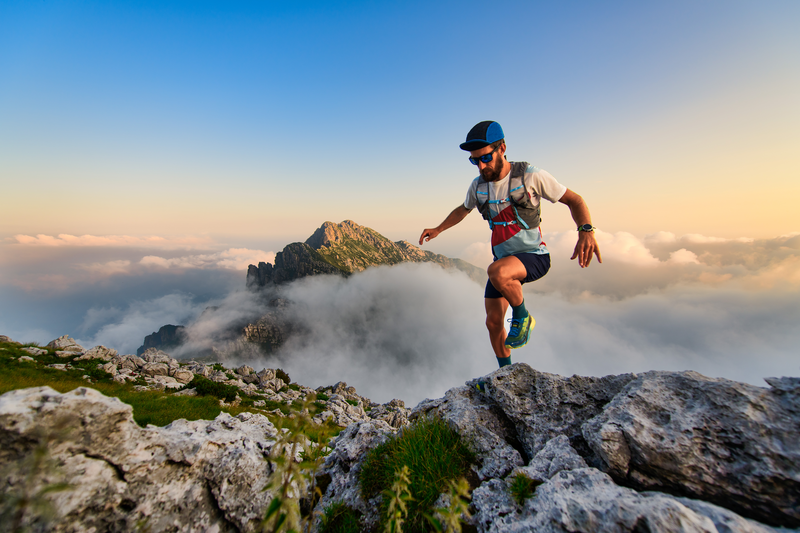
94% of researchers rate our articles as excellent or good
Learn more about the work of our research integrity team to safeguard the quality of each article we publish.
Find out more
SYSTEMATIC REVIEW article
Front. Built Environ. , 05 February 2025
Sec. Fire Resistant Engineering
Volume 10 - 2024 | https://doi.org/10.3389/fbuil.2024.1495594
Background: Hospitals are complex and dynamic facilities that house vulnerable people, medical equipment, and hazardous materials, making them susceptible to fires and explosions with potentially catastrophic effects. This study aims to understand and address fire and explosion risks in hospital environments using biomedical engineering, safety, and fire prevention concepts to develop effective mitigation strategies.
Materials and Methods: The study employed a mixed-methods approach, integrating quantitative and qualitative techniques. Data from an insurance and reinsurance company in Peru were analyzed, and surveys, data analysis, and interviews were conducted. The preferred reporting items for systematic review and meta-analysis (PRISMA) methodology was also applied to conduct a thorough literature review.
Results: The results revealed the most frequent risk factors in hospital settings and identified the most effective mitigation strategies. Implementing these strategies resulted in a considerable decrease in the incidences of fires and explosions.
Discussion: The research provides a comprehensive explanation of hospital fire and explosion risks and proposes evidence-based strategies to improve the safety. These results underscore the relevance of biomedical engineering in managing risks within hospital settings. Despite certain limitations, the study lays a firm foundation for future research aimed at improving hospital safety.
Fire and explosion risk mitigation in hospital environments is crucial due to the high concentration of electrical equipment and flammable materials in these places. Effective strategies are required to prevent and manage incidents that may compromise the integrity of patients, medical equipment, and visitors.
This research focuses on identifying, assessing, and mitigating fire and explosion risks in hospital environments. Several studies have contributed to the understanding of these risks, highlighting the following aspects (Table 1).
Several factors contribute to the risk of fires and explosions in hospitals, such as combustible materials, ignition sources, and poor environmental conditions (Organización Mundial de la Salud OMS, 2023). These incidents can severely affect patients, staff, and hospital infrastructure. Among the most common causes of fires in these environments are electrical faults, friction and rubbing, mechanical sparks, smoking, use of matches, spontaneous ignition, high-temperature surfaces, and sparks from combustible materials (Prevención de riesgos laborales en el sector sanitario, 2024).
In hospitals, the risk of fires and explosions is intensified due to the presence of flammable products, hazardous materials, and a high concentration of people and medical equipment sensitive to high temperatures and smoke (Palomino Marín, 2014). In addition, the possibility of explosions is especially significant in places such as laboratories, operating rooms, boiler areas, hydrogen or oxygen cylinder storage, refrigerated facilities with flammable refrigerants, and spaces where equipment with autoclaves or compressors for sterilization systems is used (Organización Mundial de la Salud, 2017). Both qualitative and quantitative methods have been developed to assess the risk of fires and explosions in hospital environments (Organización Mundial de la Salud OMS, 2023). Various preventive and protective measures have been implemented to decrease these risks, such as fire warning and control systems, evacuation plans, and training programs for personnel (Barbeiro and Pérez, 2021).
In addition, bibliometric analysis in research on explosions and associated risks has shown a significant increase in academic and practical interest in this field. Research such as that by Di Benedetto et al. (2009) has contributed to understanding the dynamics of explosions in gas mixtures, which applies to improving safety in hospital environments.
Fire and explosion consequence modeling is fundamental to risk assessment. Di Benedetto et al. (2009) developed models to understand the behavior of explosions in gas mixtures, which are applicable for strengthening safety in hospital environments (Di Benedetto et al., 2009). These models allow for predicting the spread of fires and the dispersion of hazardous substances, facilitating the design of more effective protection systems.
Biomedical engineering plays a crucial role in hospital fire safety. The integration of advanced monitoring and alarm systems and the adoption of fire-resistant materials in the construction of medical equipment are examples of how technology can strengthen safety. Research by Liu et al. (2021) on thermal hazard trends in lithium-ion batteries highlights the importance of addressing specific risks associated with medical devices (Liu et al., 2021).
