- 1Department of Civil and Environmental Engineering, Norwegian University of Science and Technology, Trondheim, Norway
- 2University of Perpignan Via Domitia, Perpignan, France
- 3Department of Mechanical and Industrial Engineering, Norwegian University of Science and Technology, Trondheim, Norway
Heritage and historical buildings often face climate vulnerabilities, decay of technical performance and energy inefficiency that threaten their use and consequently their long-term preservation. Due to urban densification and escalating energy consumption, addressing these vulnerabilities is crucial to protect, rehabilitate and keeping in use historical buildings in cities and utilizing renewable energy sources like solar energy should have a pivotal role towards sustainable cities and communities. However, integrating solar technology into historical buildings faces unique challenges mainly because of the significance, non-standard construction methods adopted, and the valuable original materials. Norway, with its Climate Action Plan and a substantial portion of cultural historic buildings in its building stock, aims to significantly reduce emissions and is seeking for novel solutions. This paper discusses challenges and barriers associated with adoption of solar energy in high-sensitive built environment in Norway, through a scoping review. The results outline conservation criteria as challenges, which include viability, feasibility, integration, reversibility, compatibility, reliability and safety, non-invasiveness, and acceptability. Additionally, the review identifies barriers such as economic, geographic, technical, conservative, legislative, and social factors. Initially, the frequency with which these challenges and barriers appear in academic papers is examined. Subsequently, the interconnections between these challenges and barriers are explored to assess their specific impacts within the Norwegian context. Recognizing these challenges and understanding their interconnection can represent the strength of the relationship between them and can allow to identify potential solutions and strategies to support stakeholders, experts, or public authorities for successfully implementation and integration of solar energy systems in high-sensitive built environments in the future.
1 Introduction
Due to climate change and low-carbon energy transition of cities and communities, nowadays decision-makers are aware that holistic and interdisciplinary approaches are necessary to design sustainable built environment. In that regard, a wider evidence base is needed to support conservation decisions in high-sensitive built environment (Hyslop, 2023). Regenerating historical areas and heritage sites with energy-efficient practices is a global imperative (Elena, Mariella, and Fabio, 2017).
It is estimated that more than 40% of the residential building stock in Europe was constructed before the 1960s (Irati and Trinomics, 2016). Currently, in Europe, around 35% of the buildings are over 50 years old and almost 75% of the building stock is energy inefficient (Bagaini et al., 2020). On the other hand, only 10% of the European building stock is listed under conservation restrictions (BIPV, 2022), meaning that energy-efficiency and retrofit interventions can be applied to a great number of historic buildings which can make them contribute to the mitigation of the climate change.
In 2015, to address global warming [5], the Paris Agreement was adopted at COP 21 (UNFCCC, 2023a), building on the principles of the United Nations Framework Convention on Climate Change (UNFCCC) (United Nations Framework Convention on Climate Change, 2022) and the Kyoto Protocol (UNFCCC, 2023b), setting a more ambitious goal to limit global warming to well below 2°C above pre-industrial levels, and to pursue efforts to limit the temperature increase to 1.5°C. This agreement includes a mechanism for countries to regularly communicate their Nationally Determined Contributions (NDCs), outlining their plans to reduce emissions, that it is also applied to heritage areas. In 2019, the European Climate Law (European Climate Law, 2023) was adopted by the EU, also based on the principles of the UNF (Hyslop, 2023) CCC (United Nations Framework Convention on Climate Change, 2022), the Kyoto Protocol and the Paris Agreement, by setting a target for the EU to be climate-neutral by 2050. Energy retrofit interventions become one of the most virtuous strategies to reduce building energy consumption and carbon emissions, but such practices are particularly challenging when they are implemented in historic and heritage-built environments (Webb, 2017). Notwithstanding, it is important to consider of renovating existing buildings, as significant energy savings can be achieved from this buildings stock which can contribute to reduce the EU (Europe)’s total energy consumption by approximately 5%–6% and lowering CO2 emissions by approximately 5% (Energy efficiency in buildings, 2019).
UNESCO emphasizes the importance of preserving historical areas and making them energy efficient to protect and promote the cultural heritage by supporting sustainable developments, for example, through energy efficiency, greenhouse gas (GHG) emissions reduction, and urban regeneration and adaptation to climate change (Centre UNESO world Heritage, 2022). Energy inefficiency poses several risks to historical buildings and urban areas. These risks include higher energy consumption, higher carbon emissions, an economic burden on building occupants or owners, and a disincentive to preserve and reuse of these buildings (Power, 2008). Making a historical building a (nearly) zero-emission building requires both, implement facility management in urban heritage (FMUH) areas in accordance to international, national, and regional heritage codes and laws [14, 15] on the one hand, and adopting a combination of strategies such as energy retrofit of the building, implementation of renewable energy sources (RES) installation and use on other hand.
In recent years the exploitation of RES, such as through the deployment of solar panels or wind turbines in historical areas, has significantly increased in Europe (Loli and Bertolin, 2018). In that regard, among the best practices case studies quoted in literature, are the Gloucester Cathedral in the UK, and the Herz-Jesus and Lutheran churches in Germany (Sudimac, Ugrinović, and Jurčević, 2020) where solar panels have been installed on sacred sites. Solar panels were also implemented on UNESCO monuments on top of Germany’s parliament, the Reichstag, on the Vatican, and also in the protected site of the Cinque Terre (Italy) (Leonardi, 2022). Renewable energy systems integrated into the façade have also been demonstrated by the case studies of Solar Silo in Switzerland (Leonardi, 2022). In particular, rooftop solar adoption has been accelerated by the current energy crisis, although the spread of solar energy systems into historical and landmarked buildings still remains limited (Pv Magazine, 2023). The reason states in the many existing challenges and barriers like the costs, aesthetic impact, space restrictions, historical preservation, and maintenance. Notwithstanding the few best practices quoted above represent a exemplary buildings of the alignment between the needs of historic preservation and the integration of solar systems in historical buildings.
Under this perspective, with the Norway’s Climate Action Plan for 2021–2030 and a number of cultural historic buildings representing 11% of its existing building stock (Building Stock, 2022), Norway aims to reduce its non-ETS emissions by 45% by 2030 (Ministry of Climate and Environment, 2020). This paper aims to provide a holistic perspective about the existing challenges and the barriers in the implementation of solar energy technology and systems in historic districts through a scoping literature review. The paper presents a novel examination of the adoption of solar energy in Norway’s highly sensitive built environments. Its uniqueness stems from a specific focus on Norway, providing insights tailored to its distinct geographical and socio-cultural constraints in deploying solar energy system because of its challenge climatic conditions and national legislation restrictions. Additionally, while it identifies challenges from a historical conservation perspective and barriers, a standout feature is its exploration of the interconnections between these challenges. This approach not only deepens our understanding, but also offers practical utility for stakeholders aiming to integrate solar solutions in projects. This paper has been organized as follow: Section 2 summarizes the background to the subject by revealing the global trends and policies for solar technology adapted to historic buildings; Section 3 clarifies the scoping methodology; Section 4 includes the results of the scoping review and the discussion and interpretation of the results from a challenges, barriers, and opportunities perspective; and Section 5 concludes the paper with some suggestions for future development.
2 Background
2.1 Norwegian policy and trends considering historical urban areas
In Norway, historic buildings represent more than 11% of the building stock (Building Stock, 2022) and the number is increasing. The government responsible for those buildings is the Ministry of Climate and Environment with the Department of Cultural Environment, which assure the protection and the sustainable use of cultural monuments and sites (Regjeringen, 2013). The Department is directly in contact with UNESCO on World Heritage Sites and delegates the local management of sites to the Riksantikvaren (RA-Norwegian Directorate of Cultural Heritage) and the Norwegian Cultural Heritage Fund [15] (Figure 1).
In Norway, electricity production is already almost entirely decarbonised, as more than 95% of the generation comes from RES, mostly produced by hydro-powerplants (almost 90%). This contribute to explain why the country has the lowest GHG emission rate (27 g/kWh in 2021) Concerned about the effects of climate change, Norway is one of the pioneer countries to have established, in 1991, the carbon tax, sanctioning carbon-equivalent emissions from industries and consumers (‘Emissions to Air’)across Europe (Nowtricity, 2022). Concerning the energy consumption, Statnett predicts an increase by 2050 due to a growth in demand for energy-intensive industries and new industries, for digital information storage in data centres and for the development of the hydrogen sector followed only by a minimal decrease in petroleum and general consumption (Statnett, 2022). This increase of energy demand enhanced by a general goal of electrification of the transport and industry sector implies a need to boost the power production in Norway. Statnett’s forecast for the future electrical mix shows a general growth of RES where solar capacity would establish itself from less than 1 TWh in 2020 up to 10 TWh predicted within 2050. In Norway, depending on the photovoltaic (PV) system purchased, the Levelized Costs of Electricity (LCOE) finds itself in-between the range of (0.69–1.13 NOK/kWh which corresponds to 0.07–0.11 US$/kWh) and is close to the international market (0.07 US$/kWh) (Global LCOE and Auction Values, 2022).
