- 1Cardiothoracic Unit, Great Ormond Street Hospital for Children NHS Foundation Trust, London, UK
- 2Centre for Cardiovascular Imaging, Institute of Cardiovascular Science, University College London, London, UK
Diastolic function is an important component of left ventricular (LV) function which is often overlooked. It can cause symptoms of heart failure in patients even in the presence of normal systolic function. The parameters used to assess diastolic function often measure flow and are affected by the loading conditions of the heart. The interpretation of diastolic function in the context of congenital heart disease requires some understanding of the effects of the lesions themselves on these parameters. Individual congenital lesions will be discussed in this paper. Recently, load-independent techniques have led to more accurate measurements of ventricular compliance and remodeling in heart disease. The combination of inflow velocities and tissue Doppler measurements can be used to estimate diastolic function and LV filling pressures. This review focuses on diastolic function and assessment in congenital heart disease.
Introduction
Myocardial function has been extensively studied in the context of congenital heart disease. The focus to date has been on systolic function due to its importance and ease of measurement. However, the heart also needs to be adequately filled in order to function optimally, and this aspect of cardiac function is relatively under-investigated. The difficulties (as with systolic function) come when attempting to measure the relaxation of the myocardium while negating any effect of pre- or afterload. This is a difficult task and has led to the development of several tools which track myocardial movement independently of the usual flow-based parameters; the latter are heavily influenced by loading conditions. This article will summarize the current assessment of diastolic function using echocardiography and cardiac magnetic resonance (CMR) imaging in the normal heart and in patients with congenital heart disease.
Diastolic Dysfunction (DD)
Diastole denotes the filling phase of the cardiac cycle. Filling is determined by myocardial relaxation as well as atrial contraction and atrial and ventricular compliance. Myocardial relaxation begins when the myofibrils return to an unstressed state and this precedes mitral valve (MV) opening (isovolumic relaxation). ATP is used to actively uncouple calcium from the contractile apparatus and return it to the sarcoplasmic reticulum. Active relaxation is only responsible for early diastolic filling, whereas compliance is important throughout filling and especially during atrial contraction.
The early part of diastole is active relaxation, which is an energy-consuming process. The latter part is due to compliance or stiffness of the ventricle. Isovolumic relaxation time (IVRT) can be measured by invasive catheterization measurements. The index used in its measurement is the time constant of isovolumic pressure decline (τ). In non-invasive measurement, IVRT is the closest measurement to assess this value. However, as with all indices of diastolic function, the loading conditions must be taken into account.
The stiffness of the myocardium also plays an important role in diastolic function. The mass of the left ventricular (LV) affects the stiffness as do the viscoelastic properties of the myocardium (cellular and extracellular components). Attempts are made to measure this increase in myocardial stiffness. However, the difficulty arises in the mechanism of measurement as well as the timing and nature of diastole. Flow-based measurements rely on a change in volume to occur, and so they are unable to quantify isovolumic relaxation as they assess only the last stage of diastole. There is also no universal measurement of diastole [equivalent to ejection fraction (EF) in systole] and torsion and dyssynchrony are difficult to quantify.
Non-Invasive Assessment Methods
Echocardiography
As the ventricle stiffens, the velocity of blood flowing into the ventricle decreases. This downward trend would continue if not for compensation which takes the form of increased heart rate or increased diastolic filling pressure to maintain stroke volume (SV). The latter causes an increase toward normal filling velocities (pseudonormalization) and a prolongation of rapid filling. Ventricular elastance is an important marker for ventricular stiffness and is correlated with increased cardiac morbidity (1). Increased strength of atrial contraction is a related compensatory mechanism to cope with increased diastolic filling pressures.
Left Atrium (LA) Volume
The LA is exposed to the LV loading pressure during MV opening and gradually remodels and increases in size. As LA remodeling takes place over time, it is a marker of the duration of DD as well as severity. The amount of dilatation also correlates with cardiovascular risk burden (2). There is a correlation between LA size and the risk of developing congestive cardiac failure (3, 4), atrial fibrillation (5), and ischemic heart disease (6). LA size is also a predictor of adverse outcome in patients with hypertrophic cardiomyopathy (7). LA size is measured from the apical four-chamber view at end-systole. It is also possible to calculate LA area from 2D four-chamber and two-chamber views.
Transmitral Doppler Inflow
The mitral inflow velocity profile helps characterize LV inflow dynamics. It is best measured from the apical four-chamber view (in both children and adults) with the cursor placed across the MV just inside the LV. The E wave is the early diastolic filling wave seen on Doppler interrogation of the MV. It is caused by the drop of LV pressure below LA pressure during the cardiac cycle and is therefore influenced by LA pressure, LV compliance, and the rate of LV relaxation. The A wave, or atrial contraction wave, is immediately after the E wave on Doppler flow analysis. This is influenced by LV compliance and LA pressure and LA contractility rate. All MV inflow velocities are affected by preload and afterload. Under normal conditions, the E velocity is greater than A velocity (Figure 1). As the ventricle becomes less compliant, the E velocity decreases and the ratio lowers. When the A velocity surpasses the E velocity, true DD is present. The mitral inflow is affected by preload, heart rate (including arrhythmias) and age (8).
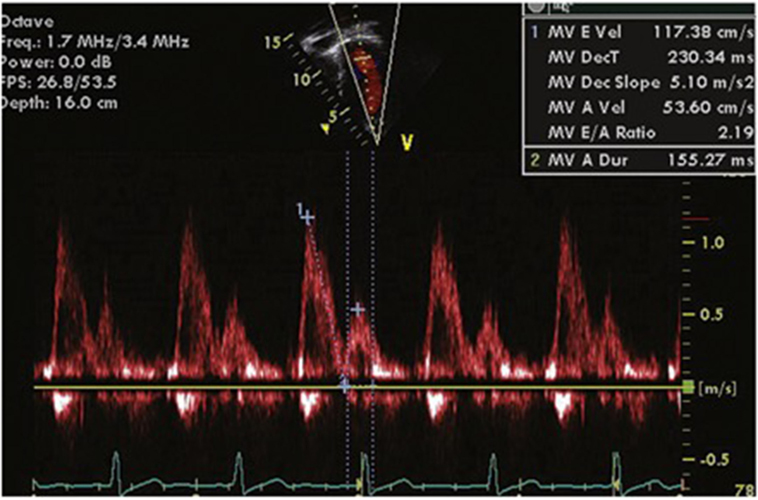
Figure 1. Normal mitral inflow velocity profile. Maximum E Velocity (cm/s) = early diastolic mitral inflow velocity. MV Deceleration Time (ms) = duration of deceleration of E wave. MV Dec Slope (m/s2) = rate of decrease of E wave. Maximum A Velocity (cm/s) = atrial component of mitral filling. A wave duration (ms) = duration of A wave. MV E/A ratio = ratio of E velocity to A velocity (normal value <8).
