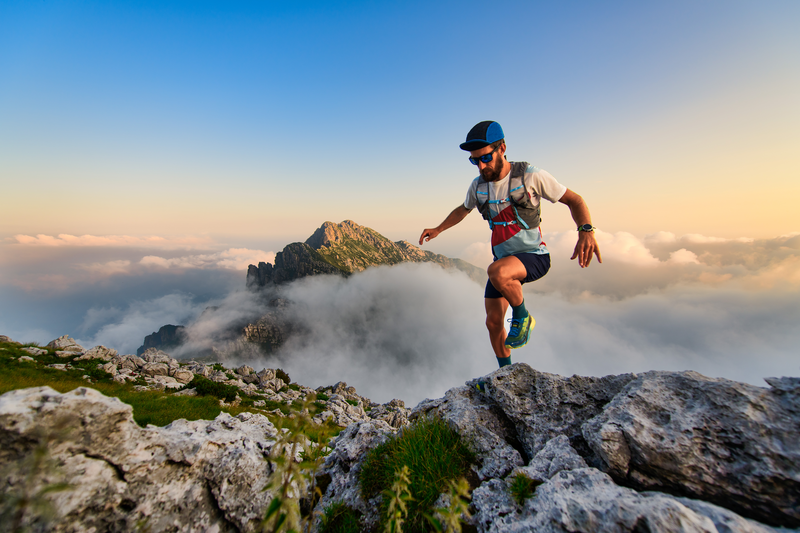
94% of researchers rate our articles as excellent or good
Learn more about the work of our research integrity team to safeguard the quality of each article we publish.
Find out more
ORIGINAL RESEARCH article
Front. Built Environ. , 08 April 2024
Sec. Sustainable Design and Construction
Volume 10 - 2024 | https://doi.org/10.3389/fbuil.2024.1343334
The built environment faces a growing number of challenges due to changing climates. A resilient built environment system (BES) can withstand disruptions and shocks, and resilient design allows communities to bounce back quickly. Considering present and future needs, BESs can be oriented to adapt to new uses or modified to handle changing climates. This study examines the resilience qualities (RQs) of built environment systems (BESs) in responding to and recovering from climate change disruptions effectively. A survey was designed to capture the views of various stakeholders about the different indicators to assess the four RQs: robustness (Rb), redundancy (Rd), inclusivity (Ic), and integration (It). Regulatory and engineering stakeholders participated in the survey, and the results were analyzed using statistical methods. Stakeholders generally agree on the need to enhance transformative capacity for addressing uncertainties and climate challenges. While stakeholders trust the role of BESs’ robustness against climate impacts, some suggest improving standards for better resilience. There is consensus on the importance of regulatory measures mandating emergency resources in BESs. The study highlights the need to enhance adaptive capacities and tools within BESs. Incorporating reconfigurability and spare capacity in BESs is crucial to prevent disruptions. Participants tend to think promoting good practices at the community level is essential to address climate impacts effectively. The analysis highlights the importance of inclusive community consultation and involvement in fostering a shared responsibility for enhancing urban ecosystems against climate change impacts. This involves aligning processes across various city systems to support cohesive decision-making and strategic investments. The study suggests developing objective engineering techniques to establish a standardized approach for evaluating the RQs of BESs.
Resilience is a critical objective for built environment systems (BESs) confronting climate change (Meerow et al., 2016; Tahir et al., 2021). A resilient built environment is crucial to protect human life, property, and economic activity and helps achieve and retain Sustainable Development Goals (SDGs) (Tahir et al., 2021). It can also help ensure communities bounce back from disruptions and continue to thrive (Al-Humaiqani and Al-Ghamdi, 2023a; Al-Humaiqani and Al-Ghamdi, 2023b). Resilience enhances a system’s ability to cope, adapt, and recover from external changes effectively and promptly (Al-Humaiqani and Al-Ghamdi, 2023c; Al-Humaiqani and Al-Ghamdi, 2023d; Serdar and Al-Ghamdi, 2023). A resilient system can swiftly return to its original state or even improve upon it after a disaster. The system’s recovery capability hinges on prior mitigation measures and preparations taken to ensure readiness to withstand such impacts (Rose, 2004; Rose et al., 2007; Zobel, 2011). Resilience encompasses various definitions, all linked to the system’s capacity to anticipate adverse effects, absorb, resist, adapt, and promptly recover from disruptive events (Ayyub, 2020; McAllister, 2013).
The resilience of a BES is directly impacted by its robustness (Rb) and ability to recover from disruptive events quickly. Resilient built environments aim to minimize the impact of shocks on their systems and enhance their adaptive capacity (Bazrkar et al., 2015). Resilience in the built environment involves a combination of essential qualities crucial for designing, constructing, and managing infrastructure that can withstand diverse climate challenges and disruptions. Al-Humaiqani and Al-Ghamdi have identified eight key qualities for BES resilience: reflectivity (Rf), robustness (Rb), redundancy (Rd), flexibility (Fx), resourcefulness (Rs), rapidity (Rp), inclusivity (Ic), and integration (It). Four resilience qualities (RQs), including reflectivity (Rf), flexibility (Fx), resourcefulness (Rs), and rapidity (Rp), have previously been studied and published by the authors (Al-Humaiqani and Al-Ghamdi, 2023e), as explained in Appendix A.
The qualities of Rb and Rd can be compared, and it is evident that structural Rd enhances Rb in uncertain conditions. As demonstrated by Kanno and Ben-Haim, Rd is strongly linked to Rb as Rb bounds strong Rd, making the two qualities identical (Kanno and Ben-Haim, 2011). In general, the Rd of any structure means a structure’s ability to withstand degradation without losing specified elements (Kanno and Ben-Haim, 2011). Rd is essential in the philosophy of structural designs. However, the literature has defined Rd into two concepts: strong and weak, where strong Rd is the lower bound of Rb and may exceed the Rb (Kanno and Ben-Haim, 2011). Strong and weak Rds can be explained against each other. On the other hand, Rb is the ability of a structure to maintain some functionality against environmental uncertainty. Although strong Rd and Rb may be equivalent in many cases, this does not mean only the survivability aspects of a structure after degradation are represented by these two characteristics.
Improving system resilience involves enhancing Rp and ensuring sufficient repair resources are available. This approach encourages assessing resilience as a combination of Rb and Rp (Ouyang, 2017). Additionally, the Rb of systems, such as infrastructure, ecosystems, policies, and strategic plans, contribute to the determination of the ability to build resilience (Birchall and Bonnett, 2021). Building robustness (Rb) is a crucial aspect of resilience, as it pertains to the system’s stability and strength in the face of both short and long-term shocks (Rus et al., 2018; Lak et al., 2020; Shafiei Dastjerdi et al., 2021). Rb is measured by evaluating the system’s performance from the onset of a disruptive event until the point just before restoration begins (Ouyang, 2017). At the urban level, resilient systems require combining planning, efficiency, adaptability, diversity, interdependence, redundancy, strength, and flexibility, as emphasized by Godschalk (2003).
Improving Rb and resistance and introducing efficient recovery strategies can minimize both direct and indirect losses caused by hazards (Bocchini et al., 2014). However, researchers like da Silva et al. (2012) have come to an understanding of the difference between robustness and resilience. For them, resilience is defined as the ability to return a system to a stable equilibrium after disturbance, while Rb focuses on maintaining functionality during the event. However, some researchers argue that the resilience of a critical system is reflected in its performance after a disaster event and before restoration, emphasizing the importance of robustness (Ouyang, 2017). For instance, Kammouh et al. (2020) found a linear relationship between resilience, robustness, and recovery capacity, where higher robustness leads to higher resilience. Similarly, Moghadas et al. (2019) emphasize that resilient cities prioritize robustness and adaptive capacities. Bruneau et al. (Bazrkar et al., 2015) believe that Rb represents the system’s strength to withstand disruption and is used to measure its absorptive capability in reducing negative impacts and consequences.
