- 1Molecular Cardiology, Whitaker Cardiovascular Institute, Boston University School of Medicine, Boston, MA, USA
- 2Cardiovascular Medicine, Whitaker Cardiovascular Institute, Boston University School of Medicine, Boston, MA, USA
Visceral adiposity is much more strongly associated with cardiometabolic disease in humans than subcutaneous adiposity. Browning, the appearance of brown-like adipocytes in the white adipose tissue (WAT), has been shown to protect mice against metabolic dysfunction, suggesting the possibility of new therapeutic approaches to treat obesity and type 2 diabetes. In mice, subcutaneous WAT depots express higher levels of browning genes when compared with visceral WAT, further suggesting that differences in WAT browning could contribute to the differences in the pathogenicity of the two depots. However, the expression of browning genes in different WAT depots of human has not been characterized. Here, it is shown that the expression of browning genes is higher in visceral than in subcutaneous WAT in humans, a pattern that is opposite to what is observed in mice. These results suggest that caution should be applied in extrapolating the results of murine browning gene expression studies to human pathophysiology.
Obesity is a major risk factor for cardiometabolic disease, and clinical studies have shown that different human adipose tissue depots contribute differentially to cardiometabolic risk. The accumulation of intra-abdominal visceral fat is a major contributor to systemic metabolic dysfunction that is strongly associated with cardiovascular risk in humans (1–3). In marked contrast, subcutaneous adipose tissue is benign or even protective with regard to cardiometabolic risk in some studies (4–6). An increasing body of evidence suggests that visceral and subcutaneous white adipose tissue (WAT) depots exhibit different intrinsic properties, which make visceral WAT a more pathogenic depot compared to subcutaneous WAT (7, 8). Surprisingly, the phenomenon of WAT “browning,” an actively investigated topic in the field of metabolism, remains ill defined in the context of human visceral and subcutaneous adiposity.
In contrast to WAT, brown adipose tissue (BAT) is a highly vascularized and has a high content of mitochondria (9). These mitochondria express high levels of uncoupling protein 1 (UCP1), allowing them to produce heat. In addition to this thermogenic function, BAT contributes significantly to systemic metabolism in rodent models because of its high energetic expenditure ratio (10–15). Recently, it has been appreciated that adult humans possess varying degrees of active BAT (16–19). Because BAT function will decrease with obesity and aging (17, 20), declining BAT function may link metabolic dysfunction and weight gain under these conditions.
“Browning” is the process by which some adipocytes within WAT depots acquire properties of brown adipocytes, i.e., an increase in mitochondrial content and oxidative capacity (21, 22). The browning of WAT occurs in response to various stimuli, including exposure to cold temperatures (23), β3-selective adrenergic agonists (24, 25), or exercise (26, 27). These adipocytes, termed “beige” or “brite” (brown in white), show an intermediate phenotype between white and brown adipocytes, exhibiting multilocular morphology, high levels of UCP1 expression, and some degree of thermogenic capacity. In addition, these cells have a distinct molecular signature, expressing markers, such as Tbx1, that are not present in white or brown adipocytes (28). Mouse studies have shown a correlation between WAT browning and decreased body weight gain and improved metabolic outcome when mice were challenged with obesogenic diets (21, 27, 29, 30). However, these systemic metabolic improvements could also be attributed to diminished BAT “whitening” and an increase in the BAT function under these conditions (9). Notably, studies have suggested the existence of major differences in the browning capacity of different WAT depots in mice (21, 30). In humans, browning has been observed in subcutaneous WAT of burned patients (31) and cancer cachexia (32); two conditions that are characterized by hypermetabolism, large increases in resting energy expenditure and whole-body catabolism and associated with increased morbidity and mortality. Thus, more information about the browning potential of human WAT depots and its metabolic implications is necessary to be able to extrapolate the effects observed in mice.
