- 1Department of Chemistry and Biochemistry and the Centre for NanoScience Research, Concordia University, Montreal, QC, Canada
- 2Quebec Centre for Advanced Materials, Department of Chemistry and Biochemistry, Concordia University, Montreal, QC, Canada
Carbon-based nanomaterials have drawn significant interest as desirable nanomaterials and composites for the adsorptive removal of various classes of pollutants from water owing to their versatile physicochemical properties. The underlying sorption mechanisms serve as the bedrock for the development of carbonaceous adsorbents for various target pollutants. Microwave-assisted synthesis can be regarded as a recent and well-advanced technique for the development of carbon-based nanomaterials, and the use of biobased materials/wastes/residues conforms with the concept of green and sustainable chemistry. For advancements in carbon-based functional nanomaterials and their industrial/field applications, it is essential to fully comprehend the sorption performance and the selective/non-selective interaction processes between the contaminants and sorbents. In this regard, research on the development of carbon-based nanomaterials for the adsorption of chemical contaminants, both organic and inorganic, in water has made considerable strides as discussed in this review. However, there are still several fundamental hurdles associated with microwave-assisted chemical synthesis and commercial/industrial scale-up applications in nano-remediation. The challenges, benefits, and prospects for further research and development of carbon-based nanomaterials/nanocomposites for the purification of water are also discussed.
1 Introduction
Over 20 years ago, the concept of “green chemistry” emerged as a result of a shift in emphasis on material research with the move towards the use of waste materials and waste-reduction strategies. The chemical industry as a whole and scientists are directed to follow and promote sustainable and environmentally friendly pathways governed by the twelve principles of green chemistry (DeVierno Kreuder et al., 2017). The seventh principle buttresses the need for designing synthetic procedures with minimal negative impacts on the environment and without the use of hazardous reagents (Kharissova et al., 2019; Forbes, 2021). Thus, using renewable feedstocks and biodegradable raw or waste materials that are typically rich in carbon, and are available from natural or biological sources, is a more acceptable approach for the environmentally friendly preparation of carbon-based materials from the bulk to the nanoscale.
The synthesis of carbon-based nanomaterials has been facilitated by enabling technologies such as hydrothermal, solvothermal, chemical vapor deposition, microwave-assisted synthesis, and other top-down/bottom-up techniques (Yan et al., 2016; Macairan et al., 2019; Adeola and Forbes, 2021a; Gulati et al., 2023). In comparison to other conventional methods, microwave-assisted synthesis of carbon-based nanomaterials is a non-invasive, easy, quick, clean, and eco-friendly process (Oladipo et al., 2018; Dutta et al., 2022a). The reaction is facilitated and accelerated by the microwave, frequently increasing relative yields. According to reports, microwave-assisted synthesis can circumvent synthesis-related challenges through energy efficiency, simplicity of experimental setup, adjustable process conditions, speedy reactions, and uniform heating/thermal processing. Microwave-assisted synthesis of carbon-based nanomaterials requires low growth temperatures and offers high surface areas, adsorption capacities, and purity while providing energy savings. As such, it presents a promising approach to addressing the challenges for industrial and municipal water treatment (Schwenke et al., 2015; Baghel et al., 2022).
Environmental pollution is unquestionably one of the major challenges facing civilization today. Every day, thousands of tons of dangerous chemicals are released into water bodies (Nassar et al., 2015; Dutta et al., 2019; Tong et al., 2022). Some dangerous contaminants that can be found in the aquatic environment include heavy metals, textile dyes, herbicides, surfactants, microplastics, hydrocarbons, pharmaceuticals, and personal care products, to name a few. To get rid of the contaminants in polluted water, new methods are constantly being researched. While developing carbon-based nanomaterials for environmental remediation, some major factors for consideration include target-specific identification, simplicity of design, affordable production, the toxicity of nanomaterial, biocompatibility, reusability, and the capacity for regeneration after usage. Therefore, new technologies are particularly intriguing because they may hold the key to addressing these challenges. As a result, numerous studies have concentrated on applying green chemistry concepts with the physicochemical surface modification of carbon-based nanomaterials to produce nanomaterials that can mitigate challenges faced during contaminant cleanup (Das et al., 2020; Dutta et al., 2022b; Mohapatra et al., 2023; Osterberg et al., 2023).
Scopus search revealed that over 62% of published data on carbon-based materials in the last decade have focused on design and application in water treatment, and well over 10,000 articles have been published on the subject (Dutta et al., 2022a). Thus, there is a need to constantly review the advances in this field of research, particularly the recent integration of microwave-assisted synthesis in carbon nanomaterials development. The current review assesses the development of advanced carbon nanomaterials (graphene, carbon nanotubes, and carbon dots) for water pollution remediation using microwave-assisted synthesis. The significant aspect of the review includes green nano-synthesis from waste/biobased resources using the microwave method and applications in water treatment, highlighting the merits, challenges, and future prospects.
2 Conventional synthesis techniques of carbon-based materials from biobased resources
Carbon-based nanomaterials have become an essential component of modern technology, with applications in fields such as electronics, environment, catalysis, energy storage, and drug delivery due to their unique qualities, such as high surface area, exceptional mechanical strength, and electrical conductivity (Fathy et al., 2020; Zakaria et al., 2022). Numerous methods are utilized to synthesize these nanomaterials, which can be classified into top-down and bottom-up approaches. The top-down approach consists in breaking down large carbon structures such as graphite, into smaller carbon nanostructures, while in the bottom-up approach, the carbon nanostructures are formed from atoms and molecules (Supplementary Figure S1–S3) (Baig et al., 2021; Abid et al., 2022). Depending on the nature of the precursor and process conditions, some conventional and microwave-assisted techniques can carry out bottom-up/top-down synthesis of carbon nanomaterials.
Arc discharge and laser ablation are among the most used top-down approaches for the synthesis of carbon nanomaterials. These were the earliest methods used for synthesizing fullerenes and carbon nanotubes (CNTs) (Kroto et al., 1985; Iijima, 1991; Arora and Sharma, 2014) In the arc discharge method, a high-voltage electric current is applied between two graphite electrodes in an inert environment/system. An electric arc is formed, generating a high temperature that is responsible for vaporizing the graphite electrodes. Then, the vapor condenses into CNTs or fullerenes (Arora and Sharma, 2014; Deng et al., 2016). On the other hand, the laser ablation technique utilizes a high-power laser to vaporize graphite under an inert atmosphere. The vapor is then carried by the inert gas and condensed into carbon nanomaterials (Deng et al., 2016; Aravind Kumar et al., 2020). Nonetheless, these techniques show several drawbacks including low yield for laser ablation, presence of impurities such as amorphous carbon and residual catalyst, limited control over size and shape, requirement of high temperatures, and consequently high energy consumption, in addition to costly setups. Thus, bottom-up approaches, including chemical vapor deposition (CVD), hydro/solvothermal, and microwave-assisted techniques are considered more promising for the production of carbon nanomaterials on a large scale due to their low cost, high control over size and shape as well as high material yields (Saputri et al., 2020; El-Khawaga et al., 2023).
CVD has been one of the conventional methods most explored for synthesizing different carbon nanomaterials owing to its simplicity and ability to produce high-quality materials (Wang et al., 2018; Fathy et al., 2020; Zakaria et al., 2022). It consists of the thermal decomposition of a carbon source under an inert atmosphere at a high temperature, in the presence of a metallic catalyst. As the carbon source decomposes, the carbon atoms deposit on the catalyst surface, and due to its low solubility in these metallic particles at high temperatures, precipitates are obtained and then carbon nanomaterials are formed (Deng et al., 2016). Although this conventional method is indeed capable of producing high-quality carbon-based nanomaterials, it relies mainly on carbon sources derived from fossil fuels for synthesizing these materials, such as ethylene, acetylene, benzene, methane, ethane, toluene, and xylene (Goswami et al., 2021; Shi et al., 2023a). In this regard, there has been a rise in interest in a more sustainable and greener synthesis of carbon nanomaterials that focuses on the development of environmentally friendly and sustainable alternatives (Deng et al., 2016; Vivekanandhan et al., 2017; Fathy et al., 2020; Shi et al., 2023a). These alternatives focus on the use of non-toxic, renewable, biodegradable, and abundant precursors, such as honey (Mandani et al., 2017) and juices (Wang et al., 2022), biomass (Zhao et al., 2020), vegetable and animal oils (Duarte et al., 2022), industrial residues (Hu et al., 2019), and agricultural waste (Somanathan et al., 2015). Supplementary Table S1 provides a summary of biobased resources that have been utilized in the synthesis of carbon nanomaterials through conventional techniques.
Owing to its high yield, high purity, and the possibility of morphological and structural control, researchers have focused on developing a more sustainable CVD process. Thus, greener solvents and more environmentally friendly solid carbon sources have been employed as precursors to the synthesis of these nanomaterials (Manawi et al., 2018; Fathy et al., 2020). However, the evolution of toxic gaseous by-products during CVD remains a drawback (Manawi et al., 2018; Ijaz et al., 2020). In this way, pyrolysis, hydro/solvothermal and microwave-assisted techniques have attracted attention due to the non-necessity of the presence of a substrate for the growth of the nanomaterial, in addition to the possibility of using different carbon sources derived from biomass sources, renewable materials, and wastes. As a result, these approaches have been widely utilized in many parts of the world, including developing countries (Wang et al., 2018; Wang et al., 2020a; Devi et al., 2021).
The pyrolysis method consists of the thermal decomposition of a carbon source at a high temperature under an inert atmosphere and has been used extensively for synthesizing carbon dots (CDs) and graphene-based materials (Devi et al., 2021; Asif and Saha, 2023). In the hydro/solvothermal methods, the carbon source is converted into nanostructured carbon materials under high pressure and mild temperatures (<350°C) in a sealed vessel (Byrappa and Adschiri, 2007; Heidari et al., 2019; Gong et al., 2023). In the hydrothermal method, the reaction occurs in an aqueous medium, while in the solvothermal approach, different solvents such as ethanol, acetone, and DMF, are used as the reaction medium. Both methods can produce carbon-based nanomaterials with controlled size and morphology, depending on the synthesis parameters, such as temperature, time, and concentration of the carbon source and solvent (Wang et al., 2020a; Huo et al., 2023).
These methods with the inclusion of microwave technology have proven to be excellent to obtain carbon nanomaterials from renewable and natural sources and residues, rendering this process more sustainable, green, and economically viable. In addition, they provide an alternative to recycling and reusing some residues, not only transforming them into value-added products but also addressing some environmental issues associated with the disposal of these materials. More information about various top-down and bottom-up synthesis approaches for carbon-based nanomaterials, including their merits and demerits are available in recent reviews by Abid et al. (Abid et al., 2022), El-Khawaga et al. (El-Khawaga et al., 2023), Gong et al. (Gong et al., 2023) and Huo et al. (Huo et al., 2023). According to these reports, these methods present some limitations such as the requirements for high temperatures and pressures, long reaction times, and difficulty in measuring, understanding, and controlling the synthesis/growth process of the nanomaterials (Abid et al., 2022; Huo et al., 2023). Hence, researchers have sought the development of microwave-assisted synthesis methods to address these shortcomings while achieving faster results through better-controlled reactions and product formation (Omoriyekomwan et al., 2019; Omoriyekomwan et al., 2021; Vignesh et al., 2022).
3 Microwave irradiation
Gedye et al., in 1986, and Mingos and Baghurst in 1991, reported an unprecedentedly quick organic reaction under microwave irradiation (Gedye et al., 1986; Mingos and Baghurst, 1991). These were two of the earliest reports on the use of microwaves in chemical synthesis. Prior to these reports, Randall and Booth invented the magnetron, a device that generated fixed microwave frequencies, ushering in the era of microwave-assisted chemical processes (Cole and Cole, 1941; Kaul, 1989). As soon as scientists realized the magnetron’s amazing capacity for rapidly heating water, they began to investigate the mechanism at play, and subsequently, its potential for domestic, laboratory, and commercial applications (de Medeiros et al., 2019; Singh et al., 2019).
Domestic microwaves were used during the development phase of microwave-assisted chemical synthesis in research laboratories and unfortunately are still in use in developing countries across the globe (Monte-Filho et al., 2019). Although advanced microwave reactors currently exist (Figure 1), the use of more affordable kitchen microwaves for research has not been completely phased out. Some prestigious publishers reject manuscripts that include household ovens as heating sources. The reason why more advanced microwave equipment has been developed includes safety features offered by modern microwave reactors for chemical synthesis. Furthermore, the main benefit of using microwaves for chemical synthesis is superheating in an air-tight reaction vessel that can withstand high temperatures and pressures, since the Arrhenius rule allows for significantly faster reaction times.
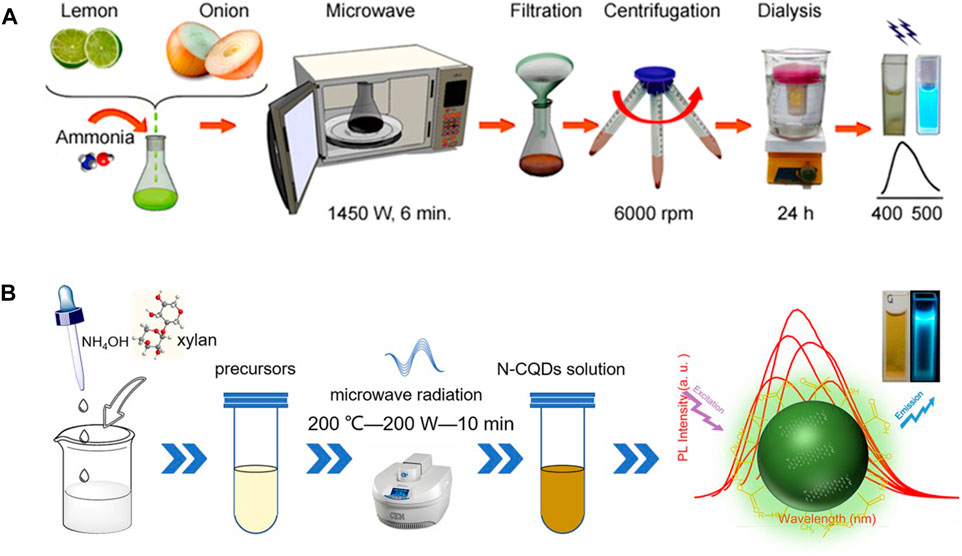
FIGURE 1. CDs synthesis using domestic microwave (A) and commercial microwave (B) with biobased materials (Yang et al., 2018a; Monte-Filho et al., 2019). Open access.
Advanced microwave reactors function as highly practical autoclaves that can swiftly and effectively heat reaction mixtures to 300°C and 80 bar while allowing the reaction medium to stay sealed throughout the duration of the reaction. In contrast, domestic microwave ovens do not offer reaction parameter control and it is almost impossible to monitor the temperature and pressure of the reaction in real-time, with IR sensors for reaction temperature control, pressure sensors to keep track of the reaction pressure in the closed vessels, and a magnetic stirrer to facilitate agitation (Figure 2). Electromagnetic radiation between 0.3 and 300 GHz is referred to as microwaves. To prevent interference with telecommunications equipment, most typical microwave equipment emits electromagnetic radiation at 2.45 GHz.
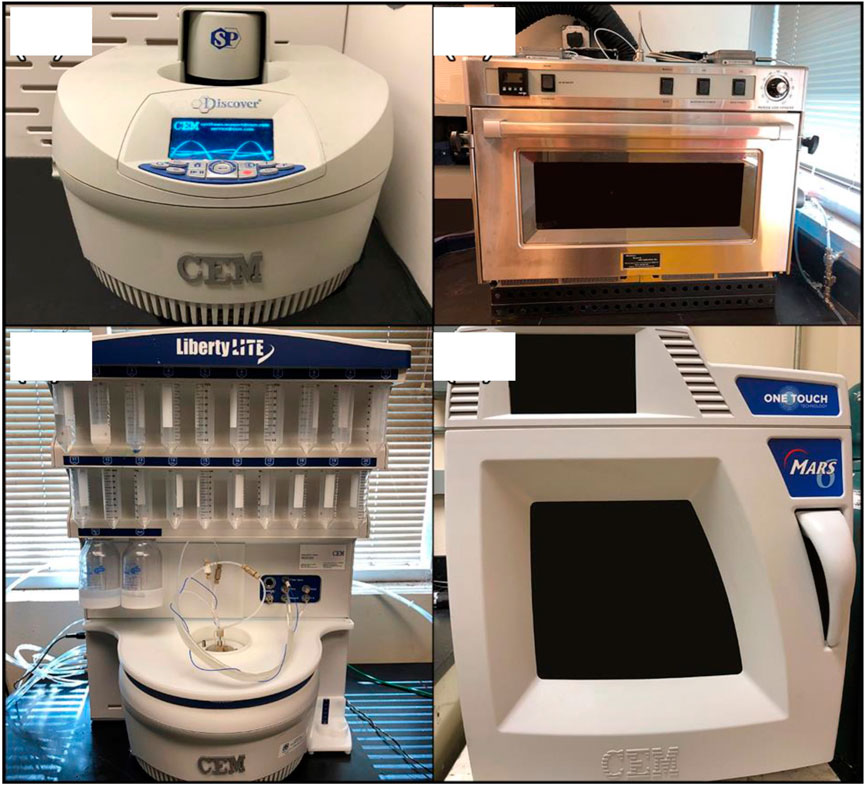
FIGURE 2. Microwave reactors with cutting-edge design features include the following: CEM Discover SP with a single-mode cavity (top left). Microwave Research and Applications BP-210 processing MW with reaction temperatures up to 1800°C (top right). CEM Liberty Blue with a critical reagent delivery system (bottom left). CEM MARS6 with a high throughput capacity and a fiber-optic temperature sensor (Bottom right). Adapted from (Chin et al., 2021). Open access.
The type of material being heated by microwaves and the amount of interaction between the sample and the radiation are key determining factors in how a reaction proceeds since conductors, dielectric materials, and insulators all have different characteristics. Following exposure of a material to microwave radiation, three different types of mechanisms have been described in the literature (Mishra and Sharma, 2016; Kahraman et al., 2020), namely reflection, absorption, and transmittance. Metals and other electrical conductors act as microwave radiation reflectors (Rafael Zamorano et al., 2019) while PTFE and quartz are two substances that permit microwave radiation transmission. Because insulators do not absorb microwave radiation, they are unable to be heated via this technology (Motasemi and Ani, 2012). In what concerns the reaction medium, or the solvent, for these reactions, polar liquids are excellent at absorbing microwaves. As such, they heat up quickly following microwave irradiation because of dipolar interactions and ionic conduction (Hu et al., 2021).
Polar molecules’ capacity to absorb microwave radiation and transform it into heat through a process known as dielectric heating is the key to the effectiveness and success of microwave-assisted chemical reactions (de Medeiros et al., 2019). When exposed to microwave radiation, polar molecules with electrical dipole moments rotate continuously in an effort to align themselves with the electric field. This phenomenon is caused by dielectric polarization and conduction loss. Through dielectric loss and molecular friction, the molecules reverse direction when the field alternates, thereby generating heat. The loss factor (tan δ) is a measure of a solvent’s capacity to transform electromagnetic energy from microwaves into heat. According to Table 1, solvents are frequently categorized as strong, medium, or weak microwave absorbers. A solvent’s microwave heating process is quicker and more effective with a higher value of tan δ. A low tan δ value, on the other hand, suggests ineffective and slow heating operation under microwave irradiation (de Medeiros et al., 2019).
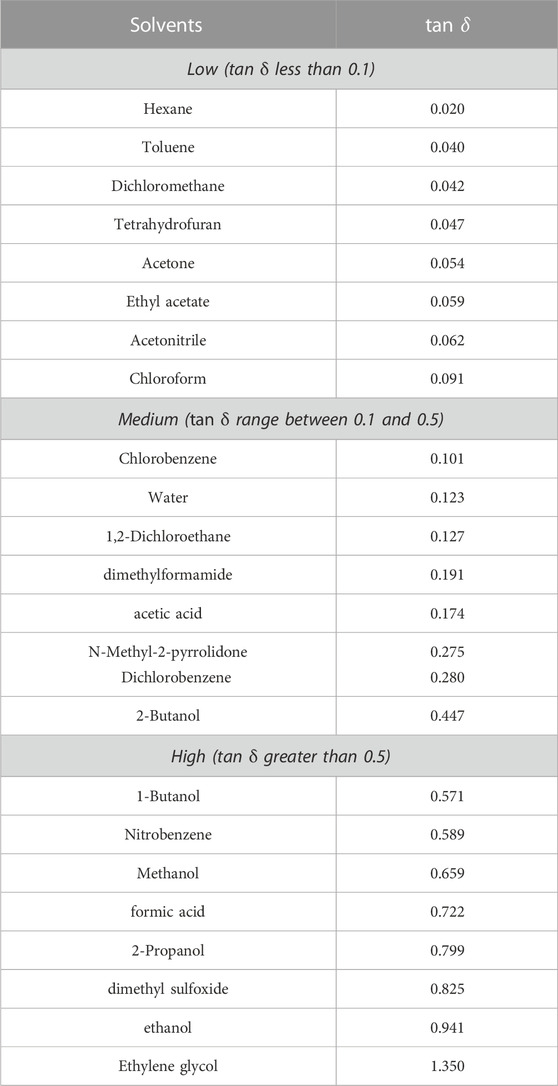
TABLE 1. Various solvents and their loss factor value (tan δ) were determined at 293 K (de Medeiros et al., 2019).