E wave deceleration time is the rate at which the atrial and ventricular pressures equilibrate after onset of the E wave and is shorter in compliant ventricles (160–240 ms in adults). The IVRT is the period between closure of the aortic valve and opening of the MV. This is normally 70–90 ms long in adults and is prolonged in the case of decreased LV compliance. It is also affected by heart rate and ventricular function. It is best recorded from the apical five-chamber view with the cursor placed to record LV outflow tract velocities and LV inflow simultaneously.
Pulmonary Venous (PV) Inflow
Pulmonary venous flow can enhance the information provided by MV inflow velocities. The pulsed-waved Doppler cursor should be placed in the right or left upper pulmonary vein from the apical four-chamber view, as distally into the vein as possible. The variables which are measured include peak systolic flow velocity (S), the peak diastolic flow velocity (D), peak atrial reversal flow velocity (AR), and AR duration (ARdur). The normal PV flow profile shows an initial, large S wave followed by a small D wave and then some retrograde flow during atrial contraction. As LA pressures increase, flow becomes predominantly diastolic and the S/D ratio reverses. A decreased systolic fraction of 40% is associated with elevated mean LA pressure of >15 mmHg (9). AR and ARdur also help, as an increase in AR velocity and duration indicates increased LA pressure.
Color M-Mode Doppler
An intraventricular pressure gradient exists between the base and apex of the ventricle, which acts to cause a suction effect on blood during diastole (10, 11). This can be measured using color M-mode across the MV (from the apical four-chamber view) and measuring the slope of the first aliasing velocity (red–blue) from the MV plane to 4 cm distal in the LV (Vp in centimeters per second) (12). This is known as the color M-mode velocity propagation index (Vp). Vp is not subject to pseudonormalization, which suggests that it is preload independent (13). It does not change with alteration of preload in dogs (14, 15) and humans (14, 16). There is an inverse correlation between the isovolumetric time constant of relaxation (τ) and Vp in humans (13, 14, 17) and dogs (14). Vp is associated with ventricular wall relaxation, becoming less steep as diastolic function worsens (17). The ratio of early LV filling (E) to Vp is a commonly used parameter, which corresponds to pulmonary capillary wedge pressure (PCWP), brain natriuretic peptide, and NYHA class (16).
Assessment of Severity
There are different grades of DD. Early (grade I/impaired relaxation) dysfunction is caused by a decrease in LV compliance, thereby leading to increased LV filling pressure. This delays atrial emptying and prolongs the E wave deceleration time (DT > 240 ms). Atrial contraction becomes more vigorous, reducing the E/A ratio to <0.9. Worsening LV DD leads to increased atrial pressure and a decrease in the pressure gradient between the LA and LV, thereby leading to a shortened DT. The E/A ratio increases (0.9–1.5), but the E/A profile may appear normal (grade II or pseudonormalization). However, the e′ velocity on tissue Doppler imaging (TDI) (see below) remains low, giving a clue to the underlying abnormality (18). In grade III dysfunction (restrictive), the E/A ratio is >2, DT <160 ms, and the inflow profile can be altered by the Valsalva maneuver. This works because LA pressure is reduced during the strain phase of the Valsalva maneuver, and this unmasks the underlying DD. In grade IV dysfunction, the abnormalities are fixed in the face of the Valsalva maneuver, as LA pressure is too elevated to respond to decreased preload (see Figure 2). The timing of onset of E and e′ waves is important. Normally, the e′ occurs at the onset of or before the E wave. If the LA pressure is elevated, the E wave may precede e′ (19, 20).
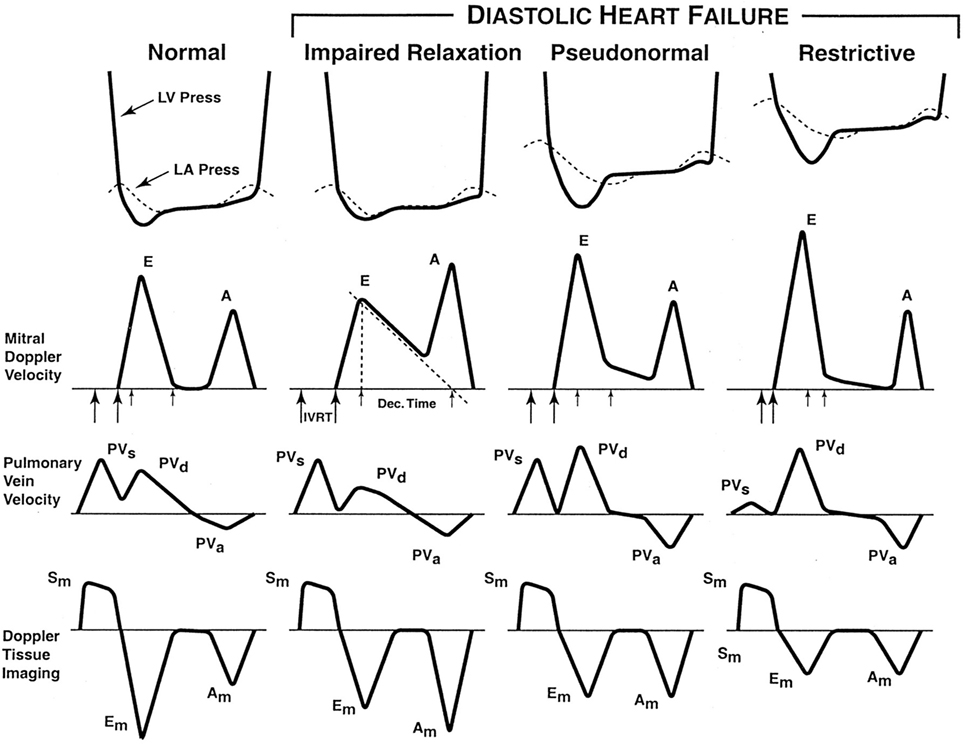
Figure 2. The stages of diastolic heart failure. LV and left atrial (LA) pressures during diastole, transmitral Doppler LV inflow velocity, pulmonary vein Doppler velocity, and Doppler tissue velocity. IVRT indicates isovolumic relaxation time; Dec. Time, e-wave deceleration time; E, early LV filling velocity; A, velocity of LV filling contributed by atrial contraction; PVs, systolic pulmonary vein velocity; PVd, diastolic pulmonary vein velocity; PVa, pulmonary vein velocity resulting from atrial contraction; Sm, myocardial velocity during systole; Em, myocardial velocity during early filling; and Am, myocardial velocity during filling produced by atrial contraction.
Tissue Doppler Imaging
Tissue Doppler imaging directly measures myocardial wall velocities by focusing on the high-amplitude, low-frequency signals reflected by the myocardium rather than the blood pool. The areas sampled include the lateral aspect of the mitral annulus in the apical four-chamber view, the basal septal region in the same view, and the lateral tricuspid valve annulus. This serves to minimize translational artifact and to align the probe with the direction of movement. Three waves are usually seen—the systolic (s′) wave, the early diastolic (e′) wave, and the late diastolic wave caused by atrial contraction (a′). Normal values and Z-scores are available for each age group in pediatrics (21).
