoma. N Engl J Med. (2010) 363:711–23. doi: 10.1056/NEJMoa1003466
2. Robert C, Long GV, Brady B, Dutriaux C, Maio M, Mortier L, et al. Nivolumab in previously untreated melanoma without BRAF mutation. N Engl J Med. (2015) 372:320–30. doi: 10.1056/NEJMoa1412082
3. Hamid O, Robert C, Daud A, Hodi FS, Hwu WJ, Kefford R, et al. Safety and tumor responses with lambrolizumab (Anti–PD-1) in melanoma. N Engl J Med. (2013) 369:134–44. doi: 10.1056/NEJMoa1305133
4. Brahmer J, Reckamp KL, Baas P, Crinò L, Eberhardt WEE, Poddubskaya E, et al. Nivolumab versus docetaxel in advanced squamous-cell non–small-cell lung cancer. N Engl J Med. (2015) 373:123–35. doi: 10.1056/NEJMoa1504627
5. Borghaei H, Paz-Ares L, Horn L, Spigel DR, Steins M, Ready NE, et al. Nivolumab versus docetaxel in advanced nonsquamous non–small-cell lung cancer. N Engl J Med. (2015) 373:1627–39. doi: 10.1056/NEJMoa1507643
6. Motzer RJ, Escudier B, McDermott DF, George S, Hammers HJ, Srinivas S, et al. Nivolumab versus everolimus in advanced renal-cell carcinoma. N Engl J Med. (2015) 373:1803–13. doi: 10.1056/NEJMoa1510665
7. Chen TW, Razak AR, Bedard PL, Siu LL, Hansen AR. A systematic review of immune-related adverse event reporting in clinical trials of immune checkpoint inhibitors. Ann Oncol Off J Eur Soc Med Oncol. (2015) 26:1824–9. doi: 10.1093/annonc/mdv182
8. Boutros C, Tarhini A, Routier E, Lambotte O, Ladurie FL, Carbonnel F, et al. Safety profiles of anti-CTLA-4 and anti-PD-1 antibodies alone and in combination. Nat Rev Clin Oncol. (2016) 13:473–86. doi: 10.1038/nrclinonc.2016.58
9. Champiat S, Lambotte O, Barreau E, Belkhir R, Berdelou A, Carbonnel F, et al. Management of immune checkpoint blockade dysimmune toxicities: a collaborative position paper. Ann Oncol. (2016) 27:559–74. doi: 10.1093/annonc/mdv623
10. Koelzer VH, Rothschild SI, Zihler D, Wicki A, Willi B, Willi N, et al. Systemic inflammation in a melanoma patient treated with immune checkpoint inhibitors—an autopsy study. J Immunother Cancer (2016) 4:13. doi: 10.1186/s40425-016-0117-1
11. Kimura T, Fukushima S, Miyashita A, Aoi J, Jinnin M, Kosaka T, et al. Myasthenic crisis and polymyositis induced by one dose of nivolumab. Cancer Sci. (2016) 107:1055–8. doi: 10.1111/cas.12961
12. Läubli H, Balmelli C, Bossard M, Pfister O, Glatz K, Zippelius A, et al. Acute heart failure due to autoimmune myocarditis under pembrolizumab treatment for metastatic melanoma. J Immunother Cancer (2015) 3:11. doi: 10.1186/s40425-015-0057-1
13. Johnson DB, Balko JM, Compton ML, Chalkias S, Gorham J, Xu Y, et al. Fulminant myocarditis with combination immune checkpoint blockade. N Engl J Med. (2016) 375:1749–55. doi: 10.1056/NEJMoa1609214
14. Tadokoro T, Keshino E, Makiyama A, Sasaguri T, Ohshima K, Katano H, et al. Acute lymphocytic myocarditis with anti-PD-1 antibody nivolumab. Circ Heart Fail. (2016) 9:e003514. doi: 10.1161/CIRCHEARTFAILURE.116.003514
15. Heinzerling L, Ott PA, Hodi FS, Husain AN, Tajmir-Riahi A, Tawbi H, et al. Cardiotoxicity associated with CTLA4 and PD1 blocking immunotherapy. J Immunother Cancer (2016) 4:50. doi: 10.1186/s40425-016-0152-y
