- 1Architecture Department, The British University in Egypt, Cairo, Egypt
- 2Center for Advanced Materials (CAM), The British University in Egypt, Cairo, Egypt
Nowadays, energy consumption is a global challenge due to the shortage of some energy sources and, consequently, their increasing prices. This paper argues that by using an integrative approach for using various energy harvesting techniques, a typical residential neighborhood can be operated as a self-generator of some or all of its needed energy. The paper follows mixed qualitative, experimental, and numerical simulation approaches to examine the practicality of the hypothesis. It uses Space syntax modeling theory (socio-spatial software) to analyze the pedestrian flow to indicate potential locations of kinetic energy harvesting piezoelectric tiles in the selected residential neighborhood. In addition, the possibility of installing photovoltaic electrical cells on rooftops of the neighborhood residential blocks is studied. The numerical simulation quantifies the capacity of the harvested energy to achieve a model of a green neighborhood. The paper measures the percentage of the residences’ energy needs that can be harvested using renewable energy resources. It reveals that 30% of the energy consumed in the residential blocks can be generated from photovoltaic electrical cells, and 38% of the energy consumed by street lighting of the entire neighborhood could be generated using the kinetic energy harvested by piezoelectric tiles.
1 Introduction
Due to global climate change, increased energy consumption by residences is a burden on cities’ consumable sources of energy. Hence, most cities have started to use other sources of renewable/non-consumable energy, such as solar, wind, hydro, and tidal, as well as energy harvesting technologies.
Recently, many countries are shifting to renewable energy sources, including active, passive, hybrid, and smart techniques for energy harvesting. Photovoltaic (PV) systems and wind power plants are examples of renewable energy, while piezoelectric tiles, triboelectric energy harvesting, and electromagnetic energy harvesting tiles are examples of energy harvesting technologies that depend on kinetic energy.
In alignment with the notion that a residential neighborhood is a self-sufficient community, this research considers the socio-spatial aspect of residential neighborhoods as a source of harvesting energy. Consequently, the neighborhood can be considered as an energy-generating community.
Many scholarly sources have studied the neighborhood in the Egyptian context in terms of its social sustainability, such as Ghonimi and Awaad (2018), Ghonimi (2021), and Azzam AO (2017). Other publications are adopting demographics, gender, education, or social class as a core of their papers, such as Royal (1985) and Mowafi et al. (2012). Other researchers covered the sustainable urban dimension of neighborhoods in Egypt, such as being green. This research paper is the first to introduce a practical approach on a scale of a residential community (rather than individual houses) to be operated as a self-generator of energy consumed in the Egyptian context.
2 Residential neighborhoods
Residential neighborhoods, as a part of the overall urban system of any city, have inclusive characteristics, such as sociability, livability, and safety (Ben-Joseph, 1995). The concept of residential neighborhoods was generated in the early 19th century by Clarence Perry as a developed planning unit to create small communities where self-sufficiency is considered in terms of functional and social needs. Literature studies included many definitions, concepts, and developing ideas on residential neighborhoods in terms of planning, size, structure, form, and components (Allen, 2018).
Many scholars and advocates promoted the idea of the neighborhood with various approaches to how the residential neighborhood is planned and perceived in terms of type, size, social mix, and location, among other aspects. Most studies promoted the safety of the neighborhood as a main principle of design reflected in its activities and walkability as a main mood of transportation rather than vehicles (Carmona et al., 2003), (Talen, 2000).
The size of the residential neighborhood was debated by Kallus and Law-Yone, who argued that it is “more than a single less than an entire city” (Kallus and Law-Yone, 1997). This argument increases the size factor from a very small to a very large urban entity. They described it as an independent urban system, which indicates its inclusiveness and self-sufficiency. The residential neighborhood was classified as a socio-spatial system or as a functional relationship by Chaskin (1997).
