- 1Escuela Superior Politécnica del Litoral ESPOL, Facultad de Ingeniería en Ciencias de la Tierra FICT, Guayaquil, Ecuador
- 2Center of Nanotechnology Research and Development (CIDNA), Escuela Superior Politécnica del Litoral (ESPOL), Campus Gustavo Galindo, Guayaquil, Ecuador
- 3Facultad de Ingeniería Mecánica y Ciencias de la Producción, Escuela Superior Politécnica del Litoral (ESPOL), Guayaquil, Ecuador
- 4School of Mechanical Engineering, Purdue University, West Lafayette, IN, United States
- 5Department of Civil and Environmental Engineering, Imperial College, London, United Kingdom
- 6Department of Civil and Environmental Engineering, The Hong Kong University of Science and Technology, Hong Kong, Hong Kong SAR, China
This study compares the seismic performance and environmental impact of a social housing structure designed with reinforced concrete with a structure using Guadua angustifolia “Kunth” cane. The aim is to contrast the implementation of an ecological material such as the Guadua cane, which is an accessible alternative due to its cost and construction time, versus the traditional reinforced concrete (RC) construction method. Both applied to social housing structures. The seismic performance of both methods is analyzed through nonlinear static analysis (pushover) with the objective of establishing the performance; structural and nonstructural damage, performance point, maximum displacements, and structural elements that induce structural failure; and acting forces, against a design earthquake (established by the NEC DS 2015 Standard), with a return period of 475 years. The environmental impact is evaluated through a life cycle assessment of the structure (LCA). Thus, the embodied carbon obtained from each structural element (foundations, beams, columns, floors, and roof support elements) was determined, considering material manufacturing, transportation, and construction. The results obtained demonstrated a higher seismic performance, with 70% less environmental impact on the Guadua cane structure.
1 Introduction
The use of materials such as the Guadua cane is of particular interest in present days since it can be used as a main construction material of social housing, replacing reinforced concrete (RC) and structural steel (SS). Both of these have been widely used for their high strength, availability, and our understanding of their mechanical properties and performance. Extensive literature studies reporting the behavior of RC and SS, stressing their good performance, can be found (Betancourt Rodríguez, 2017), which has led to pertinent comprehensive design standards. However, a major disadvantage of concrete and steel is the environmental impact of their production and use. This has motivated scholars to investigate alternative construction materials that have a lower carbon footprint. An example of such a material is bamboo, which is naturally available in continents such as Asia, Africa, America, and Oceania (Manandhar et al., 2019), with more than 25 species used in construction (Scurlock et al., 2000; Benton, 2015). Bamboo is lighter than concrete, with a high strength-to-mass ratio (Blankendaal et al., 2014). Moreover, it is a low-cost material and a natural resource that can form ready-to-use structural members, resulting in a reduced carbon footprint compared to its traditional counterparts (González and Gutiérrez, 2005).
The construction industry plays a significant role in the worldwide economy, thus requiring a substantial quantity of materials, whose production imposes a high toll on the environment (Koca, 2019). For instance, in the United States, the construction sector is responsible for 41% of the total energy consumption and 38% of the total gas emissions (Basbagill et al., 2013). Furthermore, the 2018 global report on the construction industry showed that buildings are responsible for 36% of the global resource and energy consumption and 39% of the worldwide gas emissions (Morsi et al., 2022).
The adoption of alternative materials such as bamboo at a larger scale shows potential to help mitigate environmental impacts produced by the construction industry. The principal environmental cost of the Guadua cane lies in transporting it (Van der Lugt et al., 2006). For example, maritime transport of Guadua accounts for an environmental impact of 92.9 mPt (millipoints) per kg Guadua, and processing, preservation, and ground transportation of Guadua have impacts of 3.2, 1.6, and 6.5 mPt per kg of Guadua, respectively (Van der Lugt et al., 2006). Therefore, bamboo can be 20 times more favorable to the environment than alternative materials such as concrete or steel, in addition to being less expensive, even when it is not readily available and needs to be imported (Van der Lugt et al., 2006). Furthermore, a study on the life cycle assessment (LCA) of bamboo buildings indicates low environmental impact (30 kg CO2Eq/m2) for both single-story and multi-story buildings, as opposed to concrete or brick buildings (240 kg CO2Eq/m2) (Escamilla et al., 2018). Additionally, in some exceptional cases, such as those of short transport distances, Guadua structures can even have a negative carbon footprint (i.e., reduction of CO2 in the atmosphere) (Escamilla et al., 2018).