16. Semper H, Muehlberg F, Schulz-Menger J, Allewelt M, Grohé C. Drug-induced myocarditis after nivolumab treatment in a patient with PDL1- negative squamous cell carcinoma of the lung. Lung Cancer (2016) 99:117–9. doi: 10.1016/j.lungcan.2016.06.025
17. Gibson R, Delaune J, Szady A, Markham M. Suspected autoimmune myocarditis and cardiac conduction abnormalities with nivolumab therapy for non-small cell lung cancer. BMJ Case Rep. (2016) 2016:bcr2016216228. doi: 10.1136/bcr-2016-216228
18. Chen L, Flies DB. Molecular mechanisms of T cell co-stimulation and co-inhibition. Nat Rev Immunol. (2013) 13:227–42. doi: 10.1038/nri3405
19. Walker LSK, Sansom DM. Confusing signals: recent progress in CTLA-4 biology. Trends Immunol. (2015) 36:63–70. doi: 10.1016/j.it.2014.12.001
20. Intlekofer AM, Thompson CB. At the bench: preclinical rationale for CTLA-4 and PD-1 blockade as cancer immunotherapy. J Leukoc Biol. (2013) 94:25–39. doi: 10.1189/jlb.1212621
21. Buchbinder EI, Desai A. CTLA-4 and PD-1 pathways: similarities, differences, and implications of their inhibition. Am J Clin Oncol. (2016) 39:98–106. doi: 10.1097/COC.0000000000000239
22. Gianchecchi E, Delfino DV, Fierabracci A. Recent insights into the role of the PD-1/PD-L1 pathway in immunological tolerance and autoimmunity. Autoimmun Rev. (2013) 12:1091–100. doi: 10.1016/j.autrev.2013.05.003
23. Keir ME, Butte MJ, Freeman GJ, Sharpe AH. PD-1 and its ligands in tolerance and immunity. Annu Rev Immunol. (2008) 26:677–704. doi: 10.1146/annurev.immunol.26.021607.090331
24. Hino R, Kabashima K, Kato Y, Yagi H, Nakamura M, Honjo T, et al. Tumor cell expression of programmed cell death-1 ligand 1 is a prognostic factor for malignant melanoma. Cancer (2010) 116:1757–66. doi: 10.1002/cncr.24899
25. Thompson RH, Gillett MD, Cheville JC, Lohse CM, Dong H, Webster WS, et al. Costimulatory B7-H1 in renal cell carcinoma patients: Indicator of tumor aggressiveness and potential therapeutic target. Proc Natl Acad Sci USA. (2004) 101:17174–9. doi: 10.1073/pnas.0406351101
26. Mu CY, Huang JA, Chen Y, Chen C, Zhang XG. High expression of PD-L1 in lung cancer may contribute to poor prognosis and tumor cells immune escape through suppressing tumor infiltrating dendritic cells maturation. Med Oncol. (2011) 28:682–8. doi: 10.1007/s12032-010-9515-2
27. Chen DS, Mellman I. Oncology meets immunology: the cancer-immunity cycle. Immunity (2013) 39:1–10. doi: 10.1016/j.immuni.2013.07.012
28. Pardoll DM. The blockade of immune checkpoints in cancer immunotherapy. Nat Rev Cancer (2012) 12:252–64. doi: 10.1038/nrc3239
29. Pitt JM, Vétizou M, Daillère R, Roberti MP, Yamazaki T, Routy B, et al. Resistance mechanisms to immune-checkpoint blockade in cancer: tumor-intrinsic and -extrinsic factors. Immunity (2016) 44:1255–69. doi: 10.1016/j.immuni.2016.06.001
30. Umezu D, Okada N, Sakoda Y, Adachi K, Ojima T, Yamaue H, et al. Inhibitory functions of PD-L1 and PD-L2 in the regulation of anti-tumor immunity in murine tumor microenvironment. Cancer Immunol Immunother. (2018) doi: 10.1007/s00262-018-2263-4. [Epub ahead of print].