Some of the research works that have been carried out in this area are as follows.
Hospital fires and explosion risks symbolize a significant danger to health and welfare. This study proposes a method for assessing such risks in hospital environments. This approach is based on a predictive model designed to identify potential risks. The authors concluded that the predictive model proved more effective than traditional risk assessment methods (García-Fernández et al., 2023).
Other researchers have developed an advanced fire detection and suppression system for hospital environments. This system is based on a predictive model and has been proven more efficient than traditional fire detection methods. This innovative approach facilitates faster and more accurate detection of potential fires, significantly improving response and risk mitigation in these critical environments (Barbeiro and Pérez, 2021).
In addition, experts have developed a fire safety training program for healthcare personnel. This program includes a module dedicated to using predictive models to detect risks. Studies indicated that this module effectively increased healthcare personnel’s understanding of predictive models (Feuerstein and Custer, 2021).
According to experts in biomedical engineering, electrical safety regulations must be considered when installing equipment in intensive care units (I.C.Us) and central surgical centers (C.S.Cs) as a preventive measure to avoid accidents such as fires or explosions related to anesthesia equipment (Prevención de riesgos laborales en el sector sanitario, 2024). Research in this area significantly improves fire and explosion safety in hospital environments, safeguarding the lives and wellbeing of patients, staff, and visitors.
Research on fire and explosion risk assessment and mitigation in hospital environments is based on biomedical engineering, safety engineering, and fire engineering principles. These principles are crucial to developing practical solutions for identifying, assessing, and mitigating risks in complex hospital environments (Table 2). After identifying risks, it is critical to determine their severity. This can be achieved through qualitative assessment, which involves identifying and ranking risks according to their severity (University of Cambridge, 2022), and quantitative evaluation, which quantifies the risk using factors such as likelihood of occurrence, severity of consequences, and exposure (University of California and Berkeley, 2022).
Risk assessment is a crucial procedure to determine which risks should be addressed as a priority (Barbeiro and Pérez, 2021). Biomedical engineering focuses on applying engineering principles to medical and biological systems. Biomedical engineers are crucial in analyzing and mitigating fire and explosion risks in hospital environments. They use their knowledge and skills to identify and assess these risks, proposing innovative solutions to reduce them.
The main objective of fire and explosion risk analysis and reduction is to minimize the risk to an acceptable level. This involves implementing various preventive actions, which focus on avoiding fires and explosions (Organización Mundial de la Salud OMS, 2023) and protective measures designed to safeguard people and property in such events (Castel Pérez and Sánchez Álvarez, 2018). Preventive measures include control of flammable materials, where hospitals should limit the presence of these materials, and ignition source monitoring, which implements equipment and technologies that result in early identification the presence of fire and alert building occupants to the hazard.
Hospital personnel must be trained in fire and explosion prevention and management. Despite the above advances, prevention and mitigation of these risks in hospital environments remain crucial. The complexity of these environments, the diversity of potential hazards, and ensuring continuity of care pose considerable challenges. The current literature on fire and explosion risk assessment and mitigation in hospitals is highly relevant for addressing these issues (García-Fernández et al., 2023). The use of predictive models and advanced detection systems is only one of several approaches available. Despite these advances, shortcomings persist in fully understanding the risks and implementing practical solutions. In addition, it highlights the importance of adopting a comprehensive approach that considers the following considerations:
• Accurate identification of risk factors: Identifying the elements contributing to the increase in fire and explosion risk in each hospital environment is crucial.
• Development of effective risk assessment methods: Risk assessment methods must be developed to be accurate, reliable, and adaptable to the unique characteristics of hospital environments.
• Implementation of specific preventive and protective measures: These measures should be adapted to the characteristics of each hospital environment, considering the needs of patients, staff, and visitors.
• Ongoing staff education and training. It is crucial to provide continuous education and training to staff to ensure proper implementation of protective and preventive measures.
In the context of an insurance and reinsurance company in Peru, the lack of controls and risk analysis can significantly affect fire risk management in hospital environments. This situation implies that adequate preventive and protective measures are possibly not being implemented, which increases the risk of fires and explosions in these critical environments. This work contributes significantly to research on analyzing and mitigating these risks in hospital environments. By adopting a biomedical engineering perspective and proposing a predictive model based on NFPA regulations, this study has the potential to generate innovative and practical solutions to safeguard these critical environments. Therefore, biomedical engineers play a crucial role in preventing fires and explosions in hospitals by ensuring the safety of medical equipment through inspections and preventive maintenance, managing risks, and providing safety training and education.
The preferred reporting items for systematic review and meta-analysis (PRISMA 2020) method was used to conduct this systematic review, which is an updated guideline that specifies 27 essential elements that should be included in a systematic review report, thus ensuring transparency, completeness, and reliability of the study. This statement is based on the original version of PRISMA published in 2009 and has been updated to incorporate the most recent advances in systematic review methodology. The structure of PRISMA 2020 comprises several main sections: introduction, method, results, or discussion, and a detailed checklist of the 27 items.
For this systematic review, the PRISMA 2020 method was adopted. An exhaustive search was conducted in several databases, including Dialnet, Google Scholar, Scopus, SciELO, and PubMed. The keywords used in the exploration were designed to identify relevant studies on the risk of fires and explosions in hospital environments from a biomedical engineering perspective.