An encouraging symptom of the feasibility of bamboo as a structural solution is its ubiquitous use in traditional architecture in areas where it is endemic and abundant. Good examples are bahareque structural systems, which are common in the traditional architecture of many Latin American countries, such as Ecuador and Colombia. Bahareque, or cement–bamboo frame systems, are walls made from bamboo and plaster, which have been adopted for centuries as solutions for low-rise buildings, although their use in construction has been mostly empirical (Alzate and Osorio, 2014).
A recent survey focused on social housing projects in the city of Cuenca, Ecuador, Palacios, and Angumba showed that within a sample of construction professionals of the city, 46% have built houses using the bahareque technique and 84% of the surveyed professionals think that the adoption of bahareque systems for housing of social interest is a feasible alternative because its construction is less time-consuming than that of concrete structures, and based on the performance of historical buildings that use similar construction techniques, the lifespan of bahareque structures can be as long as that of concrete structures (Palacios and Angumba, 2021).
Kakkad compared three structural solutions for a school in India: bahareque (locally known as Ikra), a reinforced brick masonry system, and a confined brick masonry system. The study evaluated the seismic strength and stress via finite element analysis and showed that the seismic strength of the bahareque option was 11.7% higher than that of the confined masonry option and 13% higher than that of the reinforced masonry option (Kakkad, 2011). This study suggests that the higher seismic performance of bahareque could be attributed to its ductility, flexibility, and lightweight.
Similarly, Varum et al. studied the seismic behavior of buildings in Nepal after the Gorkha earthquake, concluding that, despite the fact that constructions with materials such as adobe, bamboo, or wood are scarce in this region, they were found to be the least damaged when compared to reinforced concrete buildings, which sustained severe damage in many cases (Varum et al., 2018).
Mite et al. evaluated a two-story housing solution of social interest built using bahareque, considering particularly the Ecuadorian design regulations. The structure was subjected to a nonlinear time history analysis to evaluate its seismic behavior under 10 different seismic records. The study showed that, for houses of two or fewer stories using structural panels with Guadua canes as diagonals, they did not exceed their maximum resistance and presented deformations that compromised neither the structure nor the nonstructural components (Mite et al., 2022).
In this paper, an evaluation of the performance of a two-story bahareque house, compared to a similar structure made of RC, is studied. The selected structure is a single-family, two-story house of social interest, designed according to the Ecuadorian standard and following international guidelines established in standards such as ACI 318-19, ASCE 7-16, IBC 2018, ASCE 41, and FEMA P58. We compare these two structural solutions in terms of seismic performance, cost, and environmental impact by conducting a nonlinear static analysis (pushover) and a life cycle assessment. Ultimately, the study aims to encourage the adoption of bahareque as a sustainable and sound structural solution by disproving the widespread notion that it is of inferior performance and mechanical characteristics compared to concrete.
2 Methodology
The considered structure is an existing two-story house located in Guayaquil, Ecuador. The structure’s plan geometry and 3D view are presented in Figures 1, 2, respectively. The first-floor level is at 2.43 m height, and the roof level is at 4.75 m height. A 3D model of the building in the structural analysis software program ETABS (CSI, 2019) is also developed here. The seismic demands are considered according to the Ecuadorian seismic structural code (NEC-SE-DS, 2014). The applied gravitational loads comply with the Ecuadorian construction code (NEC-SE-CG, 2015) and include live loads (the dwelling house load is 2 kN/m2, and the roof live load is 0.7 kN/m2). The dead loads are described in Table 1.
First, a spectral analysis was performed for the design of the structural elements. Then, using the design information obtained in the nonlinear static analysis, the equivalent linearization method was adopted, as follows in FEMA-440, 2005. Two cases are examined; in the first, the structure is made of reinforced concrete (RC), and in the second, the same structure is built using the bahareque structural system.
The performance levels of the RC framed and bahareque systems are determined from the performance seismic levels, according to (Vision, 2000), which are shown in Figure 3. The performance points obtained from the nonlinear static analysis are compared in the capacity curve of each model. In addition, the performance level due to the rare earthquake is determined by placing the performance points on both capacity curves, which are divided into sections based on the roof displacement. In this study, the objective is to compare the rare earthquake and the performance point with the building performance level recommended by Vision, 2000. Since this is a residential-type building, it would be expected that, for the aforementioned earthquake, the performance level would not exceed the life safety level.
