- Spatial Perception and Cognitive Experience (SPACE) Laboratory, Department of Design and Merchandising, Colorado State University, Fort Collins, CO, United States
This perspective article, looking through the lens of neurodiversity, discusses the benefits and challenges of implementing virtual environments and wearable technologies in interior design and related fields. While the relationship between human perception and built environments has long been studied in the environmental design disciplines, the direct impact on occupant performance related to neurodiversity has been underexplored in research, with a shortage of knowledge supporting how it can be applied in design practice concerning the end users. Individuals’ perceptual, cognitive, and affective responses to their surroundings vary, as neurodiversity plays a key role in the invisible, human-environment interaction. Thus, measuring, analyzing, and understanding affective, perceptual, and cognitive experiences is a challenging process in which various factors come into play, and no single method or measurement can adequately work for all. Due to such challenges, research has also utilized various biometric measurements and tools for immersive experiments in physical and virtual environments, e.g., eye tracking used in studies on gaze behaviors and immersive virtual reality (IVR) used in studies on the spatial perception of dementia patients. Along with empirical methods, studies have stressed the contribution of phenomenology to looking into the hidden dimension, the ‘why factors’ of perception, cognition, and affectivity. Concerning the methodological approach, this perspective article shares insights into a novel process model, Participatory Neurodesign (PND) framework, used in wayfinding research and design processes utilizing eye tracking and IVR. Opportunities for neurodesign research and design practice are also discussed, focusing on the health, safety, and wellbeing of end-users.
1 Introduction
Individuals experience their surroundings differently, forming their minds, emotions, and behaviors. In this phenomenon, the body is not separate from the mind, and how one perceives and responds to the surroundings is interdependent on the physical structure of the body in its unique way. A built environment is apprehended through the perceptual and sensorial mechanisms. The invisible and intangible interactions between the environmental stimuli and brain responses have challenged designers when they attempt to integrate neurodiversity into the traditional design methods typically set around the physical body and visual aesthetics. While evidence-based design has become the standard practice in the leading design industry, tacit knowledge often plays a significant role in design approaches to the realm of emotion, perception, and cognition. As strives to better inform designers about human-environment interactions, design research has adopted theories and methods from various disciplines, including neuroscience and psychology, which have contributed to investigating the behavioral, cognitive, and social aspects of human-environment relationships. In recent years, neurodesign research has increasingly adopted wearable technologies, often utilizing immersive virtual reality in which the body plays a different role from that in the real (or physical) world. While neurodesign can establish its position as an interdisciplinary inquiry and practice of design, encompassing psychology, neuroscience, and related domains of design (Auernhammer et al., 2021), studies have not sufficiently provided tangible frameworks or guidelines for design practice that directly contributes to people’s health, safety, and wellbeing.
2 Neurodiversity in design dialogues
In recent years, the design industry has acknowledged the impact of neurodiversity on occupant experiences in built environments: e.g., HKS, one of the leading architectural design firms, has promoted brain-healthy workplace design. Studies on workplace and educational environments have also suggested how interior design can respond to spatial needs for hypersensitive and hypo-sensitive individuals and different types of work such as collaboration, respite, and independent tasks (Brinzea, 2019; Bruyère and Colella, 2022; Krzeminska et al., 2019). Research has shown overstimulating environments can trigger strong reactions—e.g., anxiety, epileptic seizures, and stress—to smells, sounds, temperature changes, harsh lighting, intense colors, and complicated visuals, risking occupant health and wellness (Shraiky et al., 2012). Studies on other aspects, e.g., visual attention or wayfinding, have focused on environmental stimuli as cognitive cues. In wayfinding, for example, considered crucial are visual contrast between background and foreground, variety and repetition of sensory stimuli, and place identity and spatial order for affordance and legibility, which heavily affect how the viewers comprehend the overall context of their surroundings (Palmer et al., 2016). As the various emphases show, design domains have paid attention to the explicit and implicit aspects of brain responses to environments, ranging from disability and deficits to differences in perceptual and cognitive patterns.
