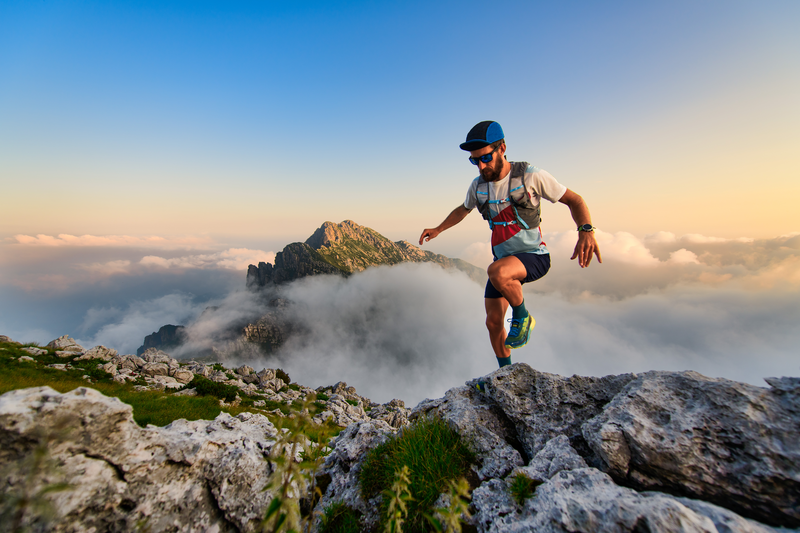
94% of researchers rate our articles as excellent or good
Learn more about the work of our research integrity team to safeguard the quality of each article we publish.
Find out more
ORIGINAL RESEARCH article
Front. Built Environ. , 22 November 2023
Sec. Construction Materials
Volume 9 - 2023 | https://doi.org/10.3389/fbuil.2023.1228321
This article is part of the Research Topic Sustainable and Eco-friendly Building Materials, Volume II View all 3 articles
As the risk of lung cancer increases with increasing radon exposure, the preferred measure of this risk is the long-term average radon level. The assessment of the radiation risk in workplaces in Egypt is constrained by the lack of experimental data about the measurements of natural radioactivity emitted from some common building materials and radon concentration within the buildings. The natural radioactive Ra-226, Th-232 series, and K-40 were computed in (Bq/kg) by a pure hyperactive germanium detector based on gamma spectroscopy to determine the potential health risk from exposure to gamma radiation induced by commonly used construction materials in Egypt such as ceramics and granite materials. The spatial variations of radon concentrations within two buildings, in over 50 rooms, made from two different types of building materials were surveyed using nuclear detectors (CR-39) to assess radon concentration for two successive 6 months. To analyze the relationship through radon concentration and floor levels for the use of ceramic tile improved construction materials, tier average ratios respecting the ground floor as a reference-grade consideration were also computed. All the findings of this research were analyzed and contrasted with the traditional ceramic construction material and it was clear that the “ceramics tile construction building” is a hygienic workplace which may be attributed to the use of improved construction materials and radiation shielding.
Some applications of ionizing radiation are useful such as fabrications of radiation dosimetric materials (Abdel Ghaffar et al., 2009; Afifi et al., 2022), but sometimes exposure to naturally occurring ionizing radiation resulting from radionuclides is unavoidable for humans. The world population receives more than 85% of its radiation dose from natural sources, owing primarily to the Uranium (238U) and Thorium (232Th) decay series radionuclides, as well as the single occurring 40K radionuclides, which all disintegrate continuously in the housing environment (Solehah and Samat, 2018; Aladeniyi et al., 2021). Based on the soil composition, the radionuclide varied up to four times between countries and locations (Goldstein et al., 1999). Various amounts of natural radioactive nuclides are present in all building materials (Fares, 2019; Venoso et al., 2020). Ceramics are one of the most widely used building (decorative) materials. The earth’s crust is replete with constituents ordinarily utilized in chemical manufacturing that include a high concentration of naturally occurring radionuclides (Lumpkin et al., 2014). In numerous nations, surveys of the natural radioactive content of construction materials have been widely debated (Mahat and Amin, 1990; Deng et al., 1997; Hobbs, 2000; Stajic and Nikezic, 2015).