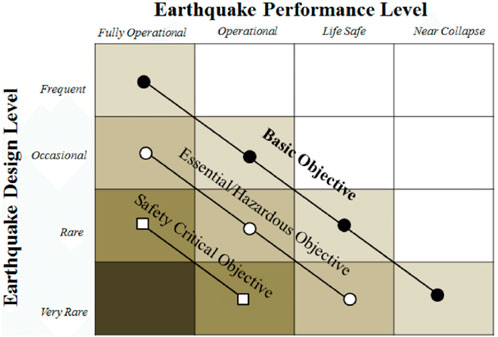
FIGURE 3. Performance objectives for buildings (Vision, 2000).
2.1 Reinforcement concrete frames
For the case of a RC structure, a compressive strength of 21 MPa for the concrete is assumed. In addition, a reinforcing steel structure with a yield strength of 420 MPa is considered. The beams and columns listed in Table 2 are adopted based on typical pre-dimensioning practice and are shown in Figure 4 in a rendered 3D perspective of the structure.
The second moment of area of the cracked section is used to perform the structural analysis, leading to equivalent reduced element stiffness. Structural columns have an inertia reduction of 20%, while beams have an inertia reduction of 50%, as established in NEC-SE-DS, 2014. The design of the reinforced concrete frames that form the superstructure is conducted considering the guidelines established in ACI 318R-19, 2019; NEC-SE-HM, 2017. Here, the load combinations examined are dead load, live load, and seismic load, which are described in NEC-SE-CG, 2015. Since the structure does not have high demands due to its short spans, the dimensions of the elements are particularly small to be designed as special moment-resisting frames (SMFs). Therefore, they are designed as ordinary moment-resisting frames (OMFs). The design of the beams is carried out for bending and shear forces, obtained from the corresponding action envelopes computed from the structural analysis computational package, to establish the necessary steel reinforcement. In addition, the reinforcement ratio on beams must not exceed 1% to keep a high strength/cost relation.
2.1.1 Dynamic spectral analysis
The seismic response spectrum of the RC framed system was defined according to NEC-SE-DS, 2014, for determining the seismic lateral loads, as shown in Figure 5. For this structural system, the response modification factor is R = 3. The mass source considers the totality of the dead load and the base shear reaction is scaled up to 100% of the linear static base shear reaction. Seismic forces are applied in two combinations: the seismic force of X and Y directions. The lateral force of each combination includes the totality of the main lateral force and 30% of the secondary forces, and both are combined by using the SRSS orthogonal direction combination.
2.1.2 Modal analysis
Modal analysis is used to verify that the proposed structure does not exceed the maximum period established by NEC-SE-DS, 2014. Accordingly, the period of the structure should not exceed 30% of that calculated by the following equation:
where Ct = 0.055, α = 0.9, and Hn is the building height. It should be noted that these parameters correspond to the case of RC structures. The periods obtained for the reinforced concrete building are 0.26 s for the first mode in the X direction and 0.25 s for the first mode in the Y direction. The mode shapes are shown in Figure 6.
2.1.3 Nonlinear static analysis
Similarly, the pushover analysis is conducted using ETABS 19 (CSI, 2019). The displacement coefficient method is considered (Naughton et al., 2017), where the structural elements, such as columns and beams, are assumed as ball joints, considering that these should be at 10% of the length of the element (Sullivan et al., 2021). The parameters and configurations used are established according to ACI 318R-19, 2019, with the definition of mechanical properties of the material described previously. The assignment of load patterns, load combinations, reactive mass, dimensions of each structural element, and their corresponding steel reinforcement is described in Table 3. The load is an imposed displacement on the last floor in the y-axis.
2.2 Bahareque
To complete the structural analysis and design of the bahareque structure shown in Figure 7, the material properties of Guadua angustifolia Kunth are defined according to the NEC-SE-GUADUA, 2016 guideline, where the mechanical properties of the material are established. Additionally, correction factors for the resistance considering aspects, such as humidity and structural configuration, of the elements are included. This design decision has also been validated considering standards extracted from the E.100 Bambu, developed in Peru, where the admissible stresses of Guadua cane elements are also established, and from the Andean standard for the design and construction of one- and two-story houses in bahareque. For this study, the minimum elasticity modulus is used for structural analysis and design. Regarding the design process of bamboo elements, allowable stress design (ASD) is used to obtain structural sections. Each stress type has its own allowable stress based on bamboo humidity, as shown in Table 4 (NEC-SE-GUADUA, 2016).