The issue is that the generally accepted, binary concept of neurodiversity—neurotypical versus neurodiverse (or divergent)—has dominated the dialogue in academic, industry, and public contexts, despite the dictionary definition “individual differences in brain functioning regarded as normal variations within the human population” (Merriam-Webster Dictionary, 2022). Primarily conceived neurodivergence are disorders or impairments such as attention deficit disorder (ADD), attention deficit hyperactivity disorder (ADHD), autism spectrum disorder (ASD), dyslexia, developmental coordination disorder (DCD; also known as dyspraxia), and epilepsy (Brinzea, 2019). Despite the notable contributions of research specialized in the conditions, misunderstanding the research purposes may result in a tendency to focus on individual brain functions in design research and approach, which may leave less-known phenomena out of the dialogue around neurodiversity. While neurodiversity lies in the complex and holistic brain-mind-body-environment interaction, certain neurological aspects may be sheer differences, not disorders of specific functions: e.g., a spectrum of synesthesia—unique cross-sensory phenomena that can inform design processes. Acknowledging such can help designers expand and reframe the traditional concept and principles of universal design from primarily focused on anthropometrics and visible disabilities to encompassing a broader spectrum of diversity, including the invisible neurodiversity.
Discussed in the following chapter are the advantages and challenges in empirical research utilizing wearable technologies and immersive environments and how such research relates to design practice concerning the neurodiversity of the end-users.
3 Implementing wearable technologies in neurodesign
Neurodesign is the intersection between neuroscience, psychology, and design, which contribute to mutual benefit. Because it is a relatively new field, a gap still exists in how knowledge is communicated between research and design practice under the umbrella (Auernhammer et al., 2021). Measuring and analyzing human experiences, especially the affective, perceptual, and cognitive, is a challenging process in which various factors play a role, and no single method can adequately work for all. Due to such challenges, environmental design disciplines have long adopted interdisciplinary approaches and methods. Self-report (e.g., interviews and surveys) and interpretive content analysis have been popular methods in investigating human perception and cognition in built environments. In recent years, experimental methods, virtual reality, and biometric measurements have increasingly been used in environmental design fields: e.g., eye tracking, electroencephalography (EEG), and functional magnetic resonance imaging (fMRI), to examine human responses or affects—e.g., sensations and emotions—to environmental stimuli (Vecchiato et al., 2011; Vartanian et al., 2015). Immersive virtual reality (IVR) has also been of growing interest to researchers, which can simulate “presence in real life.” As IVR allows researchers to control the setting and versatility to implement multisensory elements, it has been used in studies on post-trauma rehabilitation and interior design for dementia patients (Adi and Aljunaidy, 2020).
Given that neurophysiological data are descriptive and do not have the explanatory capacity, researchers have paid attention to experimental phenomenology that attempts to identify “what” as well as to uncover “how” and “why.” Neurophenomenology grounded in the approach combines neuroscience and phenomenology, with an emphasis on subjective experience, mind, and consciousness (Varela, 1996; Petitmengin et al., 2007). When it comes to virtual environments (VE), one may experience fast changes in feelings and perceptions in the sequence of spaces or abrupt shifts between spaces in the VE. Measuring the emotional, perceptual, and cognitive responses to an unfamiliar or less-usual experience may involve a complicated setup and process utilizing multiple devices to leverage different technologies. Despite the attempt, they might still not be able to capture the covert aspects of the subjective spatial experience, which may be better expressed through interviews or think-aloud.