Figure 3A demonstrates how microwave heating differs from traditional heating in that the center of the material is heated to a greater temperature than the medium allowing for a quick and efficient reaction. In contrast, under conventional heating, the vessel must be heated first followed by heating of the medium and eventually the heating of the sample. Under microwave heating, the molecules’ dipoles attempt to align with the applied electric field during irradiation in microwave-assisted synthesis (Figure 3B). Higher frequency field oscillations cause the dipoles to continually realign with the alternating electric field, which generates heat through molecular friction and dielectric loss. No heating occurs if the dipole doesn’t have time to realign (at much higher frequencies), or if it reorients too quickly (during low-frequency irradiation) (Sun et al., 2016; Jiang et al., 2023). Ionic conduction can also contribute to the heating mechanism. Under the influence of the microwave energy field, charged particles that are dissolved into the sample, in ionic form, bounce back and forth and subsequently produce heat by interacting with nearby molecules or atoms (Gabriel et al., 1998; Singh et al., 2019). This technique have been used to prepare different types of nanomaterials including metallic nanoparticles (Zhu and Chen, 2014; Tsuji, 2017), semiconductor quantum dots (Xuan et al., 2015), metal-organic nanomaterials (Thi Dang et al., 2020), polymeric nanomaterials (An et al., 2006), carbon-based nanomaterials (de Medeiros et al., 2019; Thakur et al., 2023), and many others.
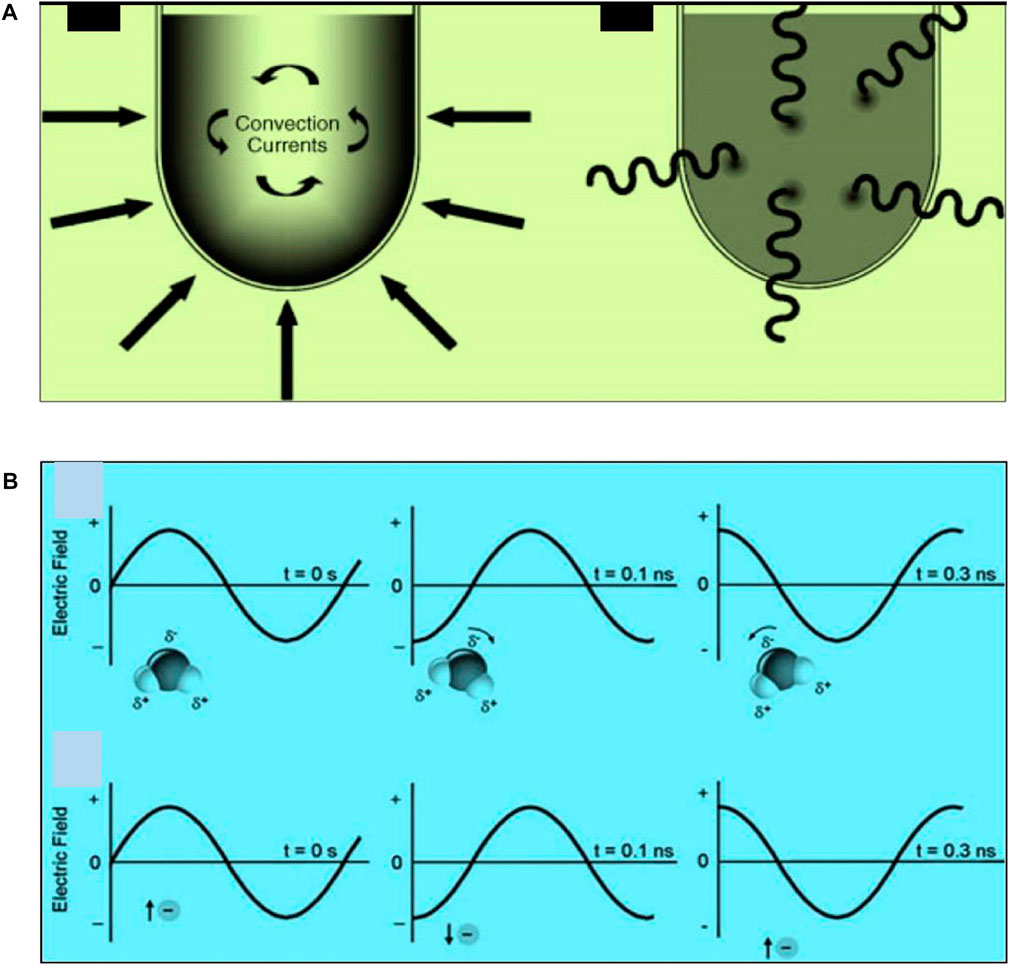
FIGURE 3. (A) Comparison of conventional heating and microwave heating (B) Mechanisms of microwave energy transfers are dipole polarization (top) and ionic conduction (down). Adapted with minor amendments and permission from Wiley (Kappe et al., 2012).
4 Microwave-assisted synthesis of carbon-based nanomaterials
Attention has been drawn to the synthesis of next-generation nano-carbon materials, including heterostructures and nanocomposites with improved performance and multifunctionality (Speranza, 2021). To determine the feasibility of prolonged utilization of microwave technology for developing advanced carbon-based nanomaterials like CDs, CNTs, and graphene-based materials (GBMs), the availability of carbon sources is crucial. These nanomaterials have so far been produced using carbon sources including citric acid, acetylene, benzene, methane, toluene, xylene, and other natural hydrocarbon resources (Liu et al., 2011; de Medeiros et al., 2019; Macairan et al., 2019; Sajid et al., 2022; Gulati et al., 2023; Liu et al., 2023). These hydrocarbons contribute to the release of CO2 which may leak into the atmosphere, leading to global warming. Methane and other volatile hydrocarbons are greenhouse gases that act as more powerful heat-traps than CO2 and contribute more to climate change than CO2, which they release following complete combustion. Furthermore, these hydrocarbons are fast depleting and valuable carbon-based nanomaterials may experience a shortage issue in the future due to their reliance on these non-renewable resources. Hence, a more practical, affordable, and environmentally benign method and resources are required to sustainably prepare advanced carbon-based nanomaterials.
Microwave irradiation, which causes polymerization and carbonization, has been used to prepare carbon-based nanomaterials (Alarfaj et al., 2018). Carbon-based nanomaterials absorb electromagnetic energy during the microwave irradiation process and transform it into heat energy (Yang et al., 2018a). The molecular rotation of polar solvent molecules, caused by the interaction of electrical dipole moment with microwave energy, produces thermal energy. During microwave-assisted heating, electromagnetic radiation transfers thermal energy to the carbon precursor (Singh et al., 2019).
The preparation of carbon-based nanomaterials from biobased resources is essentially a top-down approach, which involves the break down of large carbonaceous materials, such as agricultural wastes, into nano-sized carbon structures (de Medeiros et al., 2019; Thakur et al., 2023). The microwave-assisted synthesis is effective for producing carbon nanomaterials and has the added benefits of rapid volumetric heating, high reaction rates, precise control over size and shape of nanomaterials by reaction parameter adjustment, and energy efficiency. Additionally, the homogeneous nucleation and growth conditions created by the homogenous heating of the precursors during microwave synthesis reduce thermal gradients and result in the development of nanomaterials with a uniform size distribution (Bacon et al., 2014).
4.1 CNTs and doped CNTs
In recent years, multiple studies have targeted the production of CNTs from waste materials to counter the more expensive and time-consuming synthesis procedures that were typically used (Liu et al., 2019). CNTs (1D nanocarbon) may now be easily grown using microwave radiation, opening up a new method for their versatile and economical synthesis. Both domestic and commercial microwave ovens have been utilized as practical plasma reactors for the quick, easy, energy-efficient, and solvent-free growth of CNTs (Jašek et al., 2006; Baghel et al., 2022). In addition to enabling the rapid growth of high-density CNTs in a matter of seconds, the unique heating mechanism of microwaves also eliminates the requirement for an expensive boiler and a source of combustible gaseous carbon (Liu et al., 2019). Under microwave irradiation, very localized heating, close to the catalyst nanoparticles, can be observed (Chin et al., 2021).
It has been reported that rice husk was successfully used to synthesize CNTs using microwave-induced plasma irradiation with a 600 W and 2.45 GHz power and frequency, respectively (Asnawi et al., 2018). The procedure was carried out at a 750°C temperature for 40 min. The pressure in the reaction tube decreased from 3 to 1 mbar as CNTs grew. The biosynthesized CNTs revealed a twisted and web-like network structure and Raman spectra showed that the ID/IG ratio was 1.013. The high ID/IG ratio between the D and G band intensities is an indication of the lower quality of the sp2 hybridized carbon nanomaterial (Figure 4). The lower quality may be due to structural defects and the amorphous nature of the carbon-based nanomaterial. This experiment demonstrates the possible use of waste biomass as a precursor for microwave-based CNTs synthesis. In addition to turning waste biomass into valuable carbon nanostructures, this would help address the environmental problems brought on by the vast amount of agricultural biomass.
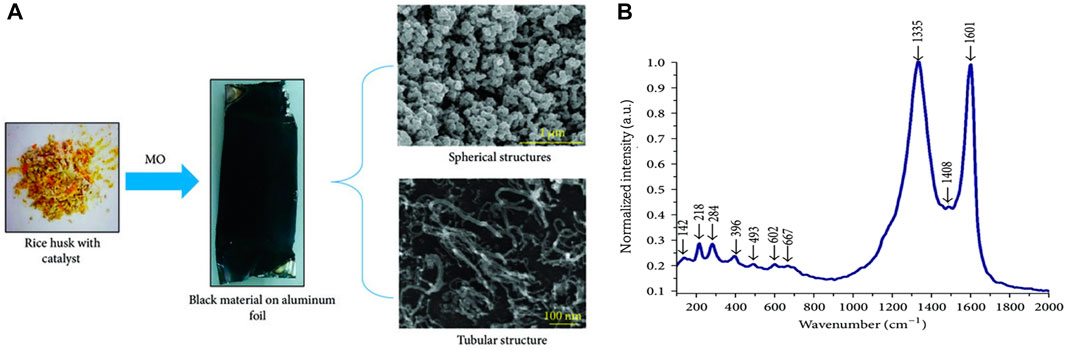
FIGURE 4. (A) The transformation of rick husk’s soot-like material onto the surface of aluminum and Fe-SEM image showing both spherical and tubular forms on a microscale (1 µm) and nanoscale (100 nm), respectively (B) Raman spectrum of the CNTs biosynthesized sample. Adapted with slight modifications from (Asnawi et al., 2018). Open access.
Similarly, Hidalgo-Oporto et al. reported the use of biochar derived from wheat straw, oats hull, rapeseed cake, and hazelnut for the preparation of CNTs (Hidalgo et al., 2019). A carbon-rich porous material is produced when biomass is subjected to a thermochemical process and can serve as a precursor for CNTs synthesis (Figure 5). A combination of biochar derived from agricultural waste and ferrocene was heated with a microwave to create CNTs at 400°C and 600°C. The findings showed that the biomass pyrolysis temperature had an impact on the physicochemical characteristics of CNTs. Higher CNTs concentration and lower hydrodynamic diameter were obtained by 600°C biochar. Moreover, CNTs prepared from biochar derived from wheat straw and hazelnut hulls had a higher degree of wall graphitization, indicating superior CNTs quality.
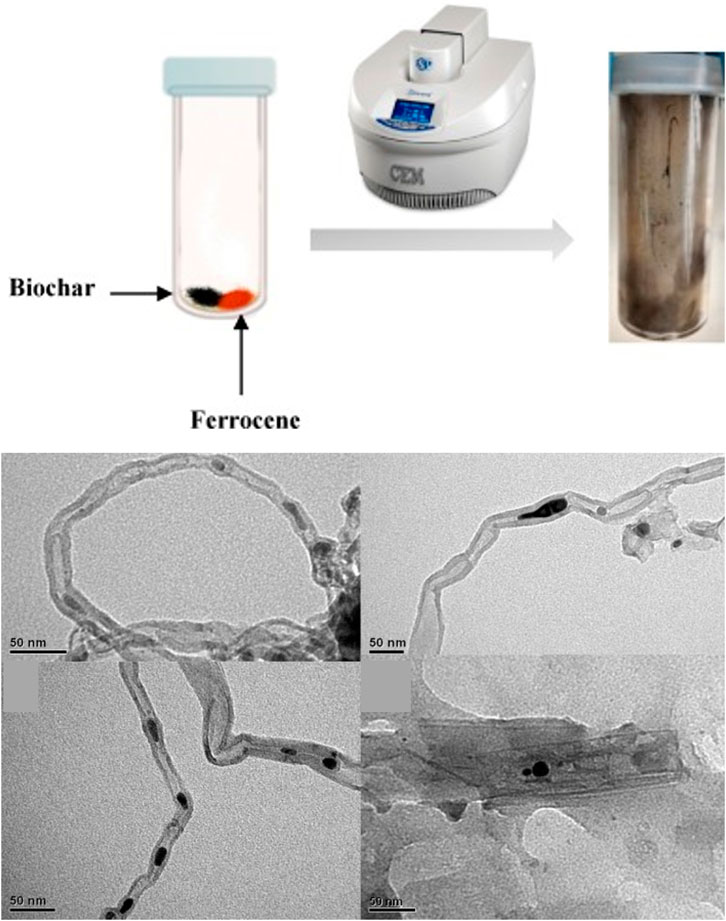
FIGURE 5. Illustration of microwave irradiation as a tool to develop CNTs from a biochar/ferrocene combination (top). TEM images of CNTs prepared from biochar derived from oat hull, wheat straw, hazelnut hull, and rapeseed cake, respectively (bottom). Adapted with minor modifications and permission from Elsevier (Hidalgo et al., 2019).
In a more recent study, Omoriyekomwan et al. reported the use of palm kernel shells to synthesize CNTs using the microwave pyrolysis process at a low temperature of 600°C (Omoriyekomwan et al., 2019). They employed the palm kernel shell (PKS) and used two isolation procedures, namely alkaline-acid and formic acid/acetic acid, to separate the components of PKS, cellulose, and lignin. They claimed that the recovery of monosaccharides in the cellulose’s pyrolysis volatiles, which served as a substantial carbon source, promoted CNTs growth. Moreover, the functional group, organic matrix, flaws, and structural quality were all improved. The mechanism of CNTs growth has been postulated to involve the self-extrusion of monosaccharides- and hydrocarbons-rich volatiles during cellulose pyrolysis proceeded by condensation and re-solidification of volatiles on the softened cellulose particles at high temperatures (Figure 6). Although a high ash content in biobased materials or agricultural wastes can operate as a catalyst to accelerate the formation of CNTs, high-purity precursors are still necessary for CNTs synthesis.
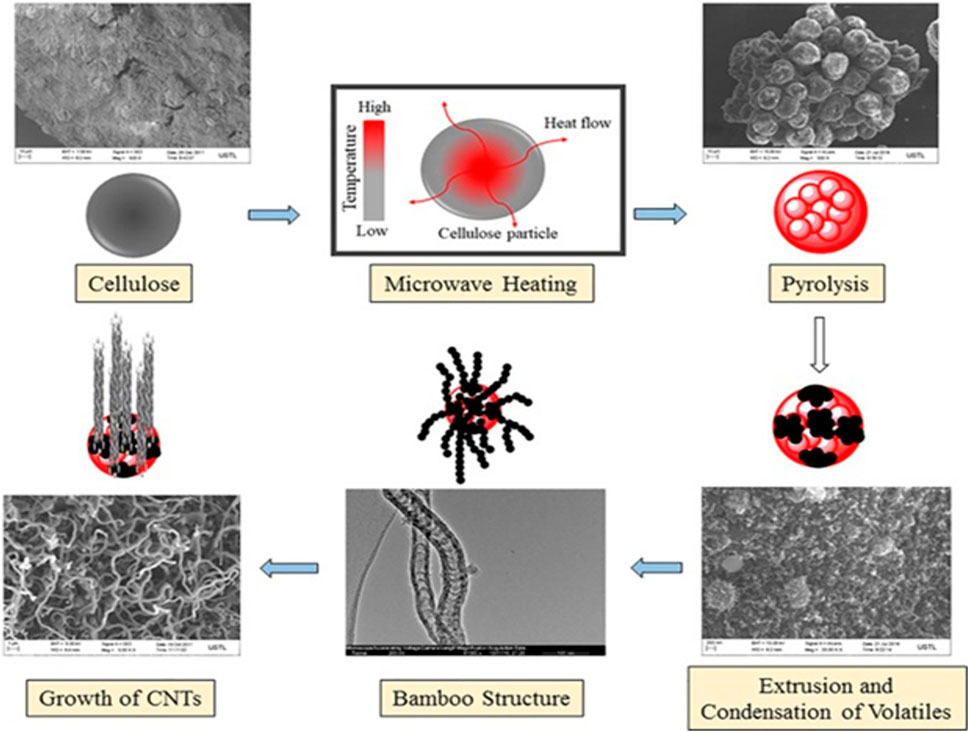
FIGURE 6. The mechanism of CNTs formation during microwave-induced pyrolysis of cellulose. Adapted with minor modifications and permission from Elsevier (Omoriyekomwan et al., 2019).
It is noteworthy to mention that while most synthesis protocols for CNTs required hours, the microwave-assisted technique generates CNTs in minutes and seconds. Interestingly, Zhan et al. revealed that in 20–40 s, CNTs can be synthesized using a domestic microwave, howbeit from iron, copper, molybdenum, steel wires, and fibers were used as precursors and not biobased materials. The as-synthesized CNTs had a diameter range of 10–120 nm (Zhan et al., 2017). CNT-based composites have been synthesized in 150 s with the aid of a microwave; they include palladium-graphene oxide-based CNTs (Kumar et al., 2017), nitrogen-iron doped CNTs (Kang et al., 2017) (Figure 7), ruthenium-based single-walled carbon nanotubes (SWCNTs-Ru) (Hemraj-Benny et al., 2020), CNTs/Fe3O4 (Li et al., 2023a), etc. Other precursors have been reported in Literature, such as graphite (Algadri et al., 2017; Guo et al., 2017), carbon fiber (Bajpai and Wagner, 2015), Mxene aerogel (Zheng et al., 2019), graphite and cobalt acetate powder (Ortega-Cervantez et al., 2016), as well as poly lactic-co-glycolic acid (PLGA) coated with polypyrrole (PPy) (Xie et al., 2014).
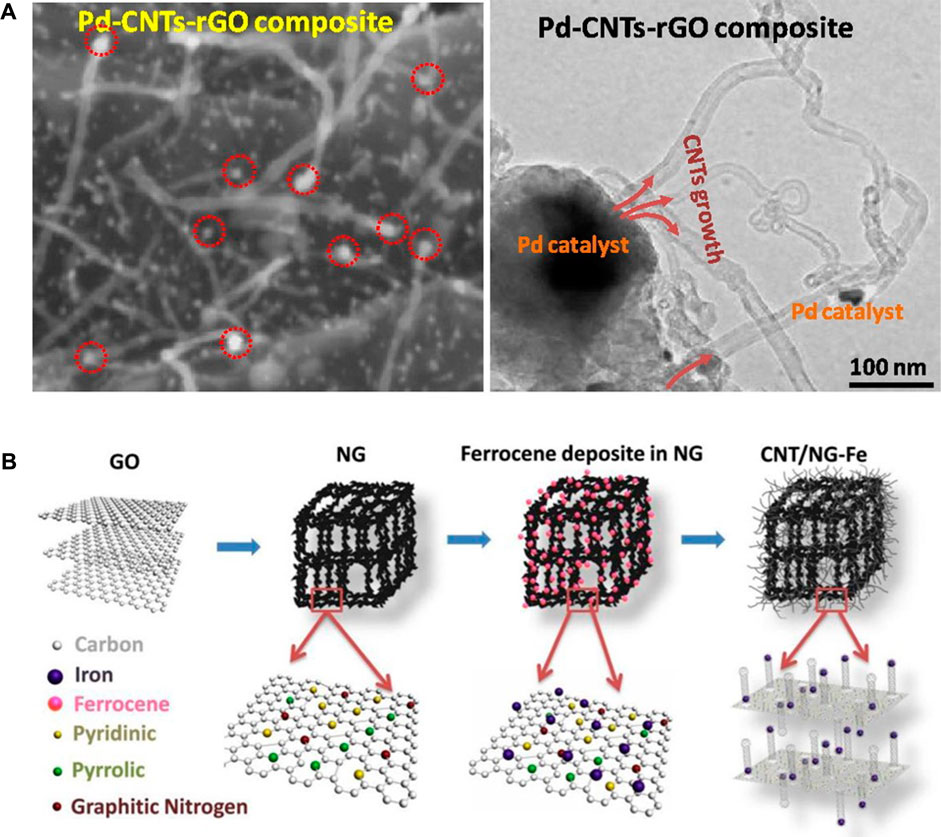
FIGURE 7. (A) Hierarchical palladium-CNTs-reduced graphene oxide composite produced with microwave (B) 3D nitrogen-incorporated reduced graphene oxide (NG)/iron oxide (CNTs/NG-Fe) CNTs nanocomposite. Adapted with permission from Elsevier (Kang et al., 2017; Kumar et al., 2017).
The affinity of carbon-based nanomaterials for specific types of contaminants in aqueous media is impacted by the different functionalities/moieties on their surface, or their hybridization/combination with other nanomaterials/molecules, which also improves their adsorption efficiency. A few chemical modification techniques and their benefits are summarized in Table 2. Functional moieties may contain oxygen-, nitrogen-, and sulfur-containing groups depending on the type of heteroatom that binds to the carbon in the nanomaterial. Through selective design with appropriate functional groups, the adsorption selectivity of contaminants may improve (Sajid et al., 2022). Typically, related functional groups are introduced to carbon compounds by oxidation, nitrogenation, and sulfonation.