The concept of Rd plays a crucial role in various design philosophies and structural designs, as it determines the extent to which a system can minimize the impact of a disruption. However, there is still debate about the precise definition of Rd (Kanno and Ben-Haim, 2011). Rd can be described as the system’s capacity to remain functional and maintain its operations in the face of disruptions (Faturechi and Miller-Hooks, 2015; Samsuddin et al., 2018). According to Tong (2021), the built environment resilience dimensions encompass robustness, redundancy, efficiency, and multi-functionality. Furthermore, the relationship between Rd and Rb is demonstrated by two key characteristics (Yazdani and Jeffrey, 2012). The strong Rd is bounded by the Rb, and in some cases, they may share identical qualities (Kanno and Ben-Haim, 2011). On the other hand, insufficient Rd of a system can lead to premature deterioration and eventual collapse. This can be observed in the case of highway bridges constructed in North America during the mid-20th century (Astaneh-Asl, 2008; Agrawal et al., 2010; Lounis and McAllister, 2016). To enhance the Rd of a system, it is important to establish comprehensive plans that ensure an abundance of disaster resources. These plans should include sufficient funding and necessary materials to mitigate future disasters effectively (Huang et al., 2021).
Inclusivity (Ic) involves consulting and engaging communities to address shocks and stresses in specific communities, sectors, or locations. Ic improves and feeds future decision-making processes through proper stakeholder feedback (Salimi and Al-Ghamdi, 2020; Sharifi, 2016). By actively involving various stakeholders, shared ownership actions promote efficient information exchange and integration within the city’s systems (Salimi and Al-Ghamdi, 2020). Community engagement in discussions about the threats to the built environment fosters collective actions, improving resilience and preventing community isolation (Arup and The Rockefeller Foundation, 2014a). Methods such as questionnaire surveys, focus group discussions, interviews with key informants, and community conversations facilitate community participation (Sharifi, 2016; US IOTWS, 2007b; Pfefferbaum et al., 2013; UNDP Drylands Development Centre, 2016; Schwind, 2020). According to the (UNDP Drylands Development Centre et al., 2014), community participants would help develop resilience tools, systems, or indicators by reaching a consensus. Surveys and stakeholders’ participation in the discussions help assess community resilience and identify improvement strategies (Sharifi, 2016; Pfefferbaum et al., 2013). Ic of people and places is supported by a place-based approach (Owens, 2005), which encourages understanding of the way of acting and behaving through collective culture and reflective past (Shafiei Dastjerdi et al., 2021).
Integrating processes across different city systems enhances decision-making reliability. This integration involves networking the systems’ information to facilitate mutual operation and rapid response (Salimi and Al-Ghamdi, 2020). The information exchange among city systems enables collective functioning and swift response to threats and disasters (Arup and The Rockefeller Foundation, 2014a). For instance, integrating natural systems with the constructed form will improve ecological resilience and reduce the occurrence of such disasters (Shafiei Dastjerdi et al., 2021; Chelleri and Marta, 2012). The alignment and integration of systems across various operational scales are crucial for maintaining consistency in decision-making and supporting positive outcomes. This involves sharing information among systems to enable them to function collectively and respond quickly to threats or disasters within a city (Arup and The Rockefeller Foundation, 2014b).
Similarly, in spatial resilience, it is critical to integrate all components of a special network and places through adaptive capacity in a multi-scale hierarchy to ensure consistency and functionality (Shafiei Dastjerdi et al., 2021). Collaboration between relevant authorities and stakeholders in adopting design approaches and integrated planning can reduce the associated implications and result in a holistic model (Morphet, 2010). Also, policies, including the functioning and nature of places, and other procedures and programs must be integrated for spatial resilience. Spatial resilience relies on the adoption of guidelines specific to the place, encompassing both bottom-up integrated and top-down institutional frameworks (Shafiei Dastjerdi et al., 2021). So, in this context, the community’s social role and the importance of integrated physical settings have been emphasized by Lynch’s theory (Lynch, 1960). Table 1 outlines the resilience qualities and characteristics covered in this study.
It is essential to integrate pertinent strategies for better system preparedness and response (Rus et al., 2018), which can be achieved through collaborative efforts of stakeholders (Samsuddin et al., 2018; Schipper and Pelling, 2006; Lin et al., 2008; Malik et al., 2019; AlQahtany and Abubakar, 2020) and by ensuring the integration of RQs into the systems (Samsuddin et al., 2018). This paper intends to quantify four different resilience qualities for the built environment’s ability to withstand climate change risks and stresses. The quantification follows procedures used in previous research by Al-Humaiqani and Al-Ghamdi for the other four resilience qualities assessed (Al-Humaiqani and Al-Ghamdi, 2023e). This study involves the second part of the survey that collects the target audience’s perception. The objective of this paper is driven by the increasing climate change threats and their direct and indirect impacts on the BESs (Schipper and Pelling, 2006; IFRC, 2003; Li et al., 2012; Sato, 2017; Alec Tzannes, 2019; Andrić et al., 2019; Halder and Bandyopadhyay, 2021; Al-Humaiqani and Al-Ghamdi, 2022; Auffhammer, 2022; Bamdad et al., 2022; Blekking et al., 2022; Hidalgo-Galvez et al., 2022; Hürlimann et al., 2022; Larsson Ivanov et al., 2022; Mayo and Lin, 2022; Wang et al., 2022; Wollschlaeger et al., 2022; Al-Humaiqani and Al-Ghamdi, 2023f; Tahir and Al-Ghamdi, 2023). Hence, understanding the built environment resilience can underscore the importance of enhancing relevant policies and strategies (Al-Humaiqani and Al-Ghamdi, 2022; Alyami et al., 2023). The different objectives include 1) the determination of stakeholders’ perceptions about the built environment resilience; 2) the investigation of the interrelations among different resilience indicators, characteristics, and measures; and 3) the determination of the importance of the BESs’ RQs.
The first objective is to assess the quality of responses and evaluate the audience’s understanding of climate change resilience. The second objective is to investigate the interrelationships and interconnections between resilience indicators. The third objective is to assess the importance level of these resilience indicators. The rationale behind these objectives is the challenges posed by climate change and the need to adapt. Numerous studies have highlighted the importance of improving resilience in the design, construction, and operation of BESs (Li et al., 2012; Sato, 2017; Alec Tzannes, 2019; Halder and Bandyopadhyay, 2021; Al-Humaiqani and Al-Ghamdi, 2022; Auffhammer, 2022; Bamdad et al., 2022; Hürlimann et al., 2022; Larsson Ivanov et al., 2022; Wollschlaeger et al., 2022; Mayo and Lin, 2022; Andrić et al., 2019; Blekking et al., 2022; Hidalgo-Galvez et al., 2022; Wang et al., 2022; Forzieri et al., 2018; Khavarian-Garmsir et al., 2019; Gallego-Schmid et al., 2020; Mallen et al., 2020; Saranko et al., 2020; Carter et al., 2021; Koc and Acar, 2021; Barnes et al., 2022; Caby et al., 2022; Gür, 2022; Hiruta et al., 2022; Manes et al., 2022; Mattauch et al., 2022; Murshed et al., 2022; Sovacool et al., 2023). To achieve these objectives, insights from urban planners, policymakers, developers, professionals, and experts on resilience indicators are required.
A questionnaire on RQs of BESs was distributed to participants from regulatory and governmental authorities, the construction industry, and academic institutes. The numerical, structured, and closed-ended questionnaire ensured the objectivity and standardization of the engagement (Cohen et al., 2018). This survey covered the four RQs: robustness (Rb), redundancy (Rd), inclusivity (Ic), and integration (It). These four RQs are visually represented in Figure 1. The remaining four RQs, depicted in grey hatch marks, were independently examined. These eight RQs form the basis of the proposed holistic RQs framework. The graphic depicting these RQs is used consistently in all publications resulting from this research, aiming to maintain connection and inform readers about the covered RQs. Figure 2 provides an explanation of the questionnaire survey distribution procedures.
