- 1Escuela Politécnica Superior, Montepríncipe Campus de Boadilla del Monte, Universidad San Pablo-CEU, CEU Universities, Madrid, Spain
- 2UDIT Universidad de Diseño y Tecnología, Madrid, Spain
In buildings, ventilation, or rather, a lack of airtightness facilitates air leaks, from the outside to the inside and vice versa, and is not controlled. Cold air enters through the enclosure, and warm air is lost to the outdoors, due to the poor hermeticity of the facades, roofs, carpentry, ducts, etc. In order to quantify the airtightness in multi-family dwellings in Madrid, 151 blower door tests have been carried out in multi-family dwellings built in different periods whose execution has been regulated by the UNE-EN 13829 standard. Through its quantification by an n50 value, the average values of 5.8 renovations per hour have been obtained in addition to detecting the main points where air infiltration occurs. The constant improvement in the transmittance of construction elements has indicated that the entry of outside air has a progressively greater relevance to the total energy consumed by the residential sector while facilitating the uncontrolled movement of air through the building envelope. This not only implies higher energy consumption but also generates a series of problems that affect the health of the occupants, such as a lack of thermal comfort, entry of pollutants and odours, noise, inadequate operation of ventilation systems, and less protection against fire.
1 Introduction
Building energy demand has become one of the most important concerns in the construction sector. The European Energy Performance of Buildings Directive (EPBD) is committed to achieving a highly efficient and decarbonized building stock, considering that almost 50% of the final energy consumption is used for heating and cooling, 80% of which is used in buildings (European Parliament, 2018).
Concerns about the airtightness of dwellings have been taken into account in the United States since before 1995 (Sherman, 1995), in view of the fact that EU member states have committed themselves to reducing the primary energy consumption by 20% by 2020. The energy consumption in residential and commercial buildings accounts for approximately 40% of the total final energy consumption (Darvish et al., 2020), 52% of which accounts for the main heat losses in dwellings due to ventilation and therefore infiltration (Almarzouq and Sakhrieh, 2018).
A lot of documentation of air infiltration is available in European countries with cold climates but little, or almost nothing, in warm countries, such as Spain, and it is important to know the main causes for the controlled and uncontrolled air infiltration through the study of houses in Madrid, in warm climates (Lozinsky and Touchie, 2018).
Leakage is a current of air that circulates through cracks, interstices, and unwanted openings, which is produced by a pressure difference, due to wind, temperature differences, or the mechanical ventilation system itself (Goubran et al., 2017). This uncontrolled flow of air can move from the outside to the inside (infiltration, which is a common form in buildings) or in the opposite direction (exfiltration, which is rare, due to unique external and internal conditions) (Sherman, 1987; Jokisalo et al., 2009).
In this field of study, airtightness is understood as the quality of the building envelope capable of preventing the entry of air or other elements, such as water, noise, and pollutants, from an external environment to an internal environment (González-Lezcano and Hormigos-Jiménez, 2016; Bhandari et al., 2018). In order to achieve good acoustics and thermal insulation, airtightness is considered necessary for the building envelope. This is one of the necessary conditions for energy savings (Hamby, 1994; Fernandez-Antolin et al., 2019a).
The lack of airtightness of the dwelling leads to other causes in addition to the energy cost, and important among them is the quality of the air, which determines that these infiltrations cannot be considered natural ventilation due to the quality of the surrounding air (Villi et al., 2013). According to Sherman (1987), the causes of leakage in a dwelling are height, area, and age. He sets the height coefficient at 1.15, i.e., adding one storey in height increases leakage rates by 15%. The greater the area, the higher the ratio of openings to the total volume of the building. The older they get, the higher the leakage will be, at a rate of 1% per year (Sadineni et al., 2011; Bramiana et al., 2016).
This paper focuses on the characterization of the residential building stock in the Madrid area. Despite the fact that no evidence was found to justify that climate is a significant variable in terms of airtightness (Synnefa et al., 2007; Fernandez-Antolin et al., 2019b), it seems clear that there are different aspects associated with the region where the building is located such as differences in construction quality, dwelling design or materials, or due to differences in the building size or age (Lee et al., 2011; Fernandez-Antolin et al., 2021). The aim of this study is to delve into the main causes of the lack of airtightness of multi-family dwellings through 151 blower door tests in the Community of Madrid.