In practice, many radiological issues arise when natural constituents are used as construction materials (Haridasan et al., 2015). Glass and ceramic goods are made from soils bearing naturally existing radioactive materials which including UO2, ThO2, and ZrO2 (Deng et al., 1997). Clay, silica, feldspars, talc, and kaolin are used to create ordinary ceramics. Due to the lanthanum series’ shine quality, zircon sand (ZrSiO4) is supplemented with the components utilized in the manufacture of glaze. Uranium is easier to penetrate and trap in the zircon crystal lattice (Jensen, 1952). To prohibit the constituents of ceramics from absorbing liquids, they are coated with a glassy coating called the glaze, which makes them smoother and easier to clean. In an area bordered with glazed ceramic tile, the overall radiation level was higher than in other ceramic components (Hofmann et al., 2000). Because employees are exposed to naturally existing radioactive compounds such as uranium and thorium, that can be found in ceramics’ raw ingredients, the creation of many types of ceramic tiles poses a possible radiation risk (Krewski et al., 2019). Natural radionuclide concentrations in building materials of the U-238, Th-232 series, and K-40 radioactive isotopes are critical for assessing radioactive pollution in public spaces. The existence of diverse physical-chemical formulations of stable components and radionuclides at the ceramic fraction supplies governs the leaching of radioactive and stable elements from ceramic components (Testa et al., 1999; Morabito, 2004). Achievement of radiation dosimetry for bioenvironmental and other application purposes has occurred with the introduction of various new detectors, which their applications depend on the qualities of radiation dosimetry they exhibit (Diab and El-Mallawany, 2014; Abd-Elghany et al., 2020; Diab et al., 2020). Radiological monitoring in the environment has been carried out through different researches to assess the radiogenic risk from radiation exposure to natural radioactivity (Abo-Elmagd and Daif, 2010; Diab, 2017; Diab, 2019; Badran, 2018; Salim and Ebrahiem, 2019).
Above all, taking into consideration the wisdom that says prevention is better than cure, we must highlight technological advancements in the field of radiation protection, such as the development of sustainable and cost-effective materials for use in the construction of radiation shields (Reda and Saleh, 2021; Saleh et al., 2021; Saleh et al., 2022), the development of environmentally friendly methods for treating radioactive waste (Dawoud et al., 2022; Abdelhamid et al., 2023) before stabilization (Saleh and Eskander, 2020; Saleh et al., 2020) and the improving the characterization of cement used in radioactive waste immobilization by including natural clay or polymer (Saleh, 2014; Eskander et al., 2021), glass (Ehab et al., 2022; Eid et al., 2022; Esawii et al., 2022) or packaging of radioactive waste to attain human-safe and clean environment (Saleh and Koller, 2021).
From the radiation protection point of view, doses above 1.0 mSv-1 should not be exceeded and controls should be based on a dose range (0.3–1.0 mSv−1). Individual doses greater than 1.0 mSv−1 should be avoided (Hofmann et al., 2000). This study examined the inherent radioactivity of granite and other raw ceramic materials, as well as the final product (ceramic tile), and how to limit exposure for building occupants and workers.
More than 50% of human exposure comes from natural sources, owing to Radon gas (222Ra) and its descendants. It is produced in rocks and soil by the alpha decay of 226Ra, and as a result, it is found throughout the Earth’s crust, though in fluctuating amounts depending on geology. Radon gas, which is responsible for up to 14% of lung cancer incidence, can enter homes through cracks in walls when it is released from the earth, where it can accumulate to dangerous levels. It can reach the bronchial tissue during the breathing process and decay there, generating radioactive alpha particles capable of harming the pulmonary epithelium and triggering lung cancer (Quarto et al., 2015). The correlation and relationship between indoor radon and lung cancer have already been identified in several countries through epidemiological studies (Darby et al., 2005; Lubin et al., 2005; Krewski et al., 2006). These studies reveal that residential radon poses a significant lung cancer risk at a mean radon concentration of 104 Bq/m3, particularly for smokers and recent ex-smokers, and that is responsible for around 2% of all cancer deaths in Europe. Lung cancer risk rises 16 percent for every 100 Bq/m3 increase in long-term average radon concentration. Quarto et al., 2015 estimated the annual effective dose is 8.2 mSv per annum with a probability of cancer risk of 148 per million inhabitants (Darby et al., 2005). Important studies (Antignani et al., 2009) present various locative variability radon level sources and these differences produce uncertainties that could have a massive influence on evaluating the dose a public receives from radon. Endogenous spatial variations in 243 homes were observed in 1-year radon level measurements (Steck, 1992). Although the task is important and affects severely the human life, limited studies are published in Egypt regarding radiation exposure from some building materials including granites (Fares, 2019). In this work, an inter-comparative study of radioactivity measurements between ceramics and granite, which are considered among the most important building materials used in workplaces, to reduce the risks of radon to achieve healthy buildings.
This study was conducted in Egypt in 2020. The studied building was constructed from advanced granite with a thickness of 2.0 cm and a surface area of 280 cm2. This granite involved petroleum compounds (benzoic acid) and Egyptian ceramics with thickness 3 cm and surface area of 380 cm2. In the ceramic floor, the raw materials consist of a blend of clay, feldspar, silica, zirconia silica (ZrSiO4), and talc kaolin.
