- Department of Civil and Infrastructure Engineering, Indian Institute of Technology Dharwad, Dharwad, India
This manuscript presents a mini review on the potential new application of geocell in the mitigation of ground vibration. An effort has been made to provide a detailed quantification and mechanisms involved in the vibration isolation performance of geocells. The latest studies on the topic are summarized in three sections, namely, experimental, numerical, and analytical studies. Details on the optimum dimension of geocells, depth of placement, and suitable infill materials for effective isolation of vibrations have been covered. Further, the mechanism responsible for the superior vibration isolation performance of the geocells is highlighted. In addition, analytical equations and machine learning-based models for predicting the isolation performance of the geocells are discussed. The study reveals that geocell is very effective in isolating ground vibrations as compared to other forms of geosynthetics. Geocells were found to enhance dynamic properties and reduce the amplitude of vibrations significantly.
1 Introduction
Geocells are 3-dimensional (3D) shaped geosynthetics made of high-density polymers and polymeric alloy material. It is popularly known as a 3D cellular confinement system. The US Army Corps of Engineers initially developed geocell for military applications in the early 1970s. Later, the use of geocells has seen significant growth in civil engineering applications. Due to its honeycomb shape, geocell offers all-round confinement to the soil, leading to enhanced strength properties. General applications of geocells include foundations (Hegde and Sithara, 2013), embankments (Sitharam and Hegde, 2013), pavements (Hegde and Palsule, 2020), earth-retaining structures (Latha and Manju, 2016) and buried pipelines (Hegde and Sithara, 2015a). Generally, geocell pockets are filled with granular materials. However, the study of Hegde and Sitharam (2015b) suggested that different infill materials, namely, gravel (coarse aggregate) and silty clay can also be used as infill materials. Some recent studies have also recommended using waste materials like recycled asphalt pavement (Han et al., 2011; Thakur et al., 2013), construction waste (Mahima and Sini, 2022), quarry waste (Pokharel et al., 2011), and steel slag (Venkateswarlu and Hegde, 2020a) to fill the geocell pockets.
Nowadays, the topic of ground vibrations and its mitigation has obtained great interest among researchers. Construction activities, transit systems, and heavy industrial equipment are some of the common sources of vibrations. The induced vibrations from the source can cause severe trouble to the buildings and surrounding environment. The vibrations can trigger smaller cracks to catastrophic damage in the structures. The harmful effects of the vibrations can be mitigated using various methods. These methods include the use of rows of solid piles (Kattis et al., 1999), gas mattresses (Massarsch, 2005), and open and in-filled trenches (Jauhari et al., 2023). Isolation techniques can be categorized into two types, namely, active and passive vibration isolations, based on the distance between the source and the barriers. In active isolation, barriers are placed near the vibration source in order to attenuate the amplitude of vibration. Whereas in passive isolation technique, the barriers are placed near the structures to be protected. The open and infilled trenches are used in both active and passive isolation schemes. However, sometimes the depth requirement in open and infilled trenches is so large that it cannot always be practically feasible. The recent research activities suggest the potential use of the geocells in mitigating the vibrations. The geocells are placed below the vibration sources and hence, it can be classified as active isolation method. Geocells diffract the vibration energy into wider areas below the vibration source and hence, prevent lateral transmission (Venkateswarlu and Hegde, 2023a). The present manuscript summarizes some of the recent advances on the use of geocells in mitigation of ground vibrations. Past research studies are summarized in different sections, namely, experimental, numerical, and analytical studies.
2 Experimental studies
Field vibration tests are the preferred method for studying the vibration mitigation performance of geocells. In this method, dynamic vibrations of different magnitudes and frequencies are applied on the foundation bed strengthened with geocells using a mechanical oscillator. The vibration response of the foundation beds is measured using geophones, accelerometers, and vibration meters. Figure 1 shows the schematic view of the field vibration test setup. Venkateswarlu et al. (2018) highlighted the isolation potential of different geosynthetics-reinforced beds using vertical mode field vibration tests. Different cases, namely, one layer geogrid reinforced, multi-layers of geogrid reinforced and geocell reinforced conditions were tested and compared with unreinforced case under vibration loading. The different cases were compared in terms of frequency and displacement corresponding to resonance. Experimental results revealed that the amplitude of the vibration corresponding to resonance extensively reduces in the presence of geogrids. As compared to geogrids, the reduction in amplitude of vibration was observed maximum in the case of the geocell reinforcement. About 61% decrease in the amplitude of vibration was observed in the case of the geocell reinforcement as compared to the unreinforced condition. Similarly, the frequency corresponding to the resonance of the soil system was increased by 1.38 times in the case of the geocell reinforcement as compared to the unreinforced condition.