The brown adipose determination factor Prdm16 has been reported to play a central role in the browning capacity of WAT. Prdm16 is differentially expressed in various mouse WAT depots, and this differential expression has been suggested to mediate differences in their browning capacity. Specifically, the higher expression of Prdm16 and other BAT-selective genes has been shown to correlate with the higher browning susceptibility of subcutaneous (inguinal) WAT compared to the visceral (epididymal) WAT in lean wild-type mice (21). Consistently, subcutaneous WAT in adipose tissue-specific Prdm16-deficient mice adopts visceral WAT-like qualities and exhibits decreased browning capacity, and this correlates with greater weight gain and insulin resistance (30). These mouse studies suggest that differences in browning susceptibility could contribute to the intrinsic differences between WAT depots that determine their differential impacts on cardiometabolic risk (33, 34). However, previous studies have almost exclusively focused on the murine system, and the expression of Prdm16 and other browning-related genes in human WAT depots has not been explored.
In this study, we compared the expression of browning genes in subcutaneous and visceral WAT collected from obese human individuals and chronically obese mice to gain insight into the susceptibility of different WAT depots to browning in a context of chronic obesity. Consistent with previously published data in lean mice (21), subcutaneous (inguinal) WAT in obese mice displayed higher transcript levels of the browning markers UCP1, Cidea, and Pdrm16, and the beige adipocyte markers Tbx1 and P2rx5, compared with their expression levels in visceral (epididymal) WAT (Figure 1A). Correspondingly, the mitochondrial genes Cox8b, Ppargc1a, Atp5a, and Ndufa1 are more highly expressed in inguinal compared to epididymal WAT. These mouse data are consistent with the prevailing notion that subcutaneous WAT is more susceptible to browning than visceral WAT.
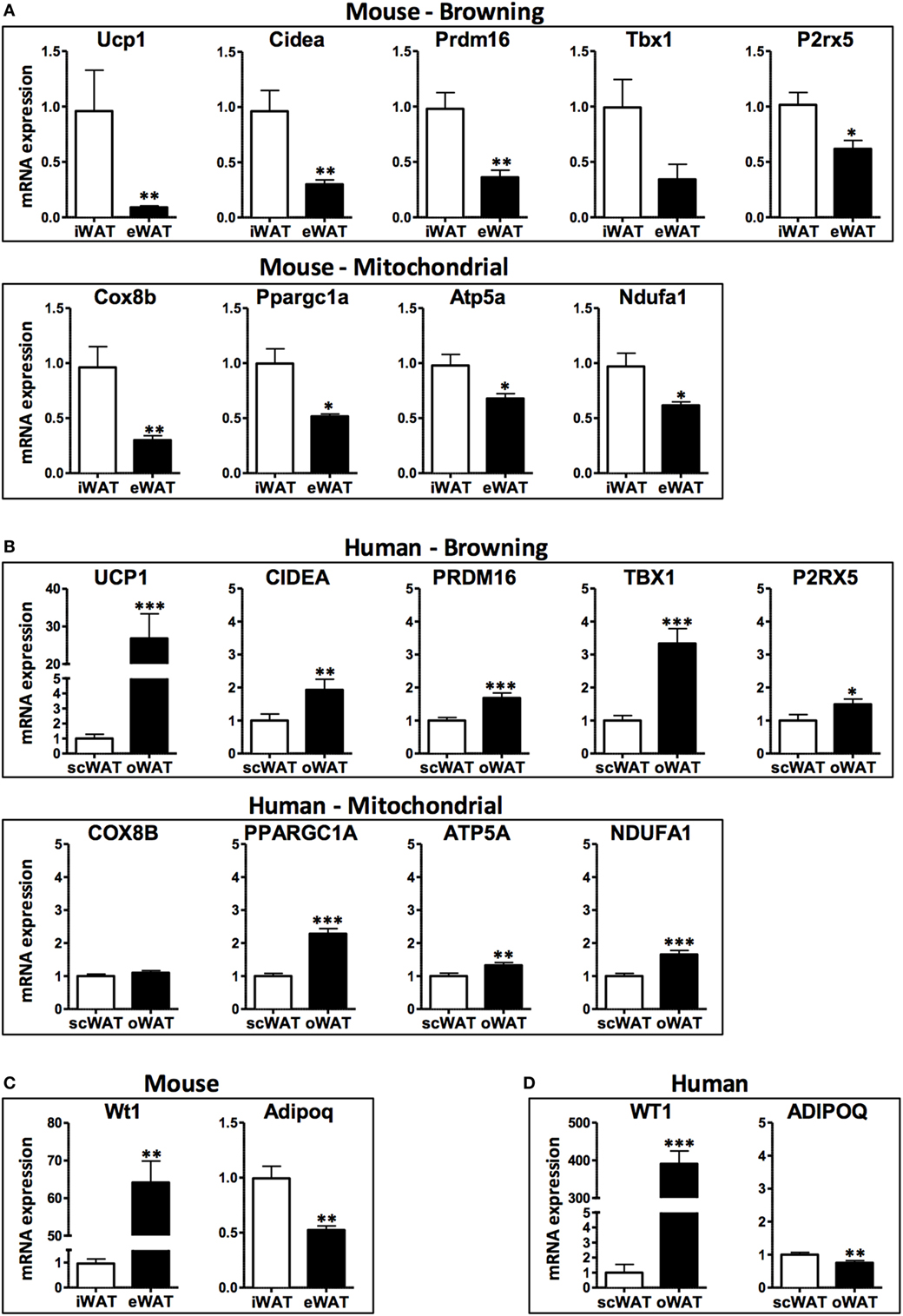