The granite is formed from feldspar, quartz, and biotite. The percentage composition of feldspar ranged from 65% to 90%, while quartz could range between 10% and 60% and biotite from 10% to 15%, along with benzoic acid. Granite is a magmatic igneous rock.
Five raw ceramic ingredients including zirconium silicates, clay, feldspar, kaolin, and talc minerals are utilized in the production of ordinary local ceramics. The glaze paste, which was produced from the minerals mentioned above, was used as the sixth sample. The final product (ceramic tile) was used as the seventh sample.
A p-type co-axial gamma spectroscopic detector with a high resolution and crystal with high-purity germanium (HPGe) used in conjunction with a multi-channel analyzer (Tennelec company, United States of America) was utilized to measure radioactivity. The samples were crushed to produce homogenious powder of average particle size. To ensure that all samples have the same volume and geometry, plastic gars (300 mL) were used. Each jar was perfectly sealed after being filled with crushed materials and left for an exposure time of 4 weeks to allow Ra-226 and Th-232 and their progeny to attain equilibrium. The radiation level and background activity of each sample were assessed.
Natural radioactive series of Ra-226 and Th-232, besides K-40 radioactive isotope concentration levels, have been determined. Isotope which emits resolute peaks of high intensity has been selected among natural radioactive chains. The Ra-226 series was supposed to be represented by Bi-214 activity at 609 keV. The Th-232 series was supposed to be represented by Ac-228 activity at (911 keV). There is only one distinct peak in K-40 (1,460 keV).
Introducing the levels of Ra-226, Th-232, and K-40 activity in a single quantity, which considered the radiation harmful effect related to each individual component, a common radiological index is called the radium equivalent activity (Raeq) and is represented by (UNSCEAR, 2000).
Where ARa, ATh and AK are the activity concentrations of Ra-226, Th-232 and K-40 respectively (Ahmad et al., 2006).
Eq. 2 was employed to determine the activity of radon gas (Alrowaili, 2023).
Where ρ is the density of the measured alpha track, illustrated in unit Track cm−2, and Te is the exposure time, which is related with the actual exposure time T, calculated in days by Eq. 3.
Where by λ (d−1) is the decay constant of Rn-222 and T is the total time exposure.
K is the calibration factor (T.cm−2. d−1/Bq.m−3), which is calculated using Eq. 4 (Amin and Hamdy, 2015).
Where, r = radius of the container in cm; θc = 35° is the critical angle of CR-39 and Rα = 4.15 cm, which is the range of particle α dispelled by the Rn-222.
The nuclear track detectors CR-39 mounted in a conductive holder were used for radon measurements. The etching process by NaOH solution which has a normality of 6.25 N at a temperature equal to 93°C of the detector required about 4.5 h, then the tracks were computed through the uses of an automated image analyzer (type Radometer 2000; by a company of Radosys, made in Hungary). Each measurement was tested for the reproducibility of track counting by counting a reference detector’s tracks. The mean of two pairs of radon measurements every 6 months was recorded for each room. The average time-weighted radon concentration was measured using an exposure time dosimeter (in days) as weights to achieve annual values from two consecutive 6-month periods (Antignani et al., 2009). A single annual average concentration of radon will therefore be collected and used for each monitoring room for this study.
Evaluation of radon measurement variation between floors represented by a unique radon value. Median, mean, and standard deviation are computed. In particular, as a monitor of the relative radon concentration variance between floors, the coefficient of variation (CV) was measured. Two different aspects of the evaluation of radon variability within the building were studied; variation among different floors monitored by recording a single radon measurement per each floor (computed as average measurements estimated per each floor) and variation across the same floor (i.e., variation from room to room). Ratios of the coefficient of variation among tier means and tier mean ratios, considering ground tier as a reference level, SD, and the tier average have been measured to determine tier-to-tier variations. Standard deviation and variation coefficient of radon density grades were measured for each tier to quantify floor variance with more than one monitored room (Antignani et al., 2009).
The radionuclides in granite and Egyptian ceramic have been identified by Steck (Steck, 1992) as shown in Table 1.
TABLE 1. Shows the particular radioactivity in Bq/kg of the samples manufactured from ceramic floor tile and granite (Steck, 1992).
The measurements were performed in 50 monitored rooms in a new building in Giza, Egypt. Long-term (CR-39) measurements are the most reliable method for measuring long-term average radon levels in schools, and should be used in deciding the need for mitigation. This study takes the duplicate measurements into account to obtained quality assurance which is an essential part of any radon measurements according to the EPA (EPA, 1993). The duplicate measurements indicated that the present data is highly precise.
Table 2, presents the mean, standard deviation, and range of the Radon concentrations at different floor levels. Values of radon concentration provide a large range of variations up to 10 times in the basement or ground floors at elevated levels (170 Bq m−3). Concentration parameters are distributed and displayed in Table 2.