3.1 Immersive virtual reality (IVR)
One’s ability to process stimulus information is optimized by the input of additional information to aid the visual information; looking at proprioceptive feedback and actions such as turning around or turning upside down affect the person’s understanding of the surroundings and objects within it (Kwon and Iedema, 2022). The large shift from physical to virtual environments has decreased people’s kinetic engagement (Morel et al., 2015), but it might have increased other types of sensory engagement in virtual environments (Gallace et al., 2012; Wilson and Soranzo, 2015). Research has informed that VR and the brain share the same mechanism: embodied simulation of the body. While studies suggest a possibility of IVR altering the experience of the physical body by designing targeted virtual environments, the “I don’t see my body where I am in” situation can cause perceptual and cognitive confusion as the person immersed in the simulated virtual world cannot see the movement of the physical body that the brain needs to reference to predict the sensory consequences (Wilson and Soranzo, 2015; Seabrook et al., 2020).
Although the terms IVR and VR are often interchangeably used, IVR is a newer technology, and VR is a more general term under which specific terms and concepts fall. Non-immersive VR and semi-immersive VR have long been incorporated into education, research, and even leisure. An example of non-immersive VR is a video game that relies on a computer or video game console, display, and input devices like keyboards, mice, and controllers. Semi-immersive VR that provides users with a partially virtual environment has long been used in design education, research, and practice: e.g., high-resolution 3D graphics that give viewers immersive feeling through partial replication of the real-world mechanism; the viewers remain connected to their physical surroundings. IVR has increasingly been used in user research on sensory engagement (Gallace et al., 2012; Wilson and Soranzo, 2015), typically focused on the visual and often involving the non-visual, e.g., auditory and haptic. The kinesthetic sense gives humans the ability to identify specific environmental characteristics based on the body’s spatial properties, including exteroception, enteroception, and proprioception (Kwon and Iedema, 2022, p. 5). How vision and proprioception contribute to retrieving information about body location and movement is to be further investigated, to which development of technologies that facilitate human navigation and inform about motor rehabilitation can contribute (Bayramova et al., 2021). IVR has also been used with biometric tools such as EEG and eye tracking. Banaei et al. (2017) used IVR and EEG to investigate how varying forms in the interior environment affected the participants’ emotional state and the aligning areas of the brain that process shape and forms that are defined “not only by the geometry and by the features such as type, location, scale, and angle, but also by the way the inhabitants experience [the] environment” (p. 12).
3.2 Eye tracking
Eye tracking is a popular tool used in studying visuoperceptual processes, which has historical roots in cognitive research on reading. Eye tracking can measure eye movements—how, where, and what people look at—with high precision providing insights into cognitive processes and attentional states. Although eye tracking is not a direct measure of visual acuity, it has been effectively used in various domains, including usability research (Manhartsberger and Zellhofer, 2005), human factors research, and safety applications (Han et al., 2020), psychological/cognitive research (Mele and Federici, 2012), education and training (Tien et al., 2014), kinesiology and sports sciences (Lim et al., 2018), and car/airplane simulations (Palinko et al., 2010). The types of eye trackers currently used in research include screen-mounted, wearable (e.g., glasses and IVR eye tracker), and web-based. One of the significant advantages of mobile eye tracking technology is the versatile application in wearable devices, including HMD-based IVR that can simulate not only a visual setting but also invisible or non-fixed attributes (e.g., movements, sounds, and human interactions) of the human environment. It can purposefully and effectively separate the subject from the implication of the real-world space where the physical body is situated. The technology allows unobtrusive or less obtrusive experience in eye tracking in 3D settings, whether physical or virtual.
The early research and some recent studies on visual attention took the eye-mind hypothesis as a strong validation for the power of eye-tracking, which claims the mind processes what eyes are seeing (Schindler and Lilienthal, 2019). A concern with the eye-mind hypothesis is that it could lead to overgeneralization or oversimplification, undermining the covert aspects of visual attention, because gaze data alone cannot explain the ‘why factors’ of the gaze behaviors, which is critical information for the design process. Individuals’ gazes respond differently to what they see in a three-dimensional space, from how they respond to visual content displayed on a two-dimensional fixed surface. Despite its advantages in certain types of research (e.g., patterns in reading a webpage), a 2-D digital display cannot sufficiently resemble a real-life space where brain responses are affected by various non-visual factors, physical depth of space, the distance between objects and thus, sense of scale in relation to the body. For this reason, wearable eye-tracking technologies have been adopted in studies concerning spatial identification, navigation, and wayfinding. Figure 1.