These allowable stress values are modified by factors that consider the size ratio and permissible imperfections in the Guadua cane, among others, which will depend on the structure and the element. Finally, the stress used for the design process is obtained from
where
2.2.1 Dynamic spectral analysis
Dynamic spectral analysis is carried out to obtain the lateral forces. For this study to develop the elastic response spectrum, there are some parameters that are defined. Soil type E is selected due to the location of the project. Additionally, the peak ground acceleration, according to NEC-SE-DS, 2014, is set to 40% of gravity acceleration. To obtain the inelastic response spectrum, the R factor should be defined; this factor is equivalent to the q factor of the Eurocode, and studies report that the q factor of timber structures is around 2 (Bedon et al., 2019) through nonlinear modeling (Bedon et al., 2015); therefore, a response modification factor, R = 2, is chosen for bahareque, considering it as a limited ductility structural system. Furthermore, the elevation and plan regularity modification factors are 1, and the elastic and inelastic response spectra are shown in Figure 8. The mass source is defined according to NEC-SE-DS, 2014, by considering 100% of the total dead load plus 25% of the live load, which consists of the self-weight of the structural elements and the dead load corresponding to nonstructural elements such as walls (entered manually). Live load was not considered in the mass source. After the analysis is completed, the base reaction is compared to the linear static base shear, and the spectrum is scaled to reach 100% of the linear static for the bahareque system base shear reaction.
In the case of seismic forces, the same methodology as in the RC structure was adopted in the bahareque structure.
2.2.2 Modal analysis
The proposed structure in bahareque shall not have a longer period than that proposed in INBAR, 2015, which is calculated by the following equation:
where H is the building height in meters. The obtained mode shapes with their associated frequencies are shown in Figure 9, where the first vibration mode moves 88% of the mass in the x-axis, and the second mode moves 70% in the y-axis.
2.2.3 Nonlinear static analysis
The nonlinear static analysis (pushover) starts by designing the building structure, considering the current guidelines. This analysis is conducted with ETABS, a finite element analysis program, taking into account the mechanical and plastic hinge properties of each element, considering that the beams are governed by moment (M3) and columns by axial load and moment (P − M2 − M3). To represent the inelastic behavior of bamboo, a moment–rotation diagram is defined by a rigid perfectly plastic model. The parameters used are element resistance and a maximum rotation of 0.05 rads, as noted in some experiments developed by Mitch (2010). The assumed element resistances are shown in Table 6.
Yield moments are obtained through the allowable stress design of the section. Regarding columns, these were compared with the experimental results shown in Mitch (2010), which evidence a yield moment of around 34,500 kgf-cm for a four-culm grouted-bar column base, the same configuration used in this study. Regarding beam and diagonal members, the same methodology was used. With the aforementioned information, the plastic hinge was defined according to Figure 10, with the yield moments shown in Table 6.
2.3 Life cycle assessment
Subsequently, this study determines the environmental impact of the structural elements and the usefulness of each construction method with respect to its useful life via a life cycle assessment (LCA). Thus, the life cycle inventory is considered, where the consumption of natural resources plus all the emissions generated while the construction is in progress are quantified. For this purpose, the open-source tool The Structural Carbon Tool Version 2 is used (Madera, 2022). This inventory tool considers 1) the biogenic carbon, which is the amount of sequestered carbon in a particular process; and 2) the product stage, which includes phases A1, related to the supply, extraction, and processing of raw materials; A2, related to external transportation of raw materials to manufacturing plants; and A3, related to the manufacturing processes of final products in the plant. These values are obtained from the environmental declarations of the products of Chilean and Chinese companies, which conducted these studies. The EDP was not found for the case of Ecuadorian companies that produce this raw material, so it was decided to obtain data from countries with similar production conditions. In the case of rebar and A36 steel sections, the EDP used was provided by Aceros (2017), while for the ready-mix concrete (
Stage A4 corresponds to the carbon emission product of the transportation of the material from the point of sale or distribution to the construction site. For our project, a distance of 8 km was considered.
Additional stages C3 and C4 provide information about on-site processing and waste disposal, and stage D includes material reuse and recovery. This information was provided by Gibbons and Orr (2020). Table 7 shows the values assigned to each stage described.
Once the carbon incorporated in each stage has been established, an inventory of each construction method is carried out, where the corresponding structural elements (foundations, frames, slabs, etc.) are determined: the expected lifetime and its mass or volume. The inventory for the reinforced concrete house is presented in Table 8. The inventory obtained for housing in bahareque is shown in Table 9.
3 Results and discussion
With respect to the seismic performance, the inter-story drift is the main parameter to relate the lateral stiffness of both systems. Additionally, inter-story drift could be directly related to the damage of nonstructural elements and repair costs. The structural weight is also related to the seismic lateral force. The results of these parameters are shown in Table 10.