In Figure 3, stakeholders were categorized into three groups based on their professional and task scope The first group consists of participants from governmental authorities and regulatory bodies. The second one includes professionals from the construction industry, while group 3 focuses on academic institutions and non-governmental organizations. The first group consists of regulatory bodies and government-owned businesses. To start, the participants’ working fields, experiences, occupations, roles, capacity-building backgrounds, and current sectors of work were determined. The survey questionnaire consists of three parts, starting with a briefing of the audience about its scope and objectives. It also declares the no-risk engagement and confidentiality of information. The survey continued with questions related to demographics and the consent to accept or decline participation in the study. The demographic questions covered education level, occupation, experience, and the sector of work, which determined the appropriate group for each participant. The third part covers four main topics: 1) Building Robustness (Rb), 2) Promoting Redundancy (Rd), 3) Broadening Inclusivity (Ic), and 4) Consolidating Integration (It). Table 2 summarizes the content and structure of the questionnaire, while Table 3 outlines the boundaries, structure of the questions, audience, subject, and Indicators. Also, it outlines the occupations and roles of the participants. Table 4 presents the respondents’ groups and statistical tests applied to the questions. Each group was allocated approximately fifty questions to address the discussed objectives in section 1.1.
Table 3. Indicators, structure, subject, and the occupations and roles of participants in question formulation.
Determining the populations of the three stakeholder groups was challenging due to the limitations of the target audience. Nevertheless, the calculations show that the population ranges between 350 and one thousand. The sample size was determined accordingly based on various scientific references, including the guidelines recommended by Cohen et al. (2018). These guidelines primarily suggest using online calculators such as those provided by Raosoft (2022), MaCorr (Research, 2022), Creative Research Systems (2022), Qualtrics (2022), and the SurveyMonkey website (SurveyMonkey, 2022). The chosen level of confidence is 95%, with a margin of error of 5%. Considering the limitations of each group, the total sample size for the three groups ranges between 169 and 278.
The participants were chosen using the probability (random sampling) method, taking into account their audience profiles, background knowledge, and experiences. Group one consists of respondents from institutions and organizations owned by the government and regulatory agencies. Group two comprises professionals in the construction sector, such as developers, consultants, design firms, contractors, project managers, and real estate professionals. On the other hand, group three consists of respondents who work for NGOs, NPOs, academic institutions, and universities. The entities were contacted directly and informed of the study’s scope and goals. They were asked to disseminate the survey among possible respondents from engineering departments in their organizations. Apart from that, the researchers contacted certain regulatory agencies and governmental authorities through phone and email and visited some others in person. In addition, the researchers made local calls to several engineering and construction firms. In addition, the participation of many NGOs, NPOs, academic institutions, and universities was requested in several ways.
The survey was conducted using the SurveyMonkey platform and distributed electronically to the audience. An introductory email and approval letter from the institutional review board (IRB) at Qatar Biomedical Research Institute (QBRI) were sent to the audience. This included a diverse group of approximately two thousand individuals from various engineering backgrounds, including decision-makers, regulators, researchers, academics, experts, and professionals. A turnover of 240+ responses was accomplished with 188 completed responses. The demographic information was discussed in section 3, including occupation, educational background, geographical exposure of expertise, roles, experience, and the entity they represent. Supplementary Figure S1 outlines the research methodology and survey distribution process applied in this study. The survey consisted of general questions for all participants and specific questions for three groups based on their affiliations: Group 1 (questions 13–69), Group 2 (questions 70–126), and Group 3 (questions 127–183). Additional instructions were provided in questions 11 and 12 to guide participants in answering the survey.
The survey questions were extracted from the detailed review conducted on the up-to-date studies on the built environment’s Rb, Rd, Ic, and It. The first draft was shared with three professionals from different groups with deep experience in urban resilience, sustainability, environmental sciences, and engineering. Specific questions against every six indicators of each resilience quality were prepared for each group separately, as shown in Table 3. The aim was to obtain responses that accurately represent the understanding and knowledge of each group. The selected indicators under each quality remain the same for all the groups, maintaining the reliability and consistency of comparisons. After a thorough review, the professionals agreed on the proposed questions, making minor adjustments based on their comments before distributing the survey to the intended audience. Survey approval was obtained from the Institutional Review Board (IRB) at Hamada Bin Khalifa University’s Qatar Biomedical Research Institute to ensure ethical standards and the protection of respondents’ privacy. The approval letter confirmed compliance with ethical guidelines and assured the confidentiality of respondents’ information and involvement.
The survey was conducted using the SurveyMonkey platform for 4 months to gather data from the audience. Various statistical techniques were employed to assess the validity and reliability of the obtained results. Pearson’s chi-squared (X2) and Students’ T-tests were used to understand the complexity and correlations among the measured indicators. Additionally, the reliability and consistency of the measures, including the Likert scales, were evaluated through Cronbach’s Alpha (α) test.
Various ranking scales were chosen based on the type of question and assessed indicator. Level 1 represents the most important resilience quality in all cases, and five indicates the lowest. The importance of the assessed quality or indicator was evaluated through the relative importance index (RII) method, with rankings ranging from 1 to 5 as per Eq. (1).
Where Wi represents the weight assigned to each variable by the respondent, A is the highest weight, and N’ is the total number of respondents.
A Pearson’s Chi-squared (X2) test was used to assess the influence of group type on evaluating the Rb extent of the BES in withstanding the climate change impacts and minimizing the losses caused by hazards.
The study utilized the procedures outlined by Al-Humaiqani and Al-Ghamdi (2023e) and Ngin et al. (2020) to assess participants’ perceptions of specific resilience characteristics and indicators of the built environment concerning climate change. The survey examined the impact of climate change on city systems, exploring coping and adaptation strategies to boost resilience against potential damage. Questions aimed to gather respondents’ views on enhancing the resilience characteristics of BESs against climate change. The participation definitions and categories are illustrated in Figure 3 and Supplementary Figure S2, while the assessment of the BESs’ robustness is based on six main indicators, detailed in Table 5. Respondents’ perceptions of these systems’ capacity to withstand climate-related disturbances and minimize their effects were collected to assess system performance.
Table 5. Summary of the typical Rb, Rd, Ic, and It resilience qualities/indicators covered in this research.
Feedback was gathered on the role of urban governance in developing the ability to adapt to uncertainty and change, particularly in BESs. Opinions were also obtained on conducting assessments to enhance the adaptive capacity of BESs and community resilience tools. Additionally, participants provided input on integrating processes within city systems to promote consistent decision-making and investments.
The collection of survey responses took place during the period from September to December 2022. The majority of responses came from construction professionals (group 2), comprising 55% of the total, while groups 1 and 3 accounted for 20% and 25%, respectively, as illustrated in Supplementary Figure S3.
The analysis presented in Figure 4A highlights the significance of urban governance in building resilience to uncertainty and change, especially in Built Environment Systems (BESs). The findings indicate a collective inclination towards considering urban governance moderately to highly important. Group 1 displayed a tendency towards moderate importance, while groups 2 and 3 leaned more towards deeming it highly important. This analysis suggests a shared agreement among respondents across all three groups on the need for urban governance to strengthen its transformative capabilities in addressing uncertainty and change within BESs. Moreover, Figure 4A depicts a strong consensus among most respondents regarding the importance of regulations that mandate emergency resources and supplies for BESs, particularly those situated in vulnerable areas.