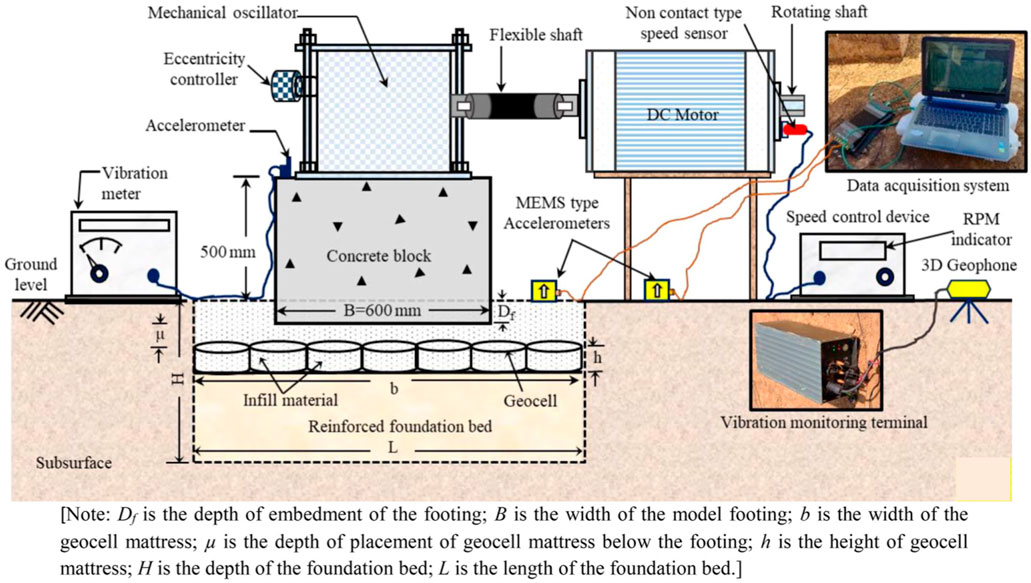
Figure 1. Schematic view of the field vibration test setup [Sourced from Venkateswarlu and Hegde (2020a)].
Hegde and Venkateswarlu (2019) highlighted traffic-induced vibration isolation using geocells. The optimal placement depth of geocell was recommended as 0.1B (B is the width of vibration source) for the effective reduction of traffic vibration. At the optimum depth of placement of geocell, the natural frequency of subgrade was increased by 1.4 times. Similarly, 96% increase in the elasticity of the subgrade was reported. Experimental investigation of Venkateswarlu and Hegde (2020a) provided the recommendations for the geocell geometry, placement depth for achieving maximum isolation efficiency. The optimum width and depth of placement of geocell were found to be 5B and 0.1B (B is width of vibration source) respectively, for obtaining the maximum isolation. Venkateswarlu and Hegde (2020b) studied the influence of infill materials on the isolation efficacy of a geocell-strengthened foundation. Different types of infill materials, namely, silty sand, sand, steel slag, and gravel, were used in the study. Among the four different infill materials, gravel infill offered the maximum isolation efficiency. More than 150% increase in the shear modulus of the foundation bed was reported in the presence of geocell with gravel infill.