The results presented show that in the short direction (X), the reinforced concrete framed system has a smaller inter-story drift. However, in the long direction (Y), the inter-story drift is smaller on the bahareque system. This is due to the diagonal bamboo elements, which could be an advantage of the bahareque system over the RC system since it is easier to put a diagonal element with bamboo. Diagonal elements increase the lateral stiffness of the bahareque system and improve its response. In addition, the fact that RC is greater in one direction while the bahareque option is more similar in both directions points to a better torsional response (i.e., a more equilibrated stiffness distribution among the two perpendicular directions). The leftover in both cases was the increase in stiffness generated by the nonstructural walls in the structure considered.
The performance levels are evaluated for both cases. For the case of the RC framed system, the results are shown in Figure 11. The earthquake used for this analysis is the 475-year return period (or 10 percent probability of exceedance in 50 years) event. This house is considered an ordinary building, according to Vision, 2000, and this type of structure should be at least within the life safety performance level. The results suggest that this structure complies with the functional performance-based seismic level.
As shown in Figure 12, the seismic performance level is located within the operational zone, which means that this structure complies with the recommendations of Vision, 2000. Thus, results suggest that the bahareque system shows a better seismic performance level than the RC framed system.
A cost comparison between the RC frame and the bahareque house, according to Ecuadorian costs, reveals that for an RC house, which is the most common system used in Ecuador, the total direct cost of the project is US$ 12,045.44, whereas for the case of the bahareque house, the cost reaches US$ 7,660.06. These results clearly show that a bahareque house is approximately 36% cheaper than a traditional RC house.
The displacement in the top vs base shear of three structures is presented in Figure 13. The first structure consists of a timber shear wall that obtained a maximum base shear of 43 kN and an approximate displacement of 160 mm (Bedon et al., 2015), while the other two structures consist of walls made of bamboo frames and diagonals with the difference that our structure consists of two floors, and the third one consists in a vernacular construction of a single floor supporting a roof (Sharma, 2010). The maximum base shears are 17 kN and 4 kN, and displacements are 200 and 150 mm, respectively. In the first case, since the wall is made entirely of wood logs, it has the behavior of a shear wall, so it can withstand greater lateral forces with less displacement, while in the other two, their resistant structures are made of bamboo frames and bracing that lighten the weight of the structure. Therefore, they receive lower lateral forces with higher displacements.
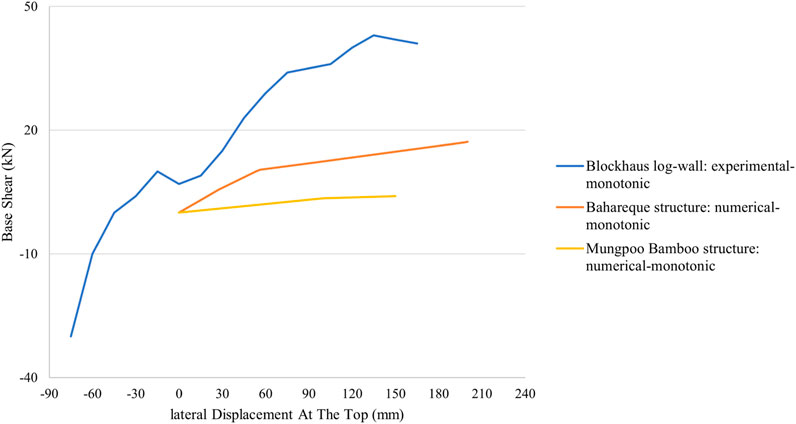
FIGURE 13. Experimental and numerical comparison of the total base shear vs. top displacement for the timber Blockhaus log-wall (Bedon et al., 2015), bahareque structure, and Mungpoo bamboo structure (Sharma, 2010).
3.1 Life cycle
For this analysis, the process was divided into seven phases: the product phase (A1–A3), the construction phase (A4), the material transport phase (C2), the waste disposal phase (C3/C4), and the resource recovery phase (D), as explained previously.
Figure 14 shows that the concrete house has a higher carbon impact than the bahareque, which is driven by the manufacturing processes of the product (A1–A3), the transportation of the material to the site, and waste that can be generated from leftovers (A4–A5). In addition, bahareque houses use materials that can be reused after the useful life of the house, which means that their long-term contamination will have a lower impact than RC housing, where elements such as concrete are not easy to process for reuse.