2.2 Photovoltaic technologies for the built environment
One of the most relevant RES for the built environment is the use of solar energy due to small-size systems, high customizability on the installation surfaces and its potential that is still highly unexploited. The advantages of PV installed into the buildings’ envelope (i.e., roofs, facades, windows) and architectural features (e.g., balconies, shading devices), aside from the integrated design (i.e., aesthetical and technological value) and market affordability (i.e., dropping down costs for PV), are: lightweight for easy transportation, quiet energy conversion, and a relatively extended lifetime requiring few maintenance interventions (Valancius et al., 2018). According to the International Renewable Energy Agency (IRENA), the LCOE of PV has decreased by more than 80% since 2010 (0.38$/kWh in 2010 and 0.07 $/kWh in 2019) (Our World in Data, 2022). Allowing a high degree of integration, Building Integrated Photovoltaics (BIPV) development was a game changer for PV applications on sensitive and historic buildings, as solar cells became not easily distinguishable through changing the colour of the PV panels (e.g., coloured BIPV) or through changing the texture of the panels (e.g., anti-reflection coating) (Light Coatings, 2022). Some most recent solutions are now considering the shape of the cells to allow further better integration such as the terracotta-style PV tiles (Pv Magazine International, 2022). Most of common customized solar solutions are BIPV technologies integrated into the roof, into the façade (although those have more limitations in their implementation in case of historic buildings), or even replacing glazed surfaces with what is called Window Integrated Photovoltaic (WIPV). Being part of the building’s envelope, BIPV systems should guarantee optimal performance as the traditional cladding materials such as building fire and safety protection, thermal comfort, noise insolation, water and air tightness and Sun shadowing (Lucchi, Polo Lopez, and Franco, 2020).
In Norway, the PV sector only started to develop over the last decade. In 2010, the Norwegian Water Resources and Energy Directorate (NVE) published a change in the Norwegian legislation which brought the possibility of self-generating electricity that was previously neglected by the law (Nordahl, 2022). After this event, the number of solar installations increased steeply: from 9 MW installed in 2010 to 225 MW in 2021. Recently, regarding top-down incentives to promote PV installations, Enova, the Norwegian energy agency owned by the government, is providing financial support for PV installations on households. At a local level, some municipalities are also providing financial help for affording the investment cost of PV, as did by the Oslo municipality (Jackson et al., 2020).
3 Methods
This review study follows the “scoping methodology” as defined by H. Arksey and L. O’Malley (Arksey and O’Malley, 2005). This method allows to examine a specific subject, then to summarise it and to share findings with concerned actors. One purpose of conducting a scoping review is the identification of knowledge gaps existing in the literature to determine the future research trajectories to have a broader societal impact. Figure 2 depicts the research steps implemented in the scoping review. The Step 0 – Scope of the study defines the scope of the study by delineating the research questions and defining the set of the keywords. Several search rounds have been carried out to do it. The Step 1 - Identification allowed the identification of articles from two scientific database engines: Google scholar and Scopus. In the Step 1, some boundaries have been set. The period has been defined to target only the most recent case studies (since 2010), as solar technologies especially BIPVs are evolving fast. Then the search has been implemented to catch the existing literature with or without “Norway” and at least one of the other keywords reported in Step 0. The diagram in Figure 2 summarises the inputs and outputs for each step of the conducted scoping review research process.
The Step 2 – Screening and filtering has involved a detailed screening and filtering of the outputs reading both the titles and the abstracts, arriving at the final Step 3 – Inclusion with a total selection of 34 articles. In this step, the target articles for the literature review were selected and their analysis was focused to: (i) chart the data into categories of interest, Step 4 – Charting the data, and (ii) clusterize the information according to the different challenges and barriers, Step 5 – Clusterizing information (Figure 3).
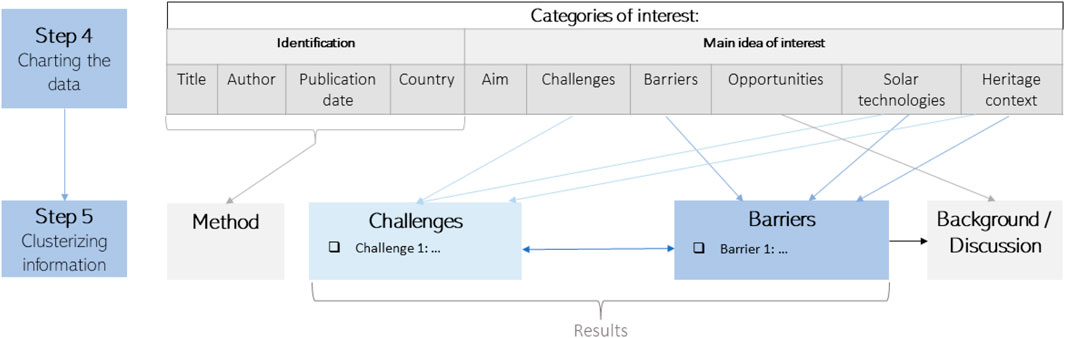
FIGURE 3. Scheme representing the process to extract and cluster information obtained by the scoping literature review.
The useful information from each paper was extracted and categorized in a database focused on the identification details and the main idea of interest as aim, challenges, barriers, opportunities for solar technologies in high-sensitive contexts which considered historical and heritage built environment (Step 4, in Figure 3). Then (Step 5, Figure 3) the information in the database was clustered and reorganized: the challenges were defined as a milestone that needs to be addressed, and they are connected to open solutions or opportunities, whereas barriers were defined as obstacles.
4 Results and discussion
The results are presented as an analysis of the background information in the selected papers (e.g., country, authors, year, topic), and then as an in-depth assessment of the articles in terms of challenges and barriers by highlighting the existing links between them via the conservation criteria. In fact, this is the connection map that allows to individuate the potential solutions and opportunities. Only three over 34 articles were included after an external recommendation by experts and the snowballing effect. In detail the recommended paper was concerning the People, Public, Private, Partnership (PPPP) approach to overcome social and cultural challenges to the diffusion of photovoltaic in Norway.
4.1 Geographical distribution and knowledge gaps
As case studies in Norway are rare concerning solar implementation in historic sites, articles from all around the world have been selected and analysed through the Norwegian legislation lens. The Figure 4 maps out the countries with the selected articles in the topic of PV in high-sensitive built environment. Most of the selected literature comes from Europe, while few cases are in US and Egypt.
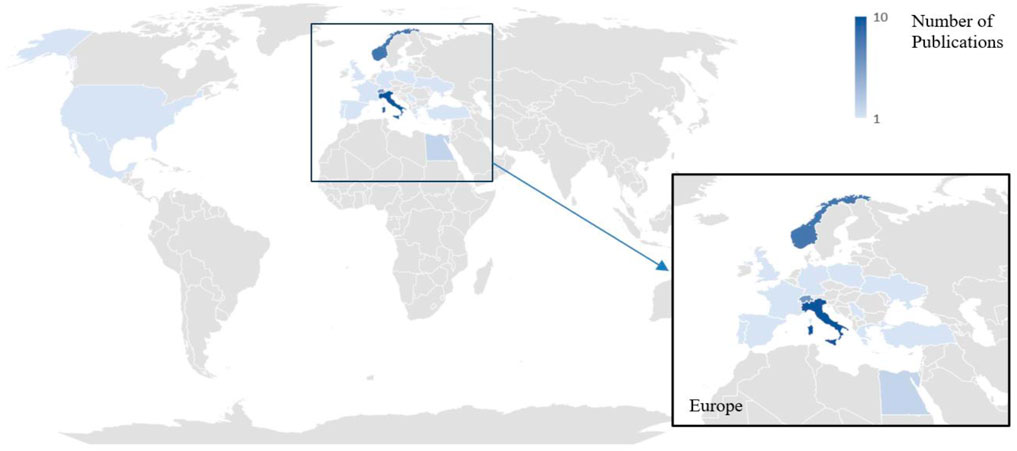
FIGURE 4. Geographical distribution of studies on the topic of PV in high-sensitive built environment.
The country with the most occurrences is Italy (eight times) followed by Switzerland (four times). Indeed, four articles are the result of a collaboration on the topic between Italy and Switzerland and Norway. In that case, two of those articles were distributed to Italy and the others two to Switzerland. Except Egypt with two papers, the other countries containing Spain, Portugal, Ukraine, Poland, Turkey, Germany, Greece, Serbia, UK, Mexico and France are represented by one paper. Looking at the main authors that stand out from the list, a researcher from Switzerland (Cristina Polo López) and from Italy (Elena Lucchi) are both listed in six articles which were four times co-written. Those two authors are mostly focusing on Italian case studies and the cooperation between Italy and Switzerland in conducting energy efficiency research. All selected articles had to fit at least two of the keywords as they are located at the intersections of the key topics (Figure 5). It is worth to notice that most of the selected references (n = 26) address solar energy applied to historic buildings with their associated challenges and barriers. Eight papers are applied to Norway and only one paper considers all three aspects of solar energy implemented in historic building in such country. However, since no paper specifically addresses challenges and barriers implementing solar technologies in high-sensitive areas, this corresponds to the knowledge gap that this review paper aims to tackle in the next sub-sections.
4.2 Challenges
4.2.1 Dualism between conservation and sustainability
In literature, when dealing with solar energy implementation on historic buildings, the major drawback is the dualism between the conservation values required by the historic buildings and the sustainability needs derived from the deployment of RES systems to improve energy efficiency and reduce GHG. Recently the awareness of compromising both aspects is urgent and necessary. In fact, conservation criteria – read at the light of a trade-off between preservation rules and sustainability targets – can be perceived as guidelines for successful BIPV implementation projects that can overcome the challenges and barriers associated with historic buildings.
According to (De Medici and Stefania, 2021; López et al., 2021a; Kalliopi et al., 2022), the historic buildings are associated with a great number of values, such as social, cultural, aesthetic, historical, symbolic and also sentimental, which explain the need for a holistic approach regarding the heritage context (Kalliopi et al., 2022). Those values underline the importance and the characteristics such as physical elements (e.g., topography, vegetation, water features, and geology), as well as cultural and historical elements such as architecture, history, and cultural significance of a site for the society and the need to preserve them (López et al., 2021b). The preservation of historical values assures the integrity as well as the authenticity of a site through time and stands as a witness of the past (Guttormsen et al., 2011; Lopez, 2020; Lucchi, 2020; López et al., 2021a).