Nagueh et al. were the first to show that E/e′ ratio (ratio of transmitral E velocity and TDI mitral annular e′ velocity) corresponded to PCWP (18). In 125 patients classified by systolic and diastolic function and symptoms, PCWP correlated strongly with E/e′ ratio r = 0.87. PCWP correlated only weakly with E velocity but not e′ velocity. Patients with abnormal relaxation and pseudonormalization of the mitral inflow E/A ratio had a decreased e′ velocity (P < 0.001). In patients with DD, a saline bolus affects the E/A wave as measured by transmitral Doppler measurement but did not affect the e′ or e′/a′ ratios (22). These studies show that e′ acts as a preload-independent marker of LV relaxation. E wave velocity on mitral inflow Doppler, corrected for e′, correlates strongly with PCWP, and can be used to estimate LA pressure non-invasively.
Tissue Doppler imaging can also differentiate between restrictive cardiomyopathy and constrictive pericarditis (23). As e′ is a property of the myocardium, theoretically, it should remain unchanged in the presence of extrinsic constriction and should only be reduced in true restrictive cardiomyopathy. This is indeed the case, with a significantly lower e′ in restrictive cardiomyopathy than constrictive pericarditis (P < 0.001) (23). This has been corroborated by other groups (24, 25).
E/e′ ratio is an independent predictor of outcome in patients assessed 1–6 days after acute myocardial infarction (26). In a population study of 2,042 patients in the community, any degree of DD was predictive of all-cause mortality, whether the patient had clinical symptoms or not (27). In the ADEPT trial, of 225 patients with symptomatic heart failure (HF), diastolic parameters including shorter deceleration time, lower S/D pulmonary vein flow ratio, and increasing E/e′ and E/Vp ratios were all independent predictors of the primary end-points of death, hospitalization, or transplantation (28).
Emerging Techniques for Assessment of Diastolic Function
Torsion
Measurement of LV torsion provides insight into an important mechanism of LV filling and ejection. LV rotation is sensitive to changes in regional and global LV function (29–31). MRI tagging can be used for this purpose but can be difficult and costly to obtain (31–34). Speckle tracking may be used to measure torsion, using the largely experimental practice of “torsion echocardiography” (35–37).
Strain
Strain is a dimensionless index of change in myocardial length in response to applied force and is expressed as a fraction or percentage change. Strain rate is the change in length over time (per second). By convention, myocardial lengthening or thinning is given a positive value. The techniques used to measure strain include echocardiography M-mode (38) or TDI (39, 40) as well as MRI tagging (41, 42). The limitations of echocardiographic measurement include beam direction, which will allow measurement of longitudinal, radial, and circumferential directions depending on the view and angle of interrogation.
Strain imaging aims to provide a high-resolution, real-time measure of myocardial deformation which is independent of loading conditions. A normal pattern of diastolic relaxation has been studied and described (43). Strain imaging can be used to distinguish between restrictive cardiomyopathy and constrictive pericarditis (44) as well as physiological hypertrophy and hypertrophic cardiomyopathy (45).
In a study of 194 patients with chronic systolic HF, global longitudinal strain (GLS) correlated with worse NYHA class and higher NT-proBNP. It also correlated with LV structure and LVEF, as well as LV and RV DD. GLS also predicted long-term adverse events after adjustment for age, ischemic etiology, E/e′ septal, and NT-proBNP with HR 2.04 (P = 0.024).
CMR Imaging
Magnetic resonance imaging can be used to assess diastolic function by the inflow of blood or the movement of myocardium in much the same way as echocardiography. A number of techniques exist which can help to evaluate diastolic function: gradient echo assesses functional dimensions; phase-contrast measures flow, and myocardial tagging measures regional dynamics (46). Flow measurements are more complete as they include the entire annulus, rather than a point as in echocardiography. Tissue phase mapping (TPM) can be used to measure myocardial velocities and obtain similar information to TDI. This process allows calculation of E/Ea ratio (E = MV inflow E wave and Ea = early myocardial relaxation on TPM).
It is also possible to evaluate tissue characteristics (47), including interstitial and replacement fibrosis using CMR. In a study of 50 patients, with reduced EF, late gadolinium enhancement (a technique which identifies replacement fibrosis) was correlated with a lower septal E/e′ ratio than patients without a scar (P = 0.05).
Measurement of dimensions and flow are evaluated similarly to echocardiographic measures, although volumes are more accurate. In tagging, the myocardium is labeled using selective saturation prepulses in specific myocardial regions perpendicular to the imaging plane. This allows strain and 3-D motion analysis (48).
Cellular Changes with Aging
Increased ventricular stiffness and a change in the microscopic structure of the myocardium are inevitable parts of aging. This, coupled with changes in vascular stiffness, may lead to increased vulnerability in certain groups to developing symptomatic HF (1). Traditional assessment of systolic heart function will not identify these patients. The increased stiffness of the myocardium is thought to be due to changes in the collagen content of the extracellular matrix and increased fibrosis (49). There are other changes including reduced phosphorylation of sarcomeric proteins (50) and changes in Titin (51), which may be of importance at a cellular level.
Congenital Heart Disease
Overview
There are five main classes of CHD, which affect diastolic function. Pressure–overload lesions such as aortic stenosis and systemic hypertension cause a decrease in compliance due to hypertrophy. Volume overload leads to increased compliance up until a point, when hypertrophy or fibrosis occurs. Mixed pressure and volume overload can combine to affect compliance, such as in repaired Tetralogy of Fallot with some pulmonary valve stenosis and incompetence. Primary or secondary myocardial diseases can decrease compliance directly, such as in amyloidosis or restrictive cardiomyopathy. Transposition of the great arteries leads to a special situation in which the RV is faced with increased afterload and the LV with a much lower pressure than normal, both of which may cause decreased compliance (52). A summary of lesions and the changes in various parameters used to quantify DD is provided in Table 1.
Tetralogy of Fallot
As with the left ventricle, the right ventricle can become stiff and restrictive due to hypertrophy. In Tetralogy of Fallot, the RV becomes a stiff conduit due to RV outflow tract obstruction, with poor diastolic function. This is evidenced by anterograde pulmonary flow with atrial contraction (end-diastolic forward flow in the PA). The presence of DD is a marker of poor short-term surgical outcome. In a study of 50 children with TOF (mean age 5.0 years), 24 had restrictive RV physiology as described. This correlated with lower E/A ratio and IVRT duration. DD also correlated with prolonged intensive care unit stay, longer duration of ionotropic support, and higher doses of diuretics. It was more commonly seen in patients after transannular patch repair (53). In a study of 112 patients, 50 were found to have a restrictive RV. This was associated with larger RV dimensions and RA dimensions, and increased LA length and LA indexed volume on echocardiography (54). There appeared to be a bigger effect on late filling than early filling of the LV and RV restriction appeared to affect LV filling and diastole (decreasing filling and increasing diastolic pressures). This may be due to mechanical effects of the RV on LV filling or increased fibrosis of the LV. Interestingly, children with restrictive RV have an increased RV volume, whereas adults have a reduced volume (55–57). After the initial period, restrictive RV physiology appears to be protective, with decreased duration of pulmonary regurgitation and better maximum oxygen uptake seen in patients with restriction (58).