Referring to a previous study published by Al-Jarallah, who provided measurements of indoor radon concentrations in old building material formations in the Kingdom of Saudi Arabia (KSA) (Al-Jarallah, 2001). The mean value of airborne radon concentration was calculated as 301 Bq/m3 on the building’s ground floor whereas, in the basement of the building, it had reached 587 Bq/m3. Out of 54 radon levels measured on the basement floor, eight concentrations reached more than 300 Bq/m3 (acceptable radon level) and were observed to be above 400 Bq/m3 in three cases (Åkerblom, 1999). The mean arithmetical radon concentration was 239.7 kBq/m3 in the non-earthen basement and 326.5 kBq/m3 in the ground. The mean value of indoor concentration owing to the entire analyzed region was 469.4 Bq/m3, which is higher than the mean KSU value (280 Bq/m3) by a factor of 1.5.
As mentioned in Table 3, the data analyzed were not evenly distributed among floor levels.
To assess floor to floor variation in the building, average radon levels on one floor are used. A single room value is used to describe this floor for every floor of a room with a concentration of radon (Antignani et al., 2009). SD and CV distributions are listed as descriptive statistics in Table 4.
In the building under study, higher SD and CV are present where the level of the ground has a high concentration of radon relative to the other three levels of the floor. Ratios of the floor mean, using the ground floor as a reference level, have been determined to investigate the potential concentration pattern of radon gas by floor level and the mean ratios as shown in Table 5.
TABLE 5. Floor to ground mean ratios to determine the radon concentration pattern versus floor (the value does not exceed recommended levels (Goldstein et al., 1999; Krewski et al., 2006; Krewski et al., 2019).
At the basement level, the mean radon concentration values were noticed and considered as high value, which is expected considering that the ground is the principal source of indoor radon gas. Extensive implementation of air conditioning systems to minimize radon levels is important. Variations within the building: variation between rooms (within floor). For each floor, SD and CV were measured and summed up by floor level to determine variations in the concentration of radon because of locative heterogeneity between rooms across the same tile as shown in Table 6.
TABLE 6. Variations between rooms in radon concentration (in total and for each floor) for the new building.
At basement and ground levels the highest values of median SD and CV were observed. The entry of indoor radon and/or exit mechanisms are affected by the characteristics of different rooms and the different behavior of their inhabitants since these properties are related to the non-neglected variance. Besides, new building materials that act as natural sources of radon can affect variations in the floor’s radon levels, essentially for once on the ground floor and in rooms linked to various air exchange techniques.
A comparison of the mean values of radionuclide activity concentrations of some building materials with those obtained from other published data is given in Tables 7, 8. In these two tables, the comparison showed that there is a significant variation from one country to another in terms of radon concentration. As previously mentioned, the radon centration depends on the presence of radionuclide series in the soil and the floor level. Therefore, the precise selection of building materials is necessary to avoid excessive exposure, especially on basement floors. The comparison with previous studies presented in Tables 7, 8 showed that the radon concentration in some European countries is quite high compared to Egypt and India. The availability of ventilation is crucial to protect inhabitants from the radiogenic risk of Radon. In addition to that, to have a valid comparison between countries, the age of the building should be considered. Now society is well aware of the radon risk, so most the recent buildings were constructed to provide reasonable safety against radon by selecting the proper materials and ceramics and reasonable ventilation (Elzain, 2015). All the results of this study were investigated and compared with usual ceramic and Indian granite building materials and it was found that the building is a healthy workplace which may be due to use of advanced building materials.
TABLE 7. Comparison of radionuclide activity concentrations (Bq.kg-1) of ceramic floor tile with other studies in different countries.
TABLE 8. Comparison of radionuclide activity concentrations (Bq.kg-1) in Granite with other studies in different countries.
On the basis of this study the following conclusions were drawn:
1) This study is the first indoor radon concentration measurement that is performed in the area of this building. Therefore, it provides preliminary data about indoor radon levels as a baseline study for a wider future national survey in the whole area
2) The overall average indoor radon concentration of the Monitored area using the SSNTDs technique was much lower than the United States intervention radon level of 150 Bq/m3. Hence there are no high potential hazards in investigated area in this study.
3) This may be attributed to the geographical location and to the ventilation as well as the climate condition.
4) Old buildings have significantly higher radon concentrations than new ones.
5) Quality assurance measurements indicated that the data obtained are of the required precision and accuracy.