On the other hand, historic buildings are not always well maintained and often they need energy system retrofitting interventions in line with the strict energy-performance requirements within the framework of the Europe’s legislation and climate-neutral strategy. Articles refer to the need of finding a balance between conservation and sustainability, with expressions such as:
“…finding balance between authenticity and contemporaneity.” (López, 2021a)
“…energy efficiency conservation-compatible solutions.” (López et al., 2021b)
“…often change requires compromise – a trade-off between adding an attractive function and impairing the ‘spirit of the place’.” (Roszczynska-Kurasinska et al., 2021)
“…compromise between conservation and energy needs.” (López, 2021a)
Indeed, sustainability in the built environment can enhance the reflection on sustainable decisions in our daily lives although the exploitation of the RES in the heritage context are still critical (López et al., 2021a). The concept of “sustainable heritage” has been explored, among the others, by the Routledge handbook of sustainable heritage (Kalliopi et al., 2022) and it can be defined by two approaches:
The “responsive approach”: reaction to the fact that heritage is threatened and needs protection.
The “dynamic approach”: sustainable heritage can act as a catalyst for environmental, social, and economic sustainable development (being the three pillars of sustainability).
To define the right compromise for retrofitting, a transformability ratio can be delimited (López et al., 2021b). Transformability ratios are used in retrofitting heritage buildings to balance the energy performance requirements with heritage elements’ protection. The aim is to find the right compromise between preserving heritage elements and providing a more sustainable and energy-efficient building. Heritage can either be protected in a responsive manner or used to create sustainable development through a dynamic approach.
4.2.2 Conservation criteria
The transformability ratio can be evaluated following the conservation criteria identified in the literature. The selected criteria Figure 6 are the following: (1) viability, (2) feasibility, (3) integration, (4) reversibility, (5) compatibility, (6) reliability & safety, (7) non-invasiveness, and (8) acceptability.
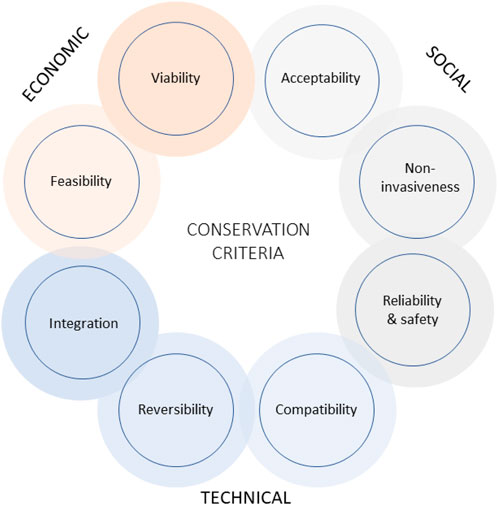
FIGURE 6. General conservation criteria for the implementation of solar technologies in historic urban areas. Blue color refers to technical criteria, light orange to economic criteria, while light grey to social criteria.
All the criteria, in the framework of sustainability, are expected to be connected either with an economic, technical, or social aspects. From the literature, the most quoted criteria are integration, compatibility, and reversibility, which we consider here as technical aspects (Figure 6, blue colour). A short definition of the conservation criteria at the light of the implementation of solar technologies in historic urban areas is as the follow:
(1) Viability: economic viability refers to the difference between the total costs in a BIPV installation (i.e., initial cost, operation & maintenance) and the income (i.e., electricity price sells to the grid defined by local feed-in tariffs). If the difference is smaller or equal to the costs of electricity consumption from the grid, then the project is viable or even profitable (López et al., 2021a).
(2) Feasibility: economic feasibility is the analysis of cost/benefit that can be evaluated through economic indicators such as the Net Present Value, the Levelized Cost of Energy, the return on investment, or the payback period (Sommerfeldt and Madani, 2017).
(3) Integration: technical integration of BIPV to the existing roof structure should address architectural and aesthetic aspects (López, 2021b). A “morphological integration” indicates that the layout and shape of the panels harmonise with the surrounding built environment (Durante, Lucchi, and Maturi, 2021).
(4) Reversibility: technical reversibility means that it is possible at any moment to take away the BIPV installation and go back to its formal condition. Reversibility also means that it is possible to replace the BIPV panels without affecting the integrity and the historic value of the site (Kandt et al., 2011). This means that – if applied to historic buildings - the PV installation should be attached as a mountable and demountable structure that would avoid affecting the features of the property while replacing them (Sudimac, Ugrinović, and Jurčević, 2020).
(5) Compatibility: the compatible criterion is covering several aspects such as technical (e.g., choice of materials, fixing system and hygrothermal components - avoiding the development of moisture), structural (to avoid excessive deflection due to e.g., snowfall, wind gusts, and ageing), aesthetic (choice of colour, texture of materials, spatial layout and morphological fit (López, 2020)), chemical (difference in chemical components and properties between old and new materials that could react together (López et al., 2021b)) and functional (more complex for BIPV that implies multi-functional properties beyond the generation of electricity, but also replacing tiles, the façade or the windows and therefore needs to fulfil its multi-function).
(6) Reliability & safety: Reliability refers to the assessment of the quality and durability of the transformation after the BIPV installation, which should be made in a way to avoid maintenance and decay (De Medici and Stefania, 2021). Whereas safety is evaluating the risks generated by the installation (e.g., fire safety, electrical safety, safe structure for maintenance operations).
(7) Non-invasiveness: this criterion has a twofold significance. On the one hand, non-invasiveness corresponds to the conservation value of keeping some authentic features on the building, on the other hand, it can be interpreted as the idea of not “tricking” the eye and exposing clearly what is authentic and what has been added (Kandt et al., 2011). The replaced parts of a building envelope should be distinguishable from the rest, while still being designed in harmony with its environment (López et al., 2021a; López, 2021b).
(8) Acceptability: Acceptability is hard to predict or measure as it is not tangible or material value. It strongly depends on the significance of a place’s values e.g., historical, sentimental, symbolic, cultural, and social and whether those values are respected through the transformation. It can be seen as an individual perception or a community’s perception with strong societal components.
The above-listed criteria highlight the need for a multidisciplinary approach concerning the implementation of solar technologies in historic or high-sensitive neighbourhoods. Starting from this definition of all the conservation criteria, the connections map between the challenges in respect to these criteria and the barriers related to the use of solar energy in high-sensitive districts can be built. It is important to underline that those conservation criteria, beyond the function of privileging certain types of solar technologies, they comply a new methodology that stakeholders can use to identify the constraints and the adequate systems which consider the conservation criteria to each specific case.
4.3 Barriers
4.3.1 Economic barriers
Among the detected barriers, the financial aspect is often reported as one of the major downsides for PV-BIPV installations (Kandt et al., 2011; Jackson et al., 2020; Rosa, 2020; López et al., 2021a; Ibrahim et al., 2021; Basar Baypinar et al., 2022). In the first instance, high initial costs are responsible for the reluctance from property owners as the investment for a PV installation is quite high and imposes a long payback period (Kandt et al., 2011; López et al., 2021b). As a point of fact, BIPV systems are more expensive than BAPVs because of their multi-functionalities. Hence, by replacing partially a building’s structure, BIPV is avoiding costs that would otherwise be spent on the building’s envelope (Jackson et al., 2020; Rosa, 2020). Costs related to experts such as architects, engineers, and specialists in historical preservation should be considered. These experts can ensure that the installation is performed correctly and in a way that preserves the building’s appearance and history. Due to historic or heritage building constraints, there is a need to proceed with a professional feasibility assessment for solar installation on historic buildings considering all technical aspects and complying with Directive 2010/31/EU that implies a case-by-case study to each retrofit intervention. Indeed, the costs for such expertise requires a large collection of data and subsequently takes time to design an implementation plan (Basar Baypinar et al., 2022). Uncertainties related to the retrofitting investment also contribute to economic barriers. Undoubtedly, PV and even more BIPV are strongly related to such financial uncertainty as the prediction on the generated income depends on the energy-performance of the technology, on the fluctuations in energy market variations in feed-in tariff (FiT) and transmission costs, on unpredictable weather conditions, and on unforeseen losses in performance due to an alteration of the technology thus that the operation and maintenance costs may become highly unpredictable. This means that the grid companies (DSOs) - adopting varying practices towards their feed-in policies and being responsible for setting tariffs for which prosumers can sell their electricity - may act as a barrier to prosumers themselves (Jackson et al., 2020). The lack of public or private economic incentives has also been proven to act as an inhibitive agent (Tzetzi, 2019; Lucchi et al., 2022). To overcome the high initial costs, financial support would motivate householders to consider proceeding with the installation (Jackson et al., 2020). Limitations can result from the average income in certain districts or countries that sets sustainability goals behind other and more urgent concerns. This leads to geo-economic barriers depending on the location of the installation site.
4.3.2 Geographical barriers
Often, the location of a building has a major impact on the feasibility of a BIPV implementation as it is subject to the local legislation protecting cultural heritage. As a matter of fact, most of the articles found in the literature come from the same continent (31/34), namely Europe. This suggests that most implementation projects on historic buildings are in EU due to the high concentration of historic buildings. Secondarily, differences in politics, policies or social behaviour may further support this geographical bias towards a lower implementation of BIPV in Mediterranean areas despite the availability of hours of sunshine as observed in the literature (Ibrahim et al., 2021). Even in Europe, the distribution of BIPV implementation is inhomogeneous, being driven mostly by project incentives coming from Belgium, Spain, Italy and Germany (Sánchez-Pantoja et al., 2021). Beside policies, intrinsic differences in PV performances from country to country are directly linked to differences affecting the local outdoor temperature. Hence the PV technology decreasing efficiency with warmer temperatures (Ibrahim et al., 2021). The geographical barriers due to variation in legislation will be discussed in Section 4.3.5.