Figure 15 shows that in terms of the manufacturing and transport of the material that is built, the structural element that causes a greater emission of carbon is the reinforced concrete foundation because both houses have this same material. For the structural elements (beams, columns, and slab), lower carbon emission in the bahareque system is due to the fact that its manufacturing does not depend on industrial processes and the number of trips required is less in addition to the material being significantly lighter than steel and cement.
Carbon incorporated per phase is shown in Figure 16. Due to their manufacturing process (A1–A3), concrete and steel must go through industrial processes to have a better quality, which will generate a greater amount of carbon. When transporting the material to the worksite (A4), it was assumed that the delivery of all the material to the site took place in one trip.
Once the building is built, the waste of concrete over time will emit a larger amount of carbon dioxide as it continues to react over time (C). One of the benefits of bahareque housing is that it can be subject to easy and cheap maintenance of nonstructural elements in comparison to the RC system (Kaminski et al., 2016); nevertheless, one benefit of reinforced concrete housing is that its maintenance is long-term (Palacios and Angumba., 2021).
The most significant biogenic carbon (Bio) of the bahareque system contributes to sequestered carbon dioxide due to its properties.
A comparison of the targets proposed by various organizations for carbon regulation incorporated into buildings is presented in Figure 17.
Both structures have been compared considering the guidelines suggested by LETI and RIBA. The first, called LETI, comprises a group of around 1,000 professionals who are involved in construction and who have proposed reducing carbon emissions in London to zero levels (Initiative, 2020). They have defined a limit in the emission of carbon in residential buildings as a maximum of 201 kgCO2 e/m2. The second organization is the RIBA, an organization formed of volunteers from the United Kingdom who seek to regulate the use of operational energy, water, and carbon. The RIBA proposes setting a challenge for 2030 of a maximum of 144 kgCO2 e/m2 of embodied carbon (RIBA, 2019).
As can be seen, no building exceeds the established maximums. However, a notable difference is observed in the emissions of the two buildings: the emissions corresponding to the bahareque alternative are barely 27% of the emissions of the reinforced concrete structure.
4 Conclusion
This study evaluates the bahareque technique as an alternative, sustainable structural solution. Specifically, the study determines the seismic performance of a two-story social housing structure made of reinforced concrete and compares it with the corresponding performance of a similar structure made of Guadua canes, following the bahareque technique. To that end, the study adopts the nonlinear static (pushover). Additionally, the study performs a life cycle analysis of both structures to determine their environmental impacts.
The nonlinear static analysis yielded the following results: for the reinforced concrete house, a displacement of 63 mm with a base shear force of 304 kN, showing a performance level of life safety, which suggests that there will be structural damage that does not compromise the stability of the structure, safeguarding the life of the occupants, while damage to nonstructural elements such as masonry is expected. For the bahareque house, a displacement of 38 mm due to a force of 7.85 kN was observed. In this case, it is expected that there will be no damage to the main structure or to any nonstructural elements, demonstrating one of the advantages of these structures, which, being lightweight, receive a lower seismic load and also have the capacity to release energy.
The components of the main structure were considered for a life cycle assessment and were analyzed from the following viewpoints: manufacture, transportation of the material to the construction site, quantities of materials, and the design period. The results showed that the reinforced concrete house has a greater amount of embodied carbon, while the Guadua house obtained an A++ rating, certifying that it is an ecological building and that the structural element that showed the greatest environmental impact was its foundation, which is made of RC.
With regard to the budget, all items were considered, corresponding to the construction of the main structure of the designed homes. The result implies that a house of bahareque is 40% less expensive than a house of concrete and that its construction time is approximately half. This would imply savings in labor and a much more efficient start-up.
This research has analyzed one of the most widely used materials in the South American region due to its ancestral applications in construction, which is currently one of the main inputs for the construction of any type of building. However, its potential has not been considered for the construction of low-cost social housing that needs to provide all the necessary services to ensure habitability. As a future direction of research, a parametric analysis is proposed where the mechanical properties of other bamboo specimens existing in the world are considered.
Data availability statement
The original contributions presented in the study are included in the article/Supplementary material; further inquiries can be directed to the corresponding author.
Author contributions
All authors listed have made a substantial, direct, and intellectual contribution to the work and approved it for publication.
Acknowledgments
The support of Eng. Johan Sudario is gratefully acknowledged.
Conflict of interest
The authors declare that the research was conducted in the absence of any commercial or financial relationships that could be construed as a potential conflict of interest.
Publisher’s note
All claims expressed in this article are solely those of the authors and do not necessarily represent those of their affiliated organizations, or those of the publisher, the editors, and the reviewers. Any product that may be evaluated in this article, or claim that may be made by its manufacturer, is not guaranteed or endorsed by the publisher.
