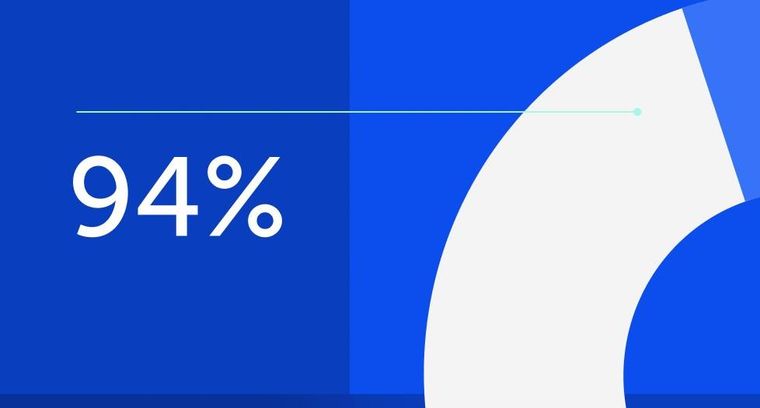
94% of researchers rate our articles as excellent or good
Learn more about the work of our research integrity team to safeguard the quality of each article we publish.
Find out more
GENERAL COMMENTARY article
Front. Cardiovasc. Med., 26 May 2016
Sec. General Cardiovascular Medicine
Volume 3 - 2016 | https://doi.org/10.3389/fcvm.2016.00015
A commentary on
Sulfur dioxide contributes to the cardiac and mitochondrial dysfunction in rats
A commentary on Sulfur dioxide contributes to the cardiac and mitochondrial dysfunction in rats by Qin G, Meiqiong W, Wang J, Xu Z, Jin X, Sang N. Toxicol Sci (2016). doi: 10.1093/toxsci/kfw048
The article by Qin et al. showed that sulfur dioxide contributes in the impairment of cardiac and mitochondrial function in rat H9C2 transformed cardiomyoblasts (1). The authors investigated the effect of 10–100 μM sodium bisulfite (NaHSO3) on the mitochondrial function of H2C9 cells and rat primary cardiomyocytes and showed that COX activities, Δψm, and ATP content were decreased by sulfite (1).
This evidence, though restricted to an in vitro cell model, should raise a fundamental concern about sulfite preservatives used in wine industry or food and the consequent role of grapes-derived flavonoids in human health. Actually, one of the main sources of sulfites in the human body comes from the addition of sulfites and sulfur dioxide to many food products. The pejorative effect of sulfite preservatives may dampen the beneficial action of wine polyphenols.
Sulfur dioxide dramatically alters grapes transcriptome, leading to mitochondria impairment and downregulation of redox homeostasis (2). Therefore, the presence of sulfur dioxide in plant sources represents an important issue for toxicology, besides any further concern about environmental pollutants. Yet, sulfur dioxide in many conventional, purchasable red wines might not alter necessarily the yield of flavonoids, such as resveratrol, when compared to sulfite-deprived organic wines (3); therefore, its presence or removal should be conventionally reappraised and related to the role than sulfites may have on human health. Yet, toxicology of this food preservative shows many controversial questions in medicine. Although it was considered as an important risk factor for the initiation and progression of liver diseases, based on its proapoptotic and oxidative stressing potential, molecular research on the apoptotic effect of sub-toxicological doses of sulfur dioxide did not appear to fulfill any good expectation, as a controversial debate may occur (4). Figure 1 shows how sulfur dioxide, sulfites, and S-sulfonation of proteins may have different effects on mitochondria and redox signaling. Yet, severe liver injury and necrotic foci were described with the exposure of sulfur dioxide between 56 and 168 mg/m3, i.e., within the range 20–60 ppm (5), though far away from the value of 1.5–5.0 ppm, reported by Qin et al. (1). As the same authors addressed in their article, plasma level of sulfites closely depends on dietary habits and the composition of human gut microbiome. Particularly in Western populations, a dietary regimen made by the presence of saturated fatty acids, refined sugars, and low fibers, resembling an experimental milk-derived diet in mice, promotes the expansion of sulfite-reducing bacteria, typically Clostridia and other anaerobic species such as Deltaproteobacteria, though the profound change in gut microbiome, with increased Bacteroides, decreased Firmicutes and a marked change in biliary salt chemistry (6). On the other hand, whey retentate, which may come from milk and fermented dairy products (7), promotes the growth of symbiontic Bifidobacteria and reduces Bacteroides fragilis and sulfite-reducing Clostridia (8). Gut microbiome is a formidable barrier for the prevention of sulfur dioxide entering into the plasma and therefore to reach the many target organs, such as myocardial muscle. Furthermore, human plasma levels of [SO2]=, often in the form of protein-linked S-sulfonate, seem to be better correlated with sulfur dioxide in the environmental air, for which an increment of about 1.1 nmol of plasma S-sulfonates for any ppm of sulfur dioxide, has been reported (9). This would mean that, besides the clearance activity by human sulfite oxidase (10), sulfur dioxide able to impair cardiovascular function and mitochondrial activity should come quite exclusively from an inner SO2 saturated microenvironment, such as in industrial contexts, despite the considerations that most of sulfur would come from diet. Table 1 summarizes some sulfur effects. Although the paper by Qin et al. used an in vitro model, their conclusion about human health may be quite premature.