Single Ventricle
The Fontan procedure suddenly offloads a previously volume-overloaded ventricle. There is evidence that even in patients with normal systolic function, diastolic function is impaired (59). This is correlated with the length of time since the Fontan operation and decreases over time. Both left and right systemic single ventricles appear to experience systolic and DD, although systemic right ventricular function is more depressed (60). This may be due to adverse remodeling of the right ventricle, which is being exposed to an abnormally high afterload. A study of 28 patients showed a significant correlation between LV filling pressures (measured by E/e′ ratio) and ventilatory efficiency (VE/VO2 slope) (r = 0.93; P < 0.01) (61). This is interesting as parameters of CPET are correlated with hospitalization in Fontan patients (62).
Atrial Septal Defect (ASD)
In the presence of an ASD with normal pulmonary pressures, the left–right shunt results in reduced LV filling and SV, reduced tissue perfusion, fluid accumulation, increased RV volume, greater RV SV, and finally normalization of LV filling and SV (63). In a small study of patients (n = 18) undergoing percutaneous device closure of their ASD, there were no significant changes in TDI and Doppler M-mode indices of diastolic function after closure. However, E wave velocity and E/e′ ratio at the MV annulus did increase significantly, thereby suggesting they are more load-dependent parameters (64). In children with ASD, TDI velocities do not change immediately after device closure. There is also no indication of elevation of left heart filling pressures after device closure in children, suggesting that children are able to accept increased preload and preserve diastolic function (65). This has implications on timing of closure, suggesting that earlier closure is beneficial.
Aortic Stenosis
In a study of patients with aortic valve disease (8–39 years), those with aortic stenosis or mixed disease were found to have DD, which was related to the degree of left ventricular hypertrophy (LVH). E/e′ correlated with LV end-diastolic pressure on catheterization. DD was found in 37% of all patients in the study, consisting of 37% of those with AS and 47% of those with mixed valve disease (66). Increased chamber stiffness is related to an increased LV mass/EDV ratio. Patients with aortic stenosis have increased interstitial fibrosis, which is related to a worse prognosis (67) and is known to be related to increased chamber stiffness. Secondary pulmonary hypertension may occur due to DD (68).
Cardiac magnetic resonance has a role in functional assessment of the LV in patients with AS. It is also used in tissue characterization and can quantify the degree of interstitial fibrosis and replacement fibrosis (69). There is some evidence that the degree of fibrosis can predict surgical outcome (67).
Aortic Regurgitation
An incompetent aortic valve increases the end-diastolic volume of the left ventricle. The LV remodels to cope with this extra volume and becomes more compliant, so that diastolic pressures remain normal. Over time, decompensation may occur, in which case diastolic pressures increase as the LV loses the ability to compensate further (70). This is due to increased myocyte cellular diameter and fibrous content of the myocardium (71).
In aortic regurgitation with normal diastolic function, MV inflow consists of predominant E wave filling and annular e′ is increased or normal due to increased LV SV. However, E/e′ is not increased and PA pressures are normal. With the onset of DD (DD), LV filling pressures are elevated on exercise initially and then at rest.
Mitral Stenosis
Mitral stenosis results in increased LV filling pressure and reduced LV filling due to the restriction of blood flow through the MV. Most patients have normal intrinsic systolic and diastolic myocardial function. Some studies have found DD using conductance catheters, which are independent of loading conditions and acutely reversed after balloon valvuloplasty (72). The mechanism for this is not clear and may be due to restriction of LV relaxation by an immobile and thickened MV.
Mitral Regurgitation
In isolated MR, LV compliance usually decreases as it dilates to accommodate an increased volume (73). In acute MR, increased LV diastolic pressure is due to increased LV dilatation and a shift upward on the pressure–volume relationship. Chronic MR often leads to remodeling and LV dilatation, thereby retaining LV SV (74).
MR, in the absence of LV DD, will cause an increase in E/A ratio (>1) and increase in transmitral E velocity (75). The e′ velocity is also increased, and E/e′ is not indicative of filling pressures (76). However, A reversal wave velocity does relate to LV diastolic pressures independently of MR (77). The ratio of IVRT to TE–e′ can also be used to assess diastolic function irrespective of MR (77).
Cardiomyopathies
HF with Preserved Ejection Fraction (HFpEF)
One theory of the mechanism of HFpEF is that it is caused by DD. Increased LV filling pressures cause back pressure on the pulmonary circulation, leading to symptoms of HF, including breathlessness. This is assumed to be the case as EF remains in the normal range, which is thought to denote normal systolic function (78). However, there have been studies which show that symptoms of HF in these patients correlate with left ventricular end-diastolic volume and that the SV is only maintained due to LV dilatation. The mechanism postulated is that of excessive LV diastolic dilatation by fiber slippage and creep (79).
The process of LV remodeling to compensate for decreased systolic function in these patients occurs due to feedback from the periphery, causing the heart to adapt with an increase in volume to maintain SV (80). Therefore, an EF of 20% in a dilated ventricle may produce the same SV of a normally sized ventricle with a normal EF (81). Patients with LVH manage to avoid this excessive distension and may be more prone to HF with reduced EF (76). Symptoms of HF, such as breathlessness on exertion, are not related to PCWP (82) or systolic function (83). Instead, the determinants appear to be musculoskeletal status, body composition, motivation, and tolerance of discomfort (82). Therefore, using symptoms alone to determine whether a patient has HF may not be valid.
A number of problems with the definition of HFpEF have been highlighted above; HF may not be reliably diagnosed using symptoms alone, and a preserved EF does not always correlate with normal systolic function. The notion of this type of disease being the definitive model for DD is flawed.
Hypertrophic Cardiomyopathy
Diastolic dysfunction is well recognized in hypertrophic cardiomyopathy, due to the active component of actin–myosin dissociation in the early filling phase and the passive compliance of the left ventricle (84–87). DD causes a reduced rate and magnitude of LV filling and reduced SV. This results in elevation of LV end-diastolic pressures leading to symptoms of HF. Evaluation of diastolic function is similar to other conditions, with values and ratios changing with severity as expected. In some patients, a restrictive phenotype is present, characterized by increased mitral inflow E/A ratio, reduced DT, and increased pulmonary vein A reversal wave velocity (88). TDI may help to distinguish mild disease in the seemingly normal hearts of disease-causing gene carriers (89, 90).
Conclusion
Diastolic dysfunction is a characteristic of many types of congenital heart disease as well as of infancy and the aging heart. Thorough and thoughtful evaluation of diastolic function can help to explain symptoms and affect the treatment of patients with seemingly normal ventricular function. Ventricular function should be thought of as a combination of ventricular filling as well as systolic ejection so that the contribution of ventricular compliance to overall heart function is taken into account. Finally, loading conditions and effects of exertion should be taken into account during evaluation.