2 Materials and methods
A blower door is the measurement technique used to determine the level of infiltration in the dwellings studied. It consists of a fan placed on an exterior door or window that generates a pressure difference, either by pressurizing or depressurizing the room, by injecting or extracting a flow of air. The number of renovations per hour of the building is calculated by dividing the flow rate at a pressure of 50 Pa by the volume of indoor air, as a way of measuring the airtightness. Once the leakage rate for a building has been measured, it is useful to estimate the total size of all leaks or openings in the building envelope (Sfakianaki et al., 2008; Fernandez-Antolin et al., 2020). TECTITE Express airtightness test analysis software is used to calculate two separate leakage areas, based on different assumptions about the physical shape of the hole. The equivalent leakage area (EqLA) is defined by Canadian researchers at the National Research Council of Canada as the area of a sharp-edged opening through which the same amount of air will leak as that measured at a pressure difference of 10 Pa. The effective leakage area (ELA) was developed by the Lawrence Berkeley Laboratory (LBL) and is used in their infiltration model. The effective leakage area is defined as the area of a rounded edge opening through which the same amount of air will leak as that measured at a pressure difference of 4 Pa (Santamouris et al., 2001; Chan et al., 2005; Frontczak et al., 2012; González-Lezcano et al., 2020; Santamaria et al., 2020).
Figure 1 shows the equipment with which all the measurements were carried out.
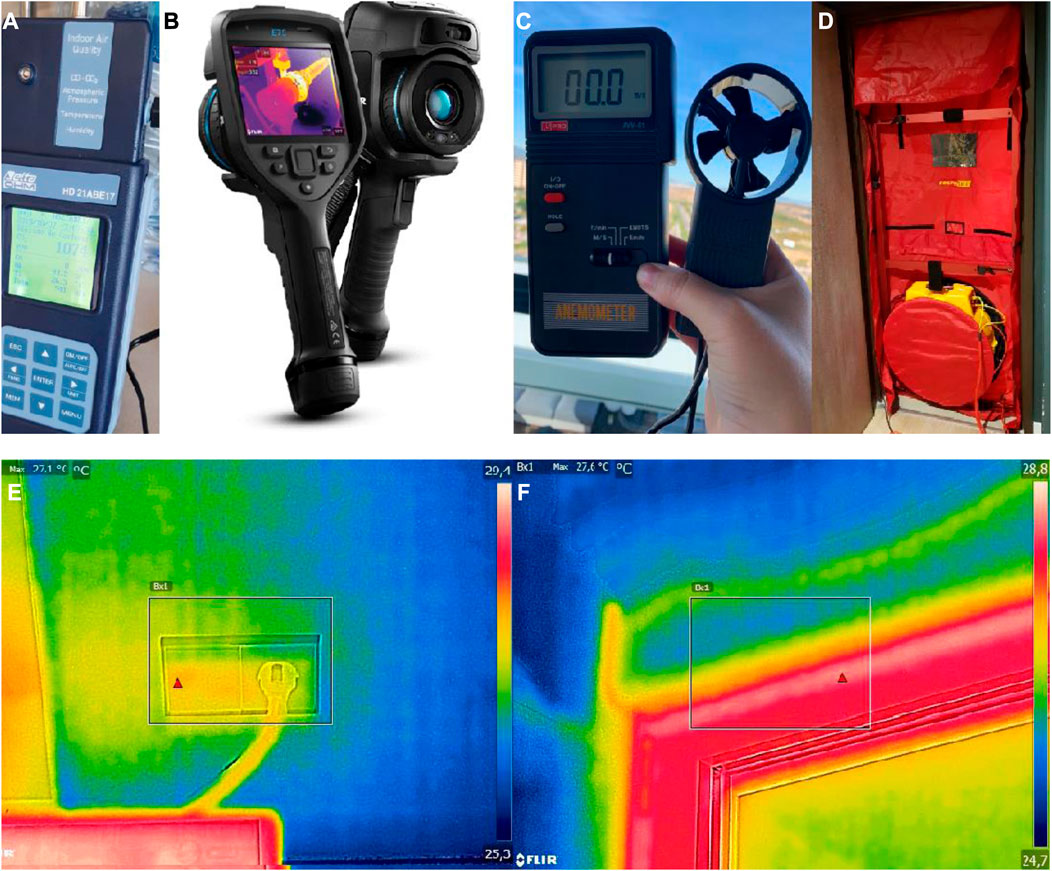
FIGURE 1. (A) Air quality, (B) thermographic camera, (C) anemometer, (D) blower door, (E) air infiltration through sockets, and (F) air infiltration through the carpentry.