Figure 1. mRNA levels in subcutaneous and visceral white adipose tissue (WAT) depots of obese mice and humans. (A) Browning and mitochondrial genes in iWAT (inguinal, subcutaneous) and eWAT (epididymal, visceral) depots of mice, average Ct values: Ucp1: 27.1; Cidea: 27.9; Prdm16: 28.6; Tbx1: 35.0; P2rx5: 28.4; Cox8b: 22.5; Ppargc1a: 27.2; Atp5a: 19.7; Ndufa1: 21.3 and (B) scWAT (subcutaneous, abdominal region) and oWAT (omental, visceral) depots of humans, average Ct values: UCP1: 28.8; CIDEA: 18.3; PRDM16: 26.5; TBX1: 23.1; P2RX5: 23.6; COX8B: 20.1; PPARGC1a: 21.8; ATP5a: 15.9; NDUFA1: 18.3. (C) Visceral marker Wt1 and adipokine adiponectin in iWAT and eWAT depots of mice, average Ct values: Wt1: 30.5; Adipoq: 18.2 and (D) scWAT and oWAT depots of humans, average Ct values: WT1: 24.0; ADIPOQ: 13.6. Samples were collected from: C57BL6/J mice (n = 9) fed a high fat and high sucrose diet for 30 weeks and housed at 21°C (average body weight: 53.5 g, 40.6% weight increase compared to lean controls); and obese humans (n = 21–32) at the time of bariatric surgery, clinical characteristics detailed in Table 1. RNA was isolated using RNeasy Lipid Tissue Mini Kit (Qiagen), and synthesis of cDNA was performed with high capacity cDNA Reverse Transcription Kit (Applied Biosystems). Quantitative real-time PCR reactions were performed in a ViiA7 System (Applied Biosystems) using TaqMan gene expression assays (Applied Biosystems) for the human samples and SYBR Green-based assays for the murine samples (primer sequences obtained from http://mouseprimerdepot.nci.nih.gov).
In contrast, the analysis of human WAT specimens suggests a markedly different scenario (Figure 1B). Compared with subcutaneous WAT, visceral (omental) WAT exhibits higher transcript levels of UCP1 and all of the browning genes examined in this study (CIDEA, PRDM16, TBX1, and P2RX5). Consistently, human visceral WAT displayed higher levels of the mitochondrial gene transcripts COX8B, PPARGC1A, ATP5A, and NDUFA1. Overall, these data suggest that human visceral fat is more likely to exhibit a higher browning capacity than subcutaneous fat.