Initially, 124 potentially relevant studies were identified. After reviewing titles and abstracts, eight studies were selected for a full review. The search strategy used was (Fire OR Explosion) AND risk AND hospital AND “Biomedical Engineering,” with no restrictions on time and categorization of health facilities.
This research also employed a risk assessment methodology to analyze and mitigate fire and explosion risks in hospital settings from a biomedical engineering perspective. Four experts in the field participated in assessing an insurance and reinsurance company in Peru. This method is based on previous studies demonstrating its effectiveness in similar contexts (Table 3).
For the risk assessment, both qualitative and quantitative analyses were used (Ebersbach and Powers, 2022). The experts rated the risks in four categories: very low, low, medium, and high, while evaluating the effectiveness, feasibility, impact, and cost of the proposed measures. The ratings obtained were high in all categories, indicating a high level of consensus and confidence in the proposed mitigation measures.
Determination of the context and scope of the risk: The principal risks of fires and explosions in hospital environments were identified, considering the infrastructure, biomedical equipment, and operating procedures.
1. Selection of experts: Four experts with extensive experience in biomedical engineering and risk management in hospital environments were selected (Table 3).
2. Qualitative analysis: The experts were interviewed and participated in participatory workshops to identify and rank potential risks. The KISS (keep it super simple) method was used to rate the risks from very low to high (Ebersbach and Powers, 2022).
3. Quantitative analysis: Quantitative analysis techniques were used to compute the probability and impact of the identified risks. This allowed numerical values to be assigned to the risks and prioritized according to their severity and mitigation potential (Ebersbach and Powers, 2022).
4. Evaluation and validation: The proposed measures were evaluated in terms of the effectiveness, feasibility, impact, and cost using an evaluation matrix that allowed experts to rate each aspect on a highly accurate scale (Table 4).
This methodological approach ensures a comprehensive and specific estimation of risks, facilitating the implementation of effective mitigation measures in hospital settings.
Twenty-six studies were excluded because they did not strictly meet the established inclusion criteria:
Inclusion criteria
• Specifically address the risk of fires and explosions in hospitals.
• Be observational or experimental studies.
• Be published in English or Spanish.
Exclusion criteria
• Incompatibility with the topic
• Not related to the object of study
Of the 32 complete studies reviewed, six met all inclusion criteria and were meticulously evaluated for final eligibility. In addition, two new studies were included by other methods, making a total of eight final studies in the review. All of them were included in the systematic review. The selected studies employed various methodologies and were conducted in several countries (Figure 1). The findings revealed significant variability in the results: some identified an increased risk compared to other building types, while other studies found no clear associations. This analysis stands out because there is a need to comprehensively and scientifically expose the risk of fires and explosions in hospital settings using interdisciplinary approaches such as biomedical engineering to promote safety and prevention.
Figure 1. PRISMA flowchart of the selected studies. The new design is adapted from flowcharts proposed by Boers, Mayo-Wilson et al., and Stovold et al. (Haddaway et al., 2022).
Within our research, the case study analyzes and manages fire and explosion risks in hospitals, exploring these situations from a biomedical engineering perspective. This study examines several property risk inspection reports in medical centers, clinics, and hospitals to evaluate the risks related to medical equipment and possible fire and explosion incidents. A mixed approach integrating quantitative and qualitative methods will be used to address these risks in hospitals. A detailed analysis of the database of an insurance and reinsurance company in Peru will be carried out using surveys, data analysis, interviews, and the PRISMA methodology for systematic literature reviews. Risk factors such as combustible materials, medical equipment, and ignition sources will be identified.
Quantitative and qualitative approaches will be used to assess the severity of the risks, and adapted preventive and protective measures will be implemented. Biomedical engineering will be vital in implementing controls through safeguards in risk inspection reports. Constant monitoring will be carried out to evaluate the effectiveness of the implemented actions, and conclusions and suggestions for improving fire safety will be provided. The study emphasizes the role of biomedical engineering in risk management and proposes areas for future research and improvements in hospital safety. These suggestions will play a key role in improving safety and preventing incidents in healthcare facilities, which will be crucial in protecting the hospital infrastructure and the safety of patients and medical staff.
The population analyzed includes various medical centers and clinics belonging to an insurance and reinsurance company in Peru, located in different regions such as Lima, Arequipa, Pucallpa, Huancayo, and Trujillo. This population of interest includes dental offices, health centers, medical offices, hospitals, and specialized clinics, among other types of insured medical establishments.
The case analysis focused on evaluating and managing risks related to fires and explosions in hospital environments to develop effective prevention methods. Several variables that can contribute to these events were identified and assessed, highlighting the following factors as potential causes of fires and explosions in hospitals (Table 5):
1. Electrical overload and poor maintenance: Overheating medical equipment, often due to excessive electrical load and lack of proper maintenance, was identified as a significant risk factor.
2. Improper use of electrical hemostatic equipment: Improper handling of these devices can lead to operational failures that increase the risk of fire.
3. Short circuits in medical equipment: Electrical short circuits caused by defective insulation or internal wiring damage represent a vital risk source.
4. Failures in the electrical insulation of medical devices: Problems with the electrical insulation of medical devices, aggravated by the presence of gases and deterioration of the insulating material, can cause fires and explosions.
5. Overheating of mechanical ventilators: Failures in ventilator control systems, problems with thermal switches, and faulty temperature sensors are all factors that can lead to overheating and possible fires.
6. Inadequate maintenance of anesthesia equipment: Lack of proper cleaning and maintenance can result in electrical insulation failures, generating sparks that can cause fires.
