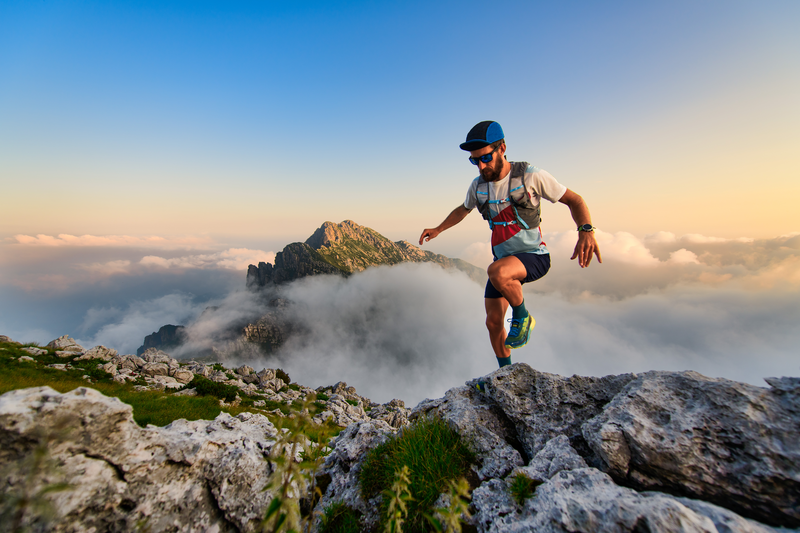
94% of researchers rate our articles as excellent or good
Learn more about the work of our research integrity team to safeguard the quality of each article we publish.
Find out more
REVIEW article
Front. Built Environ.
Sec. Sustainable Design and Construction
Volume 11 - 2025 | doi: 10.3389/fbuil.2025.1534284
The final, formatted version of the article will be published soon.
You have multiple emails registered with Frontiers:
Please enter your email address:
If you already have an account, please login
You don't have a Frontiers account ? You can register here
This study explores the geometrical design of windcatchers, a traditional architectural element offering a sustainable solution for natural ventilation in hot, arid climates. The growing demand for energy-efficient cooling has renewed interest in windcatchers, but their integration into contemporary architecture remains limited due to knowledge gaps and practical challenges. The study aims to: (1) offer architects practical guidelines for incorporating windcatchers into their designs, (2) create a research roadmap to address underexplored geometrical design parameters, and (3) standardize design parameters for each windcatcher component. The review identified well-studied components, such as aerodynamic advantages of curved top surfaces. It highlighted inconsistencies in literature and unvalidated findings, such as conflicting findings on the optimal outlet-to-inlet area ratio. It also revealed unexplored design parameters that require further investigation. The study developed a structured research roadmap with standardized design parameters, facilitating the creation of a comprehensive design guide for architects that ultimately enabling the more widespread and effective use of windcatchers in contemporary architectural practice.
Keywords: Windcatcher, Natural ventilation, geometrical design parameters, Primary components, Supplementary components, Wind tower
Received: 25 Nov 2024; Accepted: 03 Mar 2025.
Copyright: © 2025 Mayhoub, Selim and Abuzaid. This is an open-access article distributed under the terms of the Creative Commons Attribution License (CC BY). The use, distribution or reproduction in other forums is permitted, provided the original author(s) or licensor are credited and that the original publication in this journal is cited, in accordance with accepted academic practice. No use, distribution or reproduction is permitted which does not comply with these terms.
* Correspondence:
Mohammed Mayhoub, Al-Azhar University, Cairo, Egypt
Disclaimer: All claims expressed in this article are solely those of the authors and do not necessarily represent those of their affiliated organizations, or those of the publisher, the editors and the reviewers. Any product that may be evaluated in this article or claim that may be made by its manufacturer is not guaranteed or endorsed by the publisher.
Research integrity at Frontiers
Learn more about the work of our research integrity team to safeguard the quality of each article we publish.