Figure 4. Groups’ responses to (A) leveling the importance of the need for urban governance and providing regulations stating mandatory emergency resources and supplies, (B) conducting the necessary assessment to improve the adaptive capacity of BESs, improve the resilience of community tools by the government authorities, and processes integration within the city’s systems.
The analysis indicates that group 1 finds the assessment of enhancing the adaptive capacities of BESs and related tools inadequate, with responses mostly falling between fair and average. Similarly, over 60% of Group 2 members feel that efforts to enhance adaptive capacities are insufficient. However, less than 30% of all respondents find the actions taken satisfactory.
In contrast, in group 3, 30% consider the actions by relevant authorities to be poor, while another 30% rate them as fair to average. Conversely, 25% view the actions as good, as depicted in Figure 4B. Respondents also shared their opinions on integrating processes among the city’s systems to ensure consistency in decision-making and investments. In group 1, 65% believe the integration level is average, with the rest strongly agreeing. Group 2 presents a different trend, with around 50% disagreeing with this notion. Group 3’s perspective aligns more with Group 2’s, but no participant strongly agrees on integrating the processes of existing BESs.
This section discusses response rates from various groups on their comprehension of the importance of enhancing resilience against the impacts of climate change. Figure 5 illustrates the extent of the Rb of the BESs in line with notes by Ouyang (2017) that emphasize the BESs’ resilience in enduring and recovering from disasters, referencing the resilience of a BES to its robustness in withstanding and recovering from a disaster. The results did not provide enough evidence to reject the null hypothesis that there are no differences in grouping and expertise types (p-value of 0.995 at 5% significance level). Most respondents in Group 1 and Group 2 perceive the Rb extent of the BESs to climate change impacts and hazard-induced losses as high. In contrast, Group 3 respondents see this Rb extent at a moderate level, suggesting potential for enhancing building standards, particularly from a research and academic standpoint. According to Kammouh et al. (2020), a system is deemed resilient when it demonstrates high robustness, low failure likelihood, and rapid recovery capabilities. Figure 6 present evaluations of the BESs’ ability to mitigate climate-related disruptions and minimize associated consequences, echoing (Bruneau et al., 2003) view that Rb is the system’s strength to withstand disruption and measure absorptive capability to reduce the negative impacts and consequences.
Figure 6. Evaluations of the (A) BESs’ ability to mitigate climate-related disruptions and minimize associated consequences and rating the capacity and (B) ranking the level of losses over time.
The robustness of a project is attributed to its early phase preparation, indicating the urban ecosystem’s capacity to maintain essential functions (Tong, 2021). In group 1, most respondents rated the capacities of the four BESs between good and excellent, averaging at about 50%. For group 2, the majority perceived the systems’ capacities as generally good, with approximately 55% rating them between average and excellent. This confirms that protective infrastructure targeting climate hazards lacks resilience and diversity, the research by Birchall and Bonnett (2021) indicates. Group 3 showed different perceptions, with their views falling within a similar range for different systems. The analysis suggests that group 2’s confidence level is high, likely influenced by their experience and skills in system construction. Conversely, the perspectives of groups 1 and 3 are notably influenced by each group’s role. Table 6 displays the results of the relative importance index analysis, ranking the BESs’ capacity to mitigate climate-related disturbances and their consequences. Group 1 ranked the four BESs equally, while groups 2 and 3 had different rankings. Group 2 prioritized life support systems, whereas Group 3 considered the remaining two systems more crucial. The results showed a consensus with the findings of the study by Salimi and Al-Ghamdi (2020), which proposes that strategic approaches to building resilient infrastructure should prioritize robustness, redundancy, and resourcefulness. The statistical analysis did not yield enough evidence to reject the null hypothesis of grouping and professionalism differences, with p-values ranging between 0.875 and 0.999 at a 5% significance level.
Table 6. Evaluating the capacity of the BESs to buffer climatic disturbances and reduce or prevent the resulting consequences (Relative importance index).
Redundancy (Rd) is fundamental in design philosophies, especially structural designs (Al-Humaiqani and Al-Ghamdi, 2022). It refers to the ability of systems and facilities to sustain functions (Samsuddin et al., 2018) and integrity in the face of disruptions (Faturechi and Miller-Hooks, 2015). The participants shared their opinions on whether authorities and organizations can prepare for expected or unexpected climate change disasters. Figure 7 indicates that 56% of respondents agree with the notion, and 44% expressed doubts about the progress in promoting good practices in the built environment. This observation may be attributed to the lack of progress due to limited community involvement in proactive measures, which authorities and regulatory bodies should address. On the other hand, Table 7 summarizes the ranking of the diversity and backup capacity of the BESs to withstand climate hazards. The results show that life support systems and open spaces are ranked highest, followed by movement and shelter systems.
Figure 7. The progress in promoting good practice in the built environment at the community level and advancing future measures to reduce the impacts of climate change.
This study determined the relationship between the diversity and backup capacity of BESs in relation to their ability to withstand climate change hazards for different built environment systems (Supplementary Figure S4). The Rb and Rp of an urban system is influenced by its Rd, with Rb often equated to strong Rd according to Kanno and Ben-Haim (2011). Based on specific questions, the analysis presented in Figure 8 identified correlations within BESs, underscoring the importance of Rd in enhancing their resilience. Participants also shared views on promoting good practices in the built environment at the community level and future strategies to mitigate the climate change impacts. Figure 9 illustrates diverse opinions among the groups, with 45% in agreement, 15% disagreement, and approximately 40% unsure about the advancement of good practices. This highlights the critical notion that inadequate Rd may result in premature system deterioration and subsequent collapse, as evidenced by Astaneh-Asl (2008); Agrawal et al. (2010); Lounis and McAllister (2016).
Figure 8. Evaluations of (A) correlation of the diversity and backup capacity of the BESs to withstand climate change hazards and (B) progress in promoting good practice in the built environment at the community level and advancing future measures to reduce the impact of climate change disturbances.
Figure 9. The progress in promoting good practice in the built environment at the community level and advancing future measures to reduce the impact of climate change disturbances.
Broad consultation and community engagement are essential for establishing a shared sense of ownership. As per the Rockefeller Foundation and Arup, community engagement encompasses all groups, including vulnerable populations, collaboratively addressing the challenges faced by various sectors and working to reduce isolation between communities. This inclusive approach promotes a cohesive vision and joint accountability in building a resilient city (Arup and The Rockefeller Foundation, 2014b). It is widely acknowledged that an inclusive strategy ensures a unified vision and collective responsibility for urban resilience, emphasizing the importance of valuable stakeholder input in shaping a decision-making process that is inclusive (Sharifi, 2016).
This section analyzes the relationship between Ic’s indicators and their correlations, which include community engagement, shared ownership action, informing future decision-making, and enhancing resilience. Achieving an inclusive decision-making process involves obtaining feedback from relevant stakeholders (Salimi and Al-Ghamdi, 2020; Sharifi, 2016). The study assessed participants’ confidence in contributing to resilience development by achieving consensus as a united community. It also evaluated agreement levels on improving urban resilience and reducing community isolation through public engagement in collecting data on climate change disasters through surveys and interviews. The results for both perceptions are displayed in Figure 10, highlighting the importance of various stakeholders participating in shared ownership actions to facilitate information exchange and promote efficient integration among different components and systems as suggested by Salimi and Al-Ghamdi (2020).
Figure 10. The public crucial role in developing resilience systems, tools, and indicators, as well as improving urban resilience and reducing community isolation through public engagement.
To evaluate community resilience effectively, it is essential to identify priorities, utilize assessment tools, and create action plans through participatory approaches and mapping techniques (Al-Humaiqani and Al-Ghamdi, 2022). Research surveys and stakeholder involvement are crucial for assessing the enhancement of community resilience performance over time (Sharifi, 2016; Pfefferbaum et al., 2013). The analysis explored the importance of involving the community in discussions to establish mutual ownership of BESs resilience, as depicted in Figure 11. It also assessed the consensus on adopting an inclusive approach to promote shared responsibility and a common vision for urban resilience.