During the depressurization process, data are captured using a thermography camera, as shown in Figure 1, thus locating the points where the greatest infiltrations occur in the dwelling, for which a thermal gap between the interior and the exterior is necessary.
2.1 Test description
The following equipment was used to carry out the test:
• Blower door equipment 5102 CP DM32 WiFi.
• FLIR E75 thermal imager.
• Anemometers (air speed 0.3–30 m/s) at −10 to 45°C (used for measuring the wind speed).
• Air quality monitor mod. Vertex HD21ABE17 (used to measure the indoor and outdoor temperature, indoor and outdoor relative humidity, and atmospheric pressure).
• Fantastic Pro software.
In all the tests, the atmospheric conditions were adequate to carry out pressurization and depressurization tests, since the pressure difference between the interior and exterior of the house, as indicated by the ISO/DIS 9972 standard, was less than 3 Pa and the outdoor wind speed was less than 3.6 m/s.
The evaluation of the permeability of the envelope has been carried out according to method B (building envelope test) described in the UNE-EN 13829 standard (AENOR, 2002) for the determination of airtightness in buildings by the method of pressurization by means of a fan, commonly known as the blower door test.
3 Results and discussion
Data collected from 151 measurements in collective dwellings in the Community of Madrid, from different years, are listed in Table 1.
The results obtained from the tests carried out in Madrid show an average n50 value of 5.80 renovations per hour. Among the factors observed in the dwellings, as in the United States, year of construction is the main factor (A Chan et al., 2013), which leads to a certain way of building and, therefore, a certain airtightness, giving better airtightness values between the years 1921 and 1950. Although 80% of the dwellings were renovated, they all coincide in a more massive way in their building design, without an air chamber. You cannot invest in increasing insulation if you have unwanted air infiltration, which will not allow you to limit the energy demand (Isaac and van Vuuren, 2009; Friedman and Matheson, 2017; Burgos et al., 2020; Lezcano and Burgos, 2021; Mutschler et al., 2021). Passivhaus is a world reference in efficient housing construction, as one of its principles is to limit the level of infiltration to 0.6 renovations per hour in new buildings and to 1 renovation per hour at 50 Pa in refurbished buildings, while studies are already underway to adapt this standard to hot climates (Sherman, 1987).
In Spain, there are no mandatory regulations regarding the level of airtightness in residential buildings. In the latest update of the Technical Building Code (CTE), it is indicated in its Basic Document on Energy Saving (DB-HE) of 2018 (de la Edificación, 2013) that to calculate the energy demand of buildings, losses due to ventilation and unwanted infiltrations should be considered, but no limit is established (Agee et al., 2021; Gonzalo et al., 2022; González-Lezcano, 2023).
The airtightness of residential buildings is limited, depending on whether it is natural or mechanical ventilation, as the latter will be more demanding in terms of airtightness. It is important to lay the foundations in Spain for these requirements, but always with a link to energy demand, setting the guidelines to achieve these savings and not just demanding a value for renovations.
In addition, the influence of several construction characteristics on permeability results was assessed. General trends have been identified. Nevertheless, no statistically significant results could be obtained, in part due to the reduced sample size for some categories and also because of the difficulty in isolating the variables.
4 Conclusion
The European Union has committed to continuing to reduce greenhouse gas emissions, establishing a sustainable, competitive, and decarbonized energy system by 2050. It is estimated that the building stock is responsible for approximately 36% of all CO2 emissions and that heating and cooling accounts for almost 50% of the Union’s final energy consumption, 80% of which is consumed in buildings (European Commission, 2018). For this reason, it seems essential to establish strategies that support the renovation of national building parks, facilitating their transformation in buildings with almost zero energy consumption.