3 Numerical studies
Due to the complexity involved in the experimental procedures, numerical modeling is a handy tool in assessing the vibration separation performance of the geocells. Ujjawal et al. (2019) performed FLAC3D based numerical analysis to investigate the effectiveness of geocell reinforcement in isolating the machine induced vibrations. In the study, two different geocell modelling approaches, namely, equivalent composite approach (Venkateswarlu and Hegde, 2018), and honeycomb shape approach (Hegde and Sitharam, 2015c; Hegde and Sitharam, 2015d) were adopted. In the equivalent composite approach, the geocell and the infill soil are treated together as a composite mass with enhanced shear strength properties. In the honeycomb shape approach, the actual curvature of the geocell pockets is considered in the modelling. In addition, the geocell materials and infill soils are modeled separately using different materials models. From the study, the honeycomb shape approach (HSA) was found to provide an accurate prediction of the experimental results. Further, the parametric study showed that the isolation performance of the geocell reinforced bed was significantly affected by the modulus of the geocell material and the interface friction angle. The increase in the geocell modulus and the interface friction angles were found to enhance the overall performance of reinforced foundation beds under vibration loading. The optimum cell depth of 0.25B was suggested for better isolation performance.
Venkateswarlu and Hegde (2023a) provided numerous insights into the vibration isolation mechanism of geocell reinforced beds using three-dimensional numerical modelling using the finite-difference package FLAC3D. Wave propagation pattern revealed that the provision of geocell mattress diffracts the induced vibration energy into a wider area beneath the geocell mattress as shown in Figure 2. As per section ‘J’ in the figure, the geocell offers confinement to the foundation soil, causing an increase in the shear strength. Section ‘K’ in the figure indicates the dispersion of the laterally moving vibration waves into downward direction due to the presence of geocell pockets. Further, due to the presence of a geocell mattress, the vibration was found to spread to the wider areas (as represented in section ‘L’) at an angle ranging between 50° and 63°. Dissipation of the vibration load into wider areas improves the isolation efficiency. Further, it was also observed that the foundation bed strengthened with the geocell can effectively mitigate vibrations up to the frequency range of 0–50 Hz. Further, Venkateswarlu and Hegde (2023b) demonstrated the influence of the shape of footing, geocell area, and the relative density of infill on the behavior of geocell reinforced bed. More than 75% reduction in foundation bed strain was observed in the presence of geocell reinforcement for different frequency and relative density conditions.
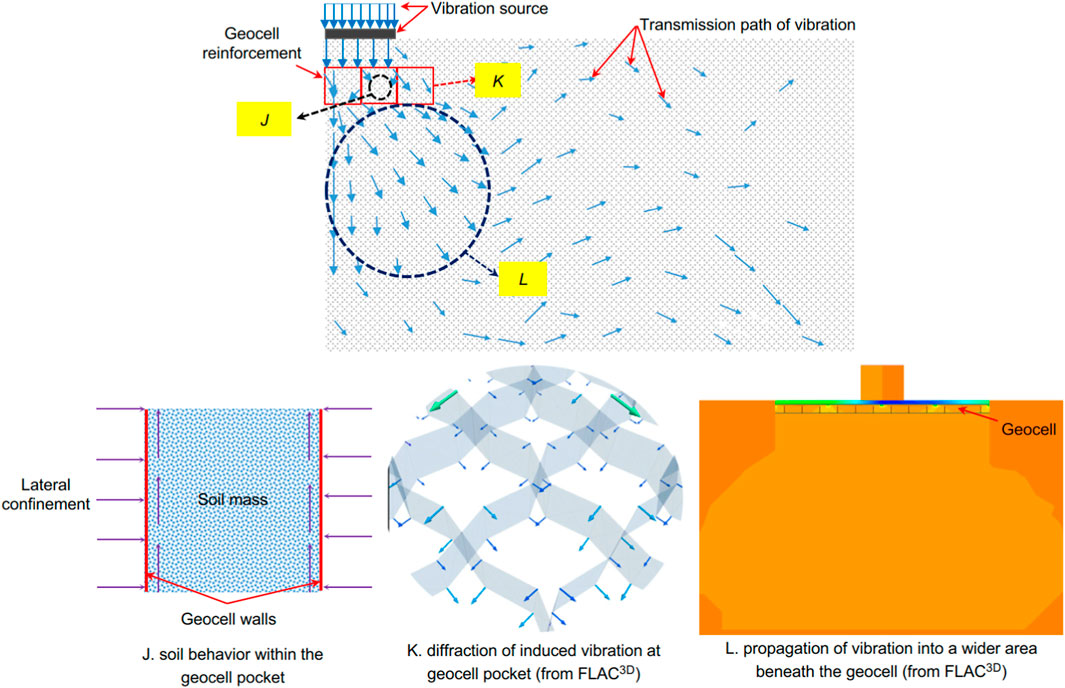
Figure 2. Vibration Isolation mechanism of geocell reinforced foundation beds [Sourced from Venkateswarlu and Hegde (2023a)].