The new strategy for sustainable neighborhood planning was discussed and published by the UN Habitat (2014). It promotes and encourages walkability as one of the key features of sustainable neighborhoods (Hu et al., 2022). The strategy aims to create healthy communities by reclaiming the social dimension in a neighborhood’s streets and places for public gatherings.
3 Energy harvesting technologies
The drawbacks of the intensive use of fossil fuels for energy generation are now a well-defined problem and a real global threat. Moreover, the exponentially increasing demand for electrical energy dictates the use of clean, renewable energy sources. Energy harvesting, also known as energy scavenging, is the process by which energy is absorbed and stored from renewable sources such as solar, kinetic, wind, and thermal energy and salinity gradients and sea waves. Energy harvesters can be solar panels, wind turbines, thermoelectric generators, or energy-scavenging tiles.
3.1 Kinetic energy
One promising harvesting technology is kinetic energy, which absorbs the energy generated from pedestrian footsteps or vehicles and transforms it into electrical energy. The phenomenon behind kinetic energy scavenging can be electromagnetic (Invernizzi et al., 2016; Anjum et al., 2018), piezoelectric, or triboelectric (Alluri et al., 2020a; 2020b). Some researchers have investigated energy scavenging from human movement or even heartbeat and respiration to charge wearable low-power devices (Khalid et al., 2019). The technology adopted in this paper emerged from the piezoelectric phenomenon (PZT). The first observation of PZT was by Pierre and Jacques Curie when they found clear electrical energy properties in a single quartz gem in 1880. Later, they proved that crystals of tourmaline, topaz quartz, and Rochelle salt generate electrical polarization under mechanical stress. Other materials were recently found to have the same phenomenon, such as natural quartz, polycrystalline piezo-ceramic, semi-crystalline polyvinylidene polymer, and even aloe vera (Visconti et al., 2022). The commonality between these materials is that the polarization vector changes under mechanical loads. This is attributed to charge separation in the atoms when they move apart due to mechanical stress, allowing the crystal to conduct an electrical current. This physical property is shown in Figure 1.
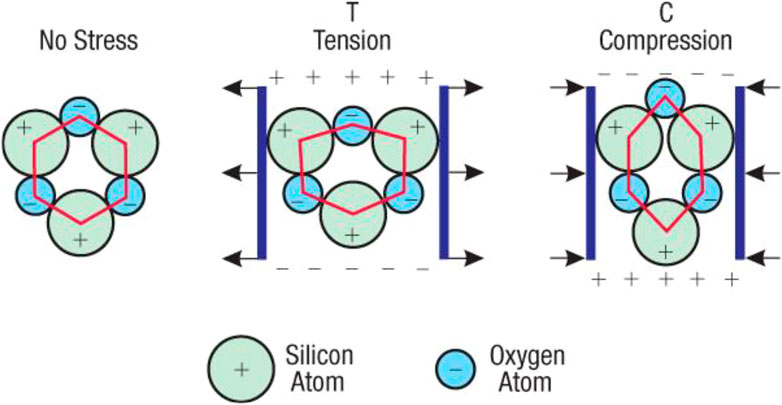
FIGURE 1. Piezoelectric effect in quartz. Source: https://components101.com/articles/how-does-a-piezoelectric-generator-works-types-and-characteristics.
The concept of generating electricity from human motion depends on applying pressure on a PZT batch during human activities to generate an electric charge. Various companies have adopted the idea and developed different tiles. One promising product was developed in 2011 by Waydep under the commercial name of Waynergy (C. Wang et al., 2018). Other products, such as Piezma Piezoelectric tiles and Sustainable Energy Floor (SEF), are also available in the market.
3.2 Solar energy
The second renewable energy resource exploited in this article is solar energy using photovoltaic cells. Solar energy collection using photovoltaic cells is a well-established technology widely used in many countries. The photovoltaic effect is a process in which a light-sensitive semiconductor converts visible light (sunlight) into voltage. This action occurs in all semiconductors that are constructed to absorb energy. Figure 2 shows the physical phenomenon behind the photovoltaic cells.