Apart from the radioactive isotope K-40, all raw ceramic materials samples, as well as the end product, exhibited significant natural levels of radioactivity from the Th-232 series and Ra-226. The largest levels of Ra-222 and Th-232, as well as K-40, are found in the ZrSiO4 sample. The zircon found in glaze, which differs in pureness and precise formula, is the primary source of radioactivity in ceramic tiles. These findings recommend that the zircon-related process be given more attention. Controls should also be limited to materials made of zirconium silicate. Ra-226 and Th-232 concentrations associated with distinct fractions were determined using sequential extraction studies. Ra was found in abundance in the carbonate and residue fractions. Th-232 was found in high concentrations in the residue, iron oxide, and acid-soluble fractions. In the building, the radon survey was performed to determine indoor radon variation due to newly constructed building material. A comparison between the old surveyed building and the granite building, which are significant sources of radon, was carried out. It could be concluded that the constructed building with some types of ceramics floor tile building material will reduce the concentration of radon gas and could be used in radiation shielding. It will be strongly recommended to register the concentration of uranium and the rate of release of radon gas specification and advice the imported and exported companies to examine it to keep the high-rate radioactive radon release away from the customers. Natural radioactive materials under certain conditions can reach hazardous radiological levels. So, it was felt necessary to study the natural radioactivity in soil to assess the dose to the population in order to know the health risks and to have a baseline for future changes in the environmental radioactivity due to human activities.
The original contributions presented in the study are included in the article/Supplementary material, further inquiries can be directed to the corresponding authors.
All authors listed have made a substantial, direct, and intellectual contribution to the work and approved it for publication.
This research was funded by the Prince Sattam Bin Abdulaziz University project number (PSAU/2023/R/1445).
This study is supported via funding from Prince Sattam Bin Abdulaziz University project number (PSAU/2023/R/1445).
The authors declare that the research was conducted in the absence of any commercial or financial relationships that could be construed as a potential conflict of interest.
All claims expressed in this article are solely those of the authors and do not necessarily represent those of their affiliated organizations, or those of the publisher, the editors and the reviewers. Any product that may be evaluated in this article, or claim that may be made by its manufacturer, is not guaranteed or endorsed by the publisher.
Abdel Ghaffar, A. M., El-Arnaouty, M. B., Diab, H. M., and Hegazy, E.-S. A. (2009). Radiation synthesis of grafted polymers for studying thermoluminescence characterization and its possible application as a dosimeter at low doses. Polym. Plast. Technol. Eng. 48, 423–431. doi:10.1080/03602550902725423
Abd-Elghany, A. A., Diab, H. M., and Sulieman, A. (2020). Determination of electron radiation dose uncertainty for strontium tetraborate doped with samarium. J. Radiat. Res. Appl. Sci. 13, 246–251. doi:10.1080/16878507.2020.1727676
Abdelhamid, A. A., Badr, M. H., Mohamed, R. A., and Saleh, H. M. (2023). Using agricultural mixed waste as a sustainable technique for removing stable isotopes and radioisotopes from the aquatic environment. Sustainability 15, 1600. doi:10.3390/su15021600
Abo-Elmagd, M., and Daif, M. M. (2010). Calibration of CR-39 for radon-related parameters using sealed cup technique. Radiat. Prot. Dosim. 139, 546–550. doi:10.1093/rpd/ncp300
Afifi, M., Abass, M. R., Diab, H. M., Abou-Mesalam, M. M., and Gaafar, M. S. (2022). Dosimetric impact of some gamma radiation-induced polymeric materials incorporated silicate using thermoluminescence and ultrasonic techniques. Silicon 14, 4391–4400. doi:10.1007/s12633-021-01229-7
Ahmad, F., Shousha, H. A., and Diab, H. M. (2006). Comparative study of natural radioactivity concentrations in soil samples from the newly developed Tushki and Giza regions in Egypt. Radiat. Eff. Defects Solids 161, 257–266. doi:10.1080/10420150600652816
Åkerblom, G. (1999). Radon legislation and national guidelines. Stockholm: Swedish Radiation Protection Institute.
Aladeniyi, K., Arogunjo, A. M., Pereira, A., Khandaker, M. U., Bradley, D. A., and Sulieman, A. (2021). Evaluation of radiometric standards of major building materials used in dwellings of South-Western Nigeria. Radiat. Phys. Chem. 178, 109021. doi:10.1016/j.radphyschem.2020.109021
Al-Jarallah, M. (2001). Radon exhalation from granites used in Saudi Arabia. J. Environ. Radioact. 53, 91–98. doi:10.1016/s0265-931x(00)00110-7
Alrowaili, Z. A. (2023). Nature of radon, radium, exhalation and uranium concentration from construction materials used in Al Jouf city, Saudi Arabia. J. Radiat. Res. Appl. Sci. 16, 100579. doi:10.1016/j.jrras.2023.100579
Al-Zahrani, J. H. (2017). Estimation of natural radioactivity in local and imported polished granite used as building materials in Saudi Arabia. J. Radiat. Res. Appl. Sci. 10, 241–245. doi:10.1016/j.jrras.2017.05.001
Amin, S. A., and Hamdy, S. M. H. (2015). Measurements of radioactive pollution in the soil near the power generators. Iran. J. Energy Environ. 6, 323–327. doi:10.5829/idosi.ijee.2015.06.04.11
Antignani, S., Bochicchio, F., Ampollini, M., Venoso, G., Bruni, B., Innamorati, S., et al. (2009). Radon concentration variations between and within buildings of a research institute. Radiat. Meas. 44, 1040–1044. doi:10.1016/j.radmeas.2009.10.074
Aykamış, A. Ş., Turhan, Ş., Aysun Ugur, F., Baykan, U. N., and Kılıç, A. M. (2013). Natural radioactivity, radon exhalation rates and indoor radon concentration of some granite samples used as construction material in Turkey. Radiat. Prot. Dosim. 157, 105–111. doi:10.1093/rpd/nct110
Badran, H. M. (2018). “Indoor radon levels and annual effective dose in dwellings of najran city, Saudi Arabia,” in Key engineering materials (Switzerland: Trans Tech Publ), 393–399.