4 Analytical and machine learning studies
The estimation of the response of the foundation beds for a given vibration loading is essential for designing the vibration barriers. Researchers have adopted various methods, such as the mass-spring dashpot method and different machine learning-based methods for predicting the vibration response of the geocell-reinforced foundation beds. Venkateswarlu and Hegde (2020b) demonstrated the suitability of the mass-spring dashpot (MSD) analogy in predicting the vibration response of geocell strengthened foundation beds. Geocell was found to enhance the damping properties of the foundation bed significantly. The total damping ratio values of 12%, 26%, 28%, 32%, and 36%, respectively, were observed for different cases, namely, unreinforced, geocell infilled with silty sand, geocell infilled with sand, geocell infilled with slag, and geocell infilled with gravel.
Venkateswarlu et al. (2021) examined the applicability of genetic programming (GP), and multivariate adaptive regression spline (MARS) models in predicting the peak particle velocity (PPV) of geocell strengthened soil beds. In total, 240 datasets obtained from field vibration tests were used to predict the PPV. Sensitivity analysis suggested that the damping properties of the foundation beds influence the PPV most. Compared to the GP model, the MARS model exhibited high precision in calculating the PPV. Venkateswarlu et al. (2023c) developed a prediction model for computing the displacement amplitude of vibration in the geocell strengthened foundation bed using the combination of the artificial neural network (ANN) with dragonfly optimizer (DFO), abbreviated as ANN-DFO. The reliable database obtained from the field vibration tests was used for the development of model. The displacement amplitude of vibration of the geocell reinforced foundation bed was predicted using the set of input parameters involving the foundation bed, geocell, and vibration loading characteristics. Figure 3 shows the schematic representation of the methodology followed in the model development. The performance of the models was evaluated using the different statistical parameters, namely, coefficient of determination (R2), mean absolute percentage error (MAPE), root mean square error (RMSE), mean absolute error (MAE), and mean arctangent absolute percentage error (MAAPE). From the results, an excellent prediction performance of the displacement amplitude was observed for the ANN-DFO model as compared to other benchmark models, namely, Gaussian process regression (GPR), random forest (RF), and M−5 rules. Based on the sensitivity analysis results, the displacement amplitude of the reinforced foundation bed was found to be significantly influenced by the frequency of the vibration source.
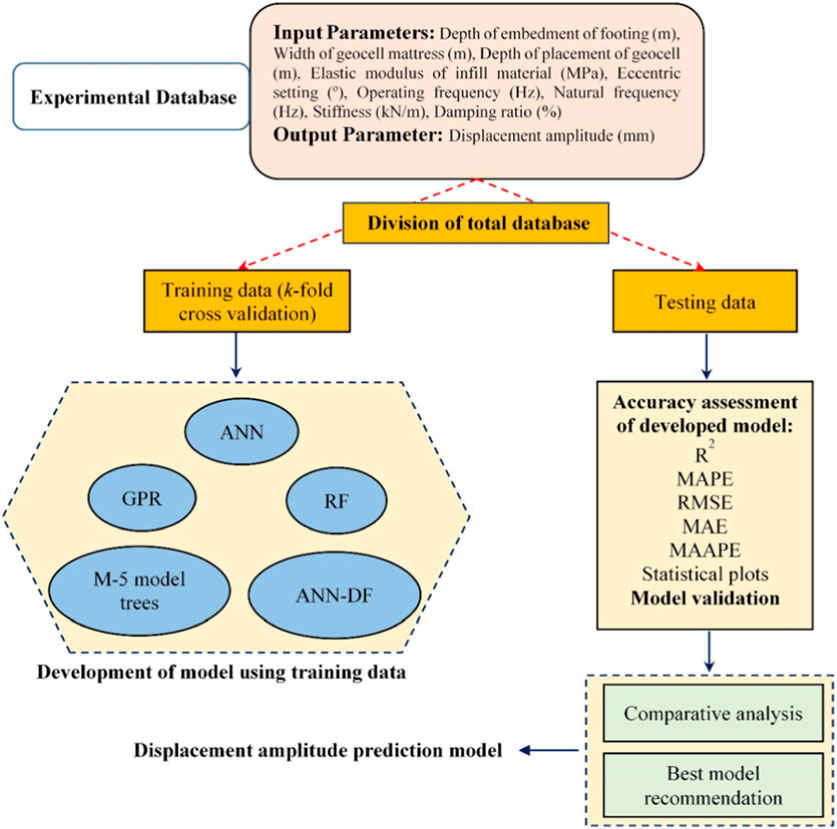
Figure 3. Schematic representation of methodology adopted by Venkateswarlu et al. (2023c).