In this context, one of the factors that have the greatest impact is the presence of uncontrolled airways through an envelope or infiltration. The constant improvement in the transmittance of construction elements has indicated that the entry of outside air has a progressively greater relevance to the total energy consumed by the residential sector, while facilitating the uncontrolled movement of air through the building envelope.
This not only implies higher energy consumption but also generates a series of problems that affect the health of the occupants, such as a lack of thermal comfort, entry of pollutants and odours, noise, inadequate operation of ventilation systems, and less protection against fire.
Author contributions
Conceptualization: GS, EL, RG-L, RS, and CI; methodology: GS, EL, RG-L, RS, and CI; validation: GS, EL, RG-L, RS, and CI; formal analysis: GS, EL, RG-L, RS, and CI; investigation: GS, EL, RG-L, RS, and CI; resources: GS and RG-L; data curation: GS, EL, RG-L, RS, and CI; writing—original draft preparation: GS, EL, RG-L, RS, and CI; writing—review and editing: GS, EL, RG-L, RS, and CI; visualization: GS, EL, RG-L, RS, and CI; supervision: RG-L. All authors contributed to the article and approved the submitted version.
Funding
The authors wish to thank the CEU San Pablo University Foundation for the funds dedicated to the GIMIDyL Research Group under Project Ref. G20/6-04 provided by CEU San Pablo University.
Acknowledgments
The authors wish to thank the CEU San Pablo University Foundation for the funds dedicated to the ARIE Research Group by CEU San Pablo University.
Conflict of interest
The authors declare that the research was conducted in the absence of any commercial or financial relationships that could be construed as a potential conflict of interest.
Publisher’s note
All claims expressed in this article are solely those of the authors and do not necessarily represent those of their affiliated organizations, or those of the publisher, the editors, and the reviewers. Any product that may be evaluated in this article, or claim that may be made by its manufacturer, is not guaranteed or endorsed by the publisher.
References
A Chan, W. R., Joh, J., and Sherman, M. H. (2013). Analysis of air leakage measurements of US houses. Energy Build. 66, 616–625. doi:10.1016/j.enbuild.2013.07.047
Agee, P., Nikdel, L., and Roberts, S. (2021). A measured energy use, solar production, and building air leakage dataset for a zero energy commercial building. Sci. Data 8 (1), 299. doi:10.1038/s41597-021-01082-8
Almarzouq, A., and Sakhrieh, A. (2018). Effects of glazing design and infiltration rate on energy consumption and thermal comfort in residential buildings. Therm. Sci. 23, 2951–2960. doi:10.2298/TSCI170910073A
Bhandari, M., Hun, D., Shrestha, S., Pallin, S., and Lapsa, M. (2018). A simplified methodology to estimate energy savings in commercial buildings from improvements in airtightness. Energies 11 (12), 3322. doi:10.3390/en11123322
Bramiana, C. N., Entrop, A. G., and Halman, J. I. M. (2016). Relationships between building characteristics and airtightness of Dutch dwellings. Energy Procedia 96, 580–591. Elsevier Ltd. doi:10.1016/j.egypro.2016.09.103
Burgos, M. J. M., de Toledo, H. S. Á., Lezcano, R. A. G., and de Mera, A. G. (2020). The sedentary process and the evolution of energy consumption in eight native American dwellings: analyzing sustainability in traditional architecture. Sustain. Switz. 12 (5), 1–28. doi:10.3390/su12051810
Cardoso, V. E. M., Pereira, P. F., Ramos, N. M. M., and Almeida, R. M. S. F. (2020). The impacts of air leakage paths and airtightness levels on air change rates. Buildings 10 (3), 55. doi:10.3390/buildings10030055
Chan, W. R., Nazaroff, W. W., Price, P. N., Sohn, M. D., and Gadgil, A. J. (2005). Analyzing a database of residential air leakage in the United States. Atmos. Environ. 39 (19), 3445–3455. doi:10.1016/j.atmosenv.2005.01.062
Darvish, A., Eghbali, S. R., Eghbali, G., and Mahlabani, Y. G. (2020). The effects of building glass facade geometry on wind infiltration and heating and cooling energy consumption. Int. J. Technol. 11 (2), 235–247. doi:10.14716/ijtech.v11i2.3201
European Parliament (2018). European directive 2018/844 amending directive 2010/31/EU on the energy performance of buildings directive 2012/27. EU on energy efficiency.