Figure 1. Cartoon describing some fundamental examples of the activity exerted by sulfur (in the form of sulfur dioxide, sulfites, or S-proteins) on mitochondria and redox signaling. (A) Sulfites and sulfur dioxide are able to inhibit the isopropylarterenol-induced apoptosis and mitochondria dysfunction, so exerting a protective potential on myocardial activity (11); (B) the same sulfur-possessing molecules may impair mitochondria permeability and their ability in producing ATP, then causing myocardial dysfunction; (C) sulfite ion impairments of mitochondrial function might be explained even with thioredoxin inactivation (12). A mechanistic possibility to explain sulfite ion impairment of mitochondrial function is the evidence that these ions can inactivate thioredoxin (12) and knowing that the thioredoxin 2 system is a major controller of H2O2 emission from mitochondria (13), sulfite ions can contribute to increased ROS emission from mitochondria that in turn may jeopardize cardiac function; (D) S-sulfonylated proteins, containing S-aminoacids, may be source of reactive sulfur species, such as cysteine hydropersulfides, which are involved in the metabolism of 8-nitroguanosine 3′,5′-cyclic monophosphate. This molecule belongs to the endogenously formed electrophiles, which are important signaling molecules in the ROS and RNS scavenging and regulation (14).
The authors used a concentration, expressed into micromoles of sodium bisulfite, just approaching the “usual” maximal concentration (≈100 μM) coming from daily intake of food and air pollution, yet the readers are not allowed to comprehend if this apparently “normal” assumption of sulfites may lead to a relevant risk of a cardiovascular damage.
The question is which is the possible meaning of the reported evidence for human health?
Actually, several organic compounds besides sulfites, such as thiosulfates and S-sulfocysteine, also coming from biological degradation, may exert a damaging action on mitochondrial permeability transition, mitochondria biogenesis, and energy homeostasis (24). Sulfites themselves then lead to degenerative disorders, e.g., by inhibiting glutamate dehydrogenase in brain (25) or causing impairment in kidney functionality (26). The observed increase in oxidative stress may derive from an impairment in ROS scavenging system, as it is well-known that glutathione S-sulfonate, generated from the reaction between glutathione disulfide and sulfite, is a competitive inhibitor of glutathione-S-transferase (GST), leading to a marked defect in the detoxification of xenobiotics. In this context, sulfur is strategic in mitochondria-dependent response to oxidative stress, when mitochondrial GST can be activated by direct S-thiolation, while thiol disulfide exchange depends on the correct glutathione redox state. This consideration may suggest that xenobiotics introduced with the diet play a major role, probably much more fundamental than gaseous SO2, in regulating mitochondria participation in the homeostasis of oxidative stressors, probably with the involvement of sulfonated-proteins. Actually, an excess of reactive species, also from NO and nitrogen, usually coming from an impairment in the detoxifying machinery, may lead to peroxynitrite-mediated disorder of mitochondrial complex II and peroxynitrite-mediated S-sulfonation, exacerbating the risk of myocardial infarction (27). The paper by Qin et al. showed a marked inhibition of any mitochondrial function by sulfite, probably depending on a different contacting area with cell macromolecules by SO2 in the anionic and non-gaseous form.
S-sulfonation is a process by which sulfite interacts with proteins; therefore, it may be a very common mechanism in the biochemistry of human body, possibly causing pathologies, e.g., S-sulfonation of transthyretin has been reported as a trigger step in the formation of amyloid fibrils (28). Protein sulfonation, if evaluated, may give important further insights.
From a toxicological point of view, dietary intake and correct information of gut microbiome should be dealt with the same emphasis of air pollutants, when addressing plant xenobiotics containing sulfur and sulfites present in daily food as preservatives.
SC planned, conceived, wrote, and submitted the manuscript; GB prepared the cartoon, searched new references, checked the manuscript, and discussed some important recent points.