4.3.3 Technical barriers
Solar systems can be successfully integrated through deep renovation processes that involve morphological and material changes, (Formolli et al., 2022), while technical barriers in BIPV implementation on historic buildings are directly linked to the integrity, feasibility, reversibility, compatibility, and reliability & safety conservation criteria. In this framework, BIPVs are still considered at their juvenal stage, as these conservation constraints are not or only partially considered in products available in the market. Compared to “conventional PV”, in which the technology has been well explored and it is close to its maximum electrical efficiency, BIPV -especially coloured BIPV and WIPV- is making its first steps on the market. Indeed, BIPV has still a low market penetration and can be qualified as a “niche market” (Rosa, 2020), still very far from a megatrend where prosumers are the default situation. Furthermore, the outreach to a whole new market considering the compatibility with historic buildings materials opens an innovation path to areas unconsidered until recently. There is limited information available on BIPV performance and operation and maintenance requirements which needs throughout its entire lifespan especially in the Norwegian context (Pelle et al., 2020). The lack of available data can complicate operation and impact the feasibility of BIPV projects, especially if annual irradiance data are missing (Imenes, 2016). It is particularly relevant for Scandinavian countries, such as Norway, where 3D irradiation maps are required to assess façade BIPV’s potential. In addition, there are a limited number of Environmental Product Declaration (EPDs) and Life Cycle Assessments (LCAs) available, which can affect the sustainability assessment and implementation of BIPV projects. (López et al., 2021a). There are several technical uncertainties associated with BIPV. In the case of coloured BIPV, there is uncertainty about how the colour will appear after lamination. In addition, there are uncertainties regarding the durability and lifespan of the technology, which may have an adverse effect on its long-term performance and cost-effectiveness (López et al., 2021b). Another technical uncertainty relates to BIPV’s energy production, which can deviate up to 20% due to various weather perturbations and soiling. Furthermore, in regions with cold climates, such as Norway, snow and ice can significantly affect energy production (Imenes, 2016).
Hence BIPVs technologies need to be highly adaptable and customizable due to the aesthetic integrity requirements from conservation regulations requiring case-by-case studies, thus enhancing the products fragmentation in the market. In addition, once in compliance with legislation and with weathered parts in needs of replacing, BIPVs by substituting parts of a building’s envelope are multi-functional as they need to protect from bad weather (roof BIPV panels or tiles), natural light (parapet for Sun shading) or provide thermal and acoustic insulation (WIPV) (Durante, Lucchi, and Maturi, 2021). However, such large range of possibilities makes it even harder for professionals and stakeholders to keep themselves updated on the BIPVs market evolution and at the same time it makes these technologies fragmented. Consequently, at present, it is difficult to quickly progress towards series production which could lower production costs (Pelle et al., 2020).
Architectonic constraints imposed by existing buildings also contribute to technical barriers. Indeed, BIPV should preserve the architectonic image and the construction requirements such as easy mounting and dismounting (i.e., fulfilling the safety and reversibility criteria), fire safety, climate resistance, hygrothermal risks reduction, thermal stability and durability (Pelle et al., 2020). Additionally, the weight of BIPV alternatives to tiles or façade can also affect the building’s historic structure which may need to be consolidated. Lastly, buildings with a long history are hard to trace when it comes to their architectural or structural transformation. Indeed, documentation on past refurbishment or energy retrofitting to which a historic building may have been exposed are not always kept or made easily accessible (Basar Baypinar et al., 2022), which makes the physical and chemical compatibility assessment hard to establish.
In a nutshell, the complex and holistic approach linked to technical barriers implies a great amount of time, effort, and motivation for carrying on such a project. The complexity of the task first arises in the data collection process for a feasibility assessment (i.e., data concerning solar radiation, environment parameters, mapping of the built environment, mapping of original types of materials and state of conservation, technical data sheets, and local/national legislation concerning historic buildings). Furthermore, the need of customization combined with the impossibility of large-scale replicability contributes to the step-by-step process of BIPV implementation on historic buildings and high-sensitive built environment.
4.3.4 Conservation barriers
The conservation aspect, not only from the legislative but also from a social-cultural point of view, can be assessed with the compatibility and non-invasiveness criteria. The main barrier when it comes to the conservation of sites is the restrictive and prohibitive rules that have the purpose of protecting the integrity and authenticity of a site (Ibrahim et al., 2021), but can have the effect of hindering energy-saving measures (Tsoumanis et al., 2021). Strict laws on aesthetic integration, compatibility, and non-invasiveness make it hard to find adapted technologies complying with the specifications which induce technical constraints. Indeed, European guidelines for RES integration encourage adapting modules to the inclination of the roof, following the roof frame and ridges, grouping the panels, covering pipes, cables as well as anchoring elements and harmonising the colours and textures with the initially built environment (De Medici and Stefana, 2021). Italian regulation even fixes a limit to the maximum elevation of the panels to 20 cm and specifies the need to hide connections of cables or pipes (López et al., 2021a). Restrictive attitudes towards historic districts are often correlated with reluctance to renovation and change. As a consequence, “conservative conservation” may end up transforming sites that carry high historical, social and cultural values into “petrified sites” (Roszczynska-Kurasinska et al., 2021). On the other hand, more tolerant and open to change sites can become breeding grounds for innovation and social evolution. Conservation site degeneration (López et al., 2021a) or site under a decaying process (Sesana et al., 2019) may alter not only the socio-cultural attractiveness of the place but also weaken the building’s structure and features making it more challenging for retrofitting purposes. This type of conservative behaviour makes echo with the principle of the “critical restoration” movement, led by Cesare Brandi in the 20th century, who developed a strict vision of restoration on heritage sites (Meraz, 2019). Conservation regulations on historic buildings are operated through legislation, which induces further barriers discussed in the following section.
4.3.5 Legislative barriers
Legislative and procedural barriers are related to the acceptability, reversibility, and compatibility of BIPV implementation in historic districts through policies. The main obstacles are the complication of the authorization process and the lack of clear legal guidelines. Strict regulations strongly contribute to the complexity of the authorization process. It has been proven that in countries with stricter regulations, such as Italy, fewer solar initiatives have been counted than in more tolerant states such as Switzerland (Lucchi et al., 2022). More than that, the legislation fragmentation, due to a great number of legislative parties and levels (e.g., European, national, regional, sometimes provincial, and municipal), is slowing down the authorization process. In Italy for example, BIPV implementation on protected sites must be approved by several institutions (López et al., 2021a), making the whole process discouraging (Lucchi et al., 2022). In the US, private owners who have the will to undertake a solar installation may need to call upon an administrative body specialized in preservation (Kandt et al., 2011). The complexity of authorization requires a great amount of motivation, which can rapidly reveal overwhelming, time-consuming, and therefore dissuading. The lack of guidelines and legal framework has been expressed in most of the case studies (Tzetzi, 2019; Pelle et al., 2020; Roosmalen and Gritzki, 2020; Rosa, 2020; Roszczynska-Kurasinska et al., 2021; Basar Baypinar et al., 2022; Lucchi et al., 2022) including Norway (Sesana et al., 2019). Hence the recentness of solar integrated technologies, existing guidelines refer exclusively to BAPV without considering less intrusive systems as BIPV (Pelle et al., 2020; Lucchi et al., 2022). In most of the cases, the legislation does not trigger the case of historic or heritage buildings for solar energy purposes (Basar Baypinar et al., 2022). The lack of a legal framework can be explained by the impossibility of universal solutions due to the uniqueness of each site. Indeed, no general rules can be drawn for solar energy installations on historic buildings as each assessment is to be led on a case-by-case basis due to differences in legislation from country to country, even within a country between levels of protection, typologies of buildings, connection with neighbouring districts and types of owners. Differences occur even at the European scale (López et al., 2021a), for example, comparing the Italian and the Swiss approach, which are two countries with high cultural values (Durante, Lucchi, and Maturi, 2021), the PV implementation is low considered through policies in Italy, while well guided in Switzerland (Lucchi et al., 2022).
In-between countries, but also within countries there are different levels of protection for buildings of importance, generally linked to the degree of significance. For example, in Switzerland, authorization is not required for PV installation on historic sites while it is the case for monuments of cantonal or national importance (Lucchi et al., 2022). Consequently, the difference in protection level leads to different possibilities of intervention, which help determine the degree of integration that must comply with the technological, energy and aesthetical aspects (Pelle et al., 2020). The uniqueness of a historic center also relies on its specific relation with surrounding districts (Mete Basar Baypinar, Enes Yasa, Selahattin Ersoy, Cem Beygo, Kerem Beygo). Indeed, regulation may also apply to un-listed buildings located close to heritage sites that may be part of a protected settlement (Lucchi et al., 2022). To develop research and development infrastructure, a public-private partnership (PPP) approach is appropriate (Abdullah et al., 2017).
Missing national guidelines may also be explained by the lack of international incentives. At the European scale, the diversity in Member States’ legislation makes it challenging to define general rules or even recommendations (De Medici and Stefania, 2021). Increasing the efficiency of buildings and utilizing renewable energy sources by BIPV is encouraged and supported by EU legislation. Building energy efficiency is a primary objective of the Energy Performance of Buildings Directive (EPBD), which requires Member States to increase the use of renewable energy sources, including BIPV (‘Energy Performance of Buildings Directive’ 2022). Furthermore, the EU provides funding for energy retrofits and BIPV projects aimed at reducing greenhouse gas emissions and promoting energy efficiency through its Horizon 20220’program.
Not only lack of general guidelines has been raised by the literature, but the existing regulations lack clearness and comprehensibility. Comparing Italy with Switzerland, the Italian legislation is very fragmented leading to unclarity in the authorization process whereas Switzerland’s laws are clear on the subject and even simplify procedures for PV implementation, which is accelerating the process (Lucchi et al., 2022).