Barescut, J., Righi, S., Guerra, R., Jeyapandian, M., Verità, S., and Albertazzi, A. (2009). Natural radioactivity in Italian ceramic tiles. Radioprotection 44, 413–419. doi:10.1051/radiopro/20095078
Darby, S., Hill, D., Auvinen, A., Barros-Dios, J. M., Baysson, H., Bochicchio, F., et al. (2005). Radon in homes and risk of lung cancer: collaborative analysis of individual data from 13 European case-control studies. Bmj 330, 223. doi:10.1136/bmj.38308.477650.63
Dawoud, M. M. A., Hegazi, M. M., Saleh, H. M., and El Helew, W. K. (2022). Removal of stable and radio isotopes from wastewater by using modified microcrystalline cellulose based on Taguchi L16. Int. J. Environ. Sci. Technol. 20, 1289–1300. doi:10.1007/s13762-022-04073-3
Deng, W., Tian, K., Zhang, Y., and Chen, D. (1997). Radioactivity in zircon and building tiles. Health Phys. 73, 369–372. doi:10.1097/00004032-199708000-00009
Diab, H. M. (2017). Measurement of radon levels in water in arar city, Saudi Arabia. Rom. J. Biophys. 27, 129–136.
Diab, H. M. (2019). Accuracy and precision of RAD7 and RAD H2O accessories radon detector in water measurement based on counting statistics for sniff and normal modes. Rom. J. Biophys. 29.
Diab, H. M., Abdelghany, A. M., and Hafez, H. S. (2020). Dosimetric behavior of modified borate bioglass containing copper for low photon dose measurements using luminescence characteristics. J. Mater. Sci. Mater. Electron. 31, 20452–20459. doi:10.1007/s10854-020-04564-4
Diab, H. M., and El-Mallawany, R. (2014). Estimation of uncertainty for sulfonated grafted low density polyethylene dosimeter using thermoluminescent dosimeter. Measurement 47, 22–25. doi:10.1016/j.measurement.2013.08.042
Ehab, M., Salama, E., Ashour, A., Attallah, M., and Saleh, H. M. (2022). Optical properties and gamma radiation shielding capability of transparent barium borosilicate glass composite. Sustainability 14, 13298. doi:10.3390/su142013298
Eid, M. S., Bondouk, I. I., Saleh, H. M., Omar, K. M., and Diab, H. M. (2022). Investigating the effect of gamma and neutron irradiation on portland cement provided with waste silicate glass. Sustainability 15, 763. doi:10.3390/su15010763
Elzain, A.-E. A. (2015). Radon exhalation rates from some building materials used in Sudan. Indoor Built Environ. 24, 852–860. doi:10.1177/1420326x14537285
Esawii, H. A., Salama, E., El-ahll, L. S., Moustafa, M., and Saleh, H. M. (2022). High impact tungsten-doped borosilicate glass composite for gamma and neutron transparent radiation shielding. Prog. Nucl. Energy 150, 104321. doi:10.1016/j.pnucene.2022.104321
Eskander, S. B., Saleh, H. M., Tawfik, M. E., and Bayoumi, T. A. (2021). Towards potential applications of cement-polymer composites based on recycled polystyrene foam wastes on construction fields: impact of exposure to water ecologies. Case Stud. Constr. Mater. 15, e00664. doi:10.1016/j.cscm.2021.e00664
Fares, S. S. (2019). Natural radioactivity measurement of bricks used in the building materials of Egypt. Arab. J. Nucl. Sci. Appl. 52, 73–82. doi:10.21608/AJNSA.2019.6648.1153
Goldstein, B. D., Eisenbud, M., Gesell, T. F., Ibrahim, S. A., Kocher, D. C., Landa, E. R., et al. (1999). “Evaluation of guidelines for exposures to technologically enhanced naturally occurring radioactive materials,” in Report to the national academy of sciences–national research council (NAS–NRC) (Washington, DC: National Academy Press).