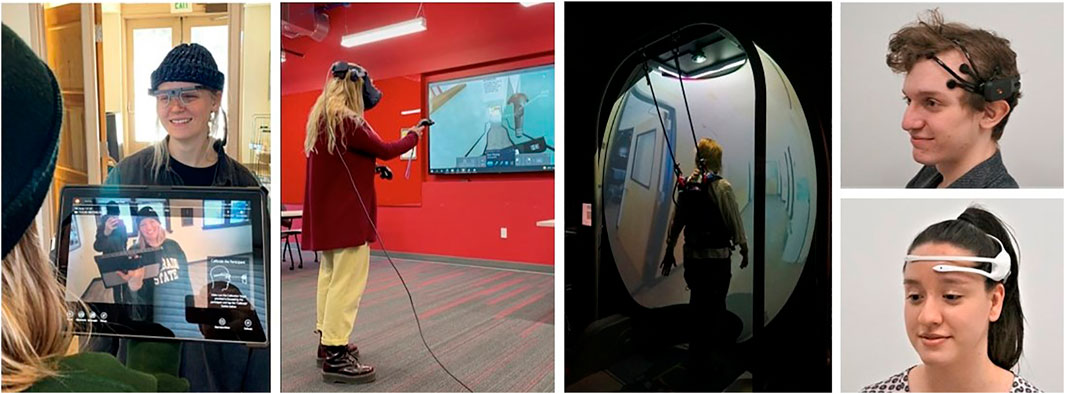
FIGURE 1. Wearable devices used in environmental design research: eye tracking, and head-mounted display immersive virtual reality (HMD IVR), computerized dynamic posturography (CDP IVR), and electroencephalography (EEG)—order from left to right (photo credit: Kwon).
3.3 Participatory Neurodesign (PND) framework
The Spatial Perception and Cognitive Experience (SPACE) Laboratory at Colorado State University has implemented in-situ eye tracking and IVR in its recent projects using a neurodesign process model grounded in experimental phenomenology to fill in the overlooked gap between neurodesign research and design practice. The Participatory Neurodesign (PND) framework (Figure 2) bridging the gap between academic research and design practice consists of six phases: 1) participatory visioning with stakeholders, 2) pre-design research, 3) co-design, prototyping, and stakeholder feedback, 4) post-design assessment, 5) fabrication and construction (installation) and 6) post-project research and post-occupancy assessment (Kwon et al., 2022; Kwon et al., 2023). While no precedents have been found, a sheer assumption might be that the PND framework is not a novel method because eye tracking and IVR have been used in research for many years. However, the mixed-method PND approach is unique in its flexibility in application and suitability to various types of evidence-based design practices that engage stakeholders.