Figure 11. The community required inclusive consultation and engagement to establish a collective sense of ownership and agreement. This approach to inclusion ensures a unified vision and shared responsibility for developing urban resilience.
Incorporating integration (It) processes into the BESs would ensure consistency in decision-making and investment placements and ensure they function together and respond quickly (Salimi and Al-Ghamdi, 2020; Al-Humaiqani and Al-Ghamdi, 2022). According to Shafiei Dastjerdi et al., preserving the integrity and functions of places and spatial networks relies on integrating all elements through adaptive capacity within a multi-scale hierarchy (Shafiei Dastjerdi et al., 2021). The survey investigates the impact of integrating city systems’ processes on decision-making and investment placement. Results indicate that 45% of Group 1 respondents sometimes believe in this integration. However, all three groups generally agree on its effectiveness, with over 45% consensus, as shown in Figure 12. The groups also prioritize different indicators, such as the adaptability of BESs to long-term changes that lead to uncertainty, illustrated in Figure 13. This figure outlines the assessments required to enhance the adaptive capacity of BESs and improve community resilience tools. Additionally, the text stresses the importance of proactive urban adaptive strategies over reactive plans and integrating disaster resilience into urban planning.
Figure 12. Responses on the integrating processes within the BESs to ensure consistency in promoting decision-making and making investments.
Figure 13. Groups’ ranking of the significance of several indicators, including the BESs’ ability to adapt to long-term changes that contribute to uncertainty, conducting the required assessment to improve the adaptive capacity of the BESs and improve the tools of community resilience assessment and level of importance of implementing proactive urban adaptive strategies instead of reactive plans and integrating disaster resilience into urban planning.
This study examines the resilience qualities of BESs, focusing on Rb, Rd, Ic, and It, along with their corresponding indicators. The research is based on a survey that gathered input from three main stakeholder groups. Responses were analyzed rigorously to assess the four resilience qualities and their relationships. Stakeholders, such as regulatory bodies, government entities, contractors, experts, academia, and NGOs, participated in the survey to assess the RQs of BESs, associated measures, and indicators. The survey explores how current built environment resilience indicators are interconnected and their perceived effectiveness in addressing climate change impacts.
The study investigates the significance of urban governance in enforcing regulations for emergency resources and supplies. The results show that respondents generally view these regulations as moderately to very important. There is a consensus across all respondent groups that urban governance needs to be able to adapt to uncertainty and change in urban environments. The study also examines the integration of processes within systems to ensure coherent decision-making and investments. The statistical analysis, however, did not provide enough evidence to reject the null hypothesis. There is a strong belief in the importance of BESs being resilient to climate change and reducing hazards. Despite this, there are opportunities to improve the resilience of BESs by enhancing standards to mitigate disturbances and their consequences. More than 40% of respondents noted a lack of progress in promoting best practices in the built environment, possibly due to limited community engagement. The study suggests that relevant authorities and regulatory bodies should address this issue. The study found that life support systems and open spaces were rated as the most important, followed by movement and shelter systems.
The study focused on participants’ readiness to contribute to developing resilience systems, indicators, or tools through community consensus. Results showed agreement on the importance of involving diverse community input to cultivate shared ownership in enhancing resilience to climate change. The study underscores the significance of gathering comprehensive stakeholder feedback to facilitate an inclusive decision-making process, ensuring a cohesive vision and collective responsibility for urban resilience. The integration analysis evaluated if city processes support consistent decision-making and investment allocation, with a general consensus that they do. The study also highlights the necessity of evaluating and strengthening the adaptive capacity of BESs and improving community resilience assessment tools. Furthermore, it suggests that proactive urban adaptive strategies are more crucial than reactive plans and emphasizes the importance of integrating disaster resilience into urban planning.
The original contributions presented in the study are included in the article/Supplementary Material, further inquiries can be directed to the corresponding author.
The studies involving humans were approved by Institutional Review Board (IRB) at Qatar Biomedical Research Institute (QBRI) at Qatar. The studies were conducted in accordance with the local legislation and institutional requirements. The participants provided their written informed consent to participate in this study.
MA-H: Writing–original draft, Visualization, Methodology, Formal Analysis, Data curation, Conceptualization. SA-G: Writing–review and editing, Supervision, Resources, Project administration, Investigation, Funding acquisition, Conceptualization.
The author(s) declare financial support was received for the research, authorship, and/or publication of this article. This publication was made possible by NPRP-Cluster (NPRP-C) Fourteenth (14th) Cycle grant NPRP14C-0909-210008 from the Qatar National Research Fund (a member of Qatar Foundation). The findings herein reflect the work and are solely the responsibility of the authors. Any opinions, findings, conclusions, or recommendations expressed in this material are those of the author(s) and do not necessarily reflect the views of QNRF or QF.
This research was supported by a scholarship from Hamad Bin Khalifa University (HBKU) a member of the Qatar Foundation (QF). Any opinions, findings, conclusions, or recommendations expressed in this material are those of the author(s) and do not necessarily reflect the views of HBKU or QF.
The authors declare that the research was conducted in the absence of any commercial or financial relationships that could be construed as a potential conflict of interest.
All claims expressed in this article are solely those of the authors and do not necessarily represent those of their affiliated organizations, or those of the publisher, the editors and the reviewers. Any product that may be evaluated in this article, or claim that may be made by its manufacturer, is not guaranteed or endorsed by the publisher.
The Supplementary Material for this article can be found online at: https://www.frontiersin.org/articles/10.3389/fbuil.2024.1343334/full#supplementary-material
Agrawal, A. K., Kawaguchi, A., and Chen, Z. (2010). Deterioration rates of typical bridge elements in New York. J. Bridg. Eng. 15 (4), 419–429. doi:10.1061/(ASCE)BE.1943-5592.0000123
Alec Tzannes, A. M. (2019). Matthaios santamouris: minimising energy consumption, energy poverty and global and local climate change in the built environment: innovating to zero: casualties and impacts in a zero concept world. J. Wind Eng. Ind. Aerodyn. 193, 103952. doi:10.1016/j.jweia.2019.103952
Al Humaiqani, M. M., and Al Ghamdi, S. G. (2023a). “Unveiling urban resilience: exploring the qualities and interconnections of urban systems,” in Sustainable cities in a changing climate (Wiley), 139–154. doi:10.1002/9781394201532.ch10
Al Humaiqani, M. M., and Al Ghamdi, S. G. (2023b). “Quantifying urban resilience: methods and approaches for comprehensive assessment,” in Sustainable cities in a changing climate (Wiley), 155–182. doi:10.1002/9781394201532.ch11
Al Humaiqani, M. M., and Al Ghamdi, S. G. (2023c). “Enhancing built environment resilience: exploring themes and dimensions,” in Sustainable cities in a changing climate (Wiley), 121–137. doi:10.1002/9781394201532.ch9
Al-Humaiqani, M. M., and Al-Ghamdi, S. G. (2023d). Exploring the significance of resilience qualities in the context of the Middle East built environment, 319–330. doi:10.1007/978-981-99-4101-8_25
Al-Humaiqani, M. M., and Al-Ghamdi, S. G. (2023e). Assessing the built environment’s reflectivity, flexibility, resourcefulness, and rapidity resilience qualities against climate change impacts from the perspective of different stakeholders. Sustainability 15 (6), 5055. doi:10.3390/su15065055
Al Humaiqani, M. M., and Al Ghamdi, S. G. (2023f). “Integrating green–blue–gray infrastructure for sustainable urban flood risk management: enhancing resilience and advantages,” in Sustainable cities in a changing climate (Wiley), 207–226. doi:10.1002/9781394201532.ch13
Al-Humaiqani, M. M., and Al-Ghamdi, S. G. (2022). The built environment resilience qualities to climate change impact: concepts, frameworks, and directions for future research. Sustain. Cities Soc. 80, 103797. doi:10.1016/j.scs.2022.103797
AlQahtany, A. M., and Abubakar, I. R. (2020). Public perception and attitudes to disaster risks in a coastal metropolis of Saudi Arabia. Int. J. Disaster Risk Reduct. 44, 101422. doi:10.1016/j.ijdrr.2019.101422
Alyami, S. H., Abd El Aal, A., Alqahtany, A., Aldossary, N., Jamil, R., Almohassen, A., et al. (2023). Developing a holistic resilience framework for critical infrastructure networks of buildings and communities in Saudi arabia. Buildings 13 (1), 179. doi:10.3390/buildings13010179
Andrić, I., Koc, M., and Al-Ghamdi, S. G. (2019). A review of climate change implications for built environment: impacts, mitigation measures and associated challenges in developed and developing countries. J. Clean. Prod. 211, 83–102. doi:10.1016/j.jclepro.2018.11.128
Arup and The Rockefeller Foundation (2014a). City Resilience Index: City Resilience Framework. London: Arup.