Author Contributions
Literature search and writing initial draft: DP. Critical revision and editing: MB. Final draft: DP.
Conflict of Interest Statement
The authors declare that the research was conducted in the absence of any commercial or financial relationships that could be construed as a potential conflict of interest.
Funding
This study was funded by Information Communication Technologies Programme (contract number 600932), part of the MD-Paedigree project.
References
1. Redfield M, Jacobsen SJ, Borlaug BA, Rodeheffer RJ, Kass DA. Age- and gender-related ventricular-vascular stiffening: a community-based study. Circulation (2005) 112(15):2254–62. doi: 10.1161/CIRCULATIONAHA.105.541078
2. Tsang TS, Barnes ME, Gersh BJ, Bailey KR, Seward JB. Left atrial volume as a morphophysiologic expression of left ventricular diastolic dysfunction and relation to cardiovascular risk burden. Am J Cardiol (2002) 90(12):1284–9. doi:10.1016/S0002-9149(02)02864-3
3. Takemoto Y. Usefulness of left atrial volume in predicting first congestive heart failure in patients > or = 65 years of age with well-preserved left ventricular systolic function. Am J Cardiol (2005) 96(6):832–6. doi:10.1016/j.amjcard.2005.05.031
4. Douglas P. The left atrium: a biomarker of chronic diastolic dysfunction and cardiovascular disease risk. J Am Coll Cardiol (2003) 42(7):1206–7. doi:10.1016/S0735-1097(03)00956-2
5. Tsang T, Gersh BJ, Appleton CP, Tajik AJ, Barnes ME, Bailey KR, et al. Left ventricular diastolic dysfunction: an important predictor of first diagnosed nonvalvular atrial fibrillation in 840 elderly men and women. J Am Coll Cardiol (2002) 39:460–460. doi:10.1016/S0735-1097(02)82067-8
6. Tsang TS, Barnes ME, Gersh BJ, Takemoto Y, Rosales AG, Bailey KR, et al. Prediction of risk for first age-related cardiovascular events in an elderly population: the incremental value of echocardiography. J Am Coll Cardiol (2003) 42(7):1199–205. doi:10.1016/S0735-1097(03)00943-4
7. Nistri S, Olivotto I, Betocchi S, Losi MA, Valsecchi G, Pinamonti B, et al. Prognostic significance of left atrial size in patients with hypertrophic cardiomyopathy (from the Italian registry for hypertrophic cardiomyopathy). Am J Cardiol (2006) 98(7):960–5. doi:10.1016/j.amjcard.2006.05.013
8. Lester S, Tajik AJ, Nishimura RA, Oh JK, Khandheria BK, Seward JB, et al. Unlocking the mysteries of diastolic function. J Am Coll Cardiol (2008) 51(7):679–89. doi:10.1016/j.jacc.2007.09.061
9. Kuecherer HF, Kuecherer HF, Muhiudeen IA, Kusumoto FM, Lee E, Moulinier LE, et al. Estimation of mean left atrial pressure from transesophageal pulsed Doppler echocardiography of pulmonary venous flow. Circulation (1990) 82(4):1127–39. doi:10.1161/01.CIR.82.4.1127
10. Ling D, Rankin JS, Edwards CH II, McHale PA, Anderson RW. Regional diastolic mechanics of the left ventricle in the conscious dog. Am J Physiol (1979) 5(2):H323–30.
11. Courtois M. Transmitral pressure-flow velocity relation. Importance of regional pressure gradients in the left ventricle during diastole. Circulation (1988) 78(3):661–71.
12. Garcia MJ, Ares MA, Asher C, Rodriguez L, Vandervoort P, Thomas JD. An index of early left ventricular filling that combined with pulsed Doppler peak e velocity may estimate capillary wedge pressure. J Am Coll Cardiol (1997) 29(2):448–54. doi:10.1016/S0735-1097(96)00496-2
13. Takatsuji H, Mikami T, Urasawa K, Teranishi J, Onozuka H, Takagi C, et al. A new approach for evaluation of left ventricular diastolic function: spatial and temporal analysis of left ventricular filling flow propagation by color M-mode Doppler echocardiography. J Am Coll Cardiol (1996) 27(2):365–71. doi:10.1016/0735-1097(96)81240-X
14. Garcia MJ, Smedira NG, Greenberg NL, Main M, Firstenberg MS, Odabashian J, et al. Color M-mode Doppler flow propagation velocity is a preload insensitive index of left ventricular relaxation: animal and human validation. J Am Coll Cardiol (2000) 35(1):201–8. doi:10.1016/S0735-1097(99)00503-3
15. Stugaard M. Intraventricular early diastolic filling during acute myocardial ischemia, assessment by multigated color m-mode Doppler echocardiography. Circulation (1993) 88(6):2705–13. doi:10.1161/01.CIR.88.6.2705
16. Firstenberg MS, Levine BD, Garcia MJ, Greenberg NL, Cardon L, Morehead AJ, et al. Relationship of echocardiographic indices to pulmonary capillary wedge pressures in healthy volunteers. J Am Coll Cardiol (2000) 36(5):1664–9. doi:10.1016/S0735-1097(00)00909-8
17. Brun P, Tribouilloy C, Duval AM, Iserin L, Meguira A, Pelle G, et al. Left ventricular flow propagation during early filling is related to wall relaxation: a color M-mode Doppler analysis. J Am Coll Cardiol (1992) 20(2):420–32. doi:10.1016/0735-1097(92)90112-Z
18. Nagueh SF, Middleton KJ, Kopelen HA, Zoghbi WA, Quiñones MA. Doppler tissue imaging: a noninvasive technique for evaluation of left ventricular relaxation and estimation of filling pressures. J Am Coll Cardiol (1997) 30(6):1527–33. doi:10.1016/S0735-1097(97)00344-6
19. Oh J, Tajik J. The return of cardiac time intervals. J Am Coll Cardiol (2003) 42(8):1471–4. doi:10.1016/S0735-1097(03)01036-2
20. Rivas Gotz C, Khoury DS, Manolios M, Rao L, Kopelen HA, Nagueh SF, et al. Time interval between onset of mitral inflow and onset of early diastolic velocity by tissue Doppler: a novel index of left ventricular relaxation. J Am Coll Cardiol (2003) 42(8):1463–70. doi:10.1016/S0735-1097(03)01034-9