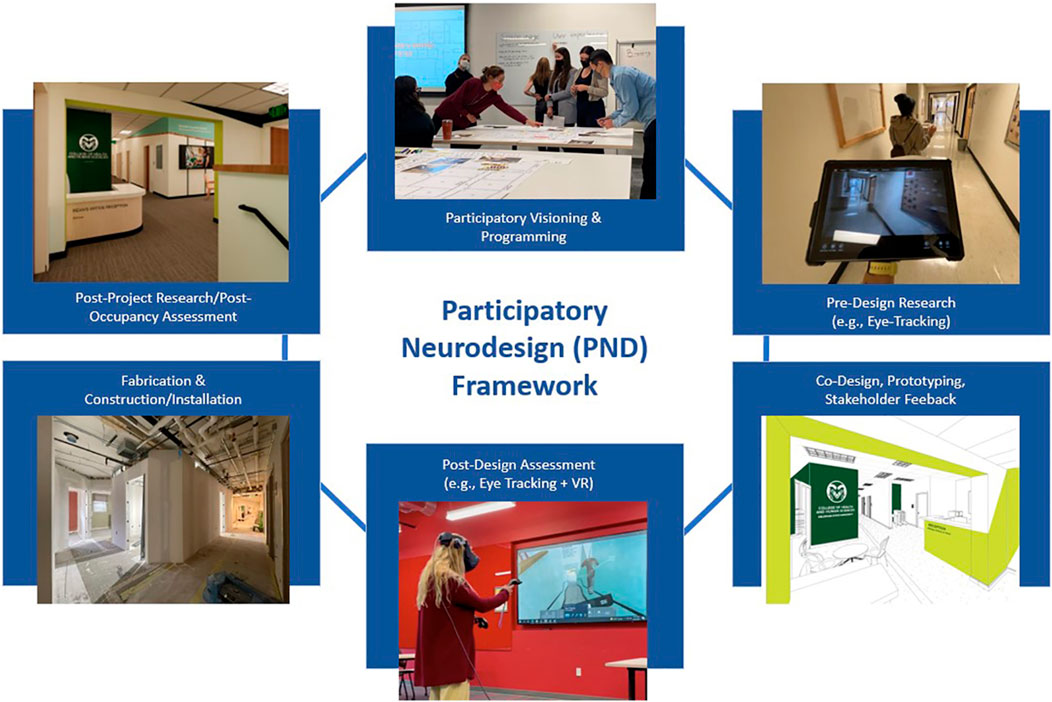
FIGURE 2. Participatory Neurodesign (PND) framework adopted in wayfinding design projects based on eye tracking research.
The SPACE Lab conducted research-based wayfinding design projects engaging the client stakeholders and current users. The PND approach was particularly important as the building users included counselors working with individuals at risk and with various conditions. In the PND process, the pre-design research implemented in-situ eye tracking involving concurrent think-aloud to examine subjects’ gaze patterns in navigating inside an unfamiliar building. Pre-design research findings were applied in the environmental graphic design process, and the design outcomes were simulated in a digital building model. For post-design research, in-lab eye tracking was conducted using a walkthrough simulation of the digital model. In tandem with eye tracking, IVR experiments using a head-mounted display (HMD) device were conducted to examine how subjects reacted to the virtual building space, which was found to deliver a better sense of the real-world scale. Both the pre-design and post-design experiments incorporated concurrent think-aloud to understand the “why factors” of the gaze behaviors. For the same purpose, Kwon and Kim. (2021) also adopted an experimental phenomenology approach using eye tracking and open-ended interview to investigate the impacts of auditory stimuli on individuals’ gaze behaviors in commercial environments. The unique strength of these studies using concurrent think-aloud is in the meaningful findings that the descriptive (or objective) gaze data alone could not explain. For example, visual attention, e.g., longer dwell time or frequent fixations appearing in gaze data, can mean not only interests but also distractors or stressors; gaze fixations indicate not only positive but also negative attention implying oddness, unfit, or confusion; gaze fixations do not always indicate that the subject notices and comprehends the content. As such, the hidden dimensions of environmental perception, cognition, and behavior are the key to designers’ adequate understanding of occupant neurodiversity.
4 Challenges with using wearable technologies in neurodesign
Despite many advantages, using wearable or immersive technologies in research experiments can be challenging due to the potential risks from direct contact between the devices and the subject’s body and the impact of associated stimuli on the brain. For example, despite the slim chance, the lights from the sensors built into a wearable eye tracker can trigger photosensitive epileptic reactions. Although such impacts might not always be noticeable immediately, they can potentially harm participants with certain conditions related to hypersensitivity: e.g., epilepsy that is rarely instantaneous and develops over time with a sequence of triggers (Petitmengin et al., 2007). While triggers are not universal among people with epilepsy, complex and intense visual stimuli are common triggers for seizures: e.g., bright light, complex patterns, and vivid colors (Shraiky et al., 2012). IVR can also cause discomfort, including feelings of sway, to which some research participants respond sensitively.