4.1.1 CNTs-based materials as adsorbents for pollution remediation
Adsorbents composed of CNTs are commonly used to remove chemical pollutants from water and wastewater (Gupta and Saleh, 2013; Yu et al., 2016; Gusain et al., 2020). Due to their large specific surface areas, tunable surface characteristics, porosities, hydrophobicity, hollow and layered architectures, many internal and external binding sites, π-conjugation, as well as simplicity of chemical activation and functionalization, CNTs exhibit excellent adsorption capabilities (Barrejón and Prato, 2022; Pyrzynska, 2023). CNTs-based adsorbents can interact with inorganic and organic pollutants through a variety of mechanisms, including complexation, ion exchange, electrostatic interaction, covalent bonding, precipitation, and van der Waal’s interaction, among others (Figure 8) (Adeola and Forbes, 2021a; Sajid et al., 2022). Chemical bonds between organic compounds and CNTs have occasionally been described as a mode of interaction (Indrawirawan et al., 2015; Duarte et al., 2022).
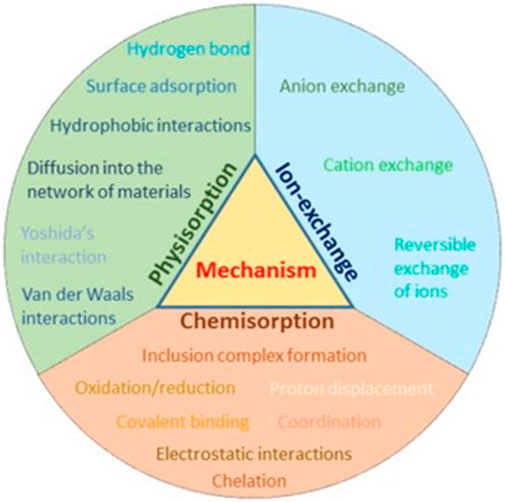
FIGURE 8. Various adsorption mechanisms of interaction between CNTs and chemical pollutants in aqueous matrices.
The removal of PFAS and other persistent and endocrine-disrupting pollutants with CNTs-based materials has been reported (Vu and Wu, 2022). As the C-F chain length increased, more perfluorinated compounds (PFCs) with the same functional group were able to bind to MWCNTs. The removal efficiency of the CNTs with hydroxyl and carboxyl groups was significantly lower than that of the pristine CNTs (Deng et al., 2012a). Hydrophobic interactions dominated the mechanism of adsorption. With an Langmuir maximum adsorption capacity of 46.2 mg/g, magnetic MWCNTs coated with chitosan were utilized to treat water containing Bisphenol A (BPA), another endocrine-disrupting substance (Mohammadi et al., 2020). The residual BPA concentration was determined using high-performance liquid chromatography (HPLC).
Pharmaceuticals present in different aquatic systems can cause serious health problems in people, including inflammation, vomiting, headaches, dizziness, and other minor and major systemic dysfunction. The main problem is that these pollutants cannot be fully removed or degraded by traditional wastewater treatment facilities (Adeola and Forbes, 2021b; Ihsanullah et al., 2022). A nanocomposite of CNTs and alumina was employed to adsorb carbamazepine and sodium diclofenac from water. The residues of diclofenac and carbamazepine were analyzed using UV–vis spectrophotometer. The CNTs were used for adsorption while the alumina improved the hybrid’s physicochemical properties and regenerability (Wei et al., 2013). In several additional instances, SWCNTs and MWCNTs were used to remove amoxicillin and SWCNTs displayed much better adsorption capacity for the selected pharmaceutical drug (Mohammadi et al., 2015; Balarak et al., 2016). Residual amoxicillin was analysed using HPLC. In a related investigation, batch experiments were used to examine the ciprofloxacin’s adsorption behavior from an aqueous solution onto MWCNTs/Al2O3 (Balarak and McKay, 2021). The spontaneity and endothermic nature of the sorption process were shown by thermodynamic studies. According to the Dubinin-Radushkevich isotherm model, the estimated mean free energy, which ranged between 0.316 and 0.707 kJ/mol, supports a physisorption process. It was established that MWCNTs/Al2O3 are potentially useful for the elimination of antibiotics from contaminated water.
Metals are a major part of the group of contaminants that have been researched extensively in CNTs-based adsorption, as they cause numerous health problems in people (such as lead poisoning), animals, and aquatic life (Sajid et al., 2018; Ihsanullah et al., 2020; Sulaiman et al., 2020). The causes of metal contamination are numerous and include both anthropogenic and natural processes and must be removed from the water to pre-determined acceptable limits (Feng et al., 2018; Ore and Adeola, 2021; Adeola et al., 2022a). Mercury was eliminated using MWCNTs that were loaded with CuS. Because of the high surface area of MWCNTs, the amount of CuS dispersed on the surface of MWCNTs increased. CuS addition to the adsorbent was intended to improve mercury affinity. Moreover, the copper can amalgamate with Hg (Wang et al., 2020b). Thus, the CuS/MWCNTs surface purportedly adsorbed mercury as stable HgS, and the sorption process was controlled or facilitated via chemisorption (Liu et al., 2022a). The continuous mercury analyzer (VM3000 Mercury Vapor Monitor) was used to analyze mercury. Recently, a mechanical method (ultra-sonification) and a chemical method (surfactant inclusion) were coupled to stabilize CNTs. The elimination of Cu(II), Ni(II), Pb(II), and Zn(II) ions was accomplished using these CNTs, and residual metal ions were analyzed using Atomic Absorption Spectrometer (AAS) Perkin Elmer 3,300. It was discovered that electronegativity and atomic radius played roles in competitive adsorption. Compared to Ni(II) and Zn(II) ions, a higher percentage of Pb(II) and Cu(II) ions were eliminated (Oliveira et al., 2021). The lead ion is the most electronegative metal with the highest atomic radii among the other cations, which favors its adsorption onto the MWCNTs structure via attractive and hydrodynamic forces.
Table 3 presents a list of CNTs-based nanomaterials that have been used to sorb organic and inorganic compounds from polluted aqueous media. It is noteworthy to mention that various forms of CNTs-based materials have been reported to have Langmuir adsorption capacities as high as 416 mg/g (Cu) for metals, 679.6 mg/g (Rhodamine B) for dyes, 227.3 mg/g (Chipton) for pesticides, 221.2 mg/g (Meloxicam) for pharmaceuticals, and 46.2 mg/g (Bisphenol A) for other compounds, which affirms the affinity of CNTs and their composites for these various classes of compounds.
4.2 Graphene and graphene-based materials
Graphene-based materials (GBMs) have attracted much scientific curiosity in the last decade due to their exceptional electronic, mechanical, and optical properties which have made them adaptable in several fields of scientific innovations (Sang et al., 2019; Adeola and Forbes, 2021a). Graphene (2D nanocarbon) and its derivatives can be functionalized for use in a wide range of fields, including environmental remediation, medicine, and electronics (Khenfouch et al., 2016; Liu et al., 2020; Papi, 2021; Hashmi et al., 2022). Figure 9 reflects the most common types/forms of graphenic materials, they are graphite, CNTs, reduced graphene oxide, and fullerenes, among others. Because of their unique features, all these graphene-based materials are very different in comparison to one another. Biomass and agricultural waste are useful carbon sources that have been used to produce graphite, which in turn serves as precursor for graphene-based materials (Vivekanandhan et al., 2017; Shi et al., 2023a).
To incorporate hydroxyl, carboxyl, and carbonyl groups on the surface of graphite, harsh chemicals like sulfuric, nitric, and phosphoric acids are typically used in chemical reactions to produce graphene-based materials. When pre-treated graphite is exposed to high temperatures, gases evolve and form graphitic layers (Hernandez et al., 2008). The acidic precursors can intercalate between the graphitic layers because they have two hydroxyl groups on either side. In addition to the emission of toxic gases, the separation of the graphitic layers is frequently haphazard and ineffective. The microwave-assisted synthesis may address these challenges via rapid heating and better control of the reduction and exfoliation of graphitic layers. The microwave-assisted synthesis of GBMs can be achieved under three categories: 1) chemical reduction of graphite with the aid of a microwave; 2) thermal reduction of graphite with a microwave; 3) simultaneous thermal exfoliation and thermal reduction of graphite with a microwave (Al-Hazmi et al., 2015; Xie et al., 2019).
Microwave-assisted chemical reduction of graphite involves the use of a reducing agent such as hydrazine hydrate under microwave irradiation to expedite the process (Hassan et al., 2009). Hassan et al. examined the reduction process using Raman spectroscopy and observed an ID/IG ratio between 0.1 and 0.12 following microwave treatment for 60 s, demonstrating a high reduction degree even after such a brief treatment time. The enhanced thermal stability showed no appreciable mass loss up to 750 °C Another study by Kumar et al. reported that a 4-h microwave exposure was comparable to a 48-h conventional reaction technique used for the synthesis of GBMs (Kumar et al., 2015). The traditional heating method led to the formation of defects in the graphene basal plane as a result of the evolution of the oxygen functional groups during reduction (Li et al., 2010). This can be addressed by the microwave-assisted thermal reduction approach either with a commercial microwave or a microwave-plasma reactor (Figure 10). Without using any reducing agents or solvents, microwave irradiation’s superior thermal action may quickly convert GO into graphene (Voiry et al., 2016; Jiang et al., 2018; Wan et al., 2018). This method offers a viable opportunity to produce graphene on a huge scale. To clarify the underlying process of GBM microwave reduction, more research on the dielectric characteristics of GBMs at various microwave frequencies is necessary.
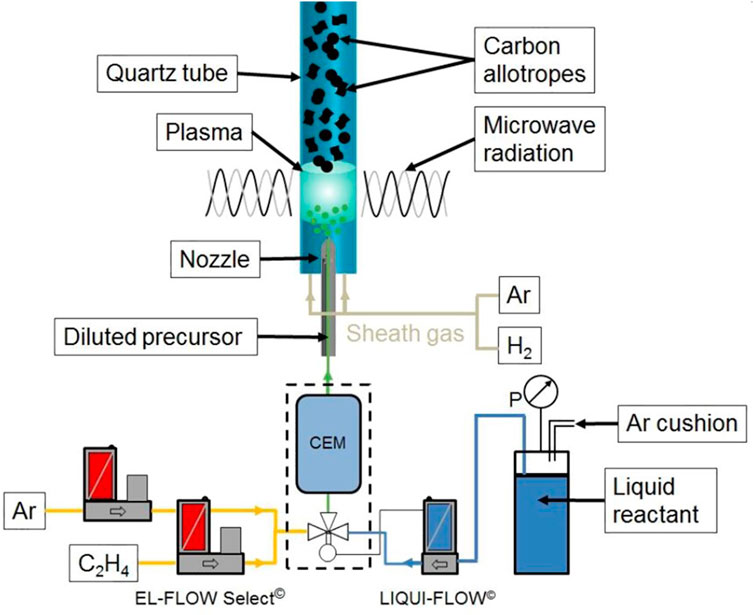
FIGURE 10. Microwave-plasma reactor including reactants supplies and metering. Adapted from (Fortugno et al., 2022). Open access.
Due to its poor microwave absorption capacity, graphite oxide itself may not be heated to a temperature that will effectively cause its exfoliation and reduction under microwave irradiation. It is not possible to produce rGO with the same level of purity by simultaneous exfoliation and reduction as it is through the thermal reduction of graphene oxides (Zhu et al., 2010a; Zhu et al., 2010b; Pokharel et al., 2014). However, the reduction degree could be significantly increased when paired with a reducing environment (i.e., H2) or pretreatment with powerful reducing reagents (NaBH4).
Microwave treatment has been used to synthesize a graphene-based material from used spent tea leaves (Abbas et al., 2020). Due to their distinctive optical characteristics, size, and quantum confinement, graphene quantum dots (GQDs) are zero-dimensional fluorescent materials that have garnered considerable attention. However, one of the associated challenges is their low reaction yields, which contribute to high production costs and restrict their large-scale applications. Using microwave treatment, spent tea was used as a low-cost, environmentally friendly, and renewable biomass resource for the high-yield synthesis of GQDs. The synthesis method uses oxidative cutting and pyrolysis under microwave irradiation to generate GQDs, with an over 84% yield recorded. The size distribution of GQDs was extremely narrow, with an average size of 1.6 ± 0.55 nm. The energy band gap and luminescence emission in the high-energy area increased as a result of the reduced size (Abbas et al., 2020). Similarly, microwave plasma irradiation was used to prepare graphene-based materials from rice husks (Wang et al., 2015a). Plasma and microwave heating was used to irradiate the powdered rice husks to a temperature of approximately 200 °C while biogas/pyrolysis gas (often CH4 or C2H4) was present (Figures 10, 11). The pressure and temperature utilized in the microwave setup had a significant impact on the dissociation of rice husks and the production of graphitic carbons (Worasuwannarak et al., 2007; Wang et al., 2011; Chen et al., 2014).
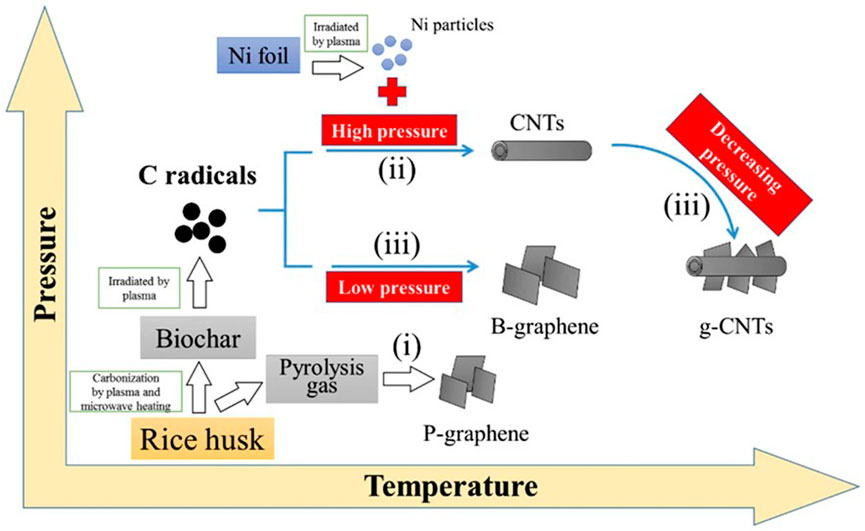
FIGURE 11. A plausible microwave plasma irradiation growth model for graphene, CNTs, and g-CNTs from rice husks Adapted with permission from Elsevier (Wang et al., 2015a).
4.2.1 Graphene-based materials as adsorbents for pollution remediation
Chemical pollutants are produced by industrial processes, agricultural technology, and synthetic substances like pharmaceuticals and personal care items. Among the main pollutants released by numerous industries are hazardous heavy metals (e.g., Cd, Cu, Hg, Ni, Pb), toxic gases, and organics (e.g., dyes, pesticides, pharmaceuticals) (Alengebawy et al., 2021; Mitra et al., 2022). Industrial growth has a considerable detrimental impact on water resources due to the excessive use of these chemical compounds, which are exceedingly dangerous.
When fossil fuels, coal, and biomass are burnt, polycyclic hydrocarbons are produced that are classified as PAHs (Adeola et al., 2022b). These chemicals are extremely hazardous because they affect human organs leading to tumors, cancer, and chronic cardiovascular problems (Hussain et al., 2018; Song et al., 2021). In addition to PAHs, dyes, surfactants, industrial additives, and pesticides are also worrisome because they interfere with the endocrine system and nerve cells. Another category of organic pollutants, including phenols, bisphenol A, biphenyls, and phenyls, threatens aquatic flora and fauna and poses a health threat to humans (Mohammadi et al., 2020; Tavengwa et al., 2021). Even at very low concentrations (e.g., part per million and part per billion), these organic contaminants are recalcitrant and persistent in the environment. Therefore, it is crucial to remove both organic and inorganic pollutants before the release of wastewater into the environment. Hence the need to develop efficient materials for their removal from aqueous matrices.
There has been extensive research into using graphene and its composites to treat water that contains organic and inorganic contaminants, as indicated in Table 4. To address the United Nations’ sustainable development goals (SDG six in particular) and the shortcomings of existing wastewater systems, graphene, and its derivatives can be combined with a variety of metallic or non-metallic components to create innovative composites that increase the efficiency of removing pollutants in water or wastewater. To effectively treat wastewater, graphene, and its composites may be modified by eliminating, or adding functional groups and facilitating the formation of polymeric membranes or nanocomposites (Wu et al., 2011; Verma et al., 2022a). The process of doping and/or functionalizing/passivation can reportedly amplify the inherent graphene features and add additional stability for enhanced performance or multifunctional activity (Adeola et al., 2021a; Singh et al., 2022). It is noteworthy to highlight that adsorption capacities as high as 1,119 mg/g (Pb) for metals, 2000.1 mg/g (MB) for dyes, 1,667 mg/g (Malathion) for pesticides, 796.8 mg/g (Doxycycline) for pharmaceuticals and 766.58 mg/g (Naph) for aromatic compounds have been reported for various forms of carbon-based materials (Table 4). The removal performance of graphene-based materials is often influenced by the physicochemical properties of the sorbent and sorbate, as well as the solution chemistry encompassing pH, temperature (thermodynamics), salinity, and other process parameters. The adsorption capacities reported for graphene-based materials for different classes of pollutants were generally higher than many of those obtained for CNTs (Tables 3, 4), suggesting that structure and atomic configurations may also play a role in the binding mechanism and capacity of various carbon-based nanomaterials/nanocomposites.
4.3 CDs and nanocomposites
CDs are zero-dimensional carbon nanomaterials with diameters smaller than 10 nm and have been touted to have minimal toxicity and excellent photostability (de Medeiros et al., 2019; Behi et al., 2022). The majority of CDs include sp2 and sp3 hybridization, which are often similar to those in crystalline graphite but lack structural identity (Georgakilas et al., 2015). CDs be prepared as hydrophilic, hydrophobic, and amphiphilic, based on the intended applications (de Medeiros et al., 2019). However, for the adsorption of chemical pollutants in aqueous matrices, hydrophobic CDs with high surface area and porosity are most desirable. Thus, the utilization of relatively water-insoluble carbon, nitrogen, and oxygen precursors is necessary for the preparation of hydrophobic CDs. In addition, CDs and other carbon-based nanomaterials must be recoverable, regenerable, and reusable from a green and sustainable chemistry point of view.
Regarding hydrophobic carbon dot-based nanomaterials for water treatment applications, researchers have reported several pristine CDs and composites, i.e., CDs (Yahaya Pudza et al., 2020), CD-modified magnetic nanotubes (Deng et al., 2019), hydrophobic nitrogen-doped CDs (Shi et al., 2023b), carbon quantum dots (Tohamy et al., 2023), and hexadecylamine-functionalized GQDs (Kubheka et al., 2022), among others. CDs and carbon quantum dots have been synthesized using green and sustainable precursors such as agricultural wastes and carbohydrates including cellulose (Yahaya Pudza et al., 2020; da Silva Souza et al., 2018), biowaste lignin (Shi et al., 2019), lemon and onion (Monte-Filho et al., 2019), wood soot (Zhong et al., 2018), dried leaf (Joshi et al., 2018), grass (Sabet and Mahdavi, 2019), broccoli (Arumugam and Kim, 2018), pomelo fruit (Ramar et al., 2018), denature milk (Athika et al., 2019), to name a few. CDs have been used as adsorbents for the removal of both organic and inorganic pollutants in water as summarized in Table 5. For instance, they have been used to monitor dangerous substances in effluents discharged from various chemical industries and adsorbed uranium, cadmium, and benzopyrene from real water samples (Li et al., 2018; Rahmanian et al., 2018; Huang et al., 2019c). Doping carbon quantum dots with nitrogen reportedly enhanced the adsorption of metal ions from wastewater due to their high specific surface area (Tohamy et al., 2023). In comparison to CNTs and graphene-based materials, CDs have not been as widely applied as adsorbents, however functionalized hydrophobic CD-based composites can equally be used as adsorbents and in the fabrication of membranes.
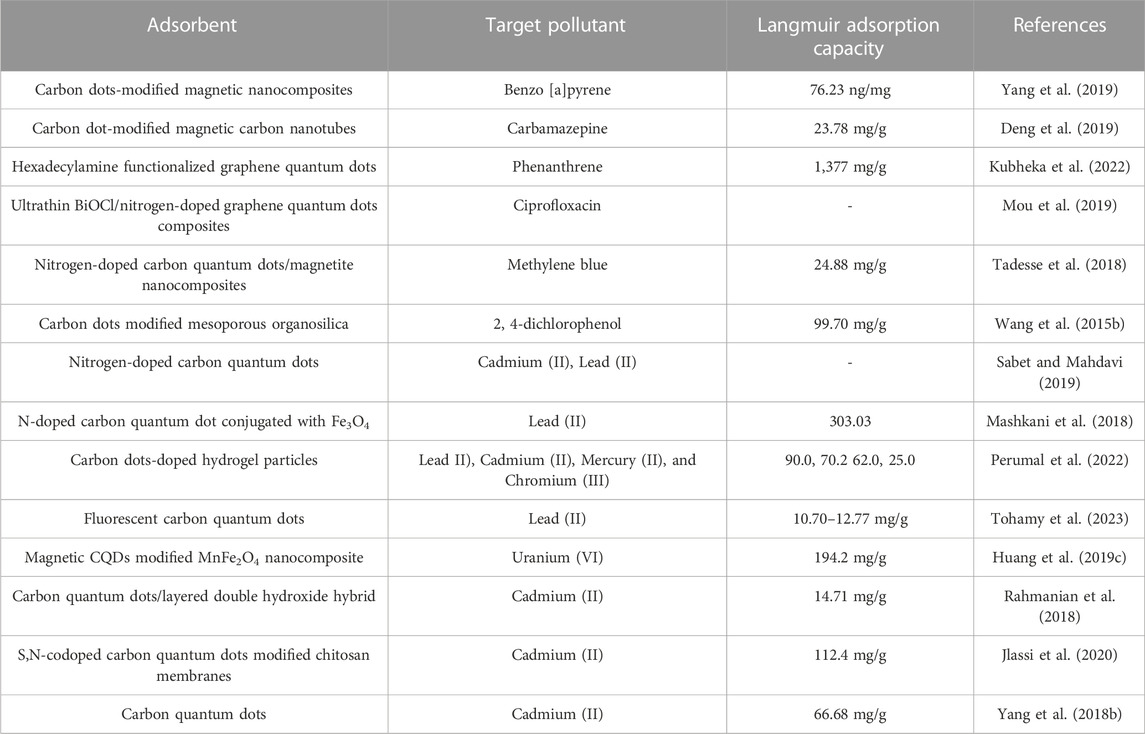
TABLE 5. CDs and derivatives as adsorbents for removal of organic and inorganic pollutants in water.