Guillén, J., Tejado, J. J., Baeza, A., Corbacho, J. A., and Muñoz, J. G. (2014). Assessment of radiological hazard of commercial granites from Extremadura (Spain). J. Environ. Radioact. 132, 81–88. doi:10.1016/j.jenvrad.2014.02.004
Haridasan, P. P., Harikumar, M., Ravi, P. M., and Tripathi, R. M. (2015). Radiological protection against exposure to naturally occurring radioactive material. Radiat. Prot. Environ. 38, 59. doi:10.4103/0972-0464.169367
Hobbs, T. G. (2000). Radioactivity measurements on glazed ceramic surfaces. J. Res. Natl. Inst. Stand. Technol. 105, 275. doi:10.6028/jres.105.031
Hofmann, J., Leicht, R., Wingender, H. J., and Wörner, J. (2000). Natural radionuclide concentrations in materials processed in the chemical industry and the related radiological impact. Luxembourg: Office for Official Publications of the European Communities.
Jensen, D. E. (1952). Uranium glass and its fluorescence. Rocks Min. 27, 230–233. doi:10.1080/00357529.1952.11768070
Krewski, D., Bird, M., Al-Zoughool, M., Birkett, N., Billard, M., Milton, B., et al. (2019). Key characteristics of 86 agents known to cause cancer in humans. J. Toxicol. Environ. Heal. Part B 22, 244–263. doi:10.1080/10937404.2019.1643536
Krewski, D., Lubin, J. H., Zielinski, J. M., Alavanja, M., Catalan, V. S., William Field, R., et al. (2006). A combined analysis of North American case-control studies of residential radon and lung cancer. J. Toxicol. Environ. Heal. Part A 69, 533–597. doi:10.1080/15287390500260945
Kumar, A., Kumar, M., Singh, B., and Singh, S. (2003). Natural activities of, and in some Indian building materials. Radiat. Meas. 36, 465–469. doi:10.1016/s1350-4487(03)00173-2
Lubin, J. H., Wang, Z. Y., Wang, L. D., Boice, J. D., Cui, H. X., Zhang, S. R., et al. (2005). Adjusting lung cancer risks for temporal and spatial variations in radon concentration in dwellings in Gansu Province, China. Radiat. Res. 163, 571–579. doi:10.1667/rr3109
Lumpkin, G. R., Gao, Y., Gieré, R., Williams, C. T., Mariano, A. N., and Geisler, T. (2014). The role of Th-U minerals in assessing the performance of nuclear waste forms. Mineral. Mag. 78, 1071–1095. doi:10.1180/minmag.2014.078.5.01
Mahat, R., and Amin, Y. (1990). Concentration of radon precursors in some Malaysian building materials. J. Radioanal. Nucl. Chem. 144, 375–378. doi:10.1007/bf02218145
Papadopoulos, A., Christofides, G., Koroneos, A., Papadopoulou, L., Papastefanou, C., and Stoulos, S. (2013). Natural radioactivity and radiation index of the major plutonic bodies in Greece. J. Environ. Radioact. 124, 227–238. doi:10.1016/j.jenvrad.2013.06.002
Quarto, M., Pugliese, M., La Verde, G., Loffredo, F., and Roca, V. (2015). Radon exposure assessment and relative effective dose estimation to inhabitants of Puglia Region, South Italy. Int. J. Environ. Res. Public Health 12, 14948–14957. doi:10.3390/ijerph121114948
Reda, S. M., and Saleh, H. M. (2021). Calculation of the gamma radiation shielding efficiency of cement-bitumen portable container using MCNPX code. Prog. Nucl. Energy 142, 104012. doi:10.1016/j.pnucene.2021.104012
Saleh, H. M. (2014). “Some applications of clays in radioactive waste management,” in Clays and clay minerals: geological origin, mechanical properties and industrial applications. Editor L. R. Wesley (New York: Nova Science Publishers, Inc), 403–415.
Saleh, H. M., Bondouk, I. I., Salama, E., and Esawii, H. A. (2021). Consistency and shielding efficiency of cement-bitumen composite for use as gamma-radiation shielding material. Prog. Nucl. Energy 137, 103764. doi:10.1016/j.pnucene.2021.103764
Saleh, H. M., Bondouk, I. I., Salama, E., Mahmoud, H. H., Omar, K., and Esawii, H. A. (2022). Asphaltene or polyvinylchloride waste blended with cement to produce a sustainable material used in nuclear safety. Sustainabilitiy 14, 3525. doi:10.3390/su14063525
Saleh, H. M., and Eskander, S. B. (2020). “Innovative cement-based materials for environmental protection and restoration,” in New materials in civil engineering (Amsterdam, Netherlands: Elsevier), 613–641.