The current scope of neurodiversity considered in interior design practice and related fields seems limited to a few conditions, such as autism and dementia. With this concern, the SPACE Lab has developed research tools integrating immersive environments illustrating distinct types of building interior configurations and factors to examine how occupant comfort and performance, including physical balancing, are affected by visual stimuli regarding neurodiversity. The development process involves researchers with varied expertise to assure the safety of research participants, as leveraging IVR in research concerning neurodiverse end-users can be a complex, sensitive, and time-consuming process with potential risks to participant health and safety. In 2022, Brinley Shultz and the SPACE Lab conducted an exploratory pilot study for an interior design project for occupants with epilepsy, using visual stimuli that consisted of interior images focused on color, light, and pattern, with subtle adjustments of the visual complexity and intensity. Due to the varying risk factors in using wearable technologies and the absence of precedent research verifying the safety of such methods in design research, the researchers used still images on a digital display despite acknowledging the effectiveness of IVR in simulating spatial experience. The image tool was used paired with an open-ended interview questionnaire developed based on a symbolic interaction framework rooted in phenomenology and psychology. The study helped understand how individuals with epileptic episodes may perceive their surroundings and how the spatial elements and factors may affect their daily functions. Specific details found from the pilot study were applied in a multi-purpose wellness center design resulting in unconventional design solutions specific to the target users. The various challenges found in the research and design processes are great opportunities for further research in the underexplored area of study.
5 Conclusion
In recent years, the concepts and contexts of human environments have evolved, and digital content and virtual reality have become part of people’s mundane lives. Wearable technologies have also rapidly evolved, making meaningful contributions to research on human behaviors and perception in various fields, including environmental design disciplines. Especially, wearable devices and immersive technologies allow subjects less obtrusive visual experience along with physical mobility at varying degrees, delivering a strong sense of presence in the virtual environment separate from the real world where the physical body is situated. Digital modeling plays an essential role in developing visual stimuli to adequately utilize VR in design research and practice, which is crucial for effective communication with participants and stakeholders. This, especially in practice, can prevent errors and unnecessary costs. With such advantages, immersive and wearable technologies can help bridge the gap between empirical research and the production of tangible outcomes for end-users. To an extent, immersive technologies can help designers explore and better understand the spatial experiences of occupants/users with a varying range of neurological (dis)abilities, which, in the long term, can contribute to shifting the climate around neurodiversity: instead of ‘neurodiverse’ being seen as having a disability, it is seen as having a difference in strengths and ways of processing experiences and stimuli.
Data availability statement
The original contributions presented in the study are included in the article/supplementary material, further inquiries can be directed to the corresponding author.
Ethics statement
The studies involving human participants were reviewed and approved by Colorado State University Institutional Review Board. The patients/participants provided their written informed consent to participate in this study.
Author contributions
JK wrote the manuscript and developed the PND process model. SL, AI, AS, CL, and KM contributed to the literature review, graphical presentation, and exampled projects. All authors contributed to the article and approved the submitted version.
Funding
The projects exampled in the manuscript were funded by the American Society of Interior Designers (ASID) Foundation, CSU’s College of Health and Human Sciences, and the Interior Design Educators Council (IDEC) Foundation.
Acknowledgments
Brinley Shultz contributed to this study by conducting the multi-purpose wellness center project exemplified in this manuscript.
Conflict of interest
The authors declare that the research was conducted in the absence of any commercial or financial relationships that could be construed as a potential conflict of interest.
Publisher’s note
All claims expressed in this article are solely those of the authors and do not necessarily represent those of their affiliated organizations, or those of the publisher, the editors and the reviewers. Any product that may be evaluated in this article, or claim that may be made by its manufacturer, is not guaranteed or endorsed by the publisher.
References
Adi, M. N., and Aljunaidy, M. M. (2020). The usefulness of using virtual reality to assess elderly and dementia friendly hospital design. IDA Int. Des. Art J. 2 (1), 137–150.