Periodic mesoporous organosilica embedded with CDs has been developed for the elimination of 2,4-dichlorophenol and heavy metal ions Hg(II), Cu(II), and Pb(II) by Wang et al. (Wang et al., 2015b). The material has a bilayer of CDs, 2D hexagonal mesostructure, high specific surface area, and pore size (∼468.46 m2/g and ∼5.50 nm). The n–π electron donor–acceptor interaction between O- and N-containing moieties in mesoporous organosilica and the benzene ring in 2,4-dichlorophenol facilitated enhanced adsorption of 2,4-dichlorophenol, while the electrostatic interaction and complex formation between metal ions and amide groups help to increase the efficiency of metal ions removal from contaminated water (Wang et al., 2015b).
Perumal et al. reported the synthesis of nitrogen-doped CDs (N-CDs) and hybrid-spherical-shaped hydrogel particles (CGCDs) from Red Malus floribunda fruits (Perumal et al., 2022). The synthesized CGCDs were utilized as sorbents for the removal of heavy metals from water. The sizes of the CD-based materials ranged from 20 to 300 μm. Approximately ∼72% and 99% of Hg(II) was sorbed by CGCDs in single metal ion and multiple metal ion systems. Furthermore, Hg(II), Cd(II), Pb(II), and Cr(III) recorded over 70% removal efficiency in multiple systems by CGCDs (Perumal et al., 2022). This suggests that CD-based materials may serve as a suitable tool for the simultaneous removal of heavy metals from industrial wastewater. In comparison to CNTs and graphene-based materials, CDs have not been as widely applied as adsorbents, however, functionalized hydrophobic CD-based composite can be equally used as adsorbent materials and in the fabrication of membranes.
In general, carbon-based nanomaterials interact with organic and inorganic compounds via one or several adsorption mechanisms. Electrostatic interaction: adsorption of charged organic and inorganic pollutants onto the charged surface of carbon-based nanomaterials (Isaeva et al., 2021). Electric potential is created on the adsorbent by the presence of positive and negative charges on the carbon adsorbents as a result of deviation from the pH point of zero charge. Under varying solution pH, organic pollutants become charged as a result of protonation and deprotonation. These electrostatic charges cause adsorption due to electrostatic interactions on both the surface of the organic pollutant and the surface of the carbon-based nanomaterial. When functional groups are protonated or deprotonated, the strength of these interactions also varies (Deline et al., 2020; Isaeva et al., 2021). π-complexation interactions involve electron sharing between contaminants and carbon-based nanomaterials, resulting in the creation of inner-sphere surface complexes. The overlap of π electrons between sorbate and carbon materials also leads to π-complexation or π-π interactions (Maitlo et al., 2019). Hydrogen bonding occurs mostly in compounds containing hydroxyl and carboxyl groups, and their adsorption by carbon nanomaterials is facilitated via this mechanism in aqueous medium (Speranza, 2021). Electron donors with an atom more electronegative than hydrogen and an acceptor atom with an unshared lone pair of electrons combine to produce a hydrogen bond between carbon nanomaterials and pollutants (Ahmed et al., 2022). Hydrophobic interactions: hydrophobic contaminants maybe driven to the surface and pores of hydrophobic carbon nanomaterials (Saji, 2021). The octanol-water partition coefficient (LogKow) of the pollutants reflects its hydrophobicity, and a partitioning mechanism often drives hydrophobic solutes in an aqueous medium onto the surface of carbon-based nanomaterials (Adeola and Forbes, 2019; Qian et al., 2020). Covalent bonding between adsorbate and adsorbent is often responsible for chemical adsorption (chemisorption). Covalent interaction between sorbates and carbon particles involves electron sharing for bond formation (Sabzehmeidani et al., 2021). On the hand, physisorption (physical adsorption) is driven by weak forces of interactions such as van der Waals interactions (Ion et al., 2021). Physisorption is often reversible and non-specific interaction between carbon nanomaterials and contaminants. It is controlled by competitive adsorption that occurs at varying rates at the heterogeneous surfaces of adsorbents (Agboola and Benson, 2021).
5 Merits and challenges of microwave-assisted synthesis of carbon-based nanomaterials
Significant advancements in microwave instrumentation have aided in the synthesis of materials with improved reaction conditions control, producing materials with more desirable physicochemical characteristics. Commercial microwave reactors are now equipped with fiber-optic probes, magnetic stirrers, and sensors for more precise monitoring of the synthesis conditions, resulting in an enhanced reaction yield with higher reproducibility (Favretto, 2003; Ferguson, 2003; Luo et al., 2013b; Dudley et al., 2015), lower energy requirements, and shorter reaction times, among others (Figure 12). However, pressure sensitivity and thresholds (typically lower than 400 psi), and penetration depth limitations are one of the main drawbacks of microwave-assisted synthesis (Antonio De La et al., 2011; de Medeiros et al., 2019). The volume needed for commercial scale-up is also a challenge as conventional microwave reactors cannot accommodate large-scale synthesis to this day. There are also some restrictions on the size of the reaction sample for the microwave-assisted synthesis of carbon nanomaterials due to the short penetration depth in particular materials. In-situ measurements of the reaction conditions, particularly with metallic substrates, are the other major restriction of microwave-assisted synthesis. Metallic substrates can’t be used for material synthesis or processing since they interfere with the electromagnetic field (Bilecka and Niederberger, 2010; Schwenke et al., 2015).
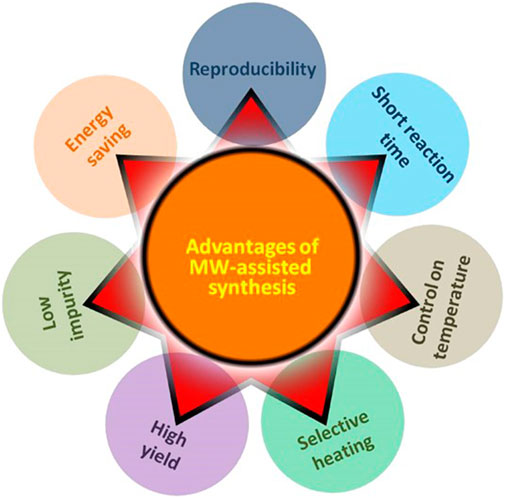
FIGURE 12. Merits of microwave-assisted synthesis of carbon-based nanomaterials. Adapted with permission from Elsevier (Singh et al., 2019).
The use of microwave reactors for chemical synthesis and industrial scale-up applications still faces several fundamental difficulties and there are knowledge gaps. Important features like heating homogeneity and rate may change during scale-up, and may significantly impact operating efficiency and product yield, yet these characteristic changes may occur via vaguely understood mechanisms (Sturm et al., 2014; Buttress et al., 2017). Some theoretical tools and predictive models have been developed to model large-scale applications of microwaves; however, the intricacy of the electromagnetic field distribution inside microwave reactors supports only qualitative results from these models (Robinson et al., 2010a; Robinson et al., 2010b; Li et al., 2023b). Despite all of this, these models provide valuable insights in addition to experimental results. A promising method of creating quantitative numerical models is now available thanks to recent developments in commercial software and computational resources (Yang and Chen, 2021). These models can guide the design of advanced reactors by predicting their performance and validating those predictions with experiments and clarifying scale-up behavior to increase industrial capacity. Techno-economic analysis such as a bottom-up or top-down cost method, can be used to estimate the budget for industrial scale-up, in terms of plant set-up, workers, electricity, waste generation, etc., to assess the technological and economic viability of carbon-based nanomaterials for water treatment (Ghosh et al., 2022; Mpongwana and Rathilal, 2022).
Carbon-based nanomaterials have demonstrated extremely high efficacy in water treatment, particularly when it comes to the elimination of both organic and inorganic pollutants. However, some carbon nanomaterials may not be the most appropriate choice for water treatment, if intended for direct use in membranes (Cao et al., 2022; Guo et al., 2022). Due to the presence of negatively charged groups, most carbon-based nanomaterials rapidly disperse in water, requiring high-speed centrifugation for their recovery (Almanassra et al., 2021; Abboud et al., 2022; Ge et al., 2023; Singh et al., 2023). Membrane fouling remains the main issue in the water treatment industry that can be reduced by utilizing carbon-based nanomaterials (Adeola and Forbes, 2021a; Singh et al., 2023). There is little techno-economic research on nanomaterials made of carbon and thus, challenging to determine the full potential of this class of nanomaterials in water treatment procedures, relying solely on adsorption performance.
In summary, microwave radiation has proven to be a very efficient heating source. It has the following benefits: 1) an effective heating source (superheating) that increases reaction rates and facilitates faster synthesis, 2) the ability to readily adjust instrumental or reaction parameters, 3) size and form control, 4) selective heating based on the idea that various materials react to microwaves differently, 5) due to homogeneous heating and improved process parameter control, chemical reactions are more reproducible under microwave heating than under traditional heating, 6) microwave chemistry may be combined with other established techniques, such as solvothermal and sonochemical techniques. On the other hand, the drawbacks of microwave-assisted synthesis include its lack of scalability (batch sizes are normally limited to a few grams), restricted applications (to materials that absorb microwaves), safety and health risks associated with the use of microwave-heating apparatus and cost of purchase of advanced microwave apparatus. Prior to industrial-scale utilization of advanced carbon nanomaterials synthesized with microwaves, commercial readiness, manufacturing scalability, availability at an economically viable cost, and post-treatment toxicity assessments are required. Furthermore, it will be useful to compare laboratory-scale and industrial site findings that will facilitate the development of carbon-based prototype filters and/or membranes for long-term wastewater treatment.
6 Concluding remarks
Advanced carbon-based nanomaterials, such as CNTs, CDs, graphene, and their composites along with other carbonaceous and non-carbonaceous materials, have been widely researched for the adsorptive removal of organic and inorganic pollutants in water. The development of effective methods for the separation and recycling of these contaminants is vital from environmental safety and process sustainability points of view. However, to facilitate the future design of carbon-based nanomaterials from bioresources via microwave technology and other techniques, it is important to have a thorough understanding of the bonding behaviors and intermolecular interactions using spectroscopic and microscopic characterization. The need for the techno-economic feasibility study for field-based/industrial-scaled applications of microwave-synthesized carbon nanomaterials from biobased materials cannot be over-emphasized.
The compendium in this comprehensive review (Tables 1–5), reveals that numerous carbon-based nanomaterials have been modified using functional groups, containing fluorine, nitrogen, oxygen, and sulfur, and via composite formation with other nanoparticles such as polymers, chitosan, magnetite, and biobased materials. These modifications create binding sites, large surface areas, and/or magnetic properties for optimal pollutant removal. In the mechanistic study of interactions between contaminants and carbon-based nanomaterials, a variety of approaches have been used, including zeta potential measurement, spectroscopic characterization, imaging techniques, theoretical calculations, and computational modeling. Electrostatics, complexation, π-π interactions, hydrogen bonds, hydrophobic interactions, covalent chemisorption, and van der Waals interactions are a few examples of potential sorbate-sorbent interaction processes that may occur. Future research can focus on several areas to address some lingering challenges or gray areas. The selective adsorption of chemical contaminants in aqueous matrices has not been thoroughly studied, and the principles underlying the selectivity are still not entirely understood.
In conclusion, due to the myriad of functional groups on the nanomaterials/nanocomposite, multifunctional carbon-based sorbents can be used to remove multiple contaminants simultaneously. It is possible to regenerate carbon-based nanomaterials depending on the nature of the sorbates, either by washing with suitable solvents, photodegrading, mechanical discharge, and/or combusting at appropriate temperatures, without inducing any significant damage to the nanomaterial. Physically adsorbed pollutants can be released more easily than chemically adsorbed ones since desorption requires less energy, thus preserving the carbon nanomaterials’ capacity for re-use. Recent developments demonstrate that under reasonably benign conditions, microwave technology would be an efficient method for producing pristine and functionalized carbon-based nanomaterials from biobased resources, with several benefits and prospective applications including the treatment of wastewater.
Author contributions
AA: Conceptualization; data curation; formal analysis, writing—original draft; writing—review and editing. MD: Data curation; formal analysis; writing—original draft. RN: Project administration; supervision; validation; writing—review and editing. All authors contributed to the article and approved the submitted version.
Acknowledgments
The authors are grateful for the funding provided by the Natural Sciences and Engineering Research Council (NSERC) through the Discovery Grant Program, and Concordia University for funding through the Research Chair Program.
Conflict of interest
The authors declare that the research was conducted in the absence of any commercial or financial relationships that could be construed as a potential conflict of interest.
Publisher’s note
All claims expressed in this article are solely those of the authors and do not necessarily represent those of their affiliated organizations, or those of the publisher, the editors and the reviewers. Any product that may be evaluated in this article, or claim that may be made by its manufacturer, is not guaranteed or endorsed by the publisher.
Supplementary material
The Supplementary Material for this article can be found online at: https://www.frontiersin.org/articles/10.3389/frcrb.2023.1220021/full#supplementary-material
References
Abbas, A., Tabish, T. A., Bull, S. J., Lim, T. M., and Phan, A. N. (2020). High yield synthesis of graphene quantum dots from biomass waste as a highly selective probe for Fe(3+) sensing. Sci. Rep. 10 (1), 21262. doi:10.1038/s41598-020-78070-2
Abboud, A. S., Ghaffarinejad, A., and Mollahosseini, A. (2022). Metformin-graphene oxide/alginate beads for the removal of toxic lead ions from aqueous media; kinetic and equilibrium studies. Environ. Prog. Sustain. Energy 41 (5), e13860. doi:10.1002/ep.13860
Abdul, G., Zhu, X., and Chen, B. (2017). Structural characteristics of biochar-graphene nanosheet composites and their adsorption performance for phthalic acid esters. Chem. Eng. J. 319, 9–20. doi:10.1016/j.cej.2017.02.074
Abdulsahib, W. K., Ganduh, S. H., Mahdi, M. A., and Jasim, L. S. (2020). Adsorptive removal of doxycycline from aqueous solution using graphene oxide/hydrogel composite. Int. J. Appl. Pharm. 12 (6), 100–106. doi:10.22159/ijap.2020v12i6.39118
Abid, N., Khan, A. M., Shujait, S., Chaudhary, K., Ikram, M., Imran, M., et al. (2022). Synthesis of nanomaterials using various top-down and bottom-up approaches, influencing factors, advantages, and disadvantages: A review. Adv. Colloid Interface Sci. 300, 102597. doi:10.1016/j.cis.2021.102597
Abo-Zahra, S. F., Abdelmonem, I. M., Siyam, T. E., El-Masry, A. M., and Abdel-Aziz, H. M. (2022). Radiation synthesis of polyacrylamide/functionalized multiwalled carbon nanotubes composites for the adsorption of Cu(II) metal ions from aqueous solution. Polym. Bull. 79 (6), 4395–4415. doi:10.1007/s00289-021-03726-6
Abu Bakar, S., Jusoh, N., Mohamed, A., Muqoyyanah, M., Othman, M. H. D., Mamat, M. H., et al. (2021). Carbon nanotubes from waste cooking palm oil as adsorbent materials for the adsorption of heavy metal ions. Environ. Sci. Pollut. Res. 28 (46), 65171–65187. doi:10.1007/s11356-021-14918-y
Abualnaja, K. M., Alprol, A. E., Abu-Saied, M. A., Mansour, A. T., and Ashour, M. (2021). Studying the adsorptive behavior of poly(acrylonitrile-co-styrene) and carbon nanotubes (nanocomposites) impregnated with adsorbent materials towards methyl orange dye. Nanomaterials 11 (5), 1144. doi:10.3390/nano11051144
Abualnaja, K. M., Alprol, A. E., Ashour, M., and Mansour, A. T. (2021). Influencing multi-walled carbon nanotubes for the removal of ismate violet 2R dye from wastewater: Isotherm, kinetics, and thermodynamic studies. Appl. Sci. 11 (11), 4786. doi:10.3390/app11114786
Adeola, A. O., de Lange, J., and Forbes, P. B. C. (2021). Adsorption of antiretroviral drugs, efavirenz and nevirapine from aqueous solution by graphene wool: Kinetic, equilibrium, thermodynamic and computational studies. Appl. Surf. Sci. Adv. 6, 100157. doi:10.1016/j.apsadv.2021.100157
Adeola, A. O., and Forbes, P. B. C. (2021). Advances in water treatment technologies for removal of polycyclic aromatic hydrocarbons: Existing concepts, emerging trends, and future prospects. Water Environ. Res. 93 (3), 343–359. doi:10.1002/wer.1420
Adeola, A. O., and Forbes, P. B. C. (2021). Antiretroviral drugs in african surface waters: Prevalence, analysis, and potential remediation. Environ. Toxicol. Chem. 41, 247–262. doi:10.1002/etc.5127
Adeola, A. O., and Forbes, P. B. C. (2019). Optimization of the sorption of selected polycyclic aromatic hydrocarbons by regenerable graphene wool. Water Sci. Technol. 80 (10), 1931–1943. doi:10.2166/wst.2020.011
Adeola, A. O., Iwuozor, K. O., Akpomie, K. G., Adegoke, K. A., Oyedotun, K. O., Ighalo, J. O., et al. (2022). Advances in the management of radioactive wastes and radionuclide contamination in environmental compartments: A review. Environ. Geochem. Health 45, 2663–2689. doi:10.1007/s10653-022-01378-7
Adeola, A. O., Kubheka, G., Chirwa, E. M. N., and Forbes, P. B. C. (2021). Facile synthesis of graphene wool doped with oleylamine-capped silver nanoparticles (GW-αAgNPs) for water treatment applications. Appl. Water Sci. 11 (11), 172. doi:10.1007/s13201-021-01493-3
Adeola, A. O., Nsibande, S. A., Osano, A. M., Maghanga, J. K., Naudé, Y., and Forbes, P. B. C. (2022). Analysis of gaseous polycyclic aromatic hydrocarbon emissions from cooking devices in selected rural and urban kitchens in Bomet and Narok counties of Kenya. Environ. Monit. Assess. 194 (6), 435. doi:10.1007/s10661-022-10062-3
Agboola, O. D., and Benson, N. U. (2021). Physisorption and chemisorption mechanisms influencing micro (nano) plastics-organic chemical contaminants interactions: A review. Front. Environ. Sci. 9. doi:10.3389/fenvs.2021.678574
Ahmed, I., Hasan, Z., Lee, G., Lee, H. J., and Jhung, S. H. (2022). Contribution of hydrogen bonding to liquid-phase adsorptive removal of hazardous organics with metal-organic framework-based materials. Chem. Eng. J. 430, 132596. doi:10.1016/j.cej.2021.132596
Al-Hazmi, F. S., Al-Harbi, G. H., Beall, G. W., Al-Ghamdi, A. A., Obaid, A. Y., and Mahmoud, W. E. (2015). One pot synthesis of graphene based on microwave assisted solvothermal technique. Synth. Met. 200, 54–57. doi:10.1016/j.synthmet.2014.12.028
Alarfaj, N. A., El-Tohamy, M. F., and Oraby, H. F. (2018). CA 19-9 pancreatic tumor marker fluorescence immunosensing detection via immobilized carbon quantum dots conjugated gold nanocomposite. Int. J. Mol. Sci. 19 (4), 1162. doi:10.3390/ijms19041162
Alengebawy, A., Abdelkhalek, S. T., Qureshi, S. R., and Wang, M. Q. (2021). Heavy metals and pesticides toxicity in agricultural soil and plants: Ecological risks and human health implications. Toxics 9 (3), 42. doi:10.3390/toxics9030042
Algadri, N. A., Ibrahim, K., Hassan, Z., and Bououdina, M. (2017). Cost-effective single-step carbon nanotube synthesis using microwave oven. Mater. Res. Express 4 (8), 085602. doi:10.1088/2053-1591/aa817b
Almanassra, I. W., Kochkodan, V., McKay, G., Atieh, M. A., and Al-Ansari, T. (2021). Review of phosphate removal from water by carbonaceous sorbents. J. Environ. Manag. 287, 112245. doi:10.1016/j.jenvman.2021.112245
Alsaiari, N. S., Amari, A., Katubi, K. M., Alzahrani, F. M., Rebah, F. B., and Tahoon, M. A. (2022). The synthesis of magnetic nitrogen-doped graphene oxide nanocomposite for the removal of reactive orange 12 dye. Adsorpt. Sci. Technol. 2022, 1–14. doi:10.1155/2022/9417542
An, Z., Tang, W., Hawker, C. J., and Stucky, G. D. (2006). One-Step microwave preparation of well-defined and functionalized polymeric nanoparticles. J. Am. Chem. Soc. 128 (47), 15054–15055. doi:10.1021/ja065250f
Antonio De La, H., Jesús, A., José, C., María, A. H., Juan De, M. M., Pilar, P., et al. (2011). “Reproducibility and scalability of microwave-assisted reactions,” in Microwave heating. Editor C. Usha (Rijeka: IntechOpen). Ch. 7.