7. Short circuits in tomography equipment: Electrical overload in these devices was also identified as a significant risk of short circuits and possible fires.
At the Peruvian insurance and reinsurance company under study, controls were implemented to prevent fires and explosions. Based on the root cause analysis of claims related to medical equipment, these controls were implemented in various stages in accordance with current best practices and standards (Table 2).
A data study evaluated the effectiveness of controls applied in hospital fire prevention. This process allowed for a detailed evaluation and in-depth understanding of how the controls work under various conditions. The method used was the “Use of Controls (Safeguards)” data analysis method, which compared the incident rate before and after their implementation.
In the methodological process, data on the frequency of fires were collected during a specific period before and after implementing the controls. Incident rates were calculated for each period, defined as the number of incidents divided by the units exposed to risk. These rates were then compared to determine the incident rates for both periods to determine whether there was a statistically significant difference. If the casualty rate was lower after implementing the controls, it could be concluded that the controls had effectively prevented fires.
Description of the validation process: Expert judgment was used to analyze and evaluate the proposed controls to mitigate fire and explosion risks in hospital facilities. The study is part of research on the analysis and mitigation of these risks from a biomedical engineering perspective. The questionnaire was the instrument used for data collection and was addressed to four experts with more than 10 years of experience as senior inspectors in property risks. The validation was carried out individually, without interaction between the experts (Table 4).
• High rating (3 or 4): This indicates that the control is a very effective measure to mitigate risk and is feasible to implement (Table 3).
• Medium rating (2): This indicates that the control is an effective measure to mitigate risk and is feasible to implement or is very viable and practical (Table 3).
• Low rating (1): This indicates that the control effectively mitigates the risk, but its implementation may be complex or costly (Table 3).
• Very low rating (0): This indicates that the control is not an effective measure to mitigate the risk or that its implementation is unfeasible (Table 3).
The present study was conducted using a qualitative and quantitative approach, combining individual interviews and participatory workshops with heritage risk inspection experts. Four experts, each with more than 10 years of experience in the field, were selected to ensure the validity and relevance of the data obtained.
Semi-structured interviews were conducted with each of the experts. These interviews focused on the identification of fire and explosion hazards in hospital environments and the evaluation of existing control measures. The questions addressed aspects such as the effectiveness, feasibility, impact, and cost of the controls.
Subsequently, participatory workshops were held where the experts discussed and validated the preliminary findings of the interviews. This format allowed for interaction among participants, promoting a dynamic exchange of ideas and experiences.
A specialized questionnaire was designed to evaluate the existing controls based on specific criteria:
• Effectiveness: capacity of the control to mitigate risk.
• Feasibility: ease of implementation of the control.
• Impact: potential consequences of the risk if not controlled.
• Cost: resources required to implement the control.
The KISS (keep it super simple) method was applied to classify the identified risks on a scale ranging from “very low” to “high.” This simplified methodology allowed the experts to assign risk levels in a clear and concise manner.
The data obtained were organized in a matrix that facilitated the calculation of averages and the identification of the most effective and practical strategies. This analysis provided a clear visualization of the prioritized risks and recommended actions.
To validate the content of the questionnaire and the criteria evaluated, the Aiken V was used. This method made it possible to measure the degree of agreement among the experts on the validity of each criterion, resulting in an index of 0.95, indicating a high level of consensus.
The reliability of the results was evaluated using the Cronbach’s Alpha coefficient. A value of 0.91 was obtained, suggesting an acceptable consistency in the answers provided by the experts.
Out of a total of 124 articles investigated, 124 were found in Dialnet (n = 14), Google Scholar (n = 37), Scopus (n = 14), SciELO (n = 19), PubMed (n = 19), and Websites (n = 2). No studies were identified in Web of Science or LILACS. In addition, 59 papers that were not closely related to the topic were excluded, leaving 44 papers for further analysis.
Eight scientific articles were reviewed in the systematic review. The distribution by country of origin of the studies was as follows: Colombia with 37.5% (three articles) (Palomino Marín, 2014; Fernández Sánchez Taype, 2016; Ruiz Ibañez et al., 2010), Spain with 50% (four articles) (Castel Pérez and Sánchez Álvarez, 2018; Cones, 2016; Romo Garrido, 2015; Ruiz Ibañez et al., 2010), and Iraq with 12.5% (one article) (Wood et al., 2021). Regarding the indexing of the articles reviewed, 25% were indexed in Scopus (two articles) (Wood et al., 2021; Lantarón-Sánchez, 2013), 12.5% in Dialnet (one article) (Ruiz Ibañez et al., 2010), and 12.5% in Website (two articles) (Cones, 2016; Romo Garrido, 2015). In addition, the remaining 50% corresponded to Google Scholar, distributed as follows: 25% from Colombia (two articles) (Palomino Marín, 2014; Fernández Sánchez Taype, 2016) and 12.5% from Spain (one article) (Castel Pérez and Sánchez Álvarez, 2018). This distribution reflects a predominance of studies in Spanish-speaking environments, with a notable contribution of research in Colombia and Spain (Table 6).
The studies reviewed used various regulations to support and validate their findings. An analysis of fire safety in Spanish hospitals was conducted, assessing its current status and proposing possible solutions using the BS1 Technical Verification Code for Fire Safety to compare the mandatory requirements outlined in the national regulations (Lantarón-Sánchez, 2013). Similarly, another study was based on the national techno-vigilance and pharmacovigilance programs, the good clinical practices led by INVIMA, and the standards established by the Colombian IPS (Ruiz Ibañez et al., 2010). In addition, the study entitled “Design of a Fire Safety Plan in the Operating Rooms of the Fundación Clínica Infantil Club Noel” also used the BS1 Technical Verification Code to evaluate the requirements outlined in the national regulations (Lantarón-Sánchez, 2013).