When interpreting the opposite browning gene expression patterns in WAT depots from human and mouse, it must be considered from the data presented herein, as most studies comparing data on adipose tissue from mice and humans, that the “visceral” designation actually involves a comparison of different intra-abdominal WAT depots. In this regard, the human omental fat depot is essentially non-existent in mice. Furthermore, the mouse epididymal or perigonadal fat depot does not drain into the portal circulation and therefore is technically not a true visceral depot. Despite these anatomical differences, mouse subcutaneous-epididymal characteristics frequently track with human subcutaneous-omental characteristics. For example, the expression of the anti-inflammatory adipokine adiponectin (ADIPOQ/Adipoq) is decreased in visceral depots relative to subcutaneous fat in both human and mice, and the transcript levels of the visceral WAT marker Wilms tumor 1 (WT1/Wt1) (30) are increased in both mouse epididymal fat and human omental fat (Figures 1C,D). Furthermore, the expression of various pro-inflammatory molecules is similarly elevated in both human and mouse visceral WAT depots compared to subcutaneous WAT (35–38).
The issue of thermoneutrality should be considered when analyzing the browning process in mice and humans. Humans have replaced the endogenous thermoregulatory mechanisms with the donning of clothing and macro-environmental manipulations (39). However, mice are commonly housed at temperatures below their thermoneutral range, leading to mild cold stress that can affect many biological processes (40, 41). For example, cold acclimatization is associated with large increases in UCP1 gene expression in beige adipose tissue in mice housed at 21°C (common conditions), but this increase is not observed in mice housed at 30°C (thermoneutrality) (42). Accordingly, mice housed at 21°C (including the mice used in this study) exhibit a perpetual activation of brown and beige adipose tissues.
Conflicting findings of mouse and human studies in the setting of browning have been reported by other groups. WAT browning has not been observed in response to exercise in humans (43), in contrast to mice (26, 27). In addition, a study of combined diet and exercise weigh loss intervention in women did not result in a higher beige adipocyte induction, but in a decrease in UCP1 expression and a remodeling of scWAT toward a more-white phenotype instead of a more-brown phenotype (44). These studies suggest that human adipose tissue does not respond to browning stimuli identified in mouse models.
Overall, our findings suggest that mouse epididymal fat mimics some of the pathogenic properties of human visceral fat, but it is not a valid surrogate for human visceral fat in the context of browning. Since most of the studies comparing subcutaneous and visceral depots in mice and humans use these WAT depots, we urge caution when extrapolating results from mice to humans. In the case of WAT browning, differences in gene expression patterns between fat depots cannot be extrapolated from mouse to humans. Our data also suggest that it is unlikely that the increased cardiometabolic risk associated with visceral adiposity in humans is due to the reduced browning capacity of visceral adipose tissue.
Ethics Statement
The human study was approved by Boston Medical Center Institutional Review Board, and all subjects gave written informed consent. The animal study was approved by the Institutional Animal Care and Use Committee at Boston University Medical Campus.
Author Contributions
MZ acquired and analyzed the data. MZ and JF drafted the paper. NG and KW provided supervision and funding and critical revisions to the manuscript.
Conflict of Interest Statement
The authors declare that the research was conducted in the absence of any commercial or financial relationships that could be construed as a potential conflict of interest.
Funding
This work was funded by NIH grants HL120160, HL131006, HL132564, and AG052160 to KW and HL081587 and HL126141 to NG and KW.