Fernandez-Antolin, M. M., del Río, J. M., and Gonzalez-Lezcano, R. A. (2021). The use of gamification in higher technical education: perception of university students on innovative teaching materials. Int. J. Technol. Des. Educ. 31 (5), 1019–1038. doi:10.1007/s10798-020-09583-0
Fernandez-Antolin, M. M., del Río, J. M., Costanzo, V., Nocera, F., and Gonzalez-Lezcano, R. A. (2019a). Passive design strategies for residential buildings in different Spanish climate zones. Sustain. Switz. 11 (18), 4816. doi:10.3390/su11184816
Fernandez-Antolin, M. M., Del-Río, J. M., Del Ama Gonzalo, F., and Gonzalez-Lezcano, R. A. (2020). The relationship between the use of building performance simulation tools by recent graduate architects and the deficiencies in architectural education. Energies 13 (5), 1134. doi:10.3390/en13051134
Fernandez-Antolin, M. M., del-Río, J. M., and Gonzalez-Lezcano, R. A. (2019b). Influence of solar reflectance and renewable energies on residential heating and cooling demand in sustainable architecture: A case study in different climate zones in Spain considering their urban contexts. Sustain. Switz. 11 (23), 6782. doi:10.3390/su11236782
Friedman, A., and Matheson, M. (2017). Selecting and installing energy-efficient windows to improve dwelling sustainability. VITRUVIO - Int. J. Archit. Technol. Sustain. 2 (2), 2. doi:10.4995/vitruvio-ijats.2017.7687
Frontczak, M., Schiavon, S., Goins, J., Arens, E., Zhang, H., and Wargocki, P. (2012). Quantitative relationships between occupant satisfaction and satisfaction aspects of indoor environmental quality and building design. Indoor Air 22 (2), 119–131. doi:10.1111/j.1600-0668.2011.00745.x
González-Lezcano, R. A. (2023). Editorial: design of efficient and healthy buildings. Front. Built Environ. 9. Frontiers Media S.A. doi:10.3389/fbuil.2023.1210956
González-Lezcano, R. A., and Hormigos-Jiménez, S. (2016). “Energy saving due to natural ventilation in housing blocks in Madrid,” in IOP Conference Series: Materials Science and Engineering (Institute of Physics Publishing). doi:10.1088/1757-899X/138/1/012002
González-Lezcano, R. A., López-Fernández, E., Cesteros-García, S., and Sanglier-Contreras, G. (2020). Evaluation of energy-saving strategies according to Spanish regulations. Int. J. Energy, Environ. Econ. 26 (3), 197–217.