Saleh, H. M., and Koller, M. (2021). “Recent remediation technologies for contaminated water,” in Water engineering modeling and mathematic tools (Amsterdam, Netherlands: Elsevier), 115–131.
Saleh, H. M., Moussa, H. R., El-Saied, F. A., Dawoud, M., Bayoumi, T. A., and Abdel Wahed, R. S. (2020). Mechanical and physicochemical evaluation of solidified dried submerged plants subjected to extreme climatic conditions to achieve an optimum waste containment. Prog. Nucl. Energy 122, 103285. doi:10.1016/j.pnucene.2020.103285
Salim, D. A., and Ebrahiem, S. A. (2019). Measurement of radon concentration in college of education, ibn Al-haitham buildings using rad-7 and CR-39 detector. Energy Procedia 157, 918–925. doi:10.1016/j.egypro.2018.11.258
Senthilkumar, G., Raghu, Y., Sivakumar, S., Chandrasekaran, A., Anand, D. P., and Ravisankar, R. (2014). Natural radioactivity measurement and evaluation of radiological hazards in some commercial flooring materials used in Thiruvannamalai, Tamilnadu, India. J. Radiat. Res. Appl. Sci. 7, 116–122. doi:10.1016/j.jrras.2013.12.009
Serradell, V., Ortiz, J., Ballesteros, L., and Zarza, I. (2007). “Radioactivity measurements campaign on ceramic industries: results and comments,” in CD of manuscripts submitted at the fifth international symposium on NORM (Seville, Spain.
Solehah, A. R., and Samat, S. B. (2018). Radiological impact from natural radionuclide activity concentrations in soil and vegetables at former tin mining area and non-mining area in Peninsular Malaysia. J. Radioanal. Nucl. Chem. 315, 127–136. doi:10.1007/s10967-017-5654-7
Stajic, J. M., and Nikezic, D. (2015). Measurement of radon exhalation rates from some building materials used in Serbian construction. J. Radioanal. Nucl. Chem. 303, 1943–1947. doi:10.1007/s10967-014-3726-5
Steck, D. J. (1992). Spatial and temporal indoor radon variations. Health Phys. 62, 351–355. doi:10.1097/00004032-199204000-00009
Testa, C., Desideri, D., Guerra, F., Meli, M. A., Roselli, C., and Degetto, S. (1999). Concentration and speciation of plutonium, americium, uranium, thorium, potassium and 137 Cs in a venice canal sediment sample. Czechoslov. J. Phys. 49, 649–656. doi:10.1007/s10582-999-1045-9
UNSCEAR (2000). Sources and effects of ionizing radiation, united nations scientific committee on the effects of atomic radiation (UNSCEAR) 2000 report, volume I: report to the general assembly, with scientific annexes-sources. United nations.
Venoso, G., Ampollini, M., Antignani, S., Caprio, M., Carpentieri, C., Di Carlo, C., et al. (2020). Short-term annual variations of Radon concentration in workplaces: some results in a research Institute. Radiat. Prot. Dosim. 191, 138–143. doi:10.1093/rpd/ncaa138
Keywords: natural radioactive material, radon concentration, building materials, workplaces, CR-39, radiation shielding
Citation: Abd-Elghany AA, Diab HM, Yousef AMM and Saleh HM (2023) Estimation of radon and natural radioactive emissions from two of the most widely used construction materials in Egypt. Front. Built Environ. 9:1228321. doi: 10.3389/fbuil.2023.1228321
Received: 24 May 2023; Accepted: 09 November 2023;
Published: 22 November 2023.
Edited by:
Paul Awoyera, Covenant University, NigeriaReviewed by:
Baris Sayin, Istanbul University-Cerrahpasa, TürkiyeCopyright © 2023 Abd-Elghany, Diab, Yousef and Saleh. This is an open-access article distributed under the terms of the Creative Commons Attribution License (CC BY). The use, distribution or reproduction in other forums is permitted, provided the original author(s) and the copyright owner(s) are credited and that the original publication in this journal is cited, in accordance with accepted academic practice. No use, distribution or reproduction is permitted which does not comply with these terms.
*Correspondence: Amr A. Abd-Elghany, YS5hZGVsQHBzYXUuZWR1LnNh; Hosam M. Saleh, aG9zYW0uc2FsZWhAZWFlYS5vcmcuZWc=, aG9zYW1zYWxlaDcwQHlhaG9vLmNvbQ==
Disclaimer: All claims expressed in this article are solely those of the authors and do not necessarily represent those of their affiliated organizations, or those of the publisher, the editors and the reviewers. Any product that may be evaluated in this article or claim that may be made by its manufacturer is not guaranteed or endorsed by the publisher.
Research integrity at Frontiers
Learn more about the work of our research integrity team to safeguard the quality of each article we publish.