Apul, O. G., Wang, Q., Zhou, Y., and Karanfil, T. (2013). Adsorption of aromatic organic contaminants by graphene nanosheets: Comparison with carbon nanotubes and activated carbon. Water Res. 47 (4), 1648–1654. doi:10.1016/j.watres.2012.12.031
Arabkhani, P., Javadian, H., Asfaram, A., Sadeghfar, F., and Sadegh, F. (2021). Synthesis of magnetic tungsten disulfide/carbon nanotubes nanocomposite (WS2/Fe3O4/CNTs-NC) for highly efficient ultrasound-assisted rapid removal of amaranth and brilliant blue FCF hazardous dyes. J. Hazard. Mater. 420, 126644. doi:10.1016/j.jhazmat.2021.126644
Aravind Kumar, J., Krithiga, T., and Venkatesan, D. (2020). “Carbon nanotubes: Synthesis, properties and applications,” in 21st century surface science. Editors P. Phuong, G. Pratibha, K. Samir, and Y. Kavita (Rijeka: IntechOpen). Ch. 2.
Arora, N., and Sharma, N. N. (2014). Arc discharge synthesis of carbon nanotubes: Comprehensive review. Diam. Relat. Mater. 50, 135–150. doi:10.1016/j.diamond.2014.10.001
Arumugam, N., and Kim, J. (2018). Synthesis of carbon quantum dots from Broccoli and their ability to detect silver ions. Mater. Lett. 219, 37–40. doi:10.1016/j.matlet.2018.02.043
Asif, F. C., and Saha, G. C. (2023). Graphene-like carbon structure synthesis from biomass pyrolysis: A critical review on feedstock–process–properties relationship. C 9 (1), 31. doi:10.3390/c9010031
Aslam, M. M. A., Den, W., and Kuo, H-W. (2021). Encapsulated chitosan-modified magnetic carbon nanotubes for aqueous-phase CrVI uptake. J. Water Process Eng. 40, 101793. doi:10.1016/j.jwpe.2020.101793
Asnawi, M., Azhari, S., Hamidon, M. N., Ismail, I., and Helina, I. (2018). Synthesis of carbon nanomaterials from rice husk via microwave oven. J. Nanomater. 2018, 1–5. doi:10.1155/2018/2898326
Athika, M., Prasath, A., Duraisamy, E., Sankar Devi, V., Selva Sharma, A., and Elumalai, P. (2019). Carbon-quantum dots derived from denatured milk for efficient chromium-ion sensing and supercapacitor applications. Mater. Lett. 241, 156–159. doi:10.1016/j.matlet.2019.01.064
Awad, F. S., AbouZied, K. M., Bakry, A. M., Abou El-Maaty, W. M., El-Wakil, A. M., and El-Shall, M. S. (2021). Polyacrylonitrile modified partially reduced graphene oxide composites for the extraction of Hg(II) ions from polluted water. J. Mater. Sci. 56 (13), 7982–7999. doi:10.1007/s10853-021-05797-2
Bacon, M., Bradley, S. J., and Nann, T. (2014). Graphene quantum dots. Part. Part. Syst. Charact. 31 (4), 415–428. doi:10.1002/ppsc.201300252
Baghel, P., Sakhiya, A. K., and Kaushal, P. (2022). Ultrafast growth of carbon nanotubes using microwave irradiation: Characterization and its potential applications. Heliyon 8 (10), e10943. doi:10.1016/j.heliyon.2022.e10943
Baig, N., Kammakakam, I., and Falath, W. (2021). Nanomaterials: A review of synthesis methods, properties, recent progress, and challenges. Mater. Adv. 2 (6), 1821–1871. doi:10.1039/D0MA00807A
Bajpai, R., and Wagner, H. D. (2015). Fast growth of carbon nanotubes using a microwave oven. Carbon 82, 327–336. doi:10.1016/j.carbon.2014.10.077
Balarak, D., Mahdavi, Y., Maleki, A., Daraei, H., and Sadeghi, S. (2016). Studies on the removal of amoxicillin by single walled carbon nanotubes. Br. J. Pharm. Res. 10 (4), 1–9. doi:10.9734/BJPR/2016/24150
Balarak, D., and McKay, G. (2021). Utilization of MWCNTs/Al2O3 as adsorbent for ciprofloxacin removal: Equilibrium, kinetics and thermodynamic studies. J. Environ. Sci. Health, Part A. 56 (3), 324–333. doi:10.1080/10934529.2021.1873674
Balarak, D., Zafariyan, M., Igwegbe, C. A., Onyechi, K. K., and Ighalo, J. O. (2021). Adsorption of acid blue 92 dye from aqueous solutions by single-walled carbon nanotubes: Isothermal, kinetic, and thermodynamic studies. Environ. Process. 8 (2), 869–888. doi:10.1007/s40710-021-00505-3
Barrejón, M., and Prato, M. (2022). Carbon nanotube membranes in water treatment applications. Adv. Mater. Interfaces 9 (1), 2101260. doi:10.1002/admi.202101260
Bayantong, A. R. B., Shih, Y-J., Ong, D. C., Abarca, R. R. M., Dong, C-D., and de Luna, M. D. G. (2021). Adsorptive removal of dye in wastewater by metal ferrite-enabled graphene oxide nanocomposites. Chemosphere 274, 129518. doi:10.1016/j.chemosphere.2020.129518
Behi, M., Gholami, L., Naficy, S., Palomba, S., and Dehghani, F. (2022). Carbon dots: A novel platform for biomedical applications. Nanoscale Adv. 4 (2), 353–376. doi:10.1039/D1NA00559F
Bilecka, I., and Niederberger, M. (2010). Microwave chemistry for inorganic nanomaterials synthesis. Nanoscale 2 (8), 1358–1374. doi:10.1039/B9NR00377K
Bloor, J. M., Handy, R. D., Awan, S. A., and Jenkins, D. F. L. (2021). Graphene oxide biopolymer aerogels for the removal of lead from drinking water using a novel nano-enhanced ion exchange cascade. Ecotoxicol. Environ. Saf. 208, 111422. doi:10.1016/j.ecoenv.2020.111422
Buttress, A. J., Katrib, J., Jones, D. A., Batchelor, A. R., Craig, D. A., Royal, T. A., et al. (2017). Towards large scale microwave treatment of ores: Part 1 – basis of design, construction and commissioning. Miner. Eng. 109, 169–183. doi:10.1016/j.mineng.2017.03.006
Byrappa, K., and Adschiri, T. (2007). Hydrothermal technology for nanotechnology. Prog. Cryst. Growth Charact. Mater. 53 (2), 117–166. doi:10.1016/j.pcrysgrow.2007.04.001
Cai, M-Q., Su, J., Hu, J-Q., Wang, Q., Dong, C-Y., Pan, S-D., et al. (2016). Planar graphene oxide-based magnetic ionic liquid nanomaterial for extraction of chlorophenols from environmental water samples coupled with liquid chromatography–tandem mass spectrometry. J. Chromatogr. A 1459, 38–46. doi:10.1016/j.chroma.2016.06.086
Cao, Y., Taghvaie Nakhjiri, A., and Ghadiri, M. (2022). Membrane desalination for water treatment: Recent developments, techno-economic evaluation and innovative approaches toward water sustainability. Eur. Phys. J. Plus 137 (7), 763. doi:10.1140/epjp/s13360-022-02999-8
Chang, Y-P., Ren, C-L., Qu, J-C., and Chen, X-G. (2012). Preparation and characterization of Fe3O4/graphene nanocomposite and investigation of its adsorption performance for aniline and p-chloroaniline. Appl. Surf. Sci. 261, 504–509. doi:10.1016/j.apsusc.2012.08.045
Chen, D., Zhou, J., and Zhang, Q. (2014). Effects of torrefaction on the pyrolysis behavior and bio-oil properties of rice husk by using TG-FTIR and py-GC/MS. Energy & Fuels 28 (9), 5857–5863. doi:10.1021/ef501189p
Chen, R., Cheng, Y., Wang, P., Wang, Q., Wan, S., Huang, S., et al. (2021). Enhanced removal of Co(II) and Ni(II) from high-salinity aqueous solution using reductive self-assembly of three-dimensional magnetic fungal hyphal/graphene oxide nanofibers. Sci. Total Environ. 756, 143871. doi:10.1016/j.scitotenv.2020.143871
Chen, X., and Chen, B. (2015). Macroscopic and spectroscopic investigations of the adsorption of nitroaromatic compounds on graphene oxide, reduced graphene oxide, and graphene nanosheets. Environ. Sci. Technol. 49 (10), 6181–6189. doi:10.1021/es5054946
Chin, C. D., Treadwell, L. J., and Wiley, J. B. (2021). Microwave synthetic routes for shape-controlled catalyst nanoparticles and nanocomposites. Molecules 26 (12), 3647. doi:10.3390/molecules26123647
Cole, K. S., and Cole, R. H. (1941). Dispersion and absorption in dielectrics I. Alternating current characteristics. J. Chem. Phys. 9 (4), 341–351. doi:10.1063/1.1750906
da Silva Souza, D. R., Caminhas, L. D., de Mesquita, J. P., and Pereira, F. V. (2018). Luminescent carbon dots obtained from cellulose. Mater. Chem. Phys. 203, 148–155. doi:10.1016/j.matchemphys.2017.10.001
Das, L., Das, P., Bhowal, A., and Bhattachariee, C. (2020). Synthesis of hybrid hydrogel nano-polymer composite using Graphene oxide, Chitosan and PVA and its application in waste water treatment. Environ. Technol. Innovation 18, 100664. doi:10.1016/j.eti.2020.100664
Das, T. R., Patra, S., Madhuri, R., and Sharma, P. K. (2018). Bismuth oxide decorated graphene oxide nanocomposites synthesized via sonochemical assisted hydrothermal method for adsorption of cationic organic dyes. J. Colloid Interface Sci. 509, 82–93. doi:10.1016/j.jcis.2017.08.102
de Almeida, AdS. V., de Figueiredo Neves, T., da Silva, M. G. C., Prediger, P., and Vieira, M. G. A. (2022). Synthesis of a novel magnetic composite based on graphene oxide, chitosan and organoclay and its application in the removal of bisphenol A, 17α-ethinylestradiol and triclosan. J. Environ. Chem. Eng. 10 (1), 107071. doi:10.1016/j.jece.2021.107071
de Medeiros, T. V., Manioudakis, J., Noun, F., Macairan, J-R., Victoria, F., and Naccache, R. (2019). Microwave-assisted synthesis of carbon dots and their applications. J. Mater. Chem. C 7 (24), 7175–7195. doi:10.1039/C9TC01640F
Dehghani, M. H., Mohammadi, M., Mohammadi, M. A., Mahvi, A. H., Yetilmezsoy, K., Bhatnagar, A., et al. (2016). Equilibrium and kinetic studies of trihalomethanes adsorption onto multi-walled carbon nanotubes. Water, Air, & Soil Pollut. 227 (9), 332. doi:10.1007/s11270-016-3029-2
Dehghani, M. H., Niasar, Z. S., Mehrnia, M. R., Shayeghi, M., Al-Ghouti, M. A., Heibati, B., et al. (2017). Optimizing the removal of organophosphorus pesticide malathion from water using multi-walled carbon nanotubes. Chem. Eng. J. 310, 22–32. doi:10.1016/j.cej.2016.10.057
Deline, A. R., Frank, B. P., Smith, C. L., Sigmon, L. R., Wallace, A. N., Gallagher, M. J., et al. (2020). Influence of oxygen-containing functional groups on the environmental properties, transformations, and toxicity of carbon nanotubes. Chem. Rev. 120 (20), 11651–11697. doi:10.1021/acs.chemrev.0c00351
Deng, J., Shao, Y., Gao, N., Deng, Y., Tan, C., Zhou, S., et al. (2012). Multiwalled carbon nanotubes as adsorbents for removal of herbicide diuron from aqueous solution. Chem. Eng. J. 193-194, 339–347. doi:10.1016/j.cej.2012.04.051
Deng, J., You, Y., Sahajwalla, V., and Joshi, R. K. (2016). Transforming waste into carbon-based nanomaterials. Carbon 96, 105–115. doi:10.1016/j.carbon.2015.09.033
Deng, S., Zhang, Q., Nie, Y., Wei, H., Wang, B., Huang, J., et al. (2012). Sorption mechanisms of perfluorinated compounds on carbon nanotubes. Environ. Pollut. 168, 138–144. doi:10.1016/j.envpol.2012.03.048
Deng, Y., Ok, Y. S., Mohan, D., Pittman, C. U., and Dou, X. (2019). Carbamazepine removal from water by carbon dot-modified magnetic carbon nanotubes. Environ. Res. 169, 434–444. doi:10.1016/j.envres.2018.11.035
Deokar, S. K., Bajad, G. S., Bhonde, P., Vijayakumar, R. P., and Mandavgane, S. A. (2017). Adsorptive removal of diuron herbicide on carbon nanotubes synthesized from plastic waste. J. Polym. Environ. 25 (2), 165–175. doi:10.1007/s10924-016-0794-3
Devi, M., Rawat, S., and Sharma, S. (2021). A comprehensive review of the pyrolysis process: From carbon nanomaterial synthesis to waste treatment. Oxf. Open Mater. Sci. 1 (1). doi:10.1093/oxfmat/itab014
DeVierno Kreuder, A., House-Knight, T., Whitford, J., Ponnusamy, E., Miller, P., Jesse, N., et al. (2017). A method for assessing greener alternatives between chemical products following the 12 principles of green chemistry. ACS Sustain. Chem. Eng. 5 (4), 2927–2935. doi:10.1021/acssuschemeng.6b02399
Dong, Z., Wang, D., Liu, X., Pei, X., Chen, L., and Jin, J. (2014). Bio-inspired surface-functionalization of graphene oxide for the adsorption of organic dyes and heavy metal ions with a superhigh capacity. J. Mater. Chem. A 2 (14), 5034–5040. doi:10.1039/C3TA14751G
Duarte, M. P., Silva, R. C. F., Medeiros, T. P. V. D., Ardisson, J. D., Cotta, A. A. C., Naccache, R., et al. (2022). Carbon nanotubes derived from waste cooking oil for the removal of emerging contaminants. New J. Chem. 46 (23), 11315–11328. doi:10.1039/D2NJ01669A
Dudley, G. B., Richert, R., and Stiegman, A. E. (2015). On the existence of and mechanism for microwave-specific reaction rate enhancement. Chem. Sci. 6 (4), 2144–2152. doi:10.1039/C4SC03372H
Dutra, F. V. A., Pires, B. C., Nascimento, T. A., and Borges, K. B. (2018). Functional polyaniline/multiwalled carbon nanotube composite as an efficient adsorbent material for removing pharmaceuticals from aqueous media. J. Environ. Manag. 221, 28–37. doi:10.1016/j.jenvman.2018.05.051
Dutta, V., Devasia, J., Chauhan, A., Jha, A., Nizam, A., Lin, K. Y. A., et al. (2022). Photocatalytic nanomaterials: Applications for remediation of toxic polycyclic aromatic hydrocarbons and green management. Chem. Eng. J. Adv. 11, 100353. doi:10.1016/j.ceja.2022.100353
Dutta, V., Singh, P., Shandilya, P., Sharma, S., Raizada, P., Saini, A. K., et al. (2019). Review on advances in photocatalytic water disinfection utilizing graphene and graphene derivatives-based nanocomposites. J. Environ. Chem. Eng. 7 (3), 103132. doi:10.1016/j.jece.2019.103132
Dutta, V., Verma, R., Gopalkrishnan, C., Yuan, M-H., Batoo, K. M., Jayavel, R., et al. (2022). Bio-Inspired synthesis of carbon-based nanomaterials and their potential environmental applications: A state-of-the-art review. Inorganics 10 (10), 169. doi:10.3390/inorganics10100169
Egbosiuba, T. C., and Abdulkareem, A. S. (2021). Highly efficient as-synthesized and oxidized multi-walled carbon nanotubes for copper(II) and zinc(II) ion adsorption in a batch and fixed-bed process. J. Mater. Res. Technol. 15, 2848–2872. doi:10.1016/j.jmrt.2021.09.094
Egbosiuba, T. C., Abdulkareem, A. S., Tijani, J. O., Ani, J. I., Krikstolaityte, V., Srinivasan, M., et al. (2021). Taguchi optimization design of diameter-controlled synthesis of multi walled carbon nanotubes for the adsorption of Pb(II) and Ni(II) from chemical industry wastewater. Chemosphere 266, 128937. doi:10.1016/j.chemosphere.2020.128937
Egbosiuba, T. C., Egwunyenga, M. C., Tijani, J. O., Mustapha, S., Abdulkareem, A. S., Kovo, A. S., et al. (2022). Activated multi-walled carbon nanotubes decorated with zero valent nickel nanoparticles for arsenic, cadmium and lead adsorption from wastewater in a batch and continuous flow modes. J. Hazard. Mater. 423, 126993. doi:10.1016/j.jhazmat.2021.126993
El Hadki, A., Ulucan-Altuntas, K., El Hadki, H., Ustundag, C. B., Kabbaj, O. K., Dahchour, A., et al. (2021). Removal of oxytetracycline by graphene oxide and boron-doped reduced graphene oxide: A combined density function theory, molecular dynamics simulation and experimental study. FlatChem 27, 100238. doi:10.1016/j.flatc.2021.100238
El-Khawaga, A. M., Zidan, A., and El-Mageed, A. I. A. A. (2023). Preparation methods of different nanomaterials for various potential applications: A review. J. Mol. Struct. 1281, 135148. doi:10.1016/j.molstruc.2023.135148
Eltaweil, A. S., Mamdouh, I. M., Abd El-Monaem, E. M., and El-Subruiti, G. M. (2021). Highly efficient removal for methylene blue and Cu2+ onto UiO-66 metal–organic framework/carboxylated graphene oxide-incorporated sodium alginate beads. ACS Omega 6 (36), 23528–23541. doi:10.1021/acsomega.1c03479
Fan, L., Luo, C., Sun, M., Li, X., and Qiu, H. (2013). Highly selective adsorption of lead ions by water-dispersible magnetic chitosan/graphene oxide composites. Colloids Surfaces B Biointerfaces 103, 523–529. doi:10.1016/j.colsurfb.2012.11.006
Farghali, M. A., Abo-Aly, M. M., and Salaheldin, T. A. (2021). Modified mesoporous zeolite-A/reduced graphene oxide nanocomposite for dual removal of methylene blue and Pb2+ ions from wastewater. Inorg. Chem. Commun. 126, 108487. doi:10.1016/j.inoche.2021.108487
Fathy, N. A., Basta, A. H., and Lotfy, V. F. (2020). “4 - novel trends for synthesis of carbon nanostructures from agricultural wastes,” in Carbon nanomaterials for agri-food and environmental applications. Editor K. A. Abd-Elsalam (Elsevier), 59–74.