Arup and The Rockefeller Foundation (2014b). City Resilience Index: Understanding the City Resilience Index. London: Arup, 24. Available at: https://www.arup.com/projects/city-resilience-index.
Asfaw, T. S., Botes, V., and Mengesha, L. G. (2017). The role of NGOs in corporate environmental responsibility practice: evidence from Ethiopia. Int. J. Corp. Soc. Responsib. 2 (1), 2. doi:10.1186/s40991-017-0013-0
Astaneh-Asl, A. (2008). Progressive collapse of steel truss bridges, the case of i-35w collapse. Seattle, United States: Semantic Scholar. Available at: https://www.semanticscholar.org/paper/PROGRESSIVE-COLLAPSE-OF-STEEL-TRUSS-BRIDGES%2C-THE-OF-Astaneh-Asl/1ba709e2b26b36ab709b89b1cb58db964794660d (Accessed February 28, 2022).
Auffhammer, M. (2022). Climate Adaptive Response Estimation: short and long run impacts of climate change on residential electricity and natural gas consumption. J. Environ. Econ. Manage. 114, 102669. doi:10.1016/j.jeem.2022.102669
Ayyub, B. M. (2020). Infrastructure resilience and sustainability: definitions and relationships. ASCE-ASME J. Risk Uncertain. Eng. Syst. Part A Civ. Eng. 6 (3), 02520001. doi:10.1061/AJRUA6.0001067
Bamdad, K., Matour, S., Izadyar, N., and Omrani, S. (2022). Impact of climate change on energy saving potentials of natural ventilation and ceiling fans in mixed-mode buildings. Build. Environ. 209, 108662. doi:10.1016/j.buildenv.2021.108662
Barnes, M. L., Datta, A., Morris, S., and Zethoven, I. (2022). Navigating climate crises in the great barrier reef. Glob. Environ. Chang. 74, 102494. doi:10.1016/j.gloenvcha.2022.102494
Bazrkar, M. H., Zamani, N., Eslamian, S., Eslamian, A., and Dehghan, Z. (2015). “Urbanization and climate change,” in Handbook of climate change adaptation (Berlin, Heidelberg: Springer Berlin Heidelberg), 619–655. doi:10.1007/978-3-642-38670-1_90
Birchall, S. J., and Bonnett, N. (2021). Climate change adaptation policy and practice: the role of agents, institutions and systems. Cities 108, 103001. doi:10.1016/J.CITIES.2020.103001
Blekking, J., Giroux, S., Waldman, K., Battersby, J., Tuholske, C., Robeson, S. M., et al. (2022). The impacts of climate change and urbanization on food retailers in urban sub-Saharan Africa. Curr. Opin. Environ. Sustain. 55, 101169. doi:10.1016/j.cosust.2022.101169
Bocchini, P., Frangopol, D. M., Ummenhofer, T., and Zinke, T. (2014). Resilience and sustainability of Civil infrastructure: toward a unified approach. J. Infrastruct. Syst. 20 (2), 04014004. doi:10.1061/(ASCE)IS.1943-555X.0000177
Bruneau, M., Chang, S. E., Eguchi, R. T., Lee, G. C., O'Rourke, T. D., Reinhorn, A. M., et al. (2003). A framework to quantitatively assess and enhance the seismic resilience of communities. Earthq. Spectra 19 (4), 733–752. doi:10.1193/1.1623497
Caby, J., Ziane, Y., and Lamarque, E. (2022). The impact of climate change management on banks profitability. J. Bus. Res. 142, 412–422. doi:10.1016/j.jbusres.2021.12.078
Carter, T. R., Benzie, M., Campiglio, E., Carlsen, H., Fronzek, S., Hildén, M., et al. (2021). A conceptual framework for cross-border impacts of climate change. Glob. Environ. Chang. 69, 102307. doi:10.1016/j.gloenvcha.2021.102307
Cohen, L., Manion, L., and Morrison, K. (2018). Research methods in education, no. 8. New York, NY, USA: Routledge.
Creative Research Systems (2022). Sample size calculator. Available at: https://www.surveysystem.com/sscalc.htm (Accessed July 18, 2022).
da Silva, J., Kernaghan, S., and Luque, A. (2012). A systems approach to meeting the challenges of urban climate change. Int. J. Urban Sustain. Dev. 4 (2), 125–145. doi:10.1080/19463138.2012.718279
Deshkar, S., and Adane, V. (2016). Community resilience approach for prioritizing infrastructure development in urban areas. Elsevier Inc. doi:10.1016/B978-0-12-802169-9.00016-1
Faturechi, R., and Miller-Hooks, E. (2015). Measuring the performance of transportation infrastructure systems in disasters: a comprehensive review. J. Infrastruct. Syst. 21 (1), 1–15. doi:10.1061/(ASCE)IS.1943-555X.0000212
Forzieri, G., Bianchi, A., Silva, F. B. e., Marin Herrera, M. A., Leblois, A., Lavalle, C., et al. (2018). Escalating impacts of climate extremes on critical infrastructures in Europe. Glob. Environ. Chang. 48, 97–107. doi:10.1016/j.gloenvcha.2017.11.007
Gallego-Schmid, A., Chen, H.-M., Sharmina, M., and Mendoza, J. M. F. (2020). Links between circular economy and climate change mitigation in the built environment. J. Clean. Prod. 260, 121115. doi:10.1016/j.jclepro.2020.121115
Godschalk, D. R. (2003). Urban hazard mitigation: creating resilient cities. Nat. Hazards Rev. 4 (3), 136–143. doi:10.1061/(ASCE)1527-6988(2003)4:3(136)
Gür, H. (2022). The future impact of climate and land-use changes on Anatolian ground squirrels under different scenarios. Ecol. Inf. 70, 101693. doi:10.1016/j.ecoinf.2022.101693
Halder, B., and Bandyopadhyay, J. (2021). Evaluating the impact of climate change on urban environment using geospatial technologies in the planning area of Bilaspur, India. Environ. Challenges 5, 100286. doi:10.1016/j.envc.2021.100286
Hidalgo-Galvez, M. D., Barkaoui, K., Volaire, F., Matías, L., Cambrollé, J., Fernández-Rebollo, P., et al. (2022). Can trees buffer the impact of climate change on pasture production and digestibility of Mediterranean dehesas? Sci. Total Environ. 835, 155535. doi:10.1016/j.scitotenv.2022.155535
Hiruta, Y., Ishizaki, N. N., Ashina, S., and Takahashi, K. (2022). Regional and temporal variations in the impacts of future climate change on Japanese electricity demand: simultaneous interactions among multiple factors considered. Energy Convers. Manag. X 14, 100172. doi:10.1016/j.ecmx.2021.100172
Huang, G., Li, D., Zhu, X., and Zhu, J. (2021). Influencing factors and their influencing mechanisms on urban resilience in China. Sustain. Cities Soc. 74, 103210–103211. doi:10.1016/J.SCS.2021.103210
Hürlimann, A. C., Nielsen, J., Moosavi, S., Bush, J., Warren-Myers, G., and March, A. (2022). Climate change preparedness across sectors of the built environment – a review of literature. Environ. Sci. Policy 128, 277–289. doi:10.1016/j.envsci.2021.11.021
IFRC (2003). “PREPAREDNESS for climate change: a study to assess the future impact of climatic changes upon the frequency and severity of disasters and the implications for humanitarian response and preparedness,” in 28th International Conference of the Red Cross and Red Crescent, 15.