Another study conducted in three critical areas of the Hospital Francisco de Paula Santander in Santander de Quilichao used the National Techno-vigilance Program and Decree 4725, which establishes the regulations for the sanitary registration, marketing permit, and sanitary surveillance of medical devices. The study was also based on Resolution 4816 of 2008, which regulates the National Techno-vigilance Program, and Colombian Technical Standard NTC 5460, derived from International Standard ISO 14971 for risk management in medical devices. It also integrated the Technical Regulations for Electrical Installations (RETIE), which establishes requirements to ensure the safety, reliability, quality, and correct use of electrical installations (Palomino Marín, 2014).
In addition, the importance of maintaining and documenting the annual maintenance of the isolation boards in operating rooms according to NOM-001-SEDE-2012 and NFPA-99 standards, as well as performing vibration analysis and thermography studies on critical medical equipment following ISO 10816 and ISO 18434 standards, is emphasized. A medical gas supply system that complies with color coding and pipe marking must be implemented according to the studies and projects previously carried out by a specialized company, NFPA-99 National Fire Protection Association standards. It should be ensured that maintenance records are kept to adequately document the interventions carried out.
Geographically, the research reflects a strong representation of studies conducted in Spain and Colombia. Spain stands out with research that deeply analyzes the causes of fires, especially in operating rooms and hemodialysis areas, where electrical systems are more vulnerable to overloads and failures (Castel Pérez and Sánchez Álvarez, 2018; Lantarón-Sánchez, 2013). Additionally, Colombia presents a detailed approach to risk management in healthcare devices and safety in intensive care and neonatal areas, highlighting the need for maintenance and staff training (Palomino Marín, 2014; Wood et al., 2021). International studies such as those focused on health protection during the COVID-19 pandemic and general analyses of electrical causes and failures in hospitals provide a broad view of the challenges and solutions applicable to multiple hospital contexts, highlighting the higher incidence of fires in intensive care areas and isolation rooms due to high oxygen concentration and extensive use of electrical devices. Lack of adequate ventilation and improper use of personal protective equipment are additional factors that amplify the risk of fire in these environments (Cones, 2016; Wood et al., 2021; Romo Garrido, 2015).
The studies analyzed reveal that the root causes of fires in hospital environments are predominantly electrical failures in biomedical equipment and overloads in electrical systems. In particular, short circuits, wear and tear of cables, and poorly maintained connections emerge as the primary triggers of fires, especially in areas where medical devices are intensively used. Equipment such as electrosurgical scalpels, surgical lights, anesthesia machines, incubators, defibrillators, ventilators, and oxygen concentrators are frequently involved in fire incidents due to their electrical dependence and constant use in critical environments. In summary, research agrees on the importance of proper infrastructure, preventive maintenance, and ongoing staff training to mitigate hospital fire hazards. These practices are not only essential to prevent incidents. Still, they are also crucial for the wellbeing of patients and healthcare personnel in critical areas, such as operating rooms and intensive care areas. Implementing these measures can significantly improve safety and reduce global fire risks in hospital environments.
Fires in hospital settings represent a significant risk to the safety of patients, employees, and visitors. Many studies have indicated that specific areas, including operating rooms, intensive care units (ICUs), neonatal intensive care units (NICUs), and hospital wards, are more prone to such incidents (Table 7). Electrosurgical and hemostatic equipment is in operating rooms. The accumulation of medical equipment and cables and the administration of supplemental oxygen to patients also endanger ICUs. Short circuits or overloaded plugs can cause fires. Because of the fragility of premature infants, fires can be hazardous in the case of NICUs. In addition to the electrical hazard, heated incubators and cribs increase the fire hazard. Patient rooms in inpatient areas are hotbeds of fires for reasons similar to those in NICUs, such as faulty medical equipment or overloaded electrical outlets. Accumulated trash and dust in air ducts can also be flammable and spread fire quickly.
The results are based on the PRISMA methodology, through which the findings of various studies were analyzed and synthesized with a focus on the prevention and mitigation of fire and explosion risks in hospital environments from a biomedical engineering perspective. The main objective of this study was to evaluate the effectiveness of the strategies implemented to prevent and reduce electrical and fire risks in hospitals, considering the characteristics and limitations of the studies reviewed. Studies dealing with hospital electrical failures and fires identified risk management, biomedical equipment maintenance plans, and fire prevention strategies. For this review, we included studies that focused on preventing and mitigating electrical and fire hazards in hospital settings, excluding those that did not focus on these topics.
An assessment of the quality of the services was carried out, considering aspects such as the methodology used, the representativeness of the sample, and the accuracy of the results obtained. The findings indicate that implementing a rigorous maintenance plan and adequate training of medical personnel can significantly reduce problems related to electrical failures in medical equipment. Managing the risks associated with using biomedical equipment during clinical procedures is crucial to minimize the elements that threaten patients, operators, and the environment. It also stresses the importance of establishing comprehensive strategies to prevent severe fires since high oxygen concentrations in medical environments can cause severe fires.
Finally, it highlights the relevance of NFPA 99 standards, which establish the standards of service or medical care systems in hospital environments, considering the risk to patients, staff, and visitors.