References
Aceros, S. A. (2017). Declaración ambiental de Producto barras de Refuerzo acero. Chile: Latin American EPD.
ACI 318R-19 (2019). ACI 318r-19. Building code requirements for structural concrete: An ACI standard; commentary on building code requirements for structural concrete. Farmington Hills, MI: Code, American Concrete Institute.
Alzate, J., and Osorio, J. (2014). Bahareque como ejemplo de sostenibilidad, una herencia que se transforma. Tech. rep.
Basbagill, J., Flager, F., Lepech, M., and Fischer, M. (2013). Application of life-cycle assessment to early stage building design for reduced embodied environmental impacts. Build. Environ. 60, 81–92. doi:10.1016/j.buildenv.2012.11.009
Bedon, C., Rinaldin, G., Fragiacomo, M., and Noé, S. (2019). q-factor estimation for 3d log-house timber buildings via finite element analyses. Soil Dyn. Earthq. Eng. 116, 215–229. doi:10.1016/j.soildyn.2018.09.040
Bedon, C., Rinaldin, G., and Fragiacomo, M. (2015). Non-linear modelling of the in-plane seismic behaviour of timber blockhaus log-walls. Eng. Struct. 91, 112–124. doi:10.1016/j.engstruct.2015.03.002
Benton, A. (2015). “Priority species of bamboo,” in Tropical Forestry. Editors W. Liese, and M. Köhl (Cham: Springer). doi:10.1007/978-3-319-14133-6_2
Betancourt Rodríguez, S. (2017). Materiales para la construcción (Feijóo). Santa Clara, Cuba: Editorial Feijóo.
Blankendaal, T., Schuur, P., and Voordijk, H. (2014). Reducing the environmental impact of concrete and asphalt: A scenario approach. J. Clean. Prod. 66, 27–36. doi:10.1016/j.jclepro.2013.10.012
CSI (2019). Etabs 19 [Software]. Retrieved from: https://www.csiespana.com/software/5/etabs.
Dasso Group (2020). Environmental product declaration of bamboo. Xinhe, Linpu, Xiaoshan, Hangzhou, Zhejiang, China: The International EPD® System, 1–10.
Escamilla, E., Habert, G., Daza, J., Archilla, H., Echeverry, J., and Trujillo, D. (2018). Industrial or traditional bamboo construction? Comparative life cycle assessment (LCA) of bamboo-based buildings. Sustain. Switz. 10, 3096. doi:10.3390/su10093096
FEMA-440 (2005). FEMA 440. Improvement of nonlinear static seismic analysis procedures. Redwood City, CA: Report, Applied Technology Council.
González, G., and Gutiérrez, J. (2005). Structural performance of bamboo ’bahareque’ walls under cyclic load. J. Bamboo Rattan 4, 353–368. doi:10.1163/156915905775008345
INBAR (2015). “Norma Andina para diseño y construcción de casas de uno y dos pisos en bahareque encementado (INBAR),” in Red Internacional para el Desarrollo del Bambú y el Ratán Ofcina para América Latina y el Caribe - inbar (Quito, Ecuador: Red Internacional para el Desarrollo del Bambú y el Ratán Ofcina para América Latina y el Caribe).
Initiative, L. E. T. (2020). Leti climate emergency design guide: How new buildings can meet UK climate change targets. London, UK: LETI.
Kakkad, M. D. (2011). “Comparative study of bamboo (Ikra) housing system with modern construction practices,” in 4th national conference on innovations in Indian science, engineering and technology (New Delhi: CSIR – National Physical Laboratory NPL).
Kaminski, S., Lawrence, A., Coates, K., and Foulkes, L. (2016). A low-cost vernacular improved housing design. Proc. Institution Civ. Eng. - Civ. Eng. 169, 25–31. doi:10.1680/jcien.15.00041
Koca, G. (2019). Evaluation of traditional sirince houses according to sustainable construction principles. Eval. Traditional Şirince Houses Accord. Sustain. Constr. Princ. 7, 30–49. doi:10.15320/iconarp.2019.65
Manandhar, R., Kim, J. H., and Kim, J. T. (2019). Environmental, social and economic sustainability of bamboo and bamboo-based construction materials in buildings. J. Asian Archit. Build. Eng. 18, 49–59. doi:10.1080/13467581.2019.1595629
Mitch, D. R. (2010). Structural behavior of grouted-bar bamboo column bases. Ph.D. thesis. Pittsburgh: University of Pittsburgh.