5 Prospects of hybrid techniques
It is understood from past studies that geocells are effective in reducing vibrations up to 50 Hz (Venkateswarlu and Hegde, 2023a). On the contrary, open and infilled trench systems are preferred to isolate the vibrations with much higher frequencies. Jauhari et al. (2023) performed field tests to assess the usefulness of open and infilled trenches in the mitigation of continuous harmonic vibrations. Furthermore, to complement the field study, comprehensive numerical studies were performed by Jauhari et al. (2024a). The aforementioned studies showed that the trench systems were notably more effective in isolating high-frequency vibrations (>30 Hz). The depth requirement of the trenches will be lower for high-frequency vibration loads. In order to isolate the vibrations of a wide range of frequencies covering the entire spectrum of very low to high-frequency vibration loads, the prospects of using a hybrid technique must be explored. In the hybrid technique, the combination of geocells and the trench system will be used. A schematic view of the hybrid system is shown in Figure 4. Below the vibration load, the geocell reinforcement is to be placed, and the trench system in the form of an open or infilled barrier is to be placed beside the vibration loads. It is expected that the combination of geocells and trench systems can isolate the vibrations of the wide range of frequencies. However, further studies are essential to obtain mechanisms and the optimum dimensions of hybrid systems. It is recommended to use the dual trenches instead of the single trench systems. As compared to single trench, the additional trench in the dual trench system helps to reflect and scatter the incoming waves, causing better screening in the isolation zone. Further, the dual trenches reduce the higher depth requirement of the single trenches.
6 Summary and conclusions
The manuscript summarized the research studies on the new application of geocells in vibration isolation. The isolation scheme developed by the researchers suggested that the foundation bed below the vibration source needs to be reinforced with geocells for isolating the vibrations. For effective vibration mitigation, the optimum cell depth, width, and depth of placement of geocell were found to be 0.25B, 5B, and 0.1B, respectively (B is the width of the vibration source). Moreover, the gravel was found to be the most suitable infill material for better performance. In the presence of geocell reinforcement, the resonant frequency of the soil system was increased by 1.38 times and the resonant amplitude was decreased by 61% as compared to the unreinforced condition. Geocells were found to spread the vibration load to the wider area below the vibration source to reduce the side effects of vibrations. Further, the vibration was found to disperse at an angle ranging between 50° and 63° below the geocell. The foundation bed reinforced with the geocell was found suitable for isolating the vibration loading with a frequency up to 50 Hz. The geocell isolation system can be effectively adopted to reduce the harmful effects of ground vibrations generated from sources such as machine foundations, pavements, and railways. As suggested in the literature, advanced machine learning-based models can be used to estimate the vibration response of the barrier system under a given vibration load. Overall, it can be concluded that initial research efforts have highlighted the positive benefits of using geocells in vibration isolation. However, further studies are essential to fully develop the design guidelines for using the geocells in vibration isolation applications. Also, there is a further need to explore the hybrid technique involving the combinations of geocells and dual trenches for isolation of the ground vibrations.