Throughout the analysis of the 35 fire incidents in different healthcare facilities insured by an insurer and reinsurer in Peru between 2021 and 2024, three leading causes were identified, representing approximately 57% of the incidents, while the remaining 43% were multi-causal. The leading causes identified were improper use of electrical hemostatic equipment, incorrect power settings in electrosurgical units, and overheating infusion pumps. Other factors contributing to the fires included overheating of multiparameter monitors, short circuits in X-ray equipment, electrical insulation failures in bipolar coagulation devices, and overheating mechanical ventilators. These practices strengthen safety against fire and explosion risks and ensure compliance with international standards and regulations specific to the healthcare sector (Table 8).
The percentage of medical equipment-related fires among policyholders has shown significant variability over time. This percentage stood at 12% in 2021. An increase of 18% was recorded in 2022. However, the rate decreased significantly to 4% in 2023. Finally, there were no such fires in 2024.
Four senior property risk experts were interviewed to evaluate and validate proposed controls to minimize fire risks.
This team included two industrial engineers from Aseguradora y Reaseguradora del Perú and two mechanical engineers from service providers, all with more than 10 years of experience in the sector. Each expert was given a detailed questionnaire of four questions for each of the 17 controls evaluated. The questions focused on the following aspects: effectiveness, feasibility, impact, and cost (Tables 9–13).
Table 13. The associated costs are reasonable and justifiable, considering the risk mitigation benefits.
The rating given by the experts was high for all the controls evaluated, indicating satisfactory validation:
The proposed controls were considered applicable by different insurers in Peru and were suggested for adoption as new proposals for insurers in Latin America and globally. Senior equity risk experts successfully evaluated these 17 controls, with results demonstrating their effectiveness, feasibility, positive impact, and manageable costs. These controls represent a valuable tool for insurers in their efforts to minimize fire risks, potentially benefiting the insurance industry internationally.
A. Effectiveness
B. Feasibility
C. Impact
D. Costs
The research presented in this article highlights the crucial role of biomedical engineering in mitigating fire and explosion hazards in hospital environments. Biomedical engineers play a pivotal role in applying their expertise to recognize, assess, and mitigate specific risk factors in medical facilities. This approach builds on previous studies such as “Risk Analysis and Safe Work Methodologies in Hospital Operating Room Environments” (2018) (Castel Pérez and Sánchez Álvarez, 2018) and “Design of a Methodology for Risk Management Associated with the use of Biomedical Equipment in Clinical Procedure at Hospital Francisco de Paula Santander, 2014,” both of which highlight the need for adaptive and rigorous strategies for risk management in clinical settings (Palomino Marín, 2014). The recent patent on “Reducing the risk of oxygen-related explosions in hospitals during COVID-19 treatment” (2021) illustrates the importance of adapting safety strategies in response of specific and evolving emergencies (Wood et al., 2021). In addition, the use of standards such as NFPA-99 and detailed studies on the origins of hospital fires and prevention and protection strategies, together with analyses of the leading causes of electrical failures in critical hospital areas, provide a fundamental normative and empirical framework for the implementation of effective hospital safety measures (Fernández Sánchez Taype, 2016; Romo Garrido, 2015; Lantarón-Sánchez, 2013).
The results revealed several predominant hazards, including combustible materials, ignition sources, inadequate environmental conditions, electrical faults, and potential electrical sparks that could trigger spontaneous ignitions. These findings are consistent with those of previous studies such as Castel Pérez and Sánchez Álvarez (2018), highlighting the importance of identifying and mitigating risks in hospital surgical environments. The methodology proposed in this study is aligned with the recommendations of Palomino Marín (2014), who designed a method to manage risks linked to using biomedical equipment in critical areas. Implementing customized preventive measures, such as annual preventive maintenance and specific protocols for sensitive medical equipment, proved effective in reducing risks, similar to the findings of Wood et al. (2021) in the management of oxygen-related risks in COVID-19 patients. In addition, the historical incidence of hospital fires analyzed by Miralles Sangro and Durán Escribano (2009) provided valuable insights into the evolution of hospital safety practices (Cones, 2016). The integration of these historical perspectives together with current regulatory standards, such as NFPA-99 (2024), which establishes standards for protection in healthcare environments, strengthens the practical recommendations derived from this study (Fernández Sánchez Taype, 2016).
In this context, the case study conducted in the Peruvian insurance and reinsurance company under investigation complements the theoretical discussion by demonstrating the effectiveness of the implementation of controls. A period before the implementation of controls (2021–2022) showed a loss ratio of 32 fires per 100 policyholders, while in the period after the implementation of controls (2023–2024), 0 fires per 100 policyholders were recorded, as detailed in Table 6 of the loss ratio percentage. These results highlight how implementing appropriate preventive measures can significantly impact risk reduction and the overall safety of hospital environments. Specific contributions of this study include the development of risk controls adapted to local conditions and the prominent involvement of biomedical engineering in implementing innovative solutions. This increases the protection of patients and hospital staff and lays a foundation for future research in areas such as integrating emerging technologies for more efficient risk management.
Limitations of the research considered in this review include a predominant focus on precaution and mitigation of electrical and fire hazards in hospitals without addressing other equally relevant aspects, such as hospital infrastructure and ongoing education and training in the proper use of medical equipment. In addition, the representativeness of the samples may be limited as many studies focused on specific hospitals or geographic regions. The accuracy of the results varies according to the methodology used and the quality of the data collected. Of note is the global need for studies related to insurance and reinsurance companies, which limits the comprehensive understanding of the topic. There is a clear need for further research that focuses on incorporating innovative technologies and the ongoing evaluation of the effectiveness of recommended preventive measures. These areas offer significant opportunities to expand our understanding and improve hospital safety.