Favretto, L. (2003). Milestone's microwave labstation. Mol. Divers. 7 (2), 287–290. doi:10.1023/B:MODI.0000006906.47100.6b
Feng, M., Zhang, P., Zhou, H. C., and Sharma, V. K. (2018). Water-stable metal-organic frameworks for aqueous removal of heavy metals and radionuclides: A review. Chemosphere 209, 783–800. doi:10.1016/j.chemosphere.2018.06.114
Ferguson, J. D. (2003). Focused™ microwave instrumentation from CEM corporation. Mol. Divers. 7 (2), 281–286. doi:10.1023/B:MODI.0000006898.71413.eb
Forbes, P. (2021). Green sample preparation methods in the environmental monitoring of aquatic organic pollutants. Curr. Opin. Green Sustain. Chem. 31, 100500. doi:10.1016/j.cogsc.2021.100500
Foroutan, R., Peighambardoust, S. J., Esvandi, Z., Khatooni, H., and Ramavandi, B. (2021). Evaluation of two cationic dyes removal from aqueous environments using cnt/MgO/CuFe2O4 magnetic composite powder: A comparative study. J. Environ. Chem. Eng. 9 (2), 104752. doi:10.1016/j.jece.2020.104752
Fortugno, P., Musikhin, S., Shi, X., Wang, H., Wiggers, H., and Schulz, C. (2022). Synthesis of freestanding few-layer graphene in microwave plasma: The role of oxygen. Carbon 186, 560–573. doi:10.1016/j.carbon.2021.10.047
Fu, W., and Huang, Z. (2018). Magnetic dithiocarbamate functionalized reduced graphene oxide for the removal of Cu(II), Cd(II), Pb(II), and Hg(II) ions from aqueous solution: Synthesis, adsorption, and regeneration. Chemosphere 209, 449–456. doi:10.1016/j.chemosphere.2018.06.087
Fu, Y., Wang, J., Liu, Q., and Zeng, H. (2014). Water-dispersible magnetic nanoparticle–graphene oxide composites for selenium removal. Carbon 77, 710–721. doi:10.1016/j.carbon.2014.05.076
Gabriel, C., Gabriel, S., H Grant, E., H Grant, E., S J Halstead, B., and Michael P Mingos, D. (1998). Dielectric parameters relevant to microwave dielectric heating. Chem. Soc. Rev. 27 (3), 213–224. doi:10.1039/A827213Z
Gan, L., Shang, S., Hu, E., Yuen, C. W. M., and Jiang, S-X. (2015). Konjac glucomannan/graphene oxide hydrogel with enhanced dyes adsorption capability for methyl blue and methyl orange. Appl. Surf. Sci. 357, 866–872. doi:10.1016/j.apsusc.2015.09.106
Ge, R., Huo, T., Gao, Z., Li, J., and Zhan, X. (2023). GO-based membranes for desalination. Membranes 13 (2), 220. doi:10.3390/membranes13020220
Gedye, R., Smith, F., Westaway, K., Ali, H., Baldisera, L., Laberge, L., et al. (1986). The use of microwave ovens for rapid organic synthesis. Tetrahedron Lett. 27 (3), 279–282. doi:10.1016/S0040-4039(00)83996-9
Georgakilas, V., Perman, J. A., Tucek, J., and Zboril, R. (2015). Broad family of carbon nanoallotropes: Classification, chemistry, and applications of fullerenes, carbon dots, nanotubes, graphene, nanodiamonds, and combined superstructures. Chem. Rev. 115 (11), 4744–4822. doi:10.1021/cr500304f
Ghosh, S., Yadav, S., Devi, A., and Thomas, T. (2022). Techno-economic understanding of Indian energy-storage market: A perspective on green materials-based supercapacitor technologies. Renew. Sustain. Energy Rev. 161, 112412. doi:10.1016/j.rser.2022.112412
Gong, Y., Xie, L., Chen, C., Liu, J., Antonietti, M., and Wang, Y. (2023). Bottom-up hydrothermal carbonization for the precise engineering of carbon materials. Prog. Mater. Sci. 132, 101048. doi:10.1016/j.pmatsci.2022.101048
Goswami, A. D., Trivedi, D. H., Jadhav, N. L., and Pinjari, D. V. (2021). Sustainable and green synthesis of carbon nanomaterials: A review. J. Environ. Chem. Eng. 9 (5), 106118. doi:10.1016/j.jece.2021.106118
Gulati, S., Baul, A., Amar, A., Wadhwa, R., Kumar, S., and Varma, R. S. (2023). Eco-friendly and sustainable pathways to photoluminescent carbon quantum dots (CQDs). Nanomaterials 13, 554. doi:10.3390/nano13030554
Guo, D., Hu, Z., Li, Q., Bian, L., Song, Y., and Liu, X. (2022). Mixed-valence manganese oxide/reduced graphene oxide composites with enhanced pseudocapacitive performance. J. Mater. Sci. 57 (1), 563–575. doi:10.1007/s10853-021-06613-7
Guo, L., Ye, P., Wang, J., Fu, F., and Wu, Z. (2015). Three-dimensional Fe3O4-graphene macroscopic composites for arsenic and arsenate removal. J. Hazard. Mater. 298, 28–35. doi:10.1016/j.jhazmat.2015.05.011
Guo, S., Dai, Q., Wang, Z., and Yao, H. (2017). Rapid microwave irradiation synthesis of carbon nanotubes on graphite surface and its application on asphalt reinforcement. Compos. Part B Eng. 124, 134–143. doi:10.1016/j.compositesb.2017.05.033
Guo, X., Du, B., Wei, Q., Yang, J., Hu, L., Yan, L., et al. (2014). Synthesis of amino functionalized magnetic graphenes composite material and its application to remove Cr(VI), Pb(II), Hg(II), Cd(II) and Ni(II) from contaminated water. J. Hazard. Mater. 278, 211–220. doi:10.1016/j.jhazmat.2014.05.075
Gupta, K., and Khatri, O. P. (2017). Reduced graphene oxide as an effective adsorbent for removal of malachite green dye: Plausible adsorption pathways. J. Colloid Interface Sci. 501, 11–21. doi:10.1016/j.jcis.2017.04.035
Gupta, K., Yasa, S. R., Khan, A., Sharma, O. P., and Khatri, O. P. (2022). Charge-driven interaction for adsorptive removal of organic dyes using ionic liquid-modified graphene oxide. J. Colloid Interface Sci. 607, 1973–1985. doi:10.1016/j.jcis.2021.10.017
Gupta, V. K., and Saleh, T. A. (2013). Sorption of pollutants by porous carbon, carbon nanotubes and fullerene- an overview. Environ. Sci. Pollut. Res. 20 (5), 2828–2843. doi:10.1007/s11356-013-1524-1
Gusain, R., Kumar, N., and Ray, S. S. (2020). Recent advances in carbon nanomaterial-based adsorbents for water purification. Coord. Chem. Rev. 405, 213111. doi:10.1016/j.ccr.2019.213111
Han, R., Zhang, L., Song, C., Zhang, M., Zhu, H., and Zhang, L. (2010). Characterization of modified wheat straw, kinetic and equilibrium study about copper ion and methylene blue adsorption in batch mode. Carbohydr. Polym. 79 (4), 1140–1149. doi:10.1016/j.carbpol.2009.10.054
Hao, L., Song, H., Zhang, L., Wan, X., Tang, Y., and Lv, Y. (2012). SiO2/graphene composite for highly selective adsorption of Pb(II) ion. J. Colloid Interface Sci. 369 (1), 381–387. doi:10.1016/j.jcis.2011.12.023
Hashmi, A., Nayak, V., Singh, K. R. B., Jain, B., Baid, M., Alexis, F., et al. (2022). Potentialities of graphene and its allied derivatives to combat against SARS-CoV-2 infection. Mater. Today Adv. 13, 100208. doi:10.1016/j.mtadv.2022.100208
Hassan, H. M. A., Abdelsayed, V., Khder, A. E. R. S., AbouZeid, K. M., Terner, J., El-Shall, M. S., et al. (2009). Microwave synthesis of graphene sheets supporting metal nanocrystals in aqueous and organic media. J. Mater. Chem. 19 (23), 3832–3837. doi:10.1039/B906253J
Heidari, M., Dutta, A., Acharya, B., and Mahmud, S. (2019). A review of the current knowledge and challenges of hydrothermal carbonization for biomass conversion. J. Energy Inst. 92 (6), 1779–1799. doi:10.1016/j.joei.2018.12.003
Hemraj-Benny, T., Pimentel, L., and Emeran, G. (2020). formation of single-walled carbon nanotube-ruthenium nanoparticles in ethanol upon microwave radiation. Inorg. Chem. Commun. 112, 107707. doi:10.1016/j.inoche.2019.107707
Hernandez, Y., Nicolosi, V., Lotya, M., Blighe, F. M., Sun, Z., De, S., et al. (2008). High-yield production of graphene by liquid-phase exfoliation of graphite. Nat. Nanotechnol. 3 (9), 563–568. doi:10.1038/nnano.2008.215
Hidalgo, P., Navia, R., Hunter, R., Coronado, G., and Gonzalez, M. (2019). Synthesis of carbon nanotubes using biochar as precursor material under microwave irradiation. J. Environ. Manag. 244, 83–91. doi:10.1016/j.jenvman.2019.03.082
Hu, Q., He, Y., Wang, F., Wu, J., Ci, Z., Chen, L., et al. (2021). Microwave technology: A novel approach to the transformation of natural metabolites. Chin. Med. 16 (1), 87. doi:10.1186/s13020-021-00500-8
Hu, X., and Cheng, Z. (2015). Removal of diclofenac from aqueous solution with multi-walled carbon nanotubes modified by nitric acid. Chin. J. Chem. Eng. 23 (9), 1551–1556. doi:10.1016/j.cjche.2015.06.010
Hu, Y., Gao, Z., Yang, J., Chen, H., and Han, L. (2019). Environmentally benign conversion of waste polyethylene terephthalate to fluorescent carbon dots for “on-off-on” sensing of ferric and pyrophosphate ions. J. Colloid Interface Sci. 538, 481–488. doi:10.1016/j.jcis.2018.12.016
Huang, S., Jiang, S., Pang, H., Wen, T., Asiri, A. M., Alamry, K. A., et al. (2019). Dual functional nanocomposites of magnetic MnFe2O4 and fluorescent carbon dots for efficient U(VI) removal. Chem. Eng. J. 368, 941–950. doi:10.1016/j.cej.2019.03.015
Huang, Y., Gong, Y., Tang, J., and Xia, S. (2019). Effective removal of inorganic mercury and methylmercury from aqueous solution using novel thiol-functionalized graphene oxide/Fe-Mn composite. J. Hazard. Mater. 366, 130–139. doi:10.1016/j.jhazmat.2018.11.074
Huang, Z-W., Li, Z-J., Zheng, L-R., Wu, W-S., Chai, Z-F., and Shi, W-Q. (2019). Adsorption of Eu(III) and Th(IV) on three-dimensional graphene-based macrostructure studied by spectroscopic investigation. Environ. Pollut. 248, 82–89. doi:10.1016/j.envpol.2019.01.050
Huo, J., Yu, G., and Wang, J. (2021). Efficient removal of Co(II) and Sr(II) from aqueous solution using polyvinyl alcohol/graphene oxide/MnO2 composite as a novel adsorbent. J. Hazard. Mater. 411, 125117. doi:10.1016/j.jhazmat.2021.125117
Huo, J-B., Yu, G., and Wang, J. (2021). Adsorptive removal of Sr(II) from aqueous solution by polyvinyl alcohol/graphene oxide aerogel. Chemosphere 278, 130492. doi:10.1016/j.chemosphere.2021.130492
Huo, Y., Xiu, S., Meng, L-Y., and Quan, B. (2023). Solvothermal synthesis and applications of micro/nano carbons: A review. Chem. Eng. J. 451, 138572. doi:10.1016/j.cej.2022.138572
Hussain, K., Hoque, R. R., Balachandran, S., Medhi, S., Idris, M. G., Rahman, M., et al. (2018). “Monitoring and risk analysis of PAHs in the environment,” in Handbook of environmental materials management. Editor C. M. Hussain (Cham: Springer International Publishing), 1–35.
Ihsanullah, I., Khan, M. T., Zubair, M., Bilal, M., and Sajid, M. (2022). Removal of pharmaceuticals from water using sewage sludge-derived biochar: A review. Chemosphere 289, 133196. doi:10.1016/j.chemosphere.2021.133196
Ihsanullah, I., Sajid, M., Kabeer, M., Shemsi, A. M., and Atieh, M. A. (2020). First investigations on the removal of tungsten species from water using multi-walled carbon nanotubes. Water, Air, & Soil Pollut. 231 (3), 119. doi:10.1007/s11270-020-04485-2
Iijima, S. (1991). Helical microtubules of graphitic carbon. Nature 354 (6348), 56–58. doi:10.1038/354056a0
Ijaz, I., Gilani, E., Nazir, A., and Bukhari, A. (2020). Detail review on chemical, physical and green synthesis, classification, characterizations and applications of nanoparticles. Green Chem. Lett. Rev. 13 (3), 223–245. doi:10.1080/17518253.2020.1802517
Indrawirawan, S., Sun, H., Duan, X., and Wang, S. (2015). Nanocarbons in different structural dimensions (0–3D) for phenol adsorption and metal-free catalytic oxidation. Appl. Catal. B Environ. 179, 352–362. doi:10.1016/j.apcatb.2015.05.049
Ion, I., Bogdan, D., Mincu, M. M., and Ion, A. C. (2021). Modified exfoliated carbon nanoplatelets as sorbents for ammonium from natural mineral waters. Molecules 26 (12), 3541. doi:10.3390/molecules26123541
Isaeva, V. I., Vedenyapina, M. D., Kurmysheva, A. Y., Weichgrebe, D., Nair, R. R., Nguyen, N. P. T., et al. (2021). Modern carbon-based materials for adsorptive removal of organic and inorganic pollutants from water and wastewater. Molecules 26 (21), 6628. doi:10.3390/molecules26216628
Jašek, O., Eliáš, M., Zajíčková, L., Kudrle, V., Bublan, M., Matějková, J., et al. (2006). Carbon nanotubes synthesis in microwave plasma torch at atmospheric pressure. Mater. Sci. Eng. C 26 (5), 1189–1193. doi:10.1016/j.msec.2005.09.024
Jiang, Q., Zhang, M., and Mujumdar, A. S. (2023). Application of physical field-assisted freezing and thawing to mitigate damage to frozen food. J. Sci. Food Agric. 103 (5), 2223–2238. doi:10.1002/jsfa.12260
Jiang, W-S., Yang, C., Chen, G-X., Yan, X-Q., Chen, S-N., Su, B-W., et al. (2018). Preparation of high-quality graphene using triggered microwave reduction under an air atmosphere. J. Mater. Chem. C 6 (7), 1829–1835. doi:10.1039/C7TC03957C
Jlassi, K., Eid, K., Sliem, M. H., Abdullah, A. M., Chehimi, M. M., and Krupa, I. (2020). Rational synthesis, characterization, and application of environmentally friendly (polymer–carbon dot) hybrid composite film for fast and efficient UV-assisted Cd2+ removal from water. Environ. Sci. Eur. 32 (1), 12. doi:10.1186/s12302-020-0292-z
Joshi, P. N., Mathias, A., and Mishra, A. (2018). Synthesis of ecofriendly fluorescent carbon dots and their biomedical and environmental applications. Mater. Technol. 33 (10), 672–680. doi:10.1080/10667857.2018.1492683
Kahraman, S., Canpolat, A. N., and Fener, M. (2020). The influence of microwave treatment on the compressive and tensile strength of igneous rocks. Int. J. Rock Mech. Min. Sci. 129, 104303. doi:10.1016/j.ijrmms.2020.104303
Kang, Y., Yu, X., Kota, M., and Park, H. S. (2017). Carbon nanotubes branched on three-dimensional, nitrogen-incorporated reduced graphene oxide/iron oxide hybrid architectures for lithium ion battery anode. J. Alloys Compd. 726, 88–94. doi:10.1016/j.jallcom.2017.07.264
Kappe, (2012). Microwave theory. Microwaves in organic and medicinal chemistry. Methods and principles in medicinal chemistry, 9–39.
Kerkez-Kuyumcu, Ö., Bayazit, Ş. S., and Salam, M. A. (2016). Antibiotic amoxicillin removal from aqueous solution using magnetically modified graphene nanoplatelets. J. Industrial Eng. Chem. 36, 198–205. doi:10.1016/j.jiec.2016.01.040
Khan, Z. U., Khan, W. U., Ullah, B., Ali, W., Ahmad, B., Ali, W., et al. (2021). Graphene oxide/PVC composite papers functionalized with p-Phenylenediamine as high-performance sorbent for the removal of heavy metal ions. J. Environ. Chem. Eng. 9 (5), 105916. doi:10.1016/j.jece.2021.105916
Kharissova, O. V., Kharisov, B. I., Oliva González, C. M., Méndez, Y. P., and López, I. (2019). Greener synthesis of chemical compounds and materials. R. Soc. Open Sci. 6 (11), 191378. doi:10.1098/rsos.191378
Khenfouch, M., Minnis Ndimba, R., Diallo, A., Khamlich, S., Hamzah, M., Dhlamini, M. S., et al. (2016). Artemisia herba-alba asso eco-friendly reduced few-layered graphene oxide nanosheets: Structural investigations and physical properties. Green Chem. Lett. Rev. 9 (2), 122–131. doi:10.1080/17518253.2016.1181791
Kong, L., Wang, Y., Andrews, C. B., and Zheng, C. (2022). One-step construction of hierarchical porous channels on electrospun MOF/polymer/graphene oxide composite nanofibers for effective arsenate removal from water. Chem. Eng. J. 435, 134830. doi:10.1016/j.cej.2022.134830
Kroto, H. W., Heath, J. R., O’Brien, S. C., Curl, R. F., and Smalley, R. E. (1985). C60: Buckminsterfullerene. Nature 318 (6042), 162–163. doi:10.1038/318162a0
Kubheka, G., Adeola, A. O., and Forbes, P. B. C. (2022). Hexadecylamine functionalised graphene quantum dots as suitable nano-adsorbents for phenanthrene removal from aqueous solution. RSC Adv. 12 (37), 23922–23936. doi:10.1039/D2RA04641E
Kumar, D., Raghavan, C. M., Sridhar, C., Shin, J-H., Ryu, S. H., Jang, K., et al. (2015). Microwave-assisted synthesis, characterization of reduced graphene oxide, and its antibacterial activity. Bull. Korean Chem. Soc. 36 (8), 2034–2038. doi:10.1002/bkcs.10394
Kumar, R., Singh, R. K., Singh, D. P., Vaz, A. R., Yadav, R. R., Rout, C. S., et al. (2017). Synthesis of self-assembled and hierarchical palladium-CNTs-reduced graphene oxide composites for enhanced field emission properties. Mater. Des. 122, 110–117. doi:10.1016/j.matdes.2017.02.089
Lazarević-Pašti, T., Anićijević, V., Baljozović, M., Anićijević, D. V., Gutić, S., Vasić, V., et al. (2018). The impact of the structure of graphene-based materials on the removal of organophosphorus pesticides from water. Environ. Sci. Nano 5 (6), 1482–1494. doi:10.1039/C8EN00171E
Lee, Y-C., and Yang, J-W. (2012). Self-assembled flower-like TiO2 on exfoliated graphite oxide for heavy metal removal. J. Industrial Eng. Chem. 18 (3), 1178–1185. doi:10.1016/j.jiec.2012.01.005
Lei, Y., Huang, Q., Dou, J., Huang, H., Yang, G., Deng, F., et al. (2021). Fast adsorptive removal of cationic organic dye by anionic group functionalized carbon nanotubes with high efficiency. Colloid Interface Sci. Commun. 40, 100328. doi:10.1016/j.colcom.2020.100328
Li, B., Fan, X., Yu, S., Xia, H., Nong, Y., Bian, J., et al. (2023). Microwave heating of biomass waste residues for sustainable bioenergy and biomass materials preparation: A parametric simulation study. Energy 274, 127347. doi:10.1016/j.energy.2023.127347
Li, G., Zhang, J., Li, Y., Liu, J., and Yan, Z. (2021). Adsorption characteristics of Pb(II), Cd(II) and Cu(II) on carbon nanotube-hydroxyapatite. Environ. Technol. 42 (10), 1560–1581. doi:10.1080/09593330.2019.1674385
Li, H-Y., Li, D., Guo, Y., Yang, Y., Wei, W., and Xie, B. (2018). On-site chemosensing and quantification of Cr(VI) in industrial wastewater using one-step synthesized fluorescent carbon quantum dots. Sensors Actuators B Chem. 277, 30–38. doi:10.1016/j.snb.2018.08.157
Li, J., Jiang, N., Cheng, C., Quan, G., Feng, H., Li, W., et al. (2023). Preparation of magnetic solvent-free carbon nanotube/Fe3O4 nanofluid sizing agent to enhance thermal conductivity and interfacial properties of carbon fiber composites. Compos. Sci. Technol. 236, 109980. doi:10.1016/j.compscitech.2023.109980
Li, Z., Chen, F., Yuan, L., Liu, Y., Zhao, Y., Chai, Z., et al. (2012). Uranium(VI) adsorption on graphene oxide nanosheets from aqueous solutions. Chem. Eng. J. 210, 539–546. doi:10.1016/j.cej.2012.09.030
Li, Z., Yao, Y., Lin, Z., Moon, K-S., Lin, W., and Wong, C. (2010). Ultrafast, dry microwave synthesis of graphene sheets. J. Mater. Chem. 20 (23), 4781–4783. doi:10.1039/C0JM00168F
Liang, W., Wang, B., Cheng, J., Xiao, D., Xie, Z., and Zhao, J. (2021). 3D, eco-friendly metal-organic frameworks@carbon nanotube aerogels composite materials for removal of pesticides in water. J. Hazard. Mater. 401, 123718. doi:10.1016/j.jhazmat.2020.123718
Lin, H., Duan, Y., Zhao, B., Feng, Q., Li, M., Wei, J., et al. (2022). Efficient Hg(II) removal to ppb level from water in wider pH based on poly-cyanoguanidine/graphene oxide: Preparation, behaviors, and mechanisms. Colloids Surfaces A Physicochem. Eng. Aspects 641, 128467. doi:10.1016/j.colsurfa.2022.128467
Lin, S., Zou, C., Liang, H., Peng, H., and Liao, Y. (2021). The effective removal of nickel ions from aqueous solution onto magnetic multi-walled carbon nanotubes modified by β-cyclodextrin. Colloids Surfaces A Physicochem. Eng. Aspects 619, 126544. doi:10.1016/j.colsurfa.2021.126544
Lin, X., Xu, Q., Gan, L., Owens, G., and Chen, Z. (2022). Cyclodextrin modified green synthesized graphene oxide@iron nanoparticle composites for enhanced removal of oxytetracycline. J. Colloid Interface Sci. 608, 3159–3167. doi:10.1016/j.jcis.2021.11.049
Liu, G., Li, L., Huang, X., Zheng, S., Xu, X., Liu, Z., et al. (2018). Adsorption and removal of organophosphorus pesticides from environmental water and soil samples by using magnetic multi-walled carbon nanotubes @ organic framework ZIF-8. J. Mater. Sci. 53 (15), 10772–10783. doi:10.1007/s10853-018-2352-y
Liu, G., Li, L., Xu, D., Huang, X., Xu, X., Zheng, S., et al. (2017). Metal–organic framework preparation using magnetic graphene oxide–β-cyclodextrin for neonicotinoid pesticide adsorption and removal. Carbohydr. Polym. 175, 584–591. doi:10.1016/j.carbpol.2017.06.074
Liu, H., Ruan, W., Zhang, Z., Zhou, Y., Shen, F., Liu, J., et al. (2022). Performance and mechanism of CuS-modified MWCNTs on mercury removal: Experimental and density functional theory study. Fuel 309, 122238. doi:10.1016/j.fuel.2021.122238
Liu, L., Li, C., Bao, C., Jia, Q., Xiao, P., Liu, X., et al. (2012). Preparation and characterization of chitosan/graphene oxide composites for the adsorption of Au(III) and Pd(II). Talanta 93, 350–357. doi:10.1016/j.talanta.2012.02.051
Liu, L., Liu, S., Zhang, Q., Li, C., Bao, C., Liu, X., et al. (2013). Adsorption of Au(III), Pd(II), and Pt(IV) from aqueous solution onto graphene oxide. J. Chem. Eng. Data 58 (2), 209–216. doi:10.1021/je300551c
Liu, W-W., Aziz, A., Chai, S-P., Mohamed, A. R., and Tye, C-T. (2011). The effect of carbon precursors (methane, benzene and camphor) on the quality of carbon nanotubes synthesised by the chemical vapour decomposition. Phys. E Low-dimensional Syst. Nanostructures 43 (8), 1535–1542. doi:10.1016/j.physe.2011.05.012
Liu, X., Guo, Y., Zhang, C., Huang, X., Ma, K., and Zhang, Y. (2022). Preparation of graphene oxide/4A molecular sieve composite and evaluation of adsorption performance for Rhodamine B. Sep. Purif. Technol. 286, 120400. doi:10.1016/j.seppur.2021.120400
Liu, X., Li, J., Luo, Y., Li, J., Wei, Z., Cao, J., et al. (2023). Efficient carbon nanotube growth from pyrolysis of citric acid-based small organic molecules. Carbon Trends 10, 100236. doi:10.1016/j.cartre.2022.100236