Kammouh, O., Gardoni, P., and Cimellaro, G. P. (2020). Probabilistic framework to evaluate the resilience of engineering systems using Bayesian and dynamic Bayesian networks. Reliab. Eng. Syst. Saf. 198, 106813–106820. doi:10.1016/J.RESS.2020.106813
Kanno, Y., and Ben-Haim, Y. (2011). Redundancy and robustness, or when is redundancy redundant? J. Struct. Eng. 137 (9), 935–945. doi:10.1061/(ASCE)ST.1943-541X.0000416
Khavarian-Garmsir, A. R., Pourahmad, A., Hataminejad, H., and Farhoodi, R. (2019). Climate change and environmental degradation and the drivers of migration in the context of shrinking cities: a case study of Khuzestan province, Iran. Sustain. Cities Soc. 47, 101480. doi:10.1016/j.scs.2019.101480
Koc, M., and Acar, A. (2021). Investigation of urban climates and built environment relations by using machine learning. Urban Clim. 37, 100820. doi:10.1016/j.uclim.2021.100820
Lak, A., Hasankhan, F., and Garakani, S. A. (2020). Principles in practice: toward a conceptual framework for resilient urban design. J. Environ. Plan. Manag. 63 (12), 2194–2226. doi:10.1080/09640568.2020.1714561
Larsson Ivanov, O., Bärring, L., and Wilcke, R. A. I. (2022). Climate change impact on snow loads in northern Europe. Struct. Saf. 97, 102231. doi:10.1016/j.strusafe.2022.102231
Li, D. H. W., Yang, L., and Lam, J. C. (2012). Impact of climate change on energy use in the built environment in different climate zones – a review. Energy 42 (1), 103–112. doi:10.1016/j.energy.2012.03.044
Lin, S., Shaw, D., and Ho, M.-C. (2008). Why are flood and landslide victims less willing to take mitigation measures than the public? Nat. Hazards 44 (2), 305–314. doi:10.1007/s11069-007-9136-z
Lounis, Z., and McAllister, T. P. (2016). Risk-based decision making for sustainable and resilient infrastructure systems. J. Struct. Eng. 142 (9). doi:10.1061/(ASCE)ST.1943-541X.0001545
Malik, K., Rahman, S. M., Khondaker, A. N., Abubakar, I. R., Aina, Y. A., and Hasan, M. A. (2019). Renewable energy utilization to promote sustainability in GCC countries: policies, drivers, and barriers. Environ. Sci. Pollut. Res. 26 (20), 20798–20814. doi:10.1007/s11356-019-05337-1
Mallen, E., Bakin, J., Stone, B., Sivakumar, R., and Lanza, K. (2020). Thermal impacts of built and vegetated environments on local microclimates in an Urban University campus. Urban Clim. 32, 100640. doi:10.1016/j.uclim.2020.100640
Manes, S., Vale, M. M., Malecha, A., and Pires, A. P. F. (2022). Nature-based solutions promote climate change adaptation safeguarding ecosystem services. Ecosyst. Serv. 55, 101439. doi:10.1016/j.ecoser.2022.101439
Mattauch, L., Hepburn, C., Spuler, F., and Stern, N. (2022). The economics of climate change with endogenous preferences. Resour. Energy Econ. 69, 101312. doi:10.1016/j.reseneeco.2022.101312
Mayo, T. L., and Lin, N. (2022). Climate change impacts to the coastal flood hazard in the northeastern United States. Weather Clim. Extrem. 36, 100453. doi:10.1016/j.wace.2022.100453
McAllister, T. (2013). Developing guidelines and standards for disaster resilience of the built environment: a research needs assessment. United States: National Institute of Standards and Technology Publications, 1–142.
Meerow, S., Newell, J. P., and Stults, M. (2016). Defining urban resilience: a review. Landsc. Urban Plan. 147, 38–49. doi:10.1016/j.landurbplan.2015.11.011
Moghadas, M., Asadzadeh, A., Vafeidis, A., Fekete, A., and Kötter, T. (2019). A multi-criteria approach for assessing urban flood resilience in Tehran, Iran. Int. J. Disaster Risk Reduct. 35, 101069. doi:10.1016/j.ijdrr.2019.101069
Morphet, J. (2010). Effective practice in spatial planning. 1. London: Routledge, Taylor and Francies Group. doi:10.4324/9780203851838
Murshed, S., Griffin, A. L., Islam, M. A., Wang, X. H., and Paull, D. (2022). Assessing multi-climate-hazard threat in the coastal region of Bangladesh by combining influential environmental and anthropogenic factors. Prog. Disaster Sci. 16, 100261. doi:10.1016/j.pdisas.2022.100261
Ngin, C., Chhom, C., and Neef, A. (2020). Climate change impacts and disaster resilience among micro businesses in the tourism and hospitality sector: the case of Kratie, Cambodia. Environ. Res. 186, 109557. doi:10.1016/j.envres.2020.109557
Nik, V. M., and Moazami, A. (2021). Using collective intelligence to enhance demand flexibility and climate resilience in urban areas. Appl. Energy 281, 116106–116117. doi:10.1016/j.apenergy.2020.116106
Ouyang, M. (2017). A mathematical framework to optimize resilience of interdependent critical infrastructure systems under spatially localized attacks. Eur. J. Oper. Res. 262 (3), 1072–1084. doi:10.1016/j.ejor.2017.04.022
Owens, P. M. (2005). Beyond density: measuring neighborhood form in new england’s upper Connecticut river valley. Berkeley: University of California.
Pfefferbaum, R. L., Pfefferbaum, B., Van Horn, R. L., Klomp, R. W., Norris, F. H., and Reissman, D. B. (2013). The communities advancing resilience toolkit (CART): an intervention to build community resilience to disasters. J. Public Heal. Manag. 19, 250–258. doi:10.1097/phh.0b013e318268aed8
Qualtrics (2022). Sample size calculator and complete guide in 2022. Available at: https://www.qualtrics.com/blog/calculating-sample-size/ (Accessed July 18, 2022).
Raosoft (2022). Sample size calculator by Raosoft, inc. Las Vegas, United States: Raosoft, Inc. Available at: http://www.raosoft.com/samplesize.html (Accessed July 18, 2022).
Research, M. (2022). Sample size calculator for market research surveys. Available at: https://www.macorr.com/sample-size-calculator.htm (Accessed January 15, 2023).