The authors declare that the research was conducted in the absence of any commercial or financial relationships that could be construed as a potential conflict of interest.
A special acknowledgment to Samuel R. Weisller (University of North Carolina, Chapel Hill, NC, USA) for his precious information about sulfur in mitochondrial permeability.
1. Qin G, Meiqiong W, Wang J, Xu Z, Jin X, Sang N. Sulfur dioxide contributes to the cardiac and mitochondrial dysfunction in rats. Toxicol Sci (2016). doi:10.1093/toxsci/kfw048
2. Giraud E, Ivanova A, Gordon CS, Whelan J, Considine MJ. Sulphur dioxide evokes a large scale reprogramming of the grape berry transcriptome associated with oxidative signalling and biotic defence responses. Plant Cell Environ (2012) 35(2):405–17. doi:10.1111/j.1365-3040.2011.02379.x
3. Garaguso I, Nardini M. Polyphenols content, phenolics profile and antioxidant activity of organic red wines produced without sulfur dioxide/sulfites addition in comparison to conventional red wines. Food Chem (2015) 179:336–42. doi:10.1016/j.foodchem.2015.01.144
4. Bai J, Lei P, Zhang J, Zhao C, Liang R. Sulfite exposure-induced hepatocyte death is not associated with alterations in p53 protein expression. Toxicology (2013) 312:142–8. doi:10.1016/j.tox.2013.08.004
5. Bai JY, Meng ZQ. Sulfur dioxide-induced liver pathology. Zhonghua Bing Li Xue Za Zhi (2004) 33(2):155–7. doi:10.3760/j.issn:0529-5807.2004.02.015
6. Devkota S, Chang EB. Interactions between diet, bile acid metabolism, gut microbiota, and inflammatory bowel diseases. Dig Dis (2015) 33(3):351–6. doi:10.1159/000371687
7. Ebringer L, Ferencík M, Krajcovic J. Beneficial health effects of milk and fermented dairy products – review. Folia Microbiol (Praha) (2008) 53(5):378–94. doi:10.1007/s12223-008-0059-1
8. Meddah AT, Yazourh A, Desmet I, Risbourg B, Verstraete W, Romond MB. The regulatory effects of whey retentate from bifidobacteria fermented milk on the microbiota of the Simulator of the Human Intestinal Microbial Ecosystem (SHIME). J Appl Microbiol (2001) 91(6):1110–7. doi:10.1046/j.1365-2672.2001.01482.x
9. Gunnison AF, Palmes ED. S-sulfonates in human plasma following inhalation of sulfur dioxide. Am Ind Hyg Assoc J (1974) 35(5):288–91. doi:10.1080/0002889748507036
10. Gunnison AE, Jacobsen DW. Sulfite hypersensitivity. A critical review. CRC Crit Rev Toxicol (1987) 17:185–214. doi:10.3109/10408448709071208
11. Jin H, Liu AD, Holmberg L, Zhao M, Chen S, Yang J, et al. The role of sulfur dioxide in the regulation of mitochondrion-related cardiomyocyte apoptosis in rats with isopropylarterenol-induced myocardial injury. Int J Mol Sci (2013) 14(5):10465–82. doi:10.3390/ijms140510465
12. Würfel M, Häberlein I, Follmann H. Inactivation of thioredoxin by sulfite ions. FEBS Lett (1990) 268(1):146–8. doi:10.1016/0014-5793(90)80994-T
13. Stanley BA, Sivakumaran V, Shi S, McDonald I, Lloyd D, Watson WH, et al. Thioredoxin reductase-2 is essential for keeping low levels of H(2)O(2) emission from isolated heart mitochondria. J Biol Chem (2011) 286(38):33669–77. doi:10.1074/jbc.M111.284612
14. Nishida M, Kumagai Y, Ihara H, Fujii S, Motohashi H, Akaike T. Redox signaling regulated by electrophiles and reactive sulfur species. J Clin Biochem Nutr (2016) 58(2):91–8. doi:10.3164/jcbn.15-111
15. Pishgoo B, Ghanei M, Harandi AA, Farahani MM, Daadjoo Y. Long term cardiac abnormality after single high dose exposure to sulfur mustard? Indian Heart J (2007) 59(2):181–4.