Gonzalo, F. D. A., Griffin, M., Laskosky, J., Yost, P., and González-lezcano, R. A. (2022). Assessment of indoor air quality in residential buildings of new england through actual data. Sustain. Switz. 14 (2), 739. doi:10.3390/su14020739
Goubran, S., Qi, D., Saleh, W. F., Wang, L., and Leon), (2017). Comparing methods of modeling air infiltration through building entrances and their impact on building energy simulations. Energy Build. 138, 579–590. doi:10.1016/j.enbuild.2016.12.071
Hamby, D. M. (1994). A review of techniques for parameter sensitivity analysis of environmental models. Environ. Monit. Assess. 32 (2), 135–154. doi:10.1007/BF00547132
Isaac, M., and van Vuuren, D. P. (2009). Modeling global residential sector energy demand for heating and air conditioning in the context of climate change. Energy Policy 37 (2), 507–521. doi:10.1016/j.enpol.2008.09.051
Jokisalo, J., Kurnitski, J., Korpi, M., Kalamees, T., and Vinha, J. (2009). Building leakage, infiltration, and energy performance analyses for Finnish detached houses. Build. Environ. 44 (2), 377–387. doi:10.1016/j.buildenv.2008.03.014
Lee, M. J., Kim, N. I., and Ryou, H. S. (2011). Air tightness measurement with transient methods using sudden expansion from a compressed chamber. Build. Environ. 46 (10), 1937–1945. doi:10.1016/j.buildenv.2011.04.001
Lezcano, R. A. G., and Burgos, M. J. M. (2021). Airflow analysis of the Haida plank house, a breathing envelope. Energies 14 (16), 4871. doi:10.3390/en14164871
Lozinsky, C. H., and Touchie, M. F. (2018). Improving energy model calibration of multi-unit residential buildings through component air infiltration testing. Build. Environ. 134, 218–229. doi:10.1016/j.buildenv.2018.02.040
Mutschler, R., Rüdisüli, M., Heer, P., and Eggimann, S. (2021). Benchmarking cooling and heating energy demands considering climate change, population growth and cooling device uptake. Appl. Energy 288, 116636. doi:10.1016/j.apenergy.2021.116636
Sadineni, S. B., Madala, S., and Boehm, R. F. (2011). Passive building energy savings: A review of building envelope components. Renew. Sustain. Energy Rev. 15, 3617–3631. Elsevier Ltd. doi:10.1016/j.rser.2011.07.014
Santamaria, B. M., Del Ama Gonzalo, F., Pinette, D., Gonzalez-Lezcano, R. A., Aguirregabiria, B. L., and Ramos, J. A. H. (2020). Application and validation of a dynamic energy simulation tool: A case study with water flow glazing envelope. Energies 13 (12), 3203. doi:10.3390/en13123203
Santamouris, M., Papanikolaou, N., Livada, I., Koronakis, I., Georgakis, C., Argiriou, A., et al. (2001). On the impact of urban climate on the energy consumption of buildings. Sol. Energy 70 (3), 201–216. doi:10.1016/S0038-092X(00)00095-5
Sfakianaki, A., Pavlou, K., Santamouris, M., Livada, I., Assimakopoulos, M. N., Mantas, P., et al. (2008). Air tightness measurements of residential houses in Athens, Greece. Build. Environ. 43 (4), 398–405. doi:10.1016/j.buildenv.2007.01.006
Sherman, M. H. (1987). Estimation of infiltration from leakage and climate indicators. Energy Build. 10 (1), 81–86. doi:10.1016/0378-7788(87)90008-9
Sherman, M. (1995). The use of blower door data. Indoor Air 5 (3), 215–224. doi:10.1111/j.1600-0668.1995.t01-1-00008.x
Synnefa, A., Santamouris, M., and Akbari, H. (2007). Estimating the effect of using cool coatings on energy loads and thermal comfort in residential buildings in various climatic conditions. Energy Build. 39 (11), 1167–1174. doi:10.1016/j.enbuild.2007.01.004
Keywords: residential buildings, natural ventilation, air infiltration, building envelope airtightness, energy leakage, indoor air quality monitoring
Citation: González-Lezcano RA, Sanglier Contreras G, Iglesias Sanz CM, Sancho Alambillaga R and López Fernández EJ (2023) Construction parameters that affect the air leaks of the envelope in dwellings in Madrid. Front. Built Environ. 9:1220559. doi: 10.3389/fbuil.2023.1220559
Received: 10 May 2023; Accepted: 28 August 2023;
Published: 13 September 2023.
Edited by:
Faming Wang, KU Leuven, BelgiumReviewed by:
Jiying Liu, Shandong Jianzhu University, ChinaCopyright © 2023 González-Lezcano, Sanglier Contreras, Iglesias Sanz, Sancho Alambillaga and López Fernández. This is an open-access article distributed under the terms of the Creative Commons Attribution License (CC BY). The use, distribution or reproduction in other forums is permitted, provided the original author(s) and the copyright owner(s) are credited and that the original publication in this journal is cited, in accordance with accepted academic practice. No use, distribution or reproduction is permitted which does not comply with these terms.
*Correspondence: Roberto Alonso González-Lezcano, rgonzalezcano@ceu.es