The complexity of terminology is also contributing to unclear regulations. The terms used to specify the sites to protect are various and are used as synonyms or interchangeably although having different meanings (e.g., historic buildings, listed buildings, heritage buildings or landscapes, cultural heritage, archaeological area) (Pelle et al., 2020). This happens also when it comes to actions on the buildings (e.g., rehabilitation, restoration, reconstruction, retrofitting, refurbishment) (Kandt et al., 2011).
Regulatory uncertainties are part of the unclarities in policies. The case study based in Ukraine found contradictions and inaccurate terminologies in legislation (Shuldan and Al-Akhmmadi, 2021), leading to confusion and uncertainties towards the legal possibility of a PV installation on ahistorical building or heritage sites.
Even further than unclarities and uncertainties is the lack of consistency in legislation, which can act as a barrier for stakeholders. It has been demonstrated that nearly Zero-Energy Buildings (nZEB) are unattainable for historic buildings due to the impossibility of intervention on their external vertical structures (Durante, Lucchi, and Maturi, 2021). Unsurprisingly, unclarity can lead to misinterpretation of the law, which is mentioned in a Norwegian case study (Sesana et al., 2019).
4.3.6 Cultural and social barriers
The cultural and social barriers are linked to the acceptability and non-invasiveness conservation criteria. Cultural and social barriers have first to be well understood before any solutions can be found and discussed. This part is divided into three subsections, namely, the lack of knowledge and awareness, then the lack of communication and co-creation between the stakeholders, and finally the lack of social acceptance.
The lack of awareness does not only concern citizens but also applies to all stakeholders as well as experts and scientists. First, there is a lack of interest in the literature due to scarce research studies. Indeed, as BIPV implementation on a historic building is a cross-disciplinary project, there is a need for scientists from different fields to sit at the same table, such as social scientists, heritage science researchers and conservators, physical scientists and engineers to collaborate, share knowledge, but also learn together (Kalliopi et al., 2022). As for now, the subject is not well enough explored, especially when it comes to social acceptance and the aesthetic impact of renewable energy technologies (Sánchez-Pantoja et al., 2021) as those are non-tangible and hard to assess factors. The lack of documentation for citizens and stakeholders concerning existing guidelines and technologies has also been highlighted, it is a drawback as it increases the time and effort that has to be invested (Kandt et al., 2011).
Pilot projects are the key to illustrate the scope of possibilities and motivate stakeholders to initiate actions in that direction. The lack of concrete examples of BIPV systems in historic areas is hindering their consideration for urban planning processes. In Italy, the relevance and need of platforms illustrating successful case studies was emphasized (Lucchi et al., 2022). Furthermore, municipalities emphasize the benefit of promoting local examples, e.g., with guided visits, as it allows to spread awareness (Shuldan and Al-Akhmmadi, 2021).
The scarcity of showcases explains the general lack of knowledge, not only concerning BIPV technologies but also when it comes to the preservation rules applied to historic buildings or the general steps for an implementation process (Kandt et al., 2011). This applies not only to citizens but also to stakeholders who need more training, for example, with post-graduate courses (Lucchi et al., 2022), and updates towards the legal framework, the conservation criteria and the authorization and implementation process (Lucchi et al., 2022), as well as to professionals (e.g., architects and designer) to gain knowledge on the latest technologies on the market (e.g., coloured BIPV, WIPV) (Pelle et al., 2020).
The lack of communication between stakeholders ends up in an absence of co-creation, although mandatory for such an inter-disciplinary project. Indeed, scientists and policymakers are often disconnected which may result in frustration or miscommunication in feedback (Basar Baypinar et al., 2022). To effectively refurbish historical buildings, it is important to adopt a cross-disciplinary approach and prioritize user-oriented design. This approach takes into account the perspectives of various stakeholders and promotes sustainability and inclusiveness (Kristl, Temeljotov Salaj, and Roumboutsos, 2020). The important variety of stakeholders involved (e.g., Public entities: governments, decision-makers, municipalities, Private entities: architects, builders, engineers, research centres, DSOs, third-party installers, technicians, renovation professionals, People: citizens, tenants & owners) explains part of the problem. The first issue in such a wide consortium of involved actors is the difference of concerns from the Public, Private and People (PPP) entities. The three types of entities have divergent approaches to the three pillars of sustainability i.e., environment, society and economy (Xue, Lindkvist, and Temeljotov-Salaj, 2021), different priorities, different understanding to the problem, and different communicative language. These differences in objectives can result in the manipulation of scientific evidence and data to fit their respective interest (Basar Baypinar et al., 2022). Furthermore, it has been observed that public administration, with the hands of heritage authorities, tends to shift their focus, during the authorization process, on conservation compatibility rather than on energy performances or technical as well as sustainable compatibility (López et al., 2021a). Those mutual oppositions are explained by the lack of space (e.g., virtual tools) to build a common understandable language between stakeholders (Pelle et al., 2020).
There is a shared consensus in the literature that a common language only makes sense in case all stakeholders (i.e., individuals as well as institutions and companies) are included and have the ability to get involved and take decisions (Pelle et al., 2020; Tsoumanis et al., 2021; Sánchez-Pantoja et al., 2021; De Medici and Stefania, 2021; López et al., 2021a; Kandt et al., 2011; Thebault et al., 2020; C. S. P. López and Frontini, 2014). End-users should not be forgotten as they both fund the project and take benefit from it (Thebault et al., 2020), a wholesome approach should keep the centre point on the end-users needs and expectations.
Resulting from a general lack of knowledge materialized by a deficit in exemplary projects comes a lack of social acceptance. Insufficient social acceptance is not only induced by individual perception and reticence but also originates from a community’s perception and influence. First, the misconceived anaesthetic perception of solar systems, is resulting from a lack of awareness about recent integrated and discrete technologies. This contributes to the first stop to consider solar on private rooftops (Pelle et al., 2020). This individual hesitation to new implementation projects could also be explained by the fear of change due to the immutable connotation of historic buildings, and the lack of participatory tools. Indeed, there is an expressed need (Tsoumanis et al., 2021) to develop visualisation technologies and smart city tools to break the ice with social misconceived ideas. Although sharing a project in conception is not enough, individuals’ opinions should be considered in the decision-making process to prevent rejection of the proposed project.
The community’s opinion can be evaluated through the intrinsic value. The intrinsic value is a concept addressing intangible cultural heritage principles and it is also part of the social values of a place as it reflects its importance towards the local community (Roszczynska-Kurasinska et al., 2021). The intrinsic value is often forgotten during the feasibility project assessment in a specific place and its measurement would allow to evaluate social acceptability before the effective implementation. A Norwegian case study (Guttormsen et al., 2011) illustrates how intrinsic value is not necessarily correlated to authenticity. Intrinsic value may have roots in past authenticity, but it is evolving over time adding new components to its intangible value coming from the community that is leaving the place over decades and centuries.
To conclude the results found in the literature, even though the panel of identified barriers seems wide and shifted towards different directions, all of them are interlinked and may induce or be induced by another barrier. This is clearly highlighted in the fishbone diagram in Figure 7 that is depicting such connections and illustrated 6 main barriers; 1) Economic, 2) Geographic, 3) Technical, 4) Conservation, 5) Legislative 6) Social and its causes to identify the roots to utilize solar systems in historical district
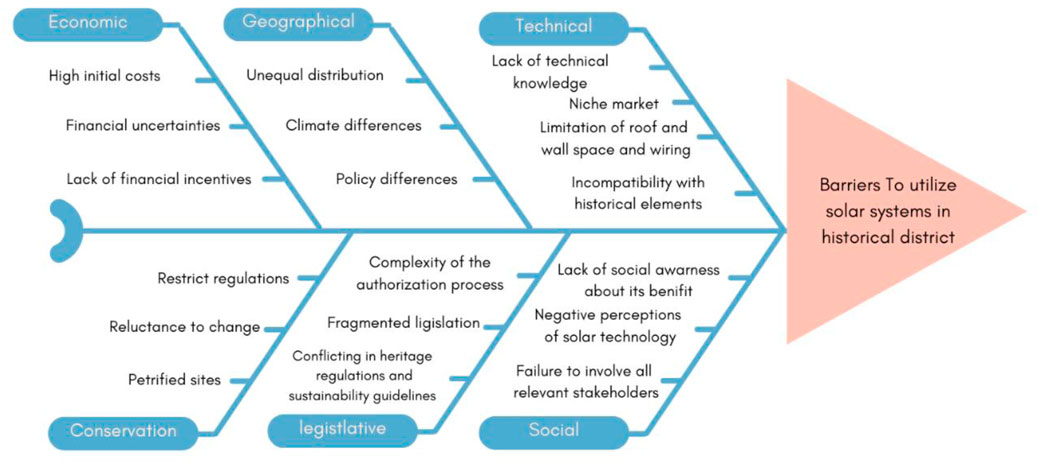
FIGURE 7. Fishbone summarizing diagram of barriers to utilize solar system in high sensitive built environment and their main roots.
In the following, the identified conservation criteria (Figure 6) that appeared from the challenges of aligning conservation with sustainability, and the relationship between those criteria with the barriers are discussed. The barriers previously discussed and reported in Table 1 obstacles today but can be seen as an opening to opportunities from now to the future. The conservation criteria identified here as challenges, can be used as part of a methodology to assess how good practice may give rise to new implementation projects as they help to identify ways to overcome the existing barriers. From the results found in the literature, each conservation criterion is linked to at least one of the barriers. Furthermore, the criteria themselves are interlinked as illustrated in the chord diagram in Figure 8 as a connection map.