31. Wang H, Yao H, Li C, Liang L, Zhang Y, Shi H, et al. PD-L2 expression in colorectal cancer: Independent prognostic effect and targetability by deglycosylation. Oncoimmunology (2017) 6:e1327494. doi: 10.1080/2162402X.2017.1327494
32. Postow MA, Sidlow R, Hellmann MD. Immune-related adverse events associated with immune checkpoint blockade. N Engl J Med. (2018) 378:158–68. doi: 10.1056/NEJMra1703481
33. Bertrand A, Kostine M, Barnetche T, Truchetet ME, Schaeverbeke T. Immune related adverse events associated with anti-CTLA-4 antibodies: systematic review and meta-analysis. BMC Med. (2015) 13:211. doi: 10.1186/s12916-015-0455-8
34. Hodi FS, Chesney J, Pavlick AC, Robert C, Grossmann KF, McDermott DF, et al. Combined nivolumab and ipilimumab versus ipilimumab alone in patients with advanced melanoma: 2-year overall survival outcomes in a multicentre, randomised, controlled, phase 2 trial. Lancet Oncol. (2016) 17:1558–68. doi: 10.1016/S1470-2045(16)30366-7
35. Palmieri DJ, Carlino MS. Immune checkpoint inhibitor toxicity. Curr Oncol Rep. (2018) 20:72. doi: 10.1007/s11912-018-0718-6
36. Lv H, Lipes MA. Role of impaired central tolerance to α-myosin in inflammatory heart disease. Trends Cardiovasc Med. (2012) 22:113–7. doi: 10.1016/j.tcm.2012.07.005
37. Strasser A, Puthalakath H, O'Reilly LA, Bouillet P. What do we know about the mechanisms of elimination of autoreactive T and B cells and what challenges remain. Immunol Cell Biol. (2008) 86:57–66. doi: 10.1038/sj.icb.7100141
38. Mueller DL. Mechanisms maintaining peripheral tolerance. Nat Immunol. (2010) 11:21–7. doi: 10.1038/ni.1817
39. Waterhouse P, Penninger JM, Timms E, Wakeham A, Shahinian A, Lee KP, et al. Lymphoproliferative disorders with early lethality in mice deficient in Ctla-4. Science (1995) 270:985–8.
40. Nishimura H, Okazaki T, Tanaka Y, Nakatani K, Hara M, Matsumori A, et al. Autoimmune dilated cardiomyopathy in PD-1 receptor-deficient mice. Science (2001) 291:319–22. doi: 10.1126/science.291.5502.319
41. Wang J, Okazaki IM, Yoshida T, Chikuma S, Kato Y, Nakaki F, et al. PD-1 deficiency results in the development of fatal myocarditis in MRL mice. Int Immunol. (2010) 22:443–52. doi: 10.1093/intimm/dxq026
42. Lucas JA, Menke J, Rabacal WA, Schoen FJ, Sharpe AH, Kelley VR. Programmed death ligand 1 regulates a critical checkpoint for autoimmune myocarditis and pneumonitis in MRL mice. J Immunol. (2008) 181:2513–21. doi: 10.4049/jimmunol.181.4.2513
43. Sznol M, Ferrucci PF, Hogg D, Atkins MB, Wolter P, Guidoboni M, et al. Pooled analysis safety profile of nivolumab and ipilimumab combination therapy in patients with advanced melanoma. J Clin Oncol. (2017) 35:3815–22. doi: 10.1200/JCO.2016.72.1167
44. Mahmood SS, Fradley MG, Cohen JV, Nohria A, Reynolds KL, Heinzerling LM, et al. Myocarditis in patients treated with immune checkpoint inhibitors. J Am Coll Cardiol. (2018) 71:1755–64. doi: 10.1016/j.jacc.2018.02.037
45. Mir H, Alhussein M, Alrashidi S, Alzayer H, Alshatti A, Valettas N, et al. Cardiac complications associated with checkpoint inhibition: a systematic review of the literature in an important emerging area. Can J Cardiol. (2018) 34:1059–68. doi: 10.1016/j.cjca.2018.03.012
46. Tajiri K, Aonuma K, Sekine I. Immune checkpoint inhibitor-related myocarditis. Jpn J Clin Oncol. (2018) 48:7–12. doi: 10.1093/jjco/hyx154