Liu, Y., Guo, N., Yin, P., and Zhang, C. (2019). Facile growth of carbon nanotubes using microwave ovens: The emerging application of highly efficient domestic plasma reactors. Nanoscale Adv. 1 (12), 4546–4559. doi:10.1039/C9NA00538B
Liu, Y., Yu, J., Guo, D., Li, Z., and Su, Y. (2020). Ti3C2Tx MXene/graphene nanocomposites: Synthesis and application in electrochemical energy storage. J. Alloys Compd. 815, 152403. doi:10.1016/j.jallcom.2019.152403
Lu, C., Chung, Y-L., and Chang, K-F. (2005). Adsorption of trihalomethanes from water with carbon nanotubes. Water Res. 39 (6), 1183–1189. doi:10.1016/j.watres.2004.12.033
Luo, P. G., Sahu, S., Yang, S-T., Sonkar, S. K., Wang, J., Wang, H., et al. (2013). Carbon “quantum” dots for optical bioimaging. J. Mater. Chem. B 1 (16), 2116–2127. doi:10.1039/C3TB00018D
Luo, X., Wang, C., Wang, L., Deng, F., Luo, S., Tu, X., et al. (2013). Nanocomposites of graphene oxide-hydrated zirconium oxide for simultaneous removal of As(III) and As(V) from water. Chem. Eng. J. 220, 98–106. doi:10.1016/j.cej.2013.01.017
Lv, X., Xue, X., Jiang, G., Wu, D., Sheng, T., Zhou, H., et al. (2014). Nanoscale Zero-Valent Iron (nZVI) assembled on magnetic Fe3O4/graphene for Chromium (VI) removal from aqueous solution. J. Colloid Interface Sci. 417, 51–59. doi:10.1016/j.jcis.2013.11.044
Macairan, J-R., Jaunky, D. B., Piekny, A., and Naccache, R. (2019). Intracellular ratiometric temperature sensing using fluorescent carbon dots. Nanoscale Adv. 1 (1), 105–113. doi:10.1039/C8NA00255J
Madadrang, C. J., Kim, H. Y., Gao, G., Wang, N., Zhu, J., Feng, H., et al. (2012). Adsorption behavior of EDTA-graphene oxide for Pb (II) removal. ACS Appl. Mater. Interfaces 4 (3), 1186–1193. doi:10.1021/am201645g
Mahmoud, M. E., Amira, M. F., Azab, M. M. H. M., and Abdelfattah, A. M. (2022). An innovative amino-magnetite@graphene oxide@amino-manganese dioxide as a nitrogen-rich nanocomposite for removal of Congo red dye. Diam. Relat. Mater. 121, 108744. doi:10.1016/j.diamond.2021.108744
Maitlo, H. A., Kim, K-H., Kumar, V., Kim, S., and Park, J-W. (2019). Nanomaterials-based treatment options for chromium in aqueous environments. Environ. Int. 130, 104748. doi:10.1016/j.envint.2019.04.020
Mallakpour, S., Behranvand, V., and Mallakpour, F. (2021). Adsorptive performance of alginate/carbon nanotube-carbon dot-magnesium fluorohydroxyapatite hydrogel for methylene blue-contaminated water. J. Environ. Chem. Eng. 9 (2), 105170. doi:10.1016/j.jece.2021.105170
Mallakpour, S., and Tabesh, F. (2021). Green and plant-based adsorbent from tragacanth gum and carboxyl-functionalized carbon nanotube hydrogel bionanocomposite for the super removal of methylene blue dye. Int. J. Biol. Macromol. 166, 722–729. doi:10.1016/j.ijbiomac.2020.10.229
Manawi, Y. M., Ihsanullah, Samara A., Samara, A., Al-Ansari, T., and Atieh, M. (2018). A review of carbon nanomaterials’ synthesis via the chemical vapor deposition (CVD) method. Materials 11 (5), 822. doi:10.3390/ma11050822
Mandani, S., Dey, D., Sharma, B., and Sarma, T. K. (2017). Natural occurrence of fluorescent carbon dots in honey. Carbon 119, 569–572. doi:10.1016/j.carbon.2017.04.075
Mashkani, M., Mehdinia, A., Jabbari, A., Bide, Y., and Nabid, M. R. (2018). Preconcentration and extraction of lead ions in vegetable and water samples by N-doped carbon quantum dot conjugated with Fe3O4 as a green and facial adsorbent. Food Chem. 239, 1019–1026. doi:10.1016/j.foodchem.2017.07.042
Mingos, D. M. P., and Baghurst, D. R. (1991). Tilden Lecture. Applications of microwave dielectric heating effects to synthetic problems in chemistry. Chem. Soc. Rev. 20 (1), 1–47. doi:10.1039/CS9912000001
Mishra, A. K., and Ramaprabhu, S. (2011). Functionalized graphene sheets for arsenic removal and desalination of sea water. Desalination 282, 39–45. doi:10.1016/j.desal.2011.01.038
Mishra, R. R., and Sharma, A. K. (2016). Microwave–material interaction phenomena: Heating mechanisms, challenges and opportunities in material processing. Compos. Part A Appl. Sci. Manuf. 81, 78–97. doi:10.1016/j.compositesa.2015.10.035
Mitra, S., Chakraborty, A. J., Tareq, A. M., Emran, T. B., Nainu, F., Khusro, A., et al. (2022). Impact of heavy metals on the environment and human health: Novel therapeutic insights to counter the toxicity. J. King Saud Univ. - Sci. 34 (3), 101865. doi:10.1016/j.jksus.2022.101865
Mohammadi, A., Kazemipour, M., Ranjbar, H., Walker, R. B., and Ansari, M. (2015). Amoxicillin removal from aqueous media using multi-walled carbon nanotubes. Fullerenes, Nanotub. Carbon Nanostructures 23 (2), 165–169. doi:10.1080/1536383X.2013.866944
Mohammadi, A. A., Dehghani, M. H., Mesdaghinia, A., Yaghmaian, K., and Es'haghi, Z. (2020). Adsorptive removal of endocrine disrupting compounds from aqueous solutions using magnetic multi-wall carbon nanotubes modified with chitosan biopolymer based on response surface methodology: Functionalization, kinetics, and isotherms studies. Int. J. Biol. Macromol. 155, 1019–1029. doi:10.1016/j.ijbiomac.2019.11.065
Mohapatra, L., Cheon, D., and Yoo, S. H. (2023). Carbon-based nanomaterials for catalytic wastewater treatment: A review. Molecules 28 (4), 1805. doi:10.3390/molecules28041805
Monte-Filho, S. S., Andrade, S. I. E., Lima, M. B., and Araujo, M. C. U. (2019). Synthesis of highly fluorescent carbon dots from lemon and onion juices for determination of riboflavin in multivitamin/mineral supplements. J. Pharm. Analysis 9 (3), 209–216. doi:10.1016/j.jpha.2019.02.003
Motasemi, F., and Ani, F. N. (2012). A review on microwave-assisted production of biodiesel. Renew. Sustain. Energy Rev. 16 (7), 4719–4733. doi:10.1016/j.rser.2012.03.069
Mou, Z., Zhang, H., Liu, Z., Sun, J., and Zhu, M. (2019). Ultrathin BiOCl/nitrogen-doped graphene quantum dots composites with strong adsorption and effective photocatalytic activity for the degradation of antibiotic ciprofloxacin. Appl. Surf. Sci. 496, 143655. doi:10.1016/j.apsusc.2019.143655
Mpongwana, N., and Rathilal, S. (2022). A review of the techno-economic feasibility of nanoparticle application for wastewater treatment. Water 14 (10), 1550. doi:10.3390/w14101550
Naghizadeh, A., Karimi, A., Derakhshani, E., and Esform, A. (2022). Single-walled carbon nanotubes (SWCNTs) as an efficient adsorbent for removal of reactive dyes from water solution: Equilibrium, kinetic, and thermodynamic. Environ. Qual. Manag. 31 (4), 133–140. doi:10.1002/tqem.21753
Nassar, N. N., Marei, N. N., Vitale, G., and Arar, L. A. (2015). Adsorptive removal of dyes from synthetic and real textile wastewater using magnetic iron oxide nanoparticles: Thermodynamic and mechanistic insights. Can. J. Chem. Eng. 93 (11), 1965–1974. doi:10.1002/cjce.22315
Nithya Priya, V., Rajkumar, M., Mobika, J., and Linto Sibi, S. P. (2021). Alginate coated layered double hydroxide/reduced graphene oxide nanocomposites for removal of toxic as (V) from wastewater. Phys. E Low-dimensional Syst. Nanostructures 127, 114527. doi:10.1016/j.physe.2020.114527
Noreen, S., Tahira, M., Ghamkhar, M., Hafiz, I., Bhatti, H. N., Nadeem, R., et al. (2021). Treatment of textile wastewater containing acid dye using novel polymeric graphene oxide nanocomposites (GO/PAN,GO/PPy, GO/PSty). J. Mater. Res. Technol. 14, 25–35. doi:10.1016/j.jmrt.2021.06.007
Osterberg, M., Henn, K. A., Farooq, M., and Valle-Delgado, J. J. (2023). Biobased Nanomaterials─The role of interfacial interactions for advanced materials. Chem. Rev. 123 (5), 2200–2241. doi:10.1021/acs.chemrev.2c00492
Oladipo, A. A. (2018). “14 - microwave-assisted synthesis of high-performance polymer-based nanoadsorbents for pollution control,” in New polymer nanocomposites for environmental remediation. Editors C. M. Hussain, and A. K. Mishra (Elsevier), 337–359.
Oliveira, A. R., Correia, A. A., and Rasteiro, M. G. (2021). Heavy metals removal from aqueous solutions by multiwall carbon nanotubes: Effect of MWCNTs dispersion. Nanomaterials 11 (8), 2082. doi:10.3390/nano11082082
Omoriyekomwan, J. E., Tahmasebi, A., Dou, J., Wang, R., and Yu, J. (2021). A review on the recent advances in the production of carbon nanotubes and carbon nanofibers via microwave-assisted pyrolysis of biomass. Fuel Process. Technol. 214, 106686. doi:10.1016/j.fuproc.2020.106686
Omoriyekomwan, J. E., Tahmasebi, A., Zhang, J., and Yu, J. (2019). Mechanistic study on direct synthesis of carbon nanotubes from cellulose by means of microwave pyrolysis. Energy Convers. Manag. 192, 88–99. doi:10.1016/j.enconman.2019.04.042
Ore, O. T., and Adeola, A. O. (2021). Toxic metals in oil sands: Review of human health implications, environmental impact, and potential remediation using membrane-based approach. Energy, Ecol. Environ. 6 (2), 81–91. doi:10.1007/s40974-020-00196-w
Ortega-Cervantez, G., Gómez-Aguilar, R., Rueda-Morales, G., and Ortiz-López, J. (2016). Microwave-assisted synthesis of sponge-like carbon nanotube arrays and their application in organic transistor devices. J. Mater. Sci. Mater. Electron. 27 (12), 12642–12648. doi:10.1007/s10854-016-5397-1
Papi, M. (2021). Graphene-based materials: Biological and biomedical applications. Int. J. Mol. Sci. 22 (2), 672. doi:10.3390/ijms22020672
Perumal, S., Atchudan, R., Thirukumaran, P., Yoon, D. H., Lee, Y. R., and Cheong, I. W. (2022). Simultaneous removal of heavy metal ions using carbon dots-doped hydrogel particles. Chemosphere 286, 131760. doi:10.1016/j.chemosphere.2021.131760
Pete, S., Kattil, R. A., and Thomas, L. (2021). Polyaniline-multiwalled carbon nanotubes (PANI- MWCNTs) composite revisited: An efficient and reusable material for methyl orange dye removal. Diam. Relat. Mater. 117, 108455. doi:10.1016/j.diamond.2021.108455
Pokharel, P., Truong, Q-T., and Lee, D. S. (2014). Multi-step microwave reduction of graphite oxide and its use in the formation of electrically conductive graphene/epoxy composites. Compos. Part B Eng. 64, 187–193. doi:10.1016/j.compositesb.2014.04.013
Pyrzynska, K. (2023). Recent applications of carbon nanotubes for separation and enrichment of lead ions. Separations 10 (3), 152. doi:10.3390/separations10030152
Qian, J., Martinez, A., Marek, R. F., Nagorzanski, M. R., Zhi, H., Furlong, E. T., et al. (2020). Polymeric nanofiber-carbon nanotube composite mats as fast-equilibrium passive samplers for polar organic contaminants. Environ. Sci. Technol. 54 (11), 6703–6712. doi:10.1021/acs.est.0c00609
Rafael Zamorano, U., MaGuadalupe Hernandez, S., and Veronica, L. V. R. (2019). “The interaction of microwaves with materials of different properties,” in Electromagnetic fields and waves. Editors Y. Kim Ho, and H. Kazuhiro (Rijeka: IntechOpen). Ch. 6.
Rahmanian, O., Dinari, M., and Abdolmaleki, M. K. (2018). Carbon quantum dots/layered double hydroxide hybrid for fast and efficient decontamination of Cd(II): The adsorption kinetics and isotherms. Appl. Surf. Sci. 428, 272–279. doi:10.1016/j.apsusc.2017.09.152
Ramar, V., Moothattu, S., and Balasubramanian, K. (2018). Metal free, sunlight and white light based photocatalysis using carbon quantum dots from citrus grandis: A green way to remove pollution. Sol. Energy 169, 120–127. doi:10.1016/j.solener.2018.04.040
Ramezanzadeh, M., Asghari, M., Ramezanzadeh, B., and Bahlakeh, G. (2018). Fabrication of an efficient system for Zn ions removal from industrial wastewater based on graphene oxide nanosheets decorated with highly crystalline polyaniline nanofibers (GO-PANI): Experimental and ab initio quantum mechanics approaches. Chem. Eng. J. 337, 385–397. doi:10.1016/j.cej.2017.12.102
Robinson, J., Kingman, S., Irvine, D., Licence, P., Smith, A., Dimitrakis, G., et al. (2010). Electromagnetic simulations of microwave heating experiments using reaction vessels made out of silicon carbide. Phys. Chem. Chem. Phys. 12 (36), 10793–10800. doi:10.1039/C0CP00080A
Robinson, J., Kingman, S., Irvine, D., Licence, P., Smith, A., Dimitrakis, G., et al. (2010). Understanding microwave heating effects in single mode type cavities—Theory and experiment. Phys. Chem. Chem. Phys. 12 (18), 4750–4758. doi:10.1039/B922797K
Sabet, M., and Mahdavi, K. (2019). Green synthesis of high photoluminescence nitrogen-doped carbon quantum dots from grass via a simple hydrothermal method for removing organic and inorganic water pollutions. Appl. Surf. Sci. 463, 283–291. doi:10.1016/j.apsusc.2018.08.223
Sabzehmeidani, M. M., Mahnaee, S., Ghaedi, M., Heidari, H., and Roy, V. A. L. (2021). Carbon based materials: A review of adsorbents for inorganic and organic compounds. Mater. Adv. 2 (2), 598–627. doi:10.1039/D0MA00087F
Saji, V. S. (2021). Carbon nanostructure-based superhydrophobic surfaces and coatings. Nanotechnol. Rev. 10 (1), 518–571. doi:10.1515/ntrev-2021-0039
Sajid, M., Asif, M., Baig, N., Kabeer, M., Ihsanullah, I., and Mohammad, A. W. (2022). Carbon nanotubes-based adsorbents: Properties, functionalization, interaction mechanisms, and applications in water purification. J. Water Process Eng. 47, 102815. doi:10.1016/j.jwpe.2022.102815
Sajid, M., Nazal, M. K., Ihsanullah, Baig N., Baig, N., and Osman, A. M. (2018). Removal of heavy metals and organic pollutants from water using dendritic polymers based adsorbents: A critical review. Sep. Purif. Technol. 191, 400–423. doi:10.1016/j.seppur.2017.09.011
Saleh, T. A., Elsharif, A. M., and Bin-Dahman, O. A. (2021). Synthesis of amine functionalization carbon nanotube-low symmetry porphyrin derivatives conjugates toward dye and metal ions removal. J. Mol. Liq. 340, 117024. doi:10.1016/j.molliq.2021.117024
Samuel, M. S., Shah, S. S., Bhattacharya, J., Subramaniam, K., and Pradeep Singh, N. D. (2018). Adsorption of Pb(II) from aqueous solution using a magnetic chitosan/graphene oxide composite and its toxicity studies. Int. J. Biol. Macromol. 115, 1142–1150. doi:10.1016/j.ijbiomac.2018.04.185
Sang, M., Shin, J., Kim, K., and Yu, K. J. (2019). Electronic and thermal properties of graphene and recent advances in graphene based electronics applications. Nanomater. (Basel) 9 (3), 374. doi:10.3390/nano9030374
Saputri, D. D., Jan’ah, A. M., and Saraswati, T. E. (2020). Synthesis of carbon nanotubes (cnt) by chemical vapor deposition (CVD) using a biogas-based carbon precursor: A review. IOP Conf. Ser. Mater. Sci. Eng. 959 (1), 012019. doi:10.1088/1757-899X/959/1/012019
Sari Yilmaz, M. (2022). Graphene oxide/hollow mesoporous silica composite for selective adsorption of methylene blue. Microporous Mesoporous Mater. 330, 111570. doi:10.1016/j.micromeso.2021.111570
Saxena, M., Lochab, A., and Saxena, R. (2021). Asparagine functionalized MWCNTs for adsorptive removal of hazardous cationic dyes: Exploring kinetics, isotherm and mechanism. Surfaces Interfaces 25, 101187. doi:10.1016/j.surfin.2021.101187
Saxena, M., Sharma, N., and Saxena, R. (2021). 4-Aminosalicylic acid functionalized multiwalled carbon nanotubes for rapid removal of crystal violet dye from wastewater using minicolumn. ChemistrySelect 6 (39), 10601–10608. doi:10.1002/slct.202102847
Schwenke, A. M., Hoeppener, S., and Schubert, U. S. (2015). Synthesis and modification of carbon nanomaterials utilizing microwave heating. Adv. Mater. 27 (28), 4113–4141. doi:10.1002/adma.201500472
Sen Gupta, S., Chakraborty, I., Maliyekkal, S. M., Adit Mark, T., Pandey, D. K., Das, S. K., et al. (2015). Simultaneous dehalogenation and removal of persistent halocarbon pesticides from water using graphene nanocomposites: A case study of lindane. ACS Sustain. Chem. Eng. 3 (6), 1155–1163. doi:10.1021/acssuschemeng.5b00080
Shahrin, S., Lau, W-J., Goh, P-S., Jaafar, J., and Ismail, A. F. (2018). Adsorptive removal of as(V) ions from water using graphene oxide-manganese ferrite and titania nanotube-manganese ferrite hybrid nanomaterials. Chem. Eng. Technol. 41 (11), 2250–2258. doi:10.1002/ceat.201800322
Shan, H., Zeng, C., Zhao, C., and Zhan, H. (2021). Iron oxides decorated graphene oxide/chitosan composite beads for enhanced Cr(VI) removal from aqueous solution. Int. J. Biol. Macromol. 172, 197–209. doi:10.1016/j.ijbiomac.2021.01.060
Shang, J., Guo, Y., He, D., Qu, W., Tang, Y., Zhou, L., et al. (2021). A novel graphene oxide-dicationic ionic liquid composite for Cr(VI) adsorption from aqueous solutions. J. Hazard. Mater. 416, 125706. doi:10.1016/j.jhazmat.2021.125706
Shi, X., Wang, X., Zhang, S., Zhang, Z., Meng, X., Liu, H., et al. (2023). Hydrophobic carbon dots derived from organic pollutants and applications in NIR anticounterfeiting and bioimaging. Langmuir 39, 5056–5064. doi:10.1021/acs.langmuir.3c00075
Shi, Y., Liu, X., Wang, M., Huang, J., Jiang, X., Pang, J., et al. (2019). Synthesis of N-doped carbon quantum dots from bio-waste lignin for selective irons detection and cellular imaging. Int. J. Biol. Macromol. 128, 537–545. doi:10.1016/j.ijbiomac.2019.01.146
Shi, Z., Wang, S., Jin, Y., Zhao, L., Chen, S., Yang, H., et al. (2023). Establishment of green graphite industry: Graphite from biomass and its various applications. SusMat. doi:10.1002/sus2.139
Shrivas, K., Ghosale, A., Nirmalkar, N., Srivastava, A., Singh, S. K., and Shinde, S. S. (2017). Removal of endrin and dieldrin isomeric pesticides through stereoselective adsorption behavior on the graphene oxide-magnetic nanoparticles. Environ. Sci. Pollut. Res. 24 (32), 24980–24988. doi:10.1007/s11356-017-0159-z
Singh, R. K., Kumar, R., Singh, D. P., Savu, R., and Moshkalev, S. A. (2019). Progress in microwave-assisted synthesis of quantum dots (graphene/carbon/semiconducting) for bioapplications: A review. Mater. Today Chem. 12, 282–314. doi:10.1016/j.mtchem.2019.03.001
Singh, S., Khasnabis, S., Anil, A. G., Kumar, V., Kumar Naik, T. S. S., Nath, B., et al. (2022). Multifunctional nanohybrid for simultaneous detection and removal of Arsenic(III) from aqueous solutions. Chemosphere 289, 133101. doi:10.1016/j.chemosphere.2021.133101
Singh, S., Naik, T. S. S. K., Shehata, N., Aguilar-Marcelino, L., Dhokne, K., Lonare, S., et al. (2023). Novel insights into graphene oxide-based adsorbents for remediation of hazardous pollutants from aqueous solutions: A comprehensive review. J. Mol. Liq. 369, 120821. doi:10.1016/j.molliq.2022.120821
Sitko, R., Turek, E., Zawisza, B., Malicka, E., Talik, E., Heimann, J., et al. (2013). Adsorption of divalent metal ions from aqueous solutions using graphene oxide. Dalton Trans. 42 (16), 5682–5689. doi:10.1039/C3DT33097D
Somanathan, T., Prasad, K., Ostrikov, K., Saravanan, A., and Krishna, V. M. (2015). Graphene oxide synthesis from agro waste. Nanomaterials 5 (2), 826–834. doi:10.3390/nano5020826
Song, T., Tian, W., Qiao, K., Zhao, J., Chu, M., Du, Z., et al. (2021). Adsorption behaviors of polycyclic aromatic hydrocarbons and oxygen derivatives in wastewater on N-doped reduced graphene oxide. Sep. Purif. Technol. 254, 117565. doi:10.1016/j.seppur.2020.117565
Speranza, G. (2021). Carbon nanomaterials: Synthesis, functionalization and sensing applications. Nanomater. (Basel) 11 (4), 967. doi:10.3390/nano11040967
Sturm, G. S. J., Verweij, M. D., Stankiewicz, A. I., and Stefanidis, G. D. (2014). Microwaves and microreactors: Design challenges and remedies. Chem. Eng. J. 243, 147–158. doi:10.1016/j.cej.2013.12.088
Sui, Z., Meng, Q., Zhang, X., Ma, R., and Cao, B. (2012). Green synthesis of carbon nanotube–graphene hybrid aerogels and their use as versatile agents for water purification. J. Mater. Chem. 22 (18), 8767–8771. doi:10.1039/C2JM00055E
Sulaiman, K. O., Sajid, M., and Alhooshani, K. (2020). Application of porous membrane bag enclosed alkaline treated Y-Zeolite for removal of heavy metal ions from water. Microchem. J. 152, 104289. doi:10.1016/j.microc.2019.104289
Sun, J., Wang, W., and Yue, Q. (2016). Review on microwave-matter interaction fundamentals and efficient microwave-associated heating strategies. Mater. (Basel) 9 (4), 231. doi:10.3390/ma9040231
Sun, Y., Yang, S., Zhao, G., Wang, Q., and Wang, X. (2013). Adsorption of polycyclic aromatic hydrocarbons on graphene oxides and reduced graphene oxides. Chem. Asian J. 8 (11), 2755–2761. doi:10.1002/asia.201300496
Tadesse, A., RamaDevi, D., Hagos, M., Battu, G., and Basavaiah, K. (2018). Synthesis of nitrogen doped carbon quantum dots/magnetite nanocomposites for efficient removal of methyl blue dye pollutant from contaminated water. RSC Adv. 8 (16), 8528–8536. doi:10.1039/C8RA00158H
Tang, X., Tang, P., and Liu, L. (2018). Preparation of tetraethylenepentamine modified magnetic graphene oxide for adsorption of dyes from aqueous solution. J. Braz Chem. Soc. 29 (2), 334–342. doi:10.21577/0103-5053.20170145
Tang, Y., Guo, H., Xiao, L., Yu, S., Gao, N., and Wang, Y. (2013). Synthesis of reduced graphene oxide/magnetite composites and investigation of their adsorption performance of fluoroquinolone antibiotics. Colloids Surfaces A Physicochem. Eng. Aspects 424, 74–80. doi:10.1016/j.colsurfa.2013.02.030
Tavengwa, N. T., and Dalu, T. (2021). “Introduction to emerging freshwater pollutants,” in Emerging freshwater pollutants: Analysis, fate and regulations. Editors T. Dalu, and N. T. Tavengwa (London: Academic Press).