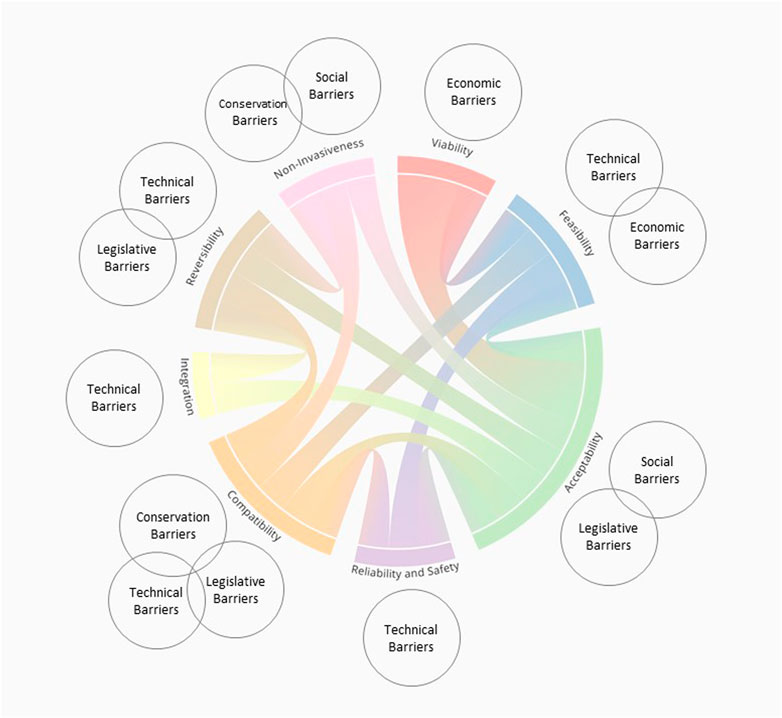
FIGURE 8. Chord diagram generated with Datasmith.org exposing connection map and interconnections between challenges (conservation criteria) and barriers (black and white circles). In this concept map, the eight conservation areas are represented as the first layer and barriers are shown as the second layer. The colorful arrows represent the relationship between the different challenges and barriers, showing how they are interconnected and affect each other. The width of each chord represents the strength of the relationship between the connected data points. This concept map can provide a helpful visual representation of the challenges, barriers, related to utilizing solar energy, and how different solutions and approaches can help in overcoming them.
From this analysis emerges that all the conservation criteria are pointing to acceptability. Even if some criteria are induced by legislative or conservative requirements, they have an impact on the overall acceptability of the project. As an example, increasing acceptability can help to overcome social and legislative barriers, and should be the primary focus of any BIPV implementation project on historic buildings as crucial step in breaking down barriers and facing other conservation criteria challenges.
4.4 Opportunities
The historical buildings and high-sensitive areas can be seen as opportunities rather than challenges for Solar System implementation. Installing solar energy in historical districts will not only be a tool to protect and revitalize high-sensitive sites, but also to add a new value system in the built environment towards sustainability and preserving their values.
4.4.1 Ways to protect fragile historic built environment
As discussed previously, BIPV systems provide further functions than producing electricity, as do “conventional” PV. Indeed, coloured BIPVs, PV tiles or WIPV also protect the existing building’s envelope against the natural exterior environment while enhancing the possibility to keep it in use. As historic buildings often need refurbishment to come back to their initial condition or retrofitting to improve the overall performances of the building, replacing defecting, or weathered existing structures with new BIPV systems will allow to improve the protection of historic buildings, reducing its obsolescence, increasing its service life thus reducing CO2 emissions. Furthermore, retrofitted historic buildings that might have been inappropriate for usage as well as historic districts at a “petrified” stage can take advantage from this type of intervention as it will attract new residents or businesses and will eventually allow districts revitalization.
4.4.2 Added values to historic centres
BIPVs being multifunctional in a way that it respects the aesthetic integrity of a place while producing clean energy is an asset for the built environment and brings a new value to it. Indeed, it allows to see the built environment as a resource where consumers can become prosumers. In Norway, contrasting to general inaccurate ideas concerning low solar potential in Nordic countries, studies have shown that PV performances align with European results if applied to vertical buildings envelopes (Imenes, 2016). To diversify sources of energy for security reasons and to meet future energy demands that are increasing, solar energy may assert its place among the Norwegian energy mix.
4.4.3 Fulfil climate goals to mitigate climate change
More than bringing security on energy production, BIPVs are classified among “clean” or “green” energy sources which may bring cities closer towards sustainability -on all three aspects: economic, social, and environmental- and align with their emissions reduction goals. Keeping that in mind, there is a potential for historic districts to contribute significantly to the elaboration of smart cities (Borda and Bowen, 2017). For that to occur, the consideration of conservation criteria should represent a compulsory step for any planning or implementation project. The respect of intrinsic value may bring citizens at the centre point of urban planning processes and allow a human-centred approach. The PPP-Partnership may enhance social acceptance and co-ownership project which allow the inclusion of a wider number of citizens and can motivate them to consider solar systems on their roof. Indeed, what López, 2020 calls the “revolution of solar architecture” (López, 2020) may materialize through BIPV technologies in the built environment, while including historic as well as heritage buildings.
5 Conclusion and further developments
The use of solar technology in historical buildings and high-sensitive areas presents a range of challenges and barriers that must be addressed to ensure their successful implementation in the built environments. The criteria of viability, feasibility, integration, reversibility, compatibility, reliability and safety, non-invasiveness, and acceptability are important factors to consider when evaluating the suitability of solar technology for a particular building or neighborhoods in a city center. In addition to these technical challenges, there are also various economic, geographical, technical, conservation, and legislative barriers that must be overcome to effectively use solar technology in historic buildings. The first critical analysis of this paper through the list of challenges and barriers found in the literature is counter-balanced by an opening to opportunities and solutions of solar energy implementation in historic districts and buildings. Challenges resulting from a compromise between conservation and sustainability values have become possible to assess through the elaboration of conservation criteria. Identified barriers are the following:
(1) Economic barriers: lack of financial incentives, high initial costs, high financial risks etc.
(2) Geographic barriers: unequal geographical distribution of exemplary implementation projects, climate differences, policy differences between countries etc.
(3) Technical barriers: recent technologies implying a lack of training for experts, lack of feedback and data, low market penetration and case-by-case studies resulting in fragmented techniques etc.
(4) Conservation barriers: restrictive, and prohibitive regulations, reluctance to change, both aspects leading to the risk of petrified sites etc.
(5) Legislative barriers: complexity of the authorization process due to fragmented legislation and lack of clear comprehensive guidelines etc.
(6) Social barriers: lack of knowledge and awareness about technical and legislative aspects, diversity of stakeholders that need to work on an inclusive common ground, lack of citizen participation and social acceptance etc.
BIPV has higher potential to solve part of these barriers and therefore it is a suitable technology to be implemented in historic and high-sensitive districts. Especially coloured BIPVs, PV tiles or WIPV are already opening opportunities of protecting and revitalizing historic cities centres or cultural landscapes while adding a new value to the built environment through the production of clean electricity. Eventually, the implementation of solar energy in historic centers also allows cities to align with their sustainability goals thus contributing to mitigate climate change. The Nordic countries have been under consideration until now in studies focusing on BIPV implementation in historic districts. Notwithstanding the annual solar potential and the forecasted growth in the electrification sector allows us to acknowledge BIPV as a promising source of additional energy. In Norway, the use of solar technology in historical buildings presents similar challenges and barriers as in other countries. However, Norway has a strong commitment to sustainability and the deployment of RES, and this is reflected in its legislative framework and cultural attitudes. Based on the earlier discussion, the Norwegian government has set ambitious targets for reducing GHG emissions and increasing more the use of RES. This has created a favorable environment for the implementation of solar technology in historic buildings, if it is done in a way that is compatible with the conservation and preservation of important cultural heritage sites. In addition, Norway has a well-established system for the preservation and protection of historical buildings, which includes guidelines for the use of modern technologies in these structures. This helps to ensure that the integration of solar technology is done in a way that is consistent with national and local cultural values and heritage. Therefore, while the challenges and barriers to deploy solar technology in historic buildings, in Norway are like those faced elsewhere, the country’s commitment to sustainability and protection of cultural heritage may provide a framework for more effective implementation of these technologies. This framework can use the result and some aspects like social acceptance, feasibility, and compatibility as main criteria to overcome barriers. From this review, the following areas of research could be further developed:
(i) Solutions to economic barriers:
o More subsidies from the state and financial help from grid companies.
o Co-investment and co-ownership.
o More stability and clearness in feed-in tariffs from DSOs.
o Further guarantees from third-party companies to overcome risk uncertainties.
o Reliable and user-friendly tools to simulate investment, pay-back period, and income for the users/owners.
(ii) Solutions to technical barriers:
o Centralization of feedback and data from existing case studies on historic sites.
o Specific training for professionals, stakeholders, and public administration entities towards BIPV application on historic buildings.
o Serie production of BIPV technologies that comply with most represented historic building typologies.
o Further engagement and improvement from distribution system operators to support prosumers in fragile areas.
o Enhancing R&D to improve the performance of BIPVs especially driving conservation criteria (e.g., on mountable/unmountable BIPV frameworks).
(iii) Solutions to conservation and legislative barriers:
o Provide clear and explicit guidelines that consider regulation at all levels (e.g., international, European, national, local) and promote retrofitting intervention by showing best case final aesthetics possibilities.
o Provide flexibility of the law to allow case-by-case adaptability.
o Improve international incentives.
o Municipalities should provide access to archive data on the history of local buildings and their transformations (if they possess such information).
(iv) Solutions to socio-cultural barriers:
o Enhance citizen participation and spread awareness through providing digital tools, which will at the same time erase false ideas about Solar System aesthetics and diminish the fear of change.
o Include all concerned actors through an interactive platform following a Public-Private-People Partnership (PPPP): a partnership between all three sectors allow to consider financial models of co-ownership or co-investment to break down the high initial costs, facilitate information exchange between stakeholders (especially involving “people” who are often excluded) and reduce risks through the involvement of the public sector by providing policies and guarantees.
o Measurement and consideration of intrinsic value as well as tangible and intangible cultural heritage significance of a place before any implementation.
o Enhance communication about existing case studies as well as promote and increase the number of lighthouses cities.
o Show scenarios of re-use/continuous use of historic buildings in the district to highlight the potential of revitalization and requalification of the district to PPP.