Auernhammer, J., Sonalkar, N., and Saggar, M. (2021). “NeuroDesign: From neuroscience research to design thinking practice,” in Design thinking research: Interrogating the doing (Charn, Switzerland: Spinger), 347–355. doi:10.1007/978-3-030-62037-0_16
Banaei, M., Hatami, J., Yazdanfar, A., and Gramann, K. (2017). Walking through architectural spaces: The impact of interior forms on human brain dynamics. Front. Hum. Neurosci. 11, 477. doi:10.3389/fnhum.2017.00477
Bayramova, R., Valori, I., McKenna-Plumley, P. E., Callegher, C. Z., and Farroni, T. (2021). The role of vision and proprioception in self-motion encoding: An immersive virtual reality study. Atten. Percept. Psychophys. 83 (7), 2865–2878. doi:10.3758/s13414-021-02344-8
Brinzea, V. M. (2019). Encouraging neurodiversity in the evolving workforce: The next frontier to a diverse workplace. Sci. Bulletin–Economic Sci. 18 (3), 13–25.
Bruyère, S. M., and Colella, A. (2022). Neurodiversity in the workplace: Interests, issues, and opportunities. New York, NY: Taylor & Francis.
Gallace, A., Ngo, M. K., Sulaitis, J., and Spence, C. (2012). “Multisensory presence in virtual reality: Possibilities & limitations,” in Multiple sensorial media advances and applications: New developments in MulSeMedia (Hershey, PA: IGI Global), 1–38. doi:10.4018/978-1-60960-821-7.ch001
Han, Y., Yin, Z., Zhang, J., Jin, R., and Yang, T. (2020). Eye-tracking experimental study investigating the influence factors of construction safety hazard recognition. J. Constr. Eng. Manag. 146 (8), 04020091. doi:10.1061/(ASCE)CO.1943-7862.0001884
Krzeminska, A., Austin, R. D., Bruyère, S. M., and Hedley, D. (2019). The advantages and challenges of neurodiversity employment inorganizations. J. Manag. Organ. Cambridge University Press 25 (4), 453–463. doi:10.1017/jmo.2019.58
Kwon, J., and Iedema, A. (2022). Body and the senses in spatial experience: The implications of kinesthetic and synesthetic perceptions for design thinking. Front. Psychol. 13, 864009. doi:10.3389/fpsyg.2022.864009
Kwon, J., and Kim, J. Y. (2021). Meaning of gaze behaviors in individuals' perception and interpretation of commercial interior environments: An experimental phenomenology approach involving eye-tracking. Front. Psychol. 12, 581918. doi:10.3389/fpsyg.2021.581918
Kwon, J., Malinin, L., Scolere, L., Schmidt, A., and Frazier, J. (2022). “Educational implication of eye-tracking research for experiential learning through participatory neurodesign practice,” in 2022 IDEC annual conference proceedings (New York: Interior Design Educators Council).
Kwon, J., Schmidt, A., Iedema, A., and Marrufo, K. (2023). “How empirical research informs design process: Learning from wayfinding design projects implementing eye tracking,” in 2023 IDEC annual conference proceedings (Washington, DC: Interior Design Educators Council).
Lim, J., Chang, S. H., and Adriane, C. T. (2018). Effects of point of aim on the accuracy and eye movement behavior in bowling: a pilot study. Int. J. Sports Sci. 6 (3), 38–44.
Manhartsberger, M., and Zellhofer, N. (2005). Eye tracking in usability research: What users really see. Usability Symp. 198 (2), 141–152.
Mele, M. L., and Federici, S. (2012). Gaze and eye-tracking solutions for psychological research. Cogn. Process. 13, 261–265. doi:10.1007/s10339-012-0499-z
Merriam-Webster Dictionary (2022). Neurodiversity. Avaliable At: https://www.merriam-webster.com/dictionary/neurodiversity (Accessed September 16, 2022).