References
1. Pou KM, Massaro JM, Hoffmann U, Vasan RS, Maurovich-Horvat P, Larson MG, et al. Visceral and subcutaneous adipose tissue volumes are cross-sectionally related to markers of inflammation and oxidative stress: the Framingham Heart Study. Circulation (2007) 116(11):1234–41. doi: 10.1161/CIRCULATIONAHA.107.710509
2. Neeland IJ, Turer AT, Ayers CR, Powell-Wiley TM, Vega GL, Farzaneh-Far R, et al. Dysfunctional adiposity and the risk of prediabetes and type 2 diabetes in obese adults. JAMA (2012) 308(11):1150–9. doi:10.1001/2012.jama.11132
3. Abraham TM, Pedley A, Massaro JM, Hoffmann U, Fox CS. Association between visceral and subcutaneous adipose depots and incident cardiovascular disease risk factors. Circulation (2015) 132(17):1639–47. doi:10.1161/CIRCULATIONAHA.114.015000
4. Snijder MB, Visser M, Dekker JM, Goodpaster BH, Harris TB, Kritchevsky SB, et al. Low subcutaneous thigh fat is a risk factor for unfavourable glucose and lipid levels, independently of high abdominal fat. The Health ABC Study. Diabetologia (2005) 48(2):301–8. doi:10.1007/s00125-004-1637-7
5. Porter SA, Massaro JM, Hoffmann U, Vasan RS, O’Donnel CJ, Fox CS. Abdominal subcutaneous adipose tissue: a protective fat depot? Diabetes Care (2009) 32(6):1068–75. doi:10.2337/dc08-2280
6. McLaughlin T, Lamendola C, Liu A, Abbasi F. Preferential fat deposition in subcutaneous versus visceral depots is associated with insulin sensitivity. J Clin Endocrinol Metab (2011) 96(11):E1756–60. doi:10.1210/jc.2011-0615
7. Tchkonia T, Thomou T, Zhu Y, Karagiannides I, Pothoulakis C, Jensen MD, et al. Mechanisms and metabolic implications of regional differences among fat depots. Cell Metab (2013) 17(5):644–56. doi:10.1016/j.cmet.2013.03.008
8. Fuster JJ, Ouchi N, Gokce N, Walsh K. Obesity-induced changes in adipose tissue microenvironment and their impact on cardiovascular disease. Circ Res (2016) 118(11):1786–807. doi:10.1161/CIRCRESAHA.115.306885
9. Shimizu I, Aprahamian T, Kikuchi R, Shimizu A, Papanicolaou KN, MacLauchlan S, et al. Vascular rarefaction mediates whitening of brown fat in obesity. J Clin Invest (2014) 124(5):2099–112. doi:10.1172/JCI71643
10. Nicholls DG. The thermogenic mechanism of brown adipose tissue. Review. Biosci Rep (1983) 3(5):431–41. doi:10.1007/BF01121954
11. Hamann A, Flier JS, Lowell BB. Decreased brown fat markedly enhances susceptibility to diet-induced obesity, diabetes, and hyperlipidemia. Endocrinology (1996) 137(1):21–9. doi:10.1210/endo.137.1.8536614
12. Nedergaard J, Bengtsson T, Cannon B. Unexpected evidence for active brown adipose tissue in adult humans. Am J Physiol Endocrinol Metab (2007) 293(2):E444–52. doi:10.1152/ajpendo.00691.2006
13. Bartelt A, Bruns OT, Reimer R, Hohenberg H, Ittrich H, Peldschus K, et al. Brown adipose tissue activity controls triglyceride clearance. Nat Med (2010) 17(2):200–5. doi:10.1038/nm.2297
14. Enerback S. Human brown adipose tissue. Cell Metab (2010) 11(4):248–52. doi:10.1016/j.cmet.2010.03.008
15. Nedergaard J, Cannon B. The changed metabolic world with human brown adipose tissue: therapeutic visions. Cell Metab (2010) 11(4):268–72. doi:10.1016/j.cmet.2010.03.007
16. Stephens M, Ludgate M, Rees DA. Brown fat and obesity: the next big thing? Clin Endocrinol (Oxf) (2011) 74(6):661–70. doi:10.1111/j.1365-2265.2011.04018.x
17. Cypess AM, Lehman S, Williams G, Tal I, Rodman D, Goldfine AB, et al. Identification and importance of brown adipose tissue in adult humans. N Engl J Med (2009) 360(15):1509–17. doi:10.1056/NEJMoa0810780