Author contributions
TA: Conceptualization, Methodology, Writing–original draft, Visualization. EM: Data Curation, Writing–original draft. CB: Supervision, Writing–review and editing. GL: Supervision, Writing–review and editing. AS: Supervision.
Funding
The author(s) declare financial support was received for the research, authorship, and/or publication of this article. The authors acknowledge the financial support from the Norwegian Research Council (research project FRIPRO-FRINATEK no. 324243 HELIOS).
Conflict of interest
The authors declare that the research was conducted in the absence of any commercial or financial relationships that could be construed as a potential conflict of interest.
Publisher’s note
All claims expressed in this article are solely those of the authors and do not necessarily represent those of their affiliated organizations, or those of the publisher, the editors and the reviewers. Any product that may be evaluated in this article, or claim that may be made by its manufacturer, is not guaranteed or endorsed by the publisher.
Abbreviations
BAPV, Building applied/attached Photovoltaics; BIPV, Building Integrated Photovoltaics; DSOs, Grid Companies, De samarbeidende organisasjoner in Norwegian Language; EPBD, Energy Performance of Buildings Directive; EPD, Environmental Product Declaration; ETS, Emissions trading system; EU, Europe; FiT, Feed-in Tariff; FMUH, Facility Management in Urban Heritage; GHG, Greenhouse gas; LCOE, Levelized Costs of Electricity; MW, Megawatts; NDCs, Nationally Determined Contributions; NOK, Norwegian Krone; NVE, Norwegian Water Resources and Energy Directorate; nZEB, nearly Zero-Energy Buildings; PPPP, People, Public, Private, Partnership; PV, Photovoltaic; RES, Renewable Energy Sources; UNFCCC, United Nations Framework Convention on Climate Change.
References
Abdullah, D. Z., Shah, T., Jebran, K., Ali, S., Ali, A., Ali, A., et al. (2017). Acceptance and willingness to pay for solar home system: survey evidence from northern area of Pakistan. Energy Rep. 3, 54–60. doi:10.1016/j.egyr.2017.03.002
Arksey, H., and O’Malley, L. (2005). Scoping Studies: towards a methodological framework. Int. J. Soc. Res. Methodol. 8 (1), 19–32. doi:10.1080/1364557032000119616
Badawy, N. M., Hosam, S.El S., and Ahmed, A. E. W. (2022). Relevance of monocrystalline and thin-film technologies in implementing efficient grid-connected photovoltaic systems in historic buildings in port fouad city, Egypt. Alexandria Eng. J. 61 (12), 12229–12246. doi:10.1016/j.aej.2022.06.007
Bagaini, A., Colelli, F., Croci, E., and Molteni, T. (2020). Assessing the relevance of barriers to energy efficiency implementation in the building and transport sectors in eight European countries. Electr. J. 33 (8), 106820. doi:10.1016/j.tej.2020.106820
Basar Baypinar, M., Yasa, E., Ersoy, S., Beygo, C., and Beygo, K. (2022). “Solar Energy Simulations in Historical Districts for Retrofitting and Evidence-Based Decision Making: data challenges for low carbon cities in the eu neighbourhood & accession countries,” in Proceedings of the Real Corp 2020 Shaping Urban Change, Aachen, Germany, April, 2020.
Bipv, (2022). BIPV_History_1.Pdf. https://task59.iea-shc.org/Data/Sites/1/publications/BIPV_History_1.pdf (Accessed October 31, 2022).
Borda, A., and Bowen, J. P. (2017). “Smart cities and cultural heritage – a review of developments and future opportunities,” in Proceedings of the EVA London 2017, London, UK, July, 2017. doi:10.14236/ewic/EVA2017.2
Build Up, (2019). Overview energy efficiency in historic buildings: a state of the art. https://www.buildup.eu/en/news/overview-energy-efficiency-historic-buildings-state-art.
Building Stock, (2022). Building stock SSB. https://www.ssb.no/en/bygg-bolig-og-eiendom/bygg-og-anlegg/statistikk/bygningsmassen (Accessed October 31, 2022).
Centre UNESCO World Heritage (2022). Norway - UNESCO world heritage convention. https://whc.unesco.org/en/statesparties/no.
Cumo, F., Nardecchia, F., Agostinelli, S., and Rosa, F. (2022). Transforming a historic public office building in the centre of Rome into nZEB: limits and potentials. Energies 15 (3), 697. doi:10.3390/en15030697
De Medici, , and Stefania, (2021). Italian architectural heritage and photovoltaic systems. Matching style with sustainability. Sustainability 13 (4), 2108. doi:10.3390/su13042108
Durante, A., Lucchi, E., and Maturi, L. (2021). Building integrated photovoltaic in heritage contexts award: an overview of best practices in Italy and Switzerland. IOP Conf. Ser. Earth Environ. Sci. 863 (1), 012018. doi:10.1088/1755-1315/863/1/012018
Elena, C., Mariella, F., and Fabio, F. (2017). “Energy resilience of historical urban districts: a state of art review towards a new approach,” in Proceedings of the Energy Procedia, 8th International Conference on Sustainability in Energy and Buildings, Turin, Italy, September 2016, 426–434. doi:10.1016/j.egypro.2017.03.204
Energy Performance of Buildings Directive, (2022). Energy performance of buildings directive. https://energy.ec.europa.eu/topics/energy-efficiency/energy-efficient-buildings/energy-performance-buildings-directive_en.
European Climate Law (2023). European climate law. https://climate.ec.europa.eu/eu-action/european-green-deal/european-climate-law_en (Accessed January 19, 2023).
Formolli, M., Croce, S., Vettorato, D., Paparella, R., Scognamiglio, A., Giovanni Mainini, A., et al. (2022). Solar Energy in Urban Planning: lesson learned and recommendations from six Italian case studies. Appl. Sci. 12 (6), 2950. doi:10.3390/app12062950
Gholami, H., Harald, N. R., Kumar, N. M., and Shauhrat, S. (2020). Lifecycle cost analysis (LCCA) of tailor-made building integrated photovoltaics (BIPV) façade: solsmaragden case study in Norway. Sol. Energy 211, 488–502. doi:10.1016/j.solener.2020.09.087
Global Lcoe and Auction Values, (2022). Global LCOE and auction values. https://www.irena.org/Data/View-data-by-topic/Costs/Global-LCOE-and-Auction-values (Accessed December 7, 2022).
Guttormsen, T. S., and Fageraas, K. (2011). The social production of “attractive authenticity” at the world heritage site of røros, Norway. Int. J. Herit. Stud. 17 (5), 442–462. doi:10.1080/13527258.2011.571270
Horizon, (2022). Horizon 2020. https://research-and-innovation.ec.europa.eu/funding/funding-opportunities/funding-programmes-and-open-calls/horizon-2020_en.
Hyslop, E. (2023). “Interdisciplinarity in Heritage Conservation: intersections with climate change and sustainability,” in Evolving heritage conservation practice in the 21st century (Singapore: Springer Nature Singapore). doi:10.1007/978-981-99-2123-2_11
Ibrahim, H. S. S., Khan, A. Z., Yehya, S., and Attia, S. (2021). Towards nearly-zero energy in heritage residential buildings retrofitting in hot, dry climates. Sustainability 13 (24), 13934. doi:10.3390/su132413934
Imenes, A. G. (2016). “Performance of BIPV and BAPV installations in Norway,” in Proceedings of the 2016 IEEE 43rd Photovoltaic Specialists Conference (PVSC), Portland, OR, USA, June 2016. doi:10.1109/PVSC.2016.7750246
Irati, ARTOLA, and Trinomics, (2016). Boosting Building Renovation: what potential and value for europe? https://www.europarl.europa.eu/RegData/etudes/STUD/2016/587326/IPOL_STU(2016)587326_EN.pdf.
Jackson, I., Håkon, T., Hanne, S., Westskog, H., and Winther, T. (2020). The Dynamics of Solar Prosuming: exploring interconnections between actor groups in Norway. Energy Res. Soc. Sci. 70, 101816. doi:10.1016/j.erss.2020.101816
Kalliopi, F., Dreyfuss, G., Kelvin, K. E. A., and May, C. (2022). “Introduction,” in Routledge handbook of sustainable heritage. 1st ed. (London, UK: Routledge). doi:10.4324/9781003038955-1
Kandt, A., Hotchkiss, E., Walker, A., Buddenborg, J., and Lindberg, J. (2011). Implementing solar PV projects on historic buildings and in historic districts. https://www.nrel.gov/docs/fy11osti/51297.pdf.
Kristl, Ž., Alenka, T. S., and Roumboutsos, A. (2020). Sustainability and universal design aspects in heritage building refurbishment. Facilities 38 (9/10), 599–623. doi:10.1108/F-07-2018-0081
Leonardi, (2022). Leonardi - conservation compatible energy retrofit Technologi.Pdf. https://task59.iea-shc.org/Data/Sites/1/publications/D.C1--Part-V-Solar.pdf (Accessed November 7, 2022).
Light Coatings, (2022). Light coatings - thin film consultancy. https://www.lightcoatings.co.uk/ (Accessed October 20, 2022).
Loli, A., and Bertolin, C. (2018). Towards zero-emission refurbishment of historic buildings: a literature review. Buildings 8 (2), 22. doi:10.3390/buildings8020022
López, C. S. P., and Frontini, F. (2014). Energy efficiency and renewable solar energy integration in heritage historic buildings. Energy Procedia 48, 1493–1502. doi:10.1016/j.egypro.2014.02.169
López, P. (2020). Acceptance of building integrated photovoltaic (bipv) in heritage buildings and landscapes: potentials. Barrier Assess. Criteria 8, 1636–1644.