47. Kumar V, Chaudhary N, Garg M, Floudas CS, Soni P, Chandra AB. Current diagnosis and management of immune related adverse events (irAEs) induced by immune checkpoint inhibitor therapy. Front Pharmacol. (2017) 8:49. doi: 10.3389/fphar.2017.00049
48. Moslehi JJ, Salem JE, Sosman JA, Lebrun-Vignes B, Johnson DB. Increased reporting of fatal immune checkpoint inhibitor-associated myocarditis. Lancet (2018) 391:933. doi: 10.1016/S0140-6736(18)30533-6
49. Escudier M, Cautela J, Malissen N, Ancedy Y, Orabona M, Pinto J, et al. Clinical features, management, and outcomes of immune checkpoint inhibitor–related cardiotoxicity. Circulation (2017) 136:2085–7. doi: 10.1161/CIRCULATIONAHA.117.030571
50. Michot JM, Bigenwald C, Champiat S, Collins M, Carbonnel F, Postel-Vinay S, et al. Immune-related adverse events with immune checkpoint blockade: a comprehensive review. Eur J Cancer (2016) 54:139–48. doi: 10.1016/j.ejca.2015.11.016
51. Thibault C, Vano Y, Soulat G, Mirabel M. Immune checkpoint inhibitors myocarditis: not all cases are clinically patent. Eur Heart J. (2018) 39:3553. doi: 10.1093/eurheartj/ehy485
52. Sarocchi M, Grossi F, Arboscello E, Bellodi A, Genova C, Dal Bello MG, et al. Serial troponin for early detection of nivolumab cardiotoxicity in advanced non-small cell lung cancer patients. Oncologist (2018) 23:936–42. doi: 10.1634/theoncologist.2017-0452
53. Caforio ALP, Pankuweit S, Arbustini E, Basso C, Gimeno-Blanes J, Felix SB, et al. Current state of knowledge on aetiology, diagnosis, management, and therapy of myocarditis: a position statement of the European Society of Cardiology Working Group on Myocardial and Pericardial Diseases. Eur Heart J. (2013) 34:2636–48. doi: 10.1093/eurheartj/eht210
Keywords: immune checkpoint inhibitors, myocarditis, cardiotoxicity, programmed cell death protein 1, cytotoxic T-lymphocyte antigen 4, immune-related adverse events, immune checkpoint, autoimmunity
Citation: Tajiri K and Ieda M (2019) Cardiac Complications in Immune Checkpoint Inhibition Therapy. Front. Cardiovasc. Med. 6:3. doi: 10.3389/fcvm.2019.00003
Received: 21 September 2018; Accepted: 07 January 2019;
Published: 23 January 2019.
Edited by:
Pietro Enea Lazzerini, Università degli Studi di Siena, ItalyReviewed by:
Ingrid E. Dumitriu, St George's, University of London, United KingdomCopyright © 2019 Tajiri and Ieda. This is an open-access article distributed under the terms of the Creative Commons Attribution License (CC BY). The use, distribution or reproduction in other forums is permitted, provided the original author(s) and the copyright owner(s) are credited and that the original publication in this journal is cited, in accordance with accepted academic practice. No use, distribution or reproduction is permitted which does not comply with these terms.
*Correspondence: Kazuko Tajiri, a3RhamlyaUBtZC50c3VrdWJhLmFjLmpw
Disclaimer: All claims expressed in this article are solely those of the authors and do not necessarily represent those of their affiliated organizations, or those of the publisher, the editors and the reviewers. Any product that may be evaluated in this article or claim that may be made by its manufacturer is not guaranteed or endorsed by the publisher.
Research integrity at Frontiers
Learn more about the work of our research integrity team to safeguard the quality of each article we publish.