Mite, F., Tello, K., García, N., Silva, C. E., Malaga, C., Arévalo, K., et al. (2022). Structural behavior of cemented bahareque for social housing: A case study in Guayaquil city, Ecuador. Front. Built Environ. 8, 922397. doi:10.3389/fbuil.2022.922397
Morsi, D., Ismaeel, W., Ehab, A., and Othman, A. (2022). BIM-based life cycle assessment for different structural system scenarios of a residential building. Ain Shams Eng. J. 13, 101802. doi:10.1016/j.asej.2022.101802
Naughton, D. T., Tsavdaridis, K. D., Maraveas, C., and Nicolaou, A. (2017). Pushover analysis of steel seismic resistant frames with reduced web section and reduced beam section connections. Front. Built Environ. 3, 59. doi:10.3389/fbuil.2017.00059
NEC-SE-CG (2015). Cargas (no sísmicas). Retrieved from: https://www.habitatyvivienda.gob.ec/documentos-normativos-nec-norma-ecuatori ana-de-la-construccion/.
NEC-SE-DS (2014). Peligro sísmico. diseño sismo resistente. Retrieved from: https://www.habitatyvivienda.gob.ec/documentos-normativos-nec-norma-ecuatori ana-de-la-construccion/.
NEC-SE-GUADUA (2016). Estructuras de guadua. Retrieved from: https://www.habitatyvivienda.gob.ec/documentos-normativos-nec-norma-ecuatori ana-de-la-construccion/.
NEC-SE-HM (2017). Estructuras de hormigon armado. Retrieved from: https://www.habitatyvivienda.gob.ec/documentos-normativos-nec-norma-ecuatori ana-de-la-construccion/.
Palacios, A., and Angumba, P. (2021). Bahareque as a sustainable construction system: Analysis of unit prices. IOP Conf. Ser. Mater. Sci. Eng. 1203, 032118. doi:10.1088/1757-899x/1203/3/032118
Scurlock, J., Dayton, D., and Hames, B. (2000). Bamboo: An overlooked biomass resource? Biomass Bioenergy 19, 229–244. doi:10.1016/S0961-9534(00)00038-6
Sharma, B. (2010). Seismic performance of bamboo structures. Ph.D. thesis. Pittsburgh: University of Pittsburgh.
Sullivan, T. J., Saborio-Romano, D., O’Reilly, G. J., Welch, D. P., and Landi, L. (2021). Simplified pushover analysis of moment resisting frame structures. J. Earthq. Eng. 25, 621–648. doi:10.1080/13632469.2018.1528911
Van der Lugt, P., Van den Dobbelsteen, A., and Janssen, J. (2006). An environmental, economic and practical assessment of bamboo as a building material for supporting structures. Constr. Build. Mater. 20, 648–656. doi:10.1016/j.conbuildmat.2005.02.023
Varum, H., Dumaru, R., Furtado, A., Barbosa, A. R., Gautam, D., and Rodrigues, H. (2018). Seismic performance of buildings in Nepal after the Gorkha earthquake. Elsevier. doi:10.1016/B978-0-12-812808-4.00003-1
Keywords: social housing, nonlinear static analysis (pushover), reinforced concrete, Guadua cane, life cycle analysis
Citation: Tello-Ayala K, Garcia-Troncoso N, Silva CE, Zúñiga-Olvera C, Narvaez-Moran J, Malaga-Chuquitaype C and Mouka T (2023) Comparative analysis of the sustainability and seismic performance of a social interest house using RC moment frames and bahareque as structural systems. Front. Built Environ. 9:1150826. doi: 10.3389/fbuil.2023.1150826
Received: 25 January 2023; Accepted: 27 June 2023;
Published: 12 July 2023.
Edited by:
Aliakbar Gholampour, Flinders University, AustraliaReviewed by:
Cristoforo Demartino, International Campus, Zhejiang University, ChinaChiara Bedon, University of Trieste, Italy
Copyright © 2023 Tello-Ayala, Garcia-Troncoso, Silva, Zúñiga-Olvera, Narvaez-Moran, Malaga-Chuquitaype and Mouka. This is an open-access article distributed under the terms of the Creative Commons Attribution License (CC BY). The use, distribution or reproduction in other forums is permitted, provided the original author(s) and the copyright owner(s) are credited and that the original publication in this journal is cited, in accordance with accepted academic practice. No use, distribution or reproduction is permitted which does not comply with these terms.
*Correspondence: Natividad Garcia-Troncoso, bmxnYXJjaWFAZXNwb2wuZWR1LmVj