Author contributions
AH: Conceptualization, Writing–original draft, Writing–review and editing.
Funding
The author(s) declare that no financial support was received for the research, authorship, and/or publication of this article.
Acknowledgments
The author would like to thank and acknowledge the contribution of Dr. Hasthi Venkateswarlu, Indian Institute of Science Bangalore, who assisted the author in performing some of the studies reported in the manuscript.
Conflict of interest
The author declares that the review was conducted in the absence of any commercial or financial relationships that could be construed as a potential conflict of interest.
Publisher’s note
All claims expressed in this article are solely those of the authors and do not necessarily represent those of their affiliated organizations, or those of the publisher, the editors and the reviewers. Any product that may be evaluated in this article, or claim that may be made by its manufacturer, is not guaranteed or endorsed by the publisher.
References
Han, J., Pokharel, S. K., Yang, X., Manandhar, C., Leshchinsky, D., Halahmi, I., et al. (2011). Performance of geocell-reinforced RAP bases over weak subgrade under full-scale moving wheel loads. ASCE J. Mater. Civ. Eng. 23 (11), 1525–1534. doi:10.1061/(asce)mt.1943-5533.0000286
Hegde, A., and Palsule, P. (2020). Performance of geosynthetics reinforced subgrade subjected to repeated vehicle loads: experimental and numerical studies. Front. Built Environ. 6, 15. doi:10.3389/fbuil.2020.00015
Hegde, A., and Sitharam, T. G. (2013). Experimental and numerical studies on footings supported on geocell reinforced sand and clay beds. Int. J. Geotechnical Eng. 7 (4), 346–354. doi:10.1179/1938636213z.00000000043
Hegde, A., and Sitharam, T. G. (2015a). Experimental and numerical studies on protection of buried pipelines and underground utilities using geocells. Geotext. Geomembranes 43, 372–381. doi:10.1016/j.geotexmem.2015.04.010
Hegde, A., and Sitharam, T. G. (2015b). Effect of infill materials on the performance of geocell reinforced soft clay beds. Geomechanics Geoengin. Int. J. 10 (3), 163–173. doi:10.1080/17486025.2014.921334
Hegde, A., and Sitharam, T. G. (2015c). Experimental and numerical studies on protection of buried pipelines and underground utilities using geocells. Geotext. Geomembranes 43, 372–381. doi:10.1016/j.geotexmem.2015.04.010
Hegde, A., and Venkateswarlu, H. (2019). Mitigation of traffic induced vibration using geocell inclusions. Front. Built Environ. 5, 136. doi:10.3389/fbuil.2019.00136
Hegde, A. M., and Sitharam, T. G. (2015d). Three-dimensional numerical analysis of geocell-reinforced soft clay beds by considering the actual geometry of geocell pockets. Can. Geotechnical J. 52 (9), 1396–1407. doi:10.1139/cgj-2014-0387
Jauhari, N., Hegde, A., and Chakrabortty, P. (2023). Full scale field studies for assessing the vibration isolation performance of single and dual trenches. Transp. Geotech. 39, 100932. doi:10.1016/j.trgeo.2023.100932
Jauhari, N., Hegde, A., and Chakrabortty, P. (2024a). Vibration mitigation using dual-open and infilled trenches in layered soil media: field tests and numerical simulations. Comput. Geotechnics 170, 106283. doi:10.1016/j.compgeo.2024.106283
Kattis, S. E., Polyzos, D., and Beskos, D. E. (1999). Vibration isolation by a row of piles using a 3D frequency domain BEM. Int. J. Numer. Methods Eng. 46 (5), 713–728. doi:10.1002/(sici)1097-0207(19991020)46:5<713::aid-nme693>3.0.co;2-u
Latha, G. M., and Manju, G. S. (2016). Seismic response of geocell retaining walls through shaking table tests. Int. J. Geosynth. Ground Eng. 2, 1–15. doi:10.1007/s40891-016-0048-4
Mahima, D., and Sini, T. (2022). Performance evaluation of demolition waste infilled geocell-reinforced subgrade by flexural and rutting analysis. Road Mater. Pavement Des. 23 (8), 1746–1761. doi:10.1080/14680629.2021.1924233
Massarsch, K. R. (2005). “Vibration isolation using gas-filled cushions,” Soil dynamics symposium in honor of professor. Proceedings of the geo-frontiers congress, American society of civil Engineers, january 24–26. Editor R. D. Woods (Austin, Texas, USA), 1–20.