21. Mori K, Hayabuchi Y, Kuroda Y, Nii M, Manabe T. Left ventricular wall motion velocities in healthy children measured by pulsed wave Doppler tissue echocardiography: normal values and relation to age and heart rate. J Am Soc Echocardiogr (2000) 13(11):1002–11. doi:10.1067/mje.2000.108131
22. Sohn DW, Chai IH, Lee DJ, Kim HC, Kim HS, Oh BH, et al. Assessment of mitral annulus velocity by Doppler tissue imaging in the evaluation of left ventricular diastolic function. J Am Coll Cardiol (1997) 30(2):474–80. doi:10.1016/S0735-1097(97)88335-0
23. Garcia MJ, Rodriguez L, Ares M, Griffin BP, Thomas JD, Klein AL. Differentiation of constrictive pericarditis from restrictive cardiomyopathy: assessment of left ventricular diastolic velocities in longitudinal axis by Doppler tissue imaging. J Am Coll Cardiol (1996) 27(1):108–14. doi:10.1016/0735-1097(95)00434-3
24. Oki T, Tabata T, Yamada H, Abe M, Onose Y, Wakatsuki T, et al. Right and left ventricular wall motion velocities as diagnostic indicators of constrictive pericarditis. Am J Cardiol (1998) 81(4):465–70. doi:10.1016/S0002-9149(97)00939-9
25. Rajagopalan N, Garcia MJ, Rodriguez L, Murray RD, Apperson-Hansen C, Stugaard M, et al. Comparison of new Doppler echocardiographic methods to differentiate constrictive pericardial heart disease and restrictive cardiomyopathy. Am J Cardiol (2001) 87(1):86–94. doi:10.1016/S0002-9149(00)01278-9
26. Hillis G, Møller JE, Pellikka PA, Gersh BJ, Wright RS, Ommen SR, et al. Noninvasive estimation of left ventricular filling pressure by E/e’: a powerful predictor of survival following acute myocardial infarction. J Am Coll Cardiol (2003) 41(6):452–452. doi:10.1016/S0735-1097(03)82510-X
27. Redfield M, Jacobsen SJ, Burnett JC Jr, Mahoney DW, Bailey KR, Rodeheffer RJ. Burden of systolic and diastolic ventricular dysfunction in the community: appreciating the scope of the heart failure epidemic. JAMA (2003) 289(2):194–202. doi:10.1001/jama.289.2.194
28. Troughton RW, Prior DL, Frampton CM, Nash PJ, Pereira JJ, Martin M, et al. Usefulness of tissue Doppler and color M-mode indexes of left ventricular diastolic function in predicting outcomes in systolic left ventricular heart failure (from the ADEPT study). Am J Cardiol (2005) 96(2):257–62. doi:10.1016/j.amjcard.2005.03.055
29. Gibbons Kroeker CA, Tyberg JV, Beyar R. Effects of load manipulations, heart rate, and contractility on left ventricular apical rotation. An experimental study in anesthetized dogs. Circulation (1995) 92(1):130–41. doi:10.1161/01.CIR.92.1.130
30. Hansen DE, Daughters GT II, Alderman EL, Ingels NB, Stinson EB, Miller DC. Effect of volume loading, pressure loading, and inotropic stimulation on left ventricular torsion in humans. Circulation (1991) 83(4):1315–26. doi:10.1161/01.CIR.83.4.1315
31. Maier SE, Fischer SE, McKinnon GC, Hess OM, Krayenbuehl HP, Boesiger P. Evaluation of left ventricular segmental wall motion in hypertrophic cardiomyopathy with myocardial tagging. Circulation (1992) 86(6):1919–28. doi:10.1161/01.CIR.86.6.1919
32. Stuber M, Scheidegger MB, Fischer SE, Nagel E, Steinemann F, Hess OM, et al. Alterations in the local myocardial motion pattern in patients suffering from pressure overload due to aortic stenosis. Circulation (1999) 100(4):361–8. doi:10.1161/01.CIR.100.4.361
33. Nagel E, Stuber M, Burkhard B, Fischer SE, Scheidegger MB, Boesiger P, et al. Cardiac rotation and relaxation in patients with aortic valve stenosis. Eur Heart J (2000) 21(7):582–9. doi:10.1053/euhj.1999.1736
34. Buchalter MB, Weiss JL, Rogers WJ, Zerhouni EA, Weisfeldt ML, Beyar R, et al. Noninvasive quantification of left ventricular rotational deformation in normal humans using magnetic resonance imaging myocardial tagging. Circulation (1990) 81(4):1236–44. doi:10.1161/01.CIR.81.4.1236
35. Behar V, Adam D, Lysyansky P, Friedman Z. The combined effect of nonlinear filtration and window size on the accuracy of tissue displacement estimation using detected echo signals. Ultrasonics (2004) 41(9):743–53. doi:10.1016/j.ultras.2003.09.003
36. Reisner SA. “Global longitudinal strain” – a novel index of left ventricular function. Circulation (2003) 108(17):659–659.
37. Leitman M, Lysyansky P, Sidenko S, Shir V, Peleg E, Binenbaum M, et al. Two-dimensional strain – a novel software for real-time quantitative echocardiographic assessment of myocardial function. J Am Soc Echocardiogr (2004) 17(10):1021–9. doi:10.1016/j.echo.2004.06.019
38. Weidemann F, Jamal F, Sutherland GR, Claus P, Kowalski M, Hatle L, et al. Myocardial function defined by strain rate and strain during alterations in inotropic states and heart rate. Am J Physiol Heart Circ Physiol (2002) 283(2):H792–9. doi:10.1152/ajpheart.00025.2002
39. Derumeaux G, Ovize M, Loufoua J, Pontier G, André-Fouet X, Cribier A. Assessment of nonuniformity of transmural myocardial velocities by color-coded tissue Doppler imaging: characterization of normal, ischemic, and stunned myocardium. Circulation (2000) 101(12):1390–5. doi:10.1161/01.CIR.101.12.1390
40. Firstenberg MS, Greenberg NL, Smedira NG, Castro P, Thomas JD, Garcia MJ. The effects of acute coronary occlusion on noninvasive echocardiographically derived systolic and diastolic myocardial strain rates. Curr Surg (2000) 57(5):466–72. doi:10.1016/S0149-7944(00)00296-8
41. Voigt J-U, Arnold MF, Karlsson M, Hübbert L, Kukulski T, Hatle L, et al. Assessment of regional longitudinal myocardial strain rate derived from Doppler myocardial imaging indexes in normal and infarcted myocardium. J Am Soc Echocardiogr (2000) 13(6):588–98. doi:10.1067/mje.2000.105631