18. Seale P, Lazar MA. Brown fat in humans: turning up the heat on obesity. Diabetes (2009) 58(7):1482–4. doi:10.2337/db09-0622
19. Tseng YH, Cypess AM, Kahn CR. Cellular bioenergetics as a target for obesity therapy. Nat Rev Drug Discov (2010) 9(6):465–82. doi:10.1038/nrd3138
20. Pfannenberg C, Werner MK, Ripkens S, Stef I, Deckert A, Schmadl M, et al. Impact of age on the relationships of brown adipose tissue with sex and adiposity in humans. Diabetes (2010) 59(7):1789–93. doi:10.2337/db10-0004
21. Seale P, Conroe HM, Estall J, Kajimura S, Frontini A, Ishibashi J, et al. Prdm16 determines the thermogenic program of subcutaneous white adipose tissue in mice. J Clin Invest (2011) 121(1):96–105. doi:10.1172/JCI44271
22. Bonet ML, Oliver P, Palou A. Pharmacological and nutritional agents promoting browning of white adipose tissue. Biochim Biophys Acta (2013) 1831(5):969–85. doi:10.1016/j.bbalip.2012.12.002
23. Petrovic N, Walden TB, Shabalina IG, Timmons JA, Cannon B, Nedergaard J. Chronic peroxisome proliferator-activated receptor gamma (PPARgamma) activation of epididymally derived white adipocyte cultures reveals a population of thermogenically competent, UCP1-containing adipocytes molecularly distinct from classic brown adipocytes. J Biol Chem (2010) 285(10):7153–64. doi:10.1074/jbc.M109.053942
24. Cousin B, Cinti S, Morroni M, Raimbault S, Ricquier D, Penicaud L, et al. Occurrence of brown adipocytes in rat white adipose tissue: molecular and morphological characterization. J Cell Sci (1992) 103(Pt 4):931–42.
25. Ghorbani M, Himms-Hagen J. Appearance of brown adipocytes in white adipose tissue during CL 316,243-induced reversal of obesity and diabetes in Zucker fa/fa rats. Int J Obes Relat Metab Disord (1997) 21(6):465–75. doi:10.1038/sj.ijo.0800432
26. Bostrom P, Wu J, Jedrychowski MP, Korde A, Ye L, Lo JC, et al. A PGC1-alpha-dependent myokine that drives brown-fat-like development of white fat and thermogenesis. Nature (2012) 481(7382):463–8. doi:10.1038/nature10777
27. Stanford KI, Middelbeek RJ, Goodyear LJ. Exercise effects on white adipose tissue: beiging and metabolic adaptations. Diabetes (2015) 64(7):2361–8. doi:10.2337/db15-0227
28. Cao L, Choi EY, Liu X, Martin A, Wang C, Xu X, et al. White to brown fat phenotypic switch induced by genetic and environmental activation of a hypothalamic-adipocyte axis. Cell Metab (2011) 14(3):324–38. doi:10.1016/j.cmet.2011.06.020
29. Feldmann HM, Golozoubova V, Cannon B, Nedergaard J. UCP1 ablation induces obesity and abolishes diet-induced thermogenesis in mice exempt from thermal stress by living at thermoneutrality. Cell Metab (2009) 9(2):203–9. doi:10.1016/j.cmet.2008.12.014
30. Cohen P, Levy JD, Zhang Y, Frontini A, Kolodin DP, Svensson KJ, et al. Ablation of PRDM16 and beige adipose causes metabolic dysfunction and a subcutaneous to visceral fat switch. Cell (2014) 156(1–2):304–16. doi:10.1016/j.cell.2013.12.021
31. Patsouris D, Qi P, Abdullahi A, Stanojcic M, Chen P, Parousis A, et al. Burn induces browning of the subcutaneous white adipose tissue in mice and humans. Cell Rep (2015) 13(8):1538–44. doi:10.1016/j.celrep.2015.10.028
32. Petruzzelli M, Schweiger M, Schreiber R, Campos-Olivas R, Tsoli M, Allen J, et al. A switch from white to brown fat increases energy expenditure in cancer-associated cachexia. Cell Metab (2014) 20(3):433–47. doi:10.1016/j.cmet.2014.06.011