López, P., Cristina, S., Lucchi, E., Leonardi, E., Durante, A., Schmidt, A., et al. (2021a). Risk-benefit assessment Scheme for renewable solar solutions in traditional and historic buildings. Sustainability 13 (9), 5246. doi:10.3390/su13095246
López, P., Cristina, S., Troia, F., and Nocera, F. (2021b). Photovoltaic BIPV Systems and Architectural Heritage: new balance between conservation and transformation. an assessment method for heritage values compatibility and energy benefits of interventions. Sustainability 13 (9), 5107. doi:10.3390/su13095107
Lucchi, E., Dall’Orto, I., Peluchetti, A., Toledo, L., Pelle, M., Cristina, P. L., et al. (2022). Photovoltaic technologies in historic buildings and protected areas: comprehensive legislative framework in Italy and Switzerland. Energy Policy 161, 112772. doi:10.1016/j.enpol.2021.112772
Lucchi, E., Polo Lopez, C. S., and Franco, G. (2020). A conceptual framework on the integration of solar energy systems in heritage sites and buildings. IOP Conf. Ser. Mater. Sci. Eng. 949 (1), 012113. doi:10.1088/1757-899X/949/1/012113
Meraz, F. (2019). Cesare Brandi (1906 to 1988): his concept of restoration and the dilemma of architecture. https://www.iccrom.org/sites/default/files/publications/2020-05/conversaciones_07_05_meraz_eng.pdf.
Ministry of Climate and Environment (2020). Norwegian Ministry of climate and environment. Meld. St. 13 (2020–2021) Report to the Storting (white paper), Norway’s Climate Action Plan for 2021–2030. https://www.regjeringen.no.
Moschella, A., Salemi, A., Lo Faro, A., Sanfilippo, G., Detommaso, M., and Privitera, A. (2013). Historic buildings in mediterranean area and solar thermal technologies: architectural integration vs preservation criteria. Energy Procedia 42, 416–425. doi:10.1016/j.egypro.2013.11.042
Nordahl, S. H. (2022). Design of roof PV installation in Oslo. https://ntnuopen.ntnu.no/ntnu-xmlui/bitstream/handle/11250/257364/566421_FULLTEXT01.pdf.
Nowtricity, (2022). CO2 Emissions per kWh in Norway - nowtricity. https://www.nowtricity.com/country/norway/ (Accessed November 1, 2022).
Our World in Data, (2022). Levelized cost of energy by technology. https://ourworldindata.org/grapher/levelized-cost-of-energy (Accessed October 19, 2022).
Pelle, M., Lucchi, E., Maturi, L., Alexander, A., and Causone, F. (2020). Coloured BIPV Technologies: methodological and experimental assessment for architecturally sensitive areas. Energies 13 (17), 4506. doi:10.3390/en13174506
Power, A. (2008). Does demolition or refurbishment of old and inefficient homes help to increase our environmental, social and economic viability?’ Energy policy. Foresight Sustain. Energy Manag. Built Environ. Proj. 36 (12), 4487–4501. doi:10.1016/j.enpol.2008.09.022
Prabowo, B. N., Alenka, T. S., and Lohne, J. (2021). Urban heritage facility management: a scoping review. Appl. Sci. 11 (20), 9443. doi:10.3390/app11209443
Pv Magazine, (2023). The Weekend Read: historical and landmarked buildings turn to solar amid changing policies, rising prices. https://www.pv-magazine.com/2022/08/27/the-weekend-read-historical-and-landmarked-buildings-turn-to-solar-amid-changing-policies-rising-prices/ (Accessed January 9, 2023).
Pv Magazine International, (2022). Terracotta solar tiles for historical buildings. https://www.pv-magazine.com/2022/05/12/intersolar-europe-day-2-terracotta-solar-tiles-to-unlock-historical-buildings/ (Accessed October 20, 2022).
Regjeringen, (2013). Environment, Ministry of the. 2013. ‘Department for cultural environment. https://www.regjeringen.no/en/dep/kld/organisation/departments/department-for-cultural-environment/id1207/.
Roosmalen, M. V., and Gritzki, A. (2020). Opportunities and challenges for the use of photovoltaics in building monuments – case study TU dresden. IOP Conf. Ser. Earth Environ. Sci. 588, 022037. doi:10.1088/1755-1315/588/2/022037
Rosa, F. (2020). Building-integrated photovoltaics (BIPV) in historical buildings: opportunities and constraints. Energies 13 (14), 3628. doi:10.3390/en13143628
Røstvik, H. N. (2013). Listed church buildings and solar energy. J. Archit. Conservation 19 (1), 49–67. doi:10.1080/13556207.2013.787019
Roszczynska-Kurasinska, M., Domaradzka, A., Anna, W., and Oleksy, T. (2021). Intrinsic value and perceived essentialism of culture heritage sites as tools for planning interventions. Sustainability 13 (9), 5078. doi:10.3390/su13095078
Sánchez-Pantoja, N., Rosario, V., and Carmen Pastor, M. (2021). EU-funded projects with actual implementation of renewable energies in cities. Analysis of their concern for aesthetic impact. Energies 14 (6), 1627. doi:10.3390/en14061627
Senior, C., Alenka, T. S., Vukmirovic, M., Jowkar, M., and Kristl, Ž. (2021). The spirit of time—the art of self-renovation to improve. Indoor Environ. Cult. Herit. Buildings’ 27. doi:10.3390/en14134056
Sesana, E., Bertolin, C., Gagnon, A., and Hughes, J. (2019). Mitigating climate change in the cultural built heritage sector. Climate 7 (7), 90. doi:10.3390/cli7070090
Shuldan, L., and Al-Akhmmadi, S. (2021). Photovoltaic systems integration rules and restrictions in the historic buildings architecture. IOP Conf. Ser. Mater. Sci. Eng. 1203 (2), 022119. doi:10.1088/1757-899X/1203/2/022119
Sommerfeldt, N., and Madani, H. (2017). Revisiting the techno-economic analysis process for building-mounted, grid-connected solar photovoltaic systems: part one – review. Renew. Sustain. Energy Rev. 74, 1379–1393. doi:10.1016/j.rser.2016.11.232
Statnett, (2022). Lma-Update-2021.Pdf. https://www.statnett.no/globalassets/for-aktorer-i-kraftsystemet/planer-og-analyser/lma/lma-update-2021.pdf (Accessed November 30, 2022).
Sudimac, B., Ugrinović, A., and Jurčević, M. (2020). The application of photovoltaic systems in sacred buildings for the purpose of electric power production: the case study of the cathedral of st. michael the archangel in belgrade. Sustainability 12 (4), 1408. doi:10.3390/su12041408
Thebault, M., Clivillé, V., Berrah, L., and Desthieux, G. (2020). Multicriteria roof sorting for the integration of photovoltaic systems in urban environments. Sustain. Cities Soc. 60, 102259. doi:10.1016/j.scs.2020.102259
Tsoumanis, G., Formiga, J., Bilo, N., Tsarchopoulos, P., Ioannidis, D., and Tzovaras, D. (2021). The Smart Evolution of Historical Cities: integrated innovative solutions supporting the energy transition while respecting cultural heritage. Sustainability 13 (16), 9358. doi:10.3390/su13169358
Tzetzi, I. (2019). Photovoltaic and Heritage: towards the energy transition of the building environment. https://repository.tudelft.nl/islandora/search/?collection=education.
Unfccc, (2023a). The Paris Agreement UNFCCC. https://unfccc.int/process-and-meetings/the-paris-agreement/the-paris-agreement (Accessed January 19, 2023).
Unfccc, (2023b). What is the Kyoto Protocol? UNFCCC. https://unfccc.int/kyoto_protocol (Accessed January 19, 2023).
United Nations Framework Convention on Climate Change, (2022). United Nations framework convention on climate change. https://unfccc.int/.
Valancius, R., Mutiari, A., Singh, A., Alexander, C., Diana Arteta De La Cruz, , and Pozo, F. E. D. (2018). Solar photovoltaic systems in the built environment: today trends and future challenges. J. Sustain. Archit. Civ. Eng. 23 (2), 25–38. doi:10.5755/j01.sace.23.2.21268
Webb, A. L. (2017). Energy retrofits in historic and traditional buildings: a review of problems and methods. Renew. Sustain. Energy Rev. 77, 748–759. doi:10.1016/j.rser.2017.01.145
Keywords: challenges, barriers, photovoltaic, BIPV, historic, heritage, Norway, solar
Citation: Akbarinejad T, Machlein E, Bertolin C, Lobaccaro G and Salaj AT (2023) Enhancing the deployment of solar energy in Norwegian high-sensitive built environments: challenges and barriers—a scoping review. Front. Built Environ. 9:1285127. doi: 10.3389/fbuil.2023.1285127
Received: 29 August 2023; Accepted: 16 October 2023;
Published: 26 October 2023.
Edited by:
Claudio Del Pero, Polytechnic University of Milan, ItalyReviewed by:
Daniel Tudor Cotfas, Transilvania University of Brașov, RomaniaHasim Altan, Prince Mohammad bin Fahd University, Saudi Arabia
Copyright © 2023 Akbarinejad, Machlein, Bertolin, Lobaccaro and Salaj. This is an open-access article distributed under the terms of the Creative Commons Attribution License (CC BY). The use, distribution or reproduction in other forums is permitted, provided the original author(s) and the copyright owner(s) are credited and that the original publication in this journal is cited, in accordance with accepted academic practice. No use, distribution or reproduction is permitted which does not comply with these terms.
*Correspondence: Tahmineh Akbarinejad, dGFobWluZWguYWtiYXJpbmVqYWRAbnRudS5ubw==