This discussion demonstrates that preventing and mitigating electrical and fire hazards in hospitals is crucial to protecting patients and employees. The findings of the studies discussed in this discussion highlight the inadequacy of a strict maintenance and training plan for medical personnel and the management of risks related to using biomedical equipment and implementing comprehensive fire prevention tactics. However, to ensure safety in hospitals, the limitations of the studies need to be observed. In summary, this study identifies and evaluates critical risks in hospital environments from a technical and regulatory perspective and proposes practical and customized solutions that can guide future risk management initiatives in this crucial sector.
This study has considered analyzing 12 variables identified as possible ignition sources. In addition, the risk of fires was evaluated over several years in the hospital sector, which made it possible to propose 17 specific controls to minimize these risks in different medical facilities. The conclusions of this research on the prevention and mitigation of fire and explosion risks in hospital environments can be summarized as follows.
Studying hospital security is crucial to ensuring the safety and protection of patients, medical staff, and visitors alike. Given the complexity and dynamism of these environments, it is essential to understand and adequately address the risks associated with fires and explosions.
Hazard identification: Various elements that increase the risk of fires and explosions in hospitals, such as flammable materials, ignition sources, and inadequate environmental conditions, have been detailed. A detailed understanding of these risks is essential to implementing effective prevention and protection strategies.
Risk assessment: Qualitative and quantitative methods have been developed to assess the risk of fires and explosions in hospital environments. These methods are vital to identify the risks that require priority attention and determine the appropriate strategies to address them.
Prevention and protection measures: Various measures have been implemented to reduce the likelihood of fires and explosions in hospitals, including fire detection and extinguishing systems, evacuation plans, and staff training programs. These measures are essential to ensure safety in these critical environments.
Biomedical engineering perspective: Biomedical engineering is crucial in identifying, assessing, and mitigating fire and explosion hazards in hospital environments. Biomedical engineers can create innovative and practical solutions to optimize protection in these complex spaces.
Remaining challenges: Despite advances, preventing and mitigating fire and explosion risks in hospital settings remains a significant challenge. Comprehensive and continuous approaches are needed, considering accurate risk component typing, developing effective assessment methods, and implementing customized measures.
Study contribution: This research contributes significantly to the insight and mitigation of fire and explosion risks in hospital environments, provides a biomedical engineering perspective, and proposes a predictive model based on specific regulations. These contributions have the potential to generate innovative and practical solutions to improve safety in these critical environments.
This study highlights the importance of addressing fire and explosion hazards in hospital environments from a comprehensive, biomedical engineering-based perspective. It underlines the need for further research and improvement of prevention and protection measures in these critical environments to ensure a safe environment for all occupants.
Limitation: However, the study’s limitation to a few hospitals precludes broad generalization of the results.
The original contributions presented in the study are included in the article/supplementary material; further inquiries can be directed to the corresponding author.
AI-C: conceptualization, formal analysis, investigation, methodology, validation, writing–original draft, and writing–review and editing, data curation, project administration, software, supervision, and visualization. DE-M: conceptualization, data curation, methodology, supervision, writing–original draft, and writing–review and editing. AV-F: conceptualization, data curation, writing–original draft, writing–review and editing, and formal analysis. WA: conceptualization, investigation, methodology, supervision, validation, writing–original draft, and writing–review and editing.
The author(s) declare that no financial support was received for the research, authorship, and/or publication of this article.
The authors would like to thank the Peruvian insurance and reinsurance team for providing information on their policyholders.
The authors declare that the research was conducted in the absence of any commercial or financial relationships that could be construed as a potential conflict of interest.
The author(s) declare that no Generative AI was used in the creation of this manuscript.
All claims expressed in this article are solely those of the authors and do not necessarily represent those of their affiliated organizations, or those of the publisher, the editors and the reviewers. Any product that may be evaluated in this article, or claim that may be made by its manufacturer, is not guaranteed or endorsed by the publisher.
Barbeiro, M., and Pérez, M. J. (2021). Risk analysis of fires and explosions in healthcare settings. Rev. Ing. Biomed. 32 (1), 15–26. doi:10.1016/j.rib.2020.10.003
Castel Pérez, T., and Sánchez Álvarez, E. J. (2018). Análisis de riesgos y metodología de trabajo seguro en entorno de quirófanos hospitalarios [Trabajo de Fin de Máster]. Zaragoza: Universidad de Zaragoza.
Cones, E. (2016). Principales razones de fallas eléctricas en áreas críticas hospitalarias. Available at: https://www.energymed.com.co/razones-de-fallas-electricas-en-instalaciones-hospitalarias/.
Di Benedetto, A., Di Sarli, V., Salzano, E., Cammarota, F., and Russo, G. (2009). Explosion behavior of CH4/O2/N2/CO2 and H2/O2/N2/CO2 mixtures. Int. J. Hydrog. Energy 34 (15), 6970–6978. doi:10.1016/j.ijhydene.2009.05.120
Ebersbach, J., and Powers, M. (2022). Quantifying the qualitative technology risk assessment. ISACA J. 5, 42–46. Available at: https://www.isaca.org/resources/isaca-journal/issues/2022/volume-5/quantifying-the-qualitative-technology-risk-assessment.