33. Harms M, Seale P. Brown and beige fat: development, function and therapeutic potential. Nat Med (2013) 19(10):1252–63. doi:10.1038/nm.3361
34. Wu J, Cohen P, Spiegelman BM. Adaptive thermogenesis in adipocytes: is beige the new brown? Genes Dev (2013) 27(3):234–50. doi:10.1101/gad.211649.112
35. Farb MG, Ganley-Leal L, Mott M, Liang Y, Ercan B, Widlansky ME, et al. Arteriolar function in visceral adipose tissue is impaired in human obesity. Arterioscler Thromb Vasc Biol (2012) 32:467–73. doi:10.1161/ATVBAHA.111.235846
36. Fried SK, Bunkin DA, Greenberg AS. Omental and subcutaneous adipose tissues of obese subjects release interleukin-6: depot difference and regulation by glucocorticoid. J Clin Endocrinol Metab (1998) 83:847–50. doi:10.1210/jcem.83.3.4660
37. Catalan V, Gomez-Ambrosi J, Rodriguez A, Perez-Hernandez AI, Gurbindo J, Ramirez B, et al. Activation of noncanonical Wnt signaling through WNT5A in visceral adipose tissue of obese subjects is related to inflammation. J Clin Endocrinol Metab (2014) 99(8):E1407–17. doi:10.1210/jc.2014-1191
38. Fuster JJ, Zuriaga MA, Ngo DT, Farb MG, Aprahamian T, Yamaguchi TP, et al. Noncanonical Wnt signaling promotes obesity-induced adipose tissue inflammation and metabolic dysfunction independent of adipose tissue expansion. Diabetes (2015) 64(4):1235–48. doi:10.2337/db14-1164
39. Cannon B, Nedergaard J. Nonshivering thermogenesis and its adequate measurement in metabolic studies. J Exp Biol (2011) 214(Pt 2):242–53. doi:10.1242/jeb.050989
40. Overton JM. Phenotyping small animals as models for the human metabolic syndrome: thermoneutrality matters. Int J Obes (Lond) (2010) 34(Suppl 2):S53–8. doi:10.1038/ijo.2010.240
41. Maloney SK, Fuller A, Mitchell D, Gordon C, Overton JM. Translating animal model research: does it matter that our rodents are cold? Physiology (Bethesda) (2014) 29(6):413–20. doi:10.1152/physiol.00029.2014
42. Kalinovich AV, de Jong JM, Cannon B, Nedergaard J. UCP1 in adipose tissues: two steps to full browning. Biochimie (2017) 134:127–37. doi:10.1016/j.biochi.2017.01.007
43. Norheim F, Langleite TM, Hjorth M, Holen T, Kielland A, Stadheim HK, et al. The effects of acute and chronic exercise on PGC-1alpha, irisin and browning of subcutaneous adipose tissue in humans. FEBS J (2014) 281(3):739–49. doi:10.1111/febs.12619
Keywords: browning, adipose tissue, gene expression, mouse, human
Citation: Zuriaga MA, Fuster JJ, Gokce N and Walsh K (2017) Humans and Mice Display Opposing Patterns of “Browning” Gene Expression in Visceral and Subcutaneous White Adipose Tissue Depots. Front. Cardiovasc. Med. 4:27. doi: 10.3389/fcvm.2017.00027
Received: 01 March 2017; Accepted: 18 April 2017;
Published: 05 May 2017
Edited by:
Mengwei Zang, University of Texas Health Science Center at San Antonio, USAReviewed by:
Jeonga Kim, University of Alabama at Birmingham, USAYu Li, Shanghai Institutes for Biological Sciences (CAS), China
Copyright: © 2017 Zuriaga, Fuster, Gokce and Walsh. This is an open-access article distributed under the terms of the Creative Commons Attribution License (CC BY). The use, distribution or reproduction in other forums is permitted, provided the original author(s) or licensor are credited and that the original publication in this journal is cited, in accordance with accepted academic practice. No use, distribution or reproduction is permitted which does not comply with these terms.
*Correspondence: Kenneth Walsh, a3h3YWxzaEBidS5lZHU=