42. Jamal F, Kukulski T, Strotmann J, Sutherland GR. Quantification of the spectrum of changes in regional myocardial function during acute ischemia in closed chest pigs: an ultrasonic strain rate and strain study. J Am Soc Echocardiogr (2001) 14(9):874–84. doi:10.1067/mje.2001.112037
43. Støylen A, Slørdahl S, Skjelvan GK, Heimdal A, Skjaerpe T. Strain rate imaging in normal and reduced diastolic function: comparison with pulsed Doppler tissue imaging of the mitral annulus. J Am Soc Echocardiogr (2001) 14(4):264–74. doi:10.1067/mje.2001.110375
44. Palka P, Lange A, Donnelly JE, Nihoyannopoulos P. Differentiation between restrictive cardiomyopathy and constrictive pericarditis by early diastolic Doppler myocardial velocity gradient at the posterior wall. Circulation (2000) 102(6):655–62. doi:10.1161/01.CIR.102.6.655
45. Afonso L, Kondur A, Simegn M, Niraj A, Hari P, Kaur R, et al. Two-dimensional strain profiles in patients with physiological and pathological hypertrophy and preserved left ventricular systolic function: a comparative analyses. BMJ Open (2012) 2(4):e001390. doi:10.1136/bmjopen-2012-001390
46. Paelinck BP, Lamb HJ, Bax JJ, Van der Wall EE, de Roos A. Assessment of diastolic function by cardiovascular magnetic resonance. Am Heart J (2002) 144(2):198–205. doi:10.1067/mhj.2002.123316
47. Moreo A, Ambrosio G, De Chiara B, Pu M, Tran T, Mauri F, et al. Influence of myocardial fibrosis on left ventricular diastolic function: noninvasive assessment by cardiac magnetic resonance and echo. Circ Cardiovasc Imaging (2009) 2(6):437–43. doi:10.1161/CIRCIMAGING.108.838367
48. Fischer SE, McKinnon GC, Scheidegger MB, Prins W, Meier D, Boesiger P. True myocardial motion tracking. Magn Reson Med (1994) 31(4):401–13. doi:10.1002/mrm.1910310409
49. van Heerebeek L, Borbély A, Niessen HW, Bronzwaer JG, van der Velden J, Stienen GJ, et al. Myocardial structure and function differ in systolic and diastolic heart failure. Circulation (2006) 113(16):1966–73. doi:10.1161/CIRCULATIONAHA.105.587519
50. Borbély A, Velden JVD, Papp Z, Bronzwaer JGF, Edes I, Stienen GJM, et al. Cardiomyocyte stiffness in diastolic heart failure. Circulation (2005) 111(6):774–81. doi:10.1161/01.CIR.0000155257.33485.6D
51. Katz A. New molecular mechanism in diastolic heart failure. Circulation (2006) 113(16):1922–5. doi:10.1161/CIRCULATIONAHA.106.620765
52. Danford DA, Huhta JC, Murphy DJ. Doppler echocardiographic approaches to ventricular diastolic function. Echocardiography (1986) 3(1):33–40. doi:10.1111/j.1540-8175.1986.tb00182.x
53. Sachdev MS, Bhagyavathy A, Varghese R, Coelho R, Kumar RS. Right ventricular diastolic function after repair of Tetralogy of Fallot. Pediatr Cardiol (2006) 27(2):250–5. doi:10.1007/s00246-005-1186-y
54. Ahmad N, Kantor PF, Grosse-Wortmann L, Seller N, Jaeggi ET, Friedberg MK, et al. Influence of RV restrictive physiology on LV diastolic function in children after Tetralogy of Fallot repair. J Am Soc Echocardiogr (2012) 25(8):866–73. doi:10.1016/j.echo.2012.05.011
55. Berg JVD, Wielopolski PA, Meijboom FJ, Witsenburg M, Bogers AJJC, Pattynama PMT, et al. Diastolic function in repaired Tetralogy of Fallot at rest and during stress: assessment with MR imaging. Radiology (2007) 243(1):212–9. doi:10.1148/radiol.2431060213
56. Helbing WA, Niezen RA, Le Cessie S, van der Geest RJ, Ottenkamp J, de Roos A. Right ventricular diastolic function in children with pulmonary regurgitation after repair of Tetralogy of Fallot: volumetric evaluation by magnetic resonance velocity mapping. J Am Coll Cardiol (1996) 28(7):1827–35. doi:10.1016/S0735-1097(96)00387-7
57. Lu JC, Cotts TB, Agarwal PP, Attili AK, Dorfman AL. Relation of right ventricular dilation, age of repair, and restrictive right ventricular physiology with patient-reported quality of life in adolescents and adults with repaired Tetralogy of Fallot. Am J Cardiol (2010) 106(12):1798–802. doi:10.1016/j.amjcard.2010.08.021
58. Gatzoulis MA, Clark AL, Cullen S, Newman CG, Redington AN, et al. Right ventricular diastolic function 15 to 35 years after repair of Tetralogy of Fallot. Restrictive physiology predicts superior exercise performance. Circulation (1995) 91(6):1775–81.
59. Vitarelli A, Conde Y, Cimino E, D’Angeli I, D’Orazio S, Ventriglia F, et al. Quantitative assessment of systolic and diastolic ventricular function with tissue Doppler imaging after Fontan type of operation. Int J Cardiol (2005) 102(1):61–9. doi:10.1016/j.ijcard.2004.04.008
60. Hershenson JA, Zaidi AN, Texter KM, Moiduddin N, Stefaniak CA, Hayes J, et al. Differences in tissue Doppler imaging between single ventricles after the Fontan operation and normal controls. Am J Cardiol (2010) 106(1):99–103. doi:10.1016/j.amjcard.2010.02.020
61. Tomkiewicz Pajak L. Single ventricle diastolic function and exercise capacity in patients after Fontan operation. Eur Heart J (2010) 31:121–2.
62. Diller GP, Giardini A, Dimopoulos K, Gargiulo G, Müller J, Derrick G, et al. Predictors of morbidity and mortality in contemporary Fontan patients: results from a multicenter study including cardiopulmonary exercise testing in 321 patients. Eur Heart J (2010) 31(24):3073–83. doi:10.1093/eurheartj/ehq356
63. Flamm MD, Cohn KE, Hancock EW. Ventricular function in atrial septal defect. Am J Med (1970) 48(3):286–94. doi:10.1016/0002-9343(70)90058-6
64. Vanoverschelde JJ, Essamri B, Vanbutsele R, d’Hondt A, Cosyns JR, Detry JR, et al. Contribution of left ventricular diastolic function to exercise capacity in normal subjects. J Appl Physiol (1993) 74(5):2225–33.