Rose, A. (2004). Defining and measuring economic resilience to disasters. Disaster Prev. Manag. Int. J. 13 (4), 307–314. doi:10.1108/09653560410556528
Rose, A., Oladosu, G., and Liao, S. Y. (2007). Business interruption impacts of a terrorist attack on the electric power system of Los Angeles: customer resilience to a total blackout. Risk Anal. 27 (3), 513–531. doi:10.1111/j.1539-6924.2007.00912.x
Rus, K., Kilar, V., and Koren, D. (2018). Resilience assessment of complex urban systems to natural disasters: a new literature review. Int. J. Disaster Risk Reduct. 31, 311–330. doi:10.1016/j.ijdrr.2018.05.015
Salimi, M., and Al-Ghamdi, S. G. (2020). Climate change impacts on critical urban infrastructure and urban resiliency strategies for the Middle East. Sustain. Cities Soc. 54, 1–17. doi:10.1016/j.scs.2019.101948
Samsuddin, N. M., Takim, R., Nawawi, A. H., and Syed Alwee, S. N. A. (2018). Disaster preparedness attributes and hospital’s resilience in Malaysia. Procedia Eng. 212, 371–378. doi:10.1016/j.proeng.2018.01.048
Saranko, O., Fortelius, C., Jylhä, K., Ruosteenoja, K., Brattich, E., Di Sabatino, S., et al. (2020). Impacts of town characteristics on the changing urban climate in Vantaa. Sci. Total Environ. 727, 138471. doi:10.1016/j.scitotenv.2020.138471
Sato, S. (2017). Climate change, the built environment and triple-helix innovation. Energy Procedia 143, 843–850. doi:10.1016/j.egypro.2017.12.772
Schipper, L., and Pelling, M. (2006). Disaster risk, climate change and international development: scope for, and challenges to, integration. Disasters 30 (1), 19–38. doi:10.1111/j.1467-9523.2006.00304.x
Schwind, K. (2020). Community resilience toolkit A workshop guide for community resilience planning.
Serdar, M. Z., and Al Ghamdi, S. G. (2023). “Assessing infrastructure resilience: approaches and considerations,” in Sustainable cities in a changing climate (Wiley), 97–109. doi:10.1002/9781394201532.ch7
Shafiei Dastjerdi, M., Lak, A., Ghaffari, A., and Sharifi, A. (2021). A conceptual framework for resilient place assessment based on spatial resilience approach: an integrative review. Urban Clim. 36, 100794. doi:10.1016/j.uclim.2021.100794
Sharifi, A. (2016). A critical review of selected tools for assessing community resilience. Ecol. Indic. 69, 629–647. doi:10.1016/j.ecolind.2016.05.023
Sovacool, B. K., Baum, C., and Low, S. (2023). The next climate war? Statecraft, security, and weaponization in the geopolitics of a low-carbon future. Energy Strateg. Rev. 45, 101031. doi:10.1016/j.esr.2022.101031
SurveyMonkey (2022). Sample size calculator: understanding sample sizes. Available at: https://www.surveymonkey.com/mp/sample-size-calculator/?ut_source=help_center&ut_source2=en%2Fsolutions%2Fcalculating-respondents&ut_source3=inline (Accessed July 18, 2022).
Tahir, F., and Al-Ghamdi, S. G. (2023). Climatic change impacts on the energy requirements for the built environment sector. Energy Rep. 9, 670–676. doi:10.1016/j.egyr.2022.11.033
Tahir, F., Ajjur, S. B., Serdar, M. Z., Al-Humaiqani, M., Kim, D., Al-Thani, S. K., et al. (2021). Proceedings of the Qatar Climate Change Conference 2021: a Platform for addressing key climate change topics facing Qatar and the world. 1. Doha: Hamad bin Khalifa University Press HBKU Press. doi:10.5339/conf_proceed_qccc2021
Tong, P. (2021). Characteristics, dimensions and methods of current assessment for urban resilience to climate-related disasters: a systematic review of the literature. Int. J. Disaster Risk Reduct. 60, 102276. doi:10.1016/j.ijdrr.2021.102276
UNDP Drylands Development Centre (2014). “Understanding community resilience: findings from community-based resilience analysis (CoBRA) assessments,” in Under the framework of the European commission directorate general for humanitarian aid and Civil protection’s drought ri. Editor C. M. Way.
UNDP Drylands Development Centre (2016). Community based resilience analysis (cobra) conceptual framework and methodology. Under the framework of humanitarian aid and Civil protection department of the European commission’s drought risk reduction action plan.
US IOTWS (2007a). How Resilient is Your Coastal Community? A guide for evaluating coastal community resilience to tsunamis and other hazards, no. September 2016.
U.S. IOTWS (2007b). How resilient is your coastal community? A guide for evaluating coastal community resilience to tsunamis and other hazards | enhanced reader.
Wang, H., Zhang, Q., Liu, R., Sun, Y., Xiao, J., Gao, L., et al. (2022). Impacts of changing climate on the distribution of Solenopsis invicta Buren in Mainland China: exposed urban population distribution and suitable habitat change. Ecol. Indic. 139, 108944. doi:10.1016/j.ecolind.2022.108944
Wardekker, A., Wilk, B., and Brown, V. (2017). Assessing urban resilience in Rotterdam using resilience principles: workshop report. Utrecht, Netherlands: Utrecht University.
Wollschlaeger, S., Sadhu, A., Ebrahimi, G., and Woo, A. (2022). Investigation of climate change impacts on long-term care facility occupants. City Environ. Interact. 13, 1–15. doi:10.1016/j.cacint.2021.100077
Yazdani, A., and Jeffrey, P. (2012). Applying network theory to quantify the redundancy and structural robustness of water distribution systems. J. Water Resour. Plan. Manag. 138 (2), 153–161. doi:10.1061/(ASCE)WR.1943-5452.0000159
Zobel, C. W. (2011). Representing perceived tradeoffs in defining disaster resilience. Decis. Support Syst. 50 (2), 394–403. doi:10.1016/j.dss.2010.10.001
In a previous study (Al-Humaiqani and Al-Ghamdi, 2023e), the authors “Al-Humaiqani and Al-Ghamdi” assessed the Reflectivity (Rf), Flexibility (Fx), Resourcefulness (Rs), and Rapidity (Rp) resilience qualities. The study entitled “Assessing the Built Environment’s Reflectivity, Flexibility, Resourcefulness, and Rapidity Resilience Qualities against Climate Change Impacts from the Perspective of Different Stakeholders” utilized responses from the three respondent groups. The study is available for downlaod at this link: https://www.mdpi.com/2071-1050/15/6/5055. This research applied a similar methodology to assess the resilience qualities of Robustness (Rb), Redundancy (Rd), Inclusivity (Ic), and Integration (It). Both studies asses the built environment’s resilience qualities from the prespective of different stakholders, aiming to establish a framework that outlines the phases, characteristics, boundaries, and relationships of all eight qualities.
Keywords: climate change, built environment, resilience qualities, redundancy, robustness, inclusivity, integration, capacity
Citation: Al-Humaiqani MM and Al-Ghamdi SG (2024) Robustness, redundancy, inclusivity, and integration of built environment systems: resilience quantification from stakeholders’ perspectives. Front. Built Environ. 10:1343334. doi: 10.3389/fbuil.2024.1343334
Received: 23 November 2023; Accepted: 11 March 2024;
Published: 08 April 2024.
Edited by:
Giridharan Renganathan, University of Kent, United KingdomReviewed by:
Giles Bruno Sioen, National Institute for Environmental Studies (NIES), JapanCopyright © 2024 Al-Humaiqani and Al-Ghamdi. This is an open-access article distributed under the terms of the Creative Commons Attribution License (CC BY). The use, distribution or reproduction in other forums is permitted, provided the original author(s) and the copyright owner(s) are credited and that the original publication in this journal is cited, in accordance with accepted academic practice. No use, distribution or reproduction is permitted which does not comply with these terms.
*Correspondence: Sami G. Al-Ghamdi, c2FtaS5hbGdoYW1kaUBrYXVzdC5lZHUuc2E=
Disclaimer: All claims expressed in this article are solely those of the authors and do not necessarily represent those of their affiliated organizations, or those of the publisher, the editors and the reviewers. Any product that may be evaluated in this article or claim that may be made by its manufacturer is not guaranteed or endorsed by the publisher.
Research integrity at Frontiers
Learn more about the work of our research integrity team to safeguard the quality of each article we publish.