Morel, M., Bideau, B., Lardy, J., and Kulpa, R. (2015). Advantages and limitations of virtual reality for balance assessment and rehabilitation. Neurophysiol. Clinique/Clinical Neurophysiol. 45 (4-5), 315–326. doi:10.1016/j.neucli.2015.09.007
Palinko, O., Kun, A. L., Shyrokov, A., and Heeman, P. (2010). “Estimating cognitive load using remote eye tracking in a driving simulator,” in Proceedings of the 2010 symposium on eye-tracking research & applications (Santa Barbara, CA: ACM), 141–144. doi:10.1145/2168556.2168650
Palmer, L., Preez, M., and Raeburn, C. (2016). Colour & wayfinding. Edinburgh, UK: Trust Housing Association, 1–52.
Petitmengin, C., Navarro, V., and Le Van Quyen, M. (2007). Anticipating seizure: Pre-reflective experience at the center of neuro-phenomenology. Conscious. cognition 16 (3), 746–764. doi:10.1016/j.concog.2007.05.006
Schindler, M., and Lilienthal, A. J. (2019). Domain-specific interpretation of eye tracking data: Towards a refined use of the eye-mind hypothesis for the field of geometry. Educ. Stud. Math. 101, 123–139. doi:10.1007/s10649-019-9878-z
Seabrook, E., Kelly, R., Foley, F., Theiler, S., Thomas, N., Wadley, G., et al. (2020). Understanding how virtual reality can support mindfulness practice: Mixed methods study. J. Med. Internet Res. 22 (3), 16106. doi:10.2196/16106
Shraiky, J., Schoonover, J., Sirven, J., and Helepololei, L. (2012). Sensory-based design & epilepsy: Analyzing effects of design innovations on patient treatment and recovery. Enq. ARCC J. Archit. Res. 9 (1). doi:10.17831/enq:arcc.v9i1.66
Tien, T., Pucher, P. H., Sodergren, M. H., Sriskandarajah, K., Yang, G. Z., and Darzi, A. (2014). Eye tracking for skills assessment and training: A systematic review. J. Surg. Res. 191 (1), 169–178. doi:10.1016/j.jss.2014.04.032
Varela, F. J. (1996). Neurophenomenology: A methodological remedy for the hard problem. J. Conscious. Stud. 3 (4), 330–349.
Vartanian, O., Navarrete, G., Chatterjee, A., Fich, L. B., Gonzalez-Mora, J. L., Leder, H., et al. (2015). Architectural design and the brain: Effects of ceiling height and perceived enclosure on beauty judgments and approach-avoidance decisions. J. Environ. Psychol. 41, 10–18. doi:10.1016/j.jenvp.2014.11.006
Vecchiato, G., Astolfi, L., Fallani, F. D. V., Toppi, J., Aloise, F., Bez, F., et al. (2011). On the use of EEG or MEG brain imaging tools in neuromarketing research. Comput. Intell. Neurosci. 2011, 643489. doi:10.1155/2011/643489
Keywords: spatial perception, eye tracking, immersive experience, interior design, neurodesign, neurodiversity, participatory neurodesign, virtual reality
Citation: Kwon J, Linihan S, Iedema A, Schmidt A, Luo C and Marrufo K (2023) How interior design responds to neurodiversity: implementing wearable technologies in neurodesign processes. Front. Built Environ. 9:1211519. doi: 10.3389/fbuil.2023.1211519
Received: 24 April 2023; Accepted: 06 June 2023;
Published: 20 June 2023.
Edited by:
Michael J. Proulx, University of Bath, United KingdomReviewed by:
Jie-Eun Hwang, University of Seoul, Republic of KoreaCopyright © 2023 Kwon, Linihan, Iedema, Schmidt, Luo and Marrufo. This is an open-access article distributed under the terms of the Creative Commons Attribution License (CC BY). The use, distribution or reproduction in other forums is permitted, provided the original author(s) and the copyright owner(s) are credited and that the original publication in this journal is cited, in accordance with accepted academic practice. No use, distribution or reproduction is permitted which does not comply with these terms.
*Correspondence: Jain Kwon, jain.kwon@colostate.edu