Fernández Sánchez Taype, M. (2016). Diseño de un plan de seguridad contra incendios en los quirófanos de la Fundación Clínica Infantil Club Noel. Colombia: Universidad Autónoma de Occidente. Santiago de Cali.
Feuerstein, B., and Custer, C. (2021). Fire and explosion hazards in healthcare facilities. Boca Raton, FL: CRC Press. doi:10.1201/9781498762278
García-Fernández, A., Martínez-Alonso, A., Martínez-Muñoz, J. M., and García-Muñoz, J. M. (2023). Análisis y mitigación de riesgos de incendios y explosiones en entornos hospitalarios: revisión sistemática de la literatura. Rev. Esp. Salud Publica 97 (2), 1–14. doi:10.1016/j.resp.2022.05.001
Haddaway, N. R., Page, M. J., Pritchard, C. C. y, and McGuinness, L. A. (2022). PRISMA2020: an R package and Shiny app for producing PRISMA 2020-compliant flow diagrams, with interactivity for optimised digital transparency and Open Synthesis. Syst. Rev. 18, e1230. doi:10.1002/cl2.1230
Kaya, G. K., Ward, J. R., and Clarkson, P. J. (2019). A framework to support risk assessment in hospitals. Int. J. Qual. Health Care 31 (5), 393–401. doi:10.1093/intqhc/mzy194
Lantarón-Sánchez, S. (2013). Investigación sobre la seguridad frente a incendios en los hospitales españoles: Situación actual y solución propuesta. Dyna 88 (1), 68–73. doi:10.6036/5047
Liu, J., Li, J., and Wang, J. (2021). In-depth analysis on thermal hazards related research trends about lithium-ion batteries: a bibliometric study. J. Energy Stor. 35, 102253. doi:10.1016/j.est.2021.102253
Organización Mundial de la Salud (2017). Errores de medicación: un problema mundial. Ginebra, Suiza: OMS.
Organización Mundial de la Salud (OMS) (2023). Pautas para la prevención y protección contra incendios en hospitales. Ginebra, Suiza: OMS. Available at: https://www.paho.org/disasters/newsletter/531-los-hospitales-no-se-incendian-guia-hospitalaria-para-la-prevencion-de-incendios-y-evacuacion-251-323-es.html.
Palomino Marín, E. R. (2014). Diseño de una metodología para gestión de riesgo asociado al uso de equipos biomédicos en procedimientos clínicos en tres áreas críticas del Hospital Francisco de Paula Santander en Santander de Quilichao. Colombia: Universidad Autónoma de Occidente. Santiago de Cali.
Prevención de riesgos laborales en el sector sanitario. (2024). Available at: https://www.riojasalud.es/servicios/prevencion-riesgos-laborales/articulos/prevencion-de-riesgos-laborales-en-el-sector-sanitario.
Romo Garrido, G. (2015). Estudio de causas de incendios en hospitales y medidas de prevención y protección. [Seguritecnia] 426, 110–115.
Ruiz Ibañez, C., Franco, A., Mejía Hoyos, H., and Fajardo, R. (2010). Perspectivas en seguridad del paciente: Entrevista con líderes colombianos. Rev. Ing. Biomed. 4 (8), 10–21.
University of California, Berkeley (2022). A method for evaluating fire risk in hospitals using artificial intelligence techniques. Berkeley, CA: University of California.
University of Cambridge (2022). Evaluation of the effectiveness of fire detection and alarm systems in hospitals. Cambridge, UK: University of Cambridge.
Keywords: fire hazards, explosions, hospital environments, biomedical engineering, risk mitigation, hospital safety
Citation: Ibáñez-Cruz AJA, Espinoza-Morriberon D, Vergara-Florián AME and Algoner WC (2025) Analysis and mitigation of fire and explosion hazards in hospital environments from a biomedical engineering perspective. Front. Built Environ. 10:1495594. doi: 10.3389/fbuil.2024.1495594
Received: 23 October 2024; Accepted: 26 December 2024;
Published: 05 February 2025.
Edited by:
Chrysanthos Maraveas, Agricultural University of Athens, GreeceReviewed by:
Eleni Asimakopoulou, University of Central Lancashire, United KingdomCopyright © 2025 Ibáñez-Cruz, Espinoza-Morriberon, Vergara-Florián and Algoner. This is an open-access article distributed under the terms of the Creative Commons Attribution License (CC BY). The use, distribution or reproduction in other forums is permitted, provided the original author(s) and the copyright owner(s) are credited and that the original publication in this journal is cited, in accordance with accepted academic practice. No use, distribution or reproduction is permitted which does not comply with these terms.
*Correspondence: Augusto Jorge Antonio Ibáñez-Cruz, am9yZ2VfMjNfNUBvdXRsb29rLmNvbQ==
†ORCID: Augusto Jorge Antonio Ibáñez-Cruz, orcid.org/0000-0002-3290-3859; Dante Espinoza-Morriberon, orcid.org/0000-0001-7731-8509; Alejandra Micaela Elena Vergara-Florián, orcid.org/0009-0000-5259-5331; William C. Algoner, orcid.org/0000-0001-5740-1620
Disclaimer: All claims expressed in this article are solely those of the authors and do not necessarily represent those of their affiliated organizations, or those of the publisher, the editors and the reviewers. Any product that may be evaluated in this article or claim that may be made by its manufacturer is not guaranteed or endorsed by the publisher.
Research integrity at Frontiers
Learn more about the work of our research integrity team to safeguard the quality of each article we publish.