65. Giardini A, Moore P, Brook M, Stratton V, Tacy T. Effect of transcatheter atrial septal defect closure in children on left ventricular diastolic function. Am J Cardiol (2005) 95(10):1255–7. doi:10.1016/j.amjcard.2005.01.062
66. Friedman KG, McElhinney DB, Rhodes J, Powell AJ, Colan SD, Lock JE, et al. Left ventricular diastolic function in children and young adults with congenital aortic valve disease. Am J Cardiol (2013) 111(2):243–9. doi:10.1016/j.amjcard.2012.09.026
67. Milano AD, Faggian G, Dodonov M, Golia G, Tomezzoli A, Bortolotti U, et al. Prognostic value of myocardial fibrosis in patients with severe aortic valve stenosis. J Thorac Cardiovasc Surg (2012) 144(4):830–7. doi:10.1016/j.jtcvs.2011.11.024
68. Lancellotti P, Magne J, Donal E, O’Connor K, Dulgheru R, Rosca M, et al. Determinants and prognostic significance of exercise pulmonary hypertension in asymptomatic severe aortic stenosis. Circulation (2012) 126(7):851–9. doi:10.1161/CIRCULATIONAHA.111.088427
69. Weidemann F, Herrmann S, Störk S, Niemann M, Frantz S, Lange V, et al. Impact of myocardial fibrosis in patients with symptomatic severe aortic stenosis. Circulation (2009) 120(7):577–84. doi:10.1161/CIRCULATIONAHA.108.847772
70. Villari B, Hess OM, Kaufmann P, Krogmann ON, Grimm J, Krayenbuehl HP. Effect of aortic valve stenosis (pressure overload) and regurgitation (volume overload) on left ventricular systolic and diastolic function. Am J Cardiol (1992) 69(9):927–34. doi:10.1016/0002-9149(92)90795-Z
71. Villari B, Campbell SE, Hess OM, Mall G, Vassalli G, Weber KT, et al. Influence of collagen network on left ventricular systolic and diastolic function in aortic valve disease. J Am Coll Cardiol (1993) 22(5):1477–84. doi:10.1016/0735-1097(93)90560-N
72. Liu CP, Ting CT, Yang TM, Chen JW, Chang MS, Maughan WL, et al. Reduced left ventricular compliance in human mitral stenosis. Role of reversible internal constraint. Circulation (1992) 85(4):1447–56. doi:10.1161/01.CIR.85.4.1447
73. Corin W, Murakami T, Monrad ES, Hess OM, Krayenbuehl HP. Left ventricular passive diastolic properties in chronic mitral regurgitation. Circulation (1991) 83(3):797–807. doi:10.1161/01.CIR.83.3.797
74. Zile MR, Tomita M, Nakano K, Mirsky I, Usher B, Lindroth J, et al. Effects of left ventricular volume overload produced by mitral regurgitation on diastolic function. Am J Physiol (1991) 261(5):H1471–80.
75. Nagueh SF, Smiseth OA, Appleton CP, Byrd BF III, Dokainish H, Edvardsen T, et al. Recommendations for the evaluation of left ventricular diastolic function by echocardiography: an update from the American society of echocardiography and the European Association of Cardiovascular Imaging. J Am Soc Echocardiogr (2016) 29(4):277–314. doi:10.1016/j.echo.2016.01.011
76. Maciver DH, Townsend M. A novel mechanism of heart failure with normal ejection fraction. Heart (2007) 94(4):446–9. doi:10.1136/hrt.2006.114082
77. Diwan A, McCulloch M, Lawrie GM, Reardon MJ, Nagueh SF. Doppler estimation of left ventricular filling pressures in patients with mitral valve disease. Circulation (2005) 111(24):3281–9. doi:10.1161/CIRCULATIONAHA.104.508812
78. Lekavich CL, Barksdale DJ, Neelon V, Wu JR. Heart failure preserved ejection fraction (HFpEF): an integrated and strategic review. Heart Fail Rev (2015) 20(6):643–53. doi:10.1007/s10741-015-9506-7
79. Weber KT. Cardiac interstitium in health and disease: the fibrillar collagen network. J Am Coll Cardiol (1989) 13(7):1637–52. doi:10.1016/0735-1097(89)90360-4
80. Hill J, Olson EN. Cardiac plasticity. N Engl J Med (2008) 358(13):1370–80. doi:10.1056/NEJMra072139
81. Maciver DH, Dayer MJ. An alternative approach to understanding the pathophysiological mechanisms of chronic heart failure. Int J Cardiol (2012) 154(2):102–10. doi:10.1016/j.ijcard.2011.05.075
82. Wilson JR, Rayos G, Yeoh TK, Gothard P, Bak K. Dissociation between exertional symptoms and circulatory function in patients with heart failure. Circulation (1995) 92(1):47–53. doi:10.1161/01.CIR.92.1.47
83. Franciosa JA, Ziesche S, Mary Wilen RN. Functional capacity of patients with chronic left ventricular failure. Am J Med (1979) 67(3):460–6. doi:10.1016/0002-9343(79)90794-0
84. Elliott P, McKenna WJ. Hypertrophic cardiomyopathy. Lancet (2004) 363(9424):1881–91. doi:10.1016/S0140-6736(04)16358-7
85. Bonow RO, Dilsizian V, Rosing DR, Maron BJ, Bacharach SL, Green MV. Verapamil-induced improvement in left ventricular diastolic filling and increased exercise tolerance in patients with hypertrophic cardiomyopathy: short- and long-term effects. Circulation (1985) 72(4):853–64. doi:10.1161/01.CIR.72.4.853
86. Chikamori T, Dickie S, Poloniecki JD, Myers MJ, Lavender JP, McKenna WJ. Prognostic significance of radionuclide-assessed diastolic function in hypertrophic cardiomyopathy. Am J Cardiol (1990) 65(7):478–82. doi:10.1016/0002-9149(90)90814-H
87. Pak PH, Maughan L, Baughman KL, Kass DA. Marked discordance between dynamic and passive diastolic pressure-volume relations in idiopathic hypertrophic cardiomyopathy. Circulation (1996) 94(1):52–60. doi:10.1161/01.CIR.94.1.52
88. Kubo T, Gimeno JR, Bahl A, Steffensen U, Steffensen M, Osman E, et al. Prevalence, clinical significance, and genetic basis of hypertrophic cardiomyopathy with restrictive phenotype. J Am Coll Cardiol (2007) 49(25):2419–26. doi:10.1016/j.jacc.2007.02.061
89. Nagueh SF, Bachinski LL, Meyer D, Hill R, Zoghbi WA, Tam JW, et al. Tissue Doppler imaging consistently detects myocardial abnormalities in patients with hypertrophic cardiomyopathy and provides a novel means for an early diagnosis before and independently of hypertrophy. Circulation (2001) 104(2):128–30. doi:10.1161/01.CIR.104.2.128
90. Poutanen T, Tikanoja T, Jääskeläinen P, Jokinen E, Silvast A, Laakso M, et al. Diastolic dysfunction without left ventricular hypertrophy is an early finding in children with hypertrophic cardiomyopathy-causing mutations in the beta-myosin heavy chain, alpha-tropomyosin, and myosin-binding protein C genes. Am Heart J (2006) 151(3):725.e1–725.e9. doi:10.1016/j.ahj.2005.12.005
Keywords: diastolic function, congenital heart disease, echocardiography, CMR, diastolic heart failure
Citation: Panesar D and Burch M (2017) Assessment of Diastolic Function in Congenital Heart Disease. Front. Cardiovasc. Med. 4:5. doi: 10.3389/fcvm.2017.00005
Received: 28 October 2016; Accepted: 23 January 2017;
Published: 15 February 2017
Edited by:
Giovanni Biglino, University of Bristol, UKReviewed by:
Yasuhiro Fujii, Okayama University, JapanMeena Nathan, Boston Children’s Hospital, USA
Copyright: © 2017 Panesar and Burch. This is an open-access article distributed under the terms of the Creative Commons Attribution License (CC BY). The use, distribution or reproduction in other forums is permitted, provided the original author(s) or licensor are credited and that the original publication in this journal is cited, in accordance with accepted academic practice. No use, distribution or reproduction is permitted which does not comply with these terms.
*Correspondence: Dilveer Kaur Panesar, ZGlsdmVlci5wYW5lc2FyJiN4MDAwNDA7dWNsLmFjLnVr