16. Zaky A, Ahmad A, Dell’Italia LJ, Jahromi L, Reisenberg LA, Matalon S, et al. Inhaled matters of the heart. Cardiovasc Regen Med (2015) 2:e997. doi:10.14800/crm.997
17. Zhang S, Du J, Jin H, Li W, Liang Y, Geng B, et al. Endogenous sulfur dioxide aggravates myocardial injury in isolated rat heart with ischemia and reperfusion. Transplantation (2009) 87(4):517–24. doi:10.1097/TP.0b013e318195fe82
18. Huang Y, Tang C, Du J, Jin H. Endogenous sulfur dioxide: a new member of gasotransmitter family in the cardiovascular system. Oxid Med Cell Longev (2016) 2016:8961951. doi:10.1155/2016/8961951
19. Shohrati M, Ghanei M, Shamspour N, Babaei F, Abadi MN, Jafari M, et al. Glutathione and malondialdehyde levels in late pulmonary complications of sulfur mustard intoxication. Lung (2010) 188(1):77–83. doi:10.1007/s00408-009-9178-y
20. Velayutham M, Hemann CF, Cardounel AJ, Zweier JL. Sulfite oxidase activity of cytochrome c: role of hydrogen peroxide. Biochem Biophys Rep (2016) 5:96–104. doi:10.1016/j.bbrep.2015.11.025
21. Noorafshan A, Asadi-Golshan R, Monjezi S, Karbalay-Doust S. Sodium metabisulphite, a preservative agent, decreases the heart capillary volume and length, and curcumin, the main component of Curcuma longa, cannot protect it. Folia Biol (Praha) (2014) 60(6):275–80.
22. Wang J, Krizowski S, Fischer-Schrader K, Niks D, Tejero J, Sparacino-Watkins C, et al. Sulfite oxidase catalyzes single-electron transfer at molybdenum domain to reduce nitrite to nitric oxide. Antioxid Redox Signal (2015) 23(4):283–94. doi:10.1089/ars.2013.5397
23. Wei H, Meng Z. Epigallocatechin-3-gallate protects Na+ channels in rat ventricular myocytes against sulfite. Cardiovasc Toxicol (2010) 10(3):166–73. doi:10.1007/s12012-010-9075-x
24. Grings M, Moura AP, Amaral AU, Parmeggiani B, Gasparotto J, Moreira JC, et al. Sulfite disrupts brain mitochondrial energy homeostasis and induces mitochondrial permeability transition pore opening via thiol group modification. Biochim Biophys Acta (2014) 1842(9):1413–22. doi:10.1016/j.bbadis.2014.04.022
25. Zhang X, Vincent AS, Halliwell B, Wong KP. A mechanism of sulfite neurotoxicity: direct inhibition of glutamate dehydrogenase. J Biol Chem (2004) 279(41):43035–45. doi:10.1074/jbc.M402759200
26. Vincent AS, Lim BG, Tan J, Whiteman M, Cheung NS, Halliwell B, et al. Sulfite-mediated oxidative stress in kidney cells. Kidney Int (2004) 65(2):393–402. doi:10.1111/j.1523-1755.2004.00391.x
27. Zhang L, Chen CL, Kang PT, Garg V, Hu K, Green-Church KB, et al. Peroxynitrite-mediated oxidative modifications of complex II: relevance in myocardial infarction. Biochemistry (2010) 49(11):2529–39. doi:10.1021/bi9018237
Keywords: mitochondria, heart, sulfur, sulfonated aromatic polymer, sulfonated protein, heart failure
Citation: Chirumbolo S and Bjørklund G (2016) Commentary: Sulfur Dioxide Contributes to the Cardiac and Mitochondrial Dysfunction in Rats. Front. Cardiovasc. Med. 3:15. doi: 10.3389/fcvm.2016.00015
Received: 20 April 2016; Accepted: 13 May 2016;
Published: 26 May 2016
Edited by:
Pietro Enea Lazzerini, University of Siena, ItalyReviewed by:
Nazareno Paolocci, Johns Hopkins University, USACopyright: © 2016 Chirumbolo and Bjørklund. This is an open-access article distributed under the terms of the Creative Commons Attribution License (CC BY). The use, distribution or reproduction in other forums is permitted, provided the original author(s) or licensor are credited and that the original publication in this journal is cited, in accordance with accepted academic practice. No use, distribution or reproduction is permitted which does not comply with these terms.
*Correspondence: Salvatore Chirumbolo, c2FsdmF0b3JlLmNoaXJ1bWJvbG9AdW5pdnIuaXQ=
Disclaimer: All claims expressed in this article are solely those of the authors and do not necessarily represent those of their affiliated organizations, or those of the publisher, the editors and the reviewers. Any product that may be evaluated in this article or claim that may be made by its manufacturer is not guaranteed or endorsed by the publisher.
Research integrity at Frontiers
Learn more about the work of our research integrity team to safeguard the quality of each article we publish.