Pokharel, S. K., Han, J., Manandhar, C., Yang, X. M., Leshchinsky, D., Halahmi, I., et al. (2011). Accelerated pavement testing of geocell-reinforced unpaved roads over weak subgrade. J. Transp. Res. Board 2204 (2), 67–75. doi:10.3141/2204-09
Sitharam, T. G., and Hegde, A. (2013). Design and construction of geocell foundation to support the embankment on settled red mud. Geotext. Geomembranes 41, 55–63. doi:10.1016/j.geotexmem.2013.08.005
Thakur, J. K., Han, J., and Parsons, R. L. (2013). Creep behavior of geocell-reinforced recycled asphalt pavement (RAP) bases. ASCE J. Mater. Civ. Eng. 25 (10), 1533–1543. doi:10.1061/(ASCE)MT.1943-5533.0000705
Ujjawal, K. N., Venkateswarlu, H., and Hegde, A. (2019). Vibration isolation using 3D cellular confinement system: a numerical investigation. Soil Dyn. Earthq. Eng. 119, 220–234. doi:10.1016/j.soildyn.2018.12.021
Venkateswarlu, H., and Hegde, A. (2018). Numerical analysis of machine foundation resting on the geocell reinforced soil beds. Geotechnical Eng. J. SEAGS and AGSSEA 49 (4), 55–62.
Venkateswarlu, H., and Hegde, A. (2020a). Effect of influencing parameters on the vibration isolation efficacy of geocell reinforced soil beds. Int. J. Geosynth. Ground Eng. 6 (16), 16–17. doi:10.1007/s40891-020-00205-2
Venkateswarlu, H., and Hegde, A. (2020b). Effect of infill materials on vibration isolation efficacy of geocell reinforced soil beds. Can. Geotechnical J. 57 (9), 1304–1319. doi:10.1139/cgj-2019-0135
Venkateswarlu, H., and Hegde, A. (2023a). Behavior of geocell reinforced bed subjected to vibration loading: insights from 3D numerical studies. Geosynth. Int. 30 (6), 651–670. doi:10.1680/jgein.21.00050
Venkateswarlu, H., and Hegde, A. (2023b). Effect of frequency of loading on vibration isolation efficiency of geocell reinforced beds. Sādhanā 48 (3), 129. doi:10.1007/s12046-023-02198-w
Venkateswarlu, H., Raja, M. N. A., Hegde, A., and Shukla, S. K. (2023c). Experimental and intelligent modelling for predicting the amplitude of footing resting on geocell-reinforced soil bed under vibratory load. Transp. Geotech., 100783. doi:10.1016/j.trgeo.2022.100783
Venkateswarlu, H., Sharma, S., and Hegde, A. (2021). Performance of genetic programming and multivariate adaptive regression spline models to predict vibration response of geocell reinforced soil bed: a comparative study. Int. J. Geosynth. Ground Eng. 7 (3), 63–17. doi:10.1007/s40891-021-00306-6
Keywords: geosynthetics, geocells, field tests, vibrations, mitigation
Citation: Hegde A (2024) Mitigation of ground vibrations using geocells: a mini-review. Front. Built Environ. 10:1428871. doi: 10.3389/fbuil.2024.1428871
Received: 07 May 2024; Accepted: 05 August 2024;
Published: 13 August 2024.
Edited by:
Jie Han, University of Kansas, United StatesCopyright © 2024 Hegde. This is an open-access article distributed under the terms of the Creative Commons Attribution License (CC BY). The use, distribution or reproduction in other forums is permitted, provided the original author(s) and the copyright owner(s) are credited and that the original publication in this journal is cited, in accordance with accepted academic practice. No use, distribution or reproduction is permitted which does not comply with these terms.
*Correspondence: Amarnath Hegde, ahegde@iitdh.ac.in