Thakur, C. K., Karthikeyan, C., Abou-Dahech, M. S., Altabakha, M. M. A. M., Al Shahwan, M. J. S., Ashby, C. R., et al. (2023). Microwave-assisted functionalization of multi-walled carbon nanotubes for biosensor and drug delivery applications. Pharmaceutics 15 (2), 335. doi:10.3390/pharmaceutics15020335
Thi Dang, Y., Hoang, H. T., Dong, H. C., Bui, K-B. T., Nguyen, L. H. T., Phan, T. B., et al. (2020). Microwave-assisted synthesis of nano Hf- and Zr-based metal-organic frameworks for enhancement of curcumin adsorption. Microporous Mesoporous Mater. 298, 110064. doi:10.1016/j.micromeso.2020.110064
Tohamy, H. A. S., El-Sakhawy, M., and Kamel, S. (2023). Eco-friendly synthesis of carbon quantum dots as an effective adsorbent. J. Fluoresc. 33 (2), 423–435. doi:10.1007/s10895-022-03085-z
Tong, X., Mohapatra, S., Zhang, J., Tran, N. H., You, L., He, Y., et al. (2022). Source, fate, transport and modelling of selected emerging contaminants in the aquatic environment: Current status and future perspectives. Water Res. 217, 118418. doi:10.1016/j.watres.2022.118418
Tsuji, M. (2017). Microwave-assisted synthesis of metallic nanomaterials in liquid phase. ChemistrySelect 2 (2), 805–819. doi:10.1002/slct.201700011
Verma, M., Lee, I., Oh, J., Kumar, V., and Kim, H. (2022). Synthesis of EDTA-functionalized graphene oxide-chitosan nanocomposite for simultaneous removal of inorganic and organic pollutants from complex wastewater. Chemosphere 287, 132385. doi:10.1016/j.chemosphere.2021.132385
Verma, S., Kim, K-H., Kumar, N., Bhattacharya, S. S., Naushad, M., and Dutta, R. K. (2022). Amine-amide functionalized graphene oxide sheets as bifunctional adsorbent for the removal of polar organic pollutants. J. Hazard. Mater. 429, 128308. doi:10.1016/j.jhazmat.2022.128308
Vignesh, N. S., Soosai, M. R., Chia, W. Y., Wahid, S. N., Varalakshmi, P., Moorthy, I. M. G., et al. (2022). Microwave-assisted pyrolysis for carbon catalyst, nanomaterials and biofuel production. Fuel 313, 123023. doi:10.1016/j.fuel.2021.123023
Vivekanandhan, S., Schreiber, M., Muthuramkumar, S., Misra, M., and Mohanty, A. K. (2017). Carbon nanotubes from renewable feedstocks: A move toward sustainable nanofabrication. J. Appl. Polym. Sci. 134 (4). doi:10.1002/app.44255
Voiry, D., Yang, J., Kupferberg, J., Fullon, R., Lee, C., Jeong, H. Y., et al. (2016). High-quality graphene via microwave reduction of solution-exfoliated graphene oxide. Science 353 (6306), 1413–1416. doi:10.1126/science.aah3398
Vu, C. T., and Wu, T. (2022). Recent progress in adsorptive removal of per- and poly-fluoroalkyl substances (PFAS) from water/wastewater. Crit. Rev. Environ. Sci. Technol. 52 (1), 90–129. doi:10.1080/10643389.2020.1816125
Wan, J., Huang, L., Wu, J., Xiong, L., Hu, Z., Yu, H., et al. (2018). Microwave combustion for rapidly synthesizing pore-size-controllable porous graphene. Adv. Funct. Mater. 28 (22), 1800382. doi:10.1002/adfm.201800382
Wang, C., Luo, H., Zhang, Z., Wu, Y., Zhang, J., and Chen, S. (2014). Removal of As(III) and As(V) from aqueous solutions using nanoscale zero valent iron-reduced graphite oxide modified composites. J. Hazard. Mater. 268, 124–131. doi:10.1016/j.jhazmat.2014.01.009
Wang, L., Cheng, C., Tapas, S., Lei, J., Matsuoka, M., Zhang, J., et al. (2015). Carbon dots modified mesoporous organosilica as an adsorbent for the removal of 2,4-dichlorophenol and heavy metal ions. J. Mater. Chem. A 3 (25), 13357–13364. doi:10.1039/C5TA01652E
Wang, S., Huo, X., Zhao, H., Dong, Y., Cheng, Q., and Li, Y. (2022). One-pot green synthesis of N,S co-doped biomass carbon dots from natural grapefruit juice for selective sensing of Cr(VI). Chem. Phys. Impact 5, 100112. doi:10.1016/j.chphi.2022.100112
Wang, Y., Ding, G., Lin, K., Liu, Y., Deng, X., and Li, Q. (2021). Facile one-pot synthesis of ultrathin carbon layer encapsulated magnetite nanoparticle and graphene oxide nanocomposite for efficient removal of metal ions. Sep. Purif. Technol. 266, 118550. doi:10.1016/j.seppur.2021.118550
Wang, Y., Hu, Y-J., Hao, X., Peng, P., Shi, J-Y., Peng, F., et al. (2020). Hydrothermal synthesis and applications of advanced carbonaceous materials from biomass: A review. Adv. Compos. Hybrid Mater. 3 (3), 267–284. doi:10.1007/s42114-020-00158-0
Wang, Y., Huo, Q., Shi, L., Feng, G., Wang, J., Han, L., et al. (2020). Adsorption of mercury species on selected CuS surfaces and the effects of HCl. Chem. Eng. J. 393, 124773. doi:10.1016/j.cej.2020.124773
Wang, Z., Ogata, H., Morimoto, S., Ortiz-Medina, J., Fujishige, M., Takeuchi, K., et al. (2015). Nanocarbons from rice husk by microwave plasma irradiation: From graphene and carbon nanotubes to graphenated carbon nanotube hybrids. Carbon 94, 479–484. doi:10.1016/j.carbon.2015.07.037
Wang, Z., Shen, D., Wu, C., and Gu, S. (2018). State-of-the-art on the production and application of carbon nanomaterials from biomass. Green Chem. 20 (22), 5031–5057. doi:10.1039/C8GC01748D
Wang, Z., Shoji, M., and Ogata, H. (2011). Carbon nanosheets by microwave plasma enhanced chemical vapor deposition in CH4–Ar system. Appl. Surf. Sci. 257 (21), 9082–9085. doi:10.1016/j.apsusc.2011.05.104
Wanjeri, V. W. O., Sheppard, C. J., Prinsloo, A. R. E., Ngila, J. C., and Ndungu, P. G. (2018). Isotherm and kinetic investigations on the adsorption of organophosphorus pesticides on graphene oxide based silica coated magnetic nanoparticles functionalized with 2-phenylethylamine. J. Environ. Chem. Eng. 6 (1), 1333–1346. doi:10.1016/j.jece.2018.01.064
Wei, H., Deng, S., Huang, Q., Nie, Y., Wang, B., Huang, J., et al. (2013). Regenerable granular carbon nanotubes/alumina hybrid adsorbents for diclofenac sodium and carbamazepine removal from aqueous solution. Water Res. 47 (12), 4139–4147. doi:10.1016/j.watres.2012.11.062
Worasuwannarak, N., Sonobe, T., and Tanthapanichakoon, W. (2007). Pyrolysis behaviors of rice straw, rice husk, and corncob by TG-MS technique. J. Anal. Appl. Pyrolysis 78 (2), 265–271. doi:10.1016/j.jaap.2006.08.002
Wu, T., Cai, X., Tan, S., Li, H., Liu, J., and Yang, W. (2011). Adsorption characteristics of acrylonitrile, p-toluenesulfonic acid, 1-naphthalenesulfonic acid and methyl blue on graphene in aqueous solutions. Chem. Eng. J. 173 (1), 144–149. doi:10.1016/j.cej.2011.07.050
Wu, W., Yang, Y., Zhou, H., Ye, T., Huang, Z., Liu, R., et al. (2012). Highly efficient removal of Cu(II) from aqueous solution by using graphene oxide. Water, Air, & Soil Pollut. 224 (1), 1372. doi:10.1007/s11270-012-1372-5
Xie, H., Poyraz, S., Thu, M., Liu, Y., Snyder, E. Y., Smith, J. W., et al. (2014). Microwave-assisted fabrication of carbon nanotubes decorated polymeric nano-medical platforms for simultaneous drug delivery and magnetic resonance imaging. RSC Adv. 4 (11), 5649–5652. doi:10.1039/C3RA45913F
Xie, X., Zhou, Y., and Huang, K. (2019). Advances in microwave-assisted production of reduced graphene oxide. Front. Chem. 7, 355. doi:10.3389/fchem.2019.00355
Xiong, S., Wu, Z., and Li, Z. (2022). Facile fabrication of robust, versatile, and recyclable biochar-graphene oxide composite monoliths for efficient removal of different contaminants in water. Chemosphere 287, 132418. doi:10.1016/j.chemosphere.2021.132418
Xu, J., Wang, L., and Zhu, Y. (2012). Decontamination of bisphenol A from aqueous solution by graphene adsorption. Langmuir 28 (22), 8418–8425. doi:10.1021/la301476p
Xuan, T-T., Liu, J-Q., Xie, R-J., Li, H-L., and Sun, Z. (2015). Microwave-assisted synthesis of CdS/ZnS:Cu quantum dots for white light-emitting diodes with high color rendition. Chem. Mater. 27 (4), 1187–1193. doi:10.1021/cm503770w
Yadav, S., Goel, N., Kumar, V., and Singhal, S. (2019). Graphene oxide as proficient adsorbent for the removal of harmful pesticides: Comprehensive experimental cum DFT investigations. Anal. Chem. Lett. 9 (3), 291–310. doi:10.1080/22297928.2019.1629999
Yahaya Pudza, M., Zainal Abidin, Z., Abdul Rashid, S., Md Yasin, F., Noor, A. S. M., and Issa, M. A. (2020). Eco-friendly sustainable fluorescent carbon dots for the adsorption of heavy metal ions in aqueous environment. Nanomaterials 10 (2), 315. doi:10.3390/nano10020315
Yan, L., Yang, Y., Ma, C-Q., Liu, X., Wang, H., and Xu, B. (2016). Synthesis of carbon quantum dots by chemical vapor deposition approach for use in polymer solar cell as the electrode buffer layer. Carbon 109, 598–607. doi:10.1016/j.carbon.2016.08.058
Yang, D., Tammina, S. K., Li, X., and Yang, Y. (2019). Enhanced removal and detection of benzo[a]pyrene in environmental water samples using carbon dots-modified magnetic nanocomposites. Ecotoxicol. Environ. Saf. 170, 383–390. doi:10.1016/j.ecoenv.2018.11.138
Yang, G., Li, Y., Yang, S., Liao, J., Cai, X., Gao, Q., et al. (2021). Surface oxidized nano-cobalt wrapped by nitrogen-doped carbon nanotubes for efficient purification of organic wastewater. Sep. Purif. Technol. 259, 118098. doi:10.1016/j.seppur.2020.118098
Yang, K., Chen, B., and Zhu, L. (2015). Graphene-coated materials using silica particles as a framework for highly efficient removal of aromatic pollutants in water. Sci. Rep. 5, 11641. doi:10.1038/srep11641
Yang, P., Zhu, Z., Chen, M., Chen, W., and Zhou, X. (2018). Microwave-assisted synthesis of xylan-derived carbon quantum dots for tetracycline sensing. Opt. Mater. 85, 329–336. doi:10.1016/j.optmat.2018.06.034
Yang, R., and Chen, J. (2021). Mechanistic and machine learning modeling of microwave heating process in domestic ovens: A review. Foods 10 (9), 2029. doi:10.3390/foods10092029
Yang, T., He, R., Nie, G., Wang, W., Zhang, G., Hu, Y., et al. (2018). Creation of hollow calcite single crystals with CQDs: Synthesis, characterization, and fast and efficient decontamination of Cd(II). Sci. Rep. 8 (1), 17603. doi:10.1038/s41598-018-36044-5
Yang, X., Li, J., Wen, T., Ren, X., Huang, Y., and Wang, X. (2013). Adsorption of naphthalene and its derivatives on magnetic graphene composites and the mechanism investigation. Colloids Surfaces A Physicochem. Eng. Aspects 422, 118–125. doi:10.1016/j.colsurfa.2012.11.063
Yi, X., Sun, F., Han, Z., Han, F., He, J., Ou, M., et al. (2018). Graphene oxide encapsulated polyvinyl alcohol/sodium alginate hydrogel microspheres for Cu (II) and U (VI) removal. Ecotoxicol. Environ. Saf. 158, 309–318. doi:10.1016/j.ecoenv.2018.04.039
yosef, M., Fahmy, A., hassan, A., El hotaby, W., and Anis, B. (2020). Porous polyvinyl formaldehyde/MWCNTs foam for Pb+2 removal from water. Egypt. J. Chem. 0 (1), 0–45. doi:10.21608/ejchem.2020.34453.2733
Yu, F., Li, Y., Han, S., and Ma, J. (2016). Adsorptive removal of antibiotics from aqueous solution using carbon materials. Chemosphere 153, 365–385. doi:10.1016/j.chemosphere.2016.03.083
Yuan, Y., Zhang, G., Li, Y., Zhang, G., Zhang, F., and Fan, X. (2013). Poly(amidoamine) modified graphene oxide as an efficient adsorbent for heavy metal ions. Polym. Chem. 4 (6), 2164–2167. doi:10.1039/C3PY21128B
Zakaria, N. Z. J., Rozali, S., Mubarak, N. M., and Ibrahim, S. (2022). A review of the recent trend in the synthesis of carbon nanomaterials derived from oil palm by-product materials. Biomass Convers. Biorefinery. doi:10.1007/s13399-022-02430-3
Zhan, M., Pan, G., Wang, Y., Kuang, T., and Zhou, F. (2017). Ultrafast carbon nanotube growth by microwave irradiation. Diam. Relat. Mater. 77, 65–71. doi:10.1016/j.diamond.2017.06.001
Zhang, K., Dwivedi, V., Chi, C., and Wu, J. (2010). Graphene oxide/ferric hydroxide composites for efficient arsenate removal from drinking water. J. Hazard. Mater. 182 (1), 162–168. doi:10.1016/j.jhazmat.2010.06.010
Zhang, Y., Cao, B., Zhao, L., Sun, L., Gao, Y., Li, J., et al. (2018). Biochar-supported reduced graphene oxide composite for adsorption and coadsorption of atrazine and lead ions. Appl. Surf. Sci. 427, 147–155. doi:10.1016/j.apsusc.2017.07.237
Zhang, Z., Yang, R., Zheng, Y., Bai, H., Shi, J., Zhang, J., et al. (2022). Graphene oxide-Fe3O4 nanocomposite used as aniline adsorbent with a wide pH range. Colloid Polym. Sci. 300 (1), 83–93. doi:10.1007/s00396-021-04926-2
Zhao, J-R., Hu, J., Li, J-F., and Chen, P. (2020). N-doped carbon nanotubes derived from waste biomass and its electrochemical performance. Mater. Lett. 261, 127146. doi:10.1016/j.matlet.2019.127146
Zheng, W., Zhang, P. G., Chen, J., Tian, W. B., Zhang, Y. M., and Sun, Z. M. (2019). Microwave-assisted synthesis of three-dimensional MXene derived metal oxide/carbon nanotube/iron hybrids for enhanced lithium-ions storage. J. Electroanal. Chem. 835, 205–211. doi:10.1016/j.jelechem.2019.01.036
Zhong, Q., Chen, Y., Su, A., and Wang, Y. (2018). Synthesis of catalytically active carbon quantum dots and its application for colorimetric detection of glutathione. Sensors Actuators B Chem. 273, 1098–1102. doi:10.1016/j.snb.2018.07.026
Zhu, Y., Murali, S., Stoller, M. D., Velamakanni, A., Piner, R. D., and Ruoff, R. S. (2010). Microwave assisted exfoliation and reduction of graphite oxide for ultracapacitors. Carbon 48 (7), 2118–2122. doi:10.1016/j.carbon.2010.02.001
Zhu, Y., Stoller, M. D., Cai, W., Velamakanni, A., Piner, R. D., Chen, D., et al. (2010). Exfoliation of graphite oxide in propylene carbonate and thermal reduction of the resulting graphene oxide platelets. ACS Nano 4 (2), 1227–1233. doi:10.1021/nn901689k
Zhu, Y-J., and Chen, F. (2014). Microwave-assisted preparation of inorganic nanostructures in liquid phase. Chem. Rev. 114 (12), 6462–6555. doi:10.1021/cr400366s
Zulfiqar, M., Rabat, N. E., Bahadar, A., Lashari, N., Mahnashi, M. H., and Alqarni, A. O. (2022). Development of Elaeis guineensis/polyvinyl alcohol/carbon nanotube composites for efficient adsorption of dye: Experimental and theoretical approach. Int. J. Environ. Sci. Technol. 19 (7), 6499–6520. doi:10.1007/s13762-021-03520-x
Keywords: adsorption, carbon-based nanomaterials, chemical pollutants, microwave-assisted synthesis, water treatment
Citation: Adeola AO, Duarte MP and Naccache R (2023) Microwave-assisted synthesis of carbon-based nanomaterials from biobased resources for water treatment applications: emerging trends and prospects. Front. Carbon 2:1220021. doi: 10.3389/frcrb.2023.1220021
Received: 09 May 2023; Accepted: 20 June 2023;
Published: 05 July 2023.
Edited by:
Oomman K. Varghese, University of Houston, United StatesReviewed by:
Luiz K. C. De Souza, Federal University of Amazonas, BrazilMamun Jamal, Khulna University of Engineering and Technology, Bangladesh
Copyright © 2023 Adeola, Duarte and Naccache. This is an open-access article distributed under the terms of the Creative Commons Attribution License (CC BY). The use, distribution or reproduction in other forums is permitted, provided the original author(s) and the copyright owner(s) are credited and that the original publication in this journal is cited, in accordance with accepted academic practice. No use, distribution or reproduction is permitted which does not comply with these terms.
*Correspondence: Adedapo O. Adeola, YWRlZGFwby5hZGVvbGFAY29uY29yZGlhLmNh; Rafik Naccache, cmFmaWsubmFjY2FjaGVAY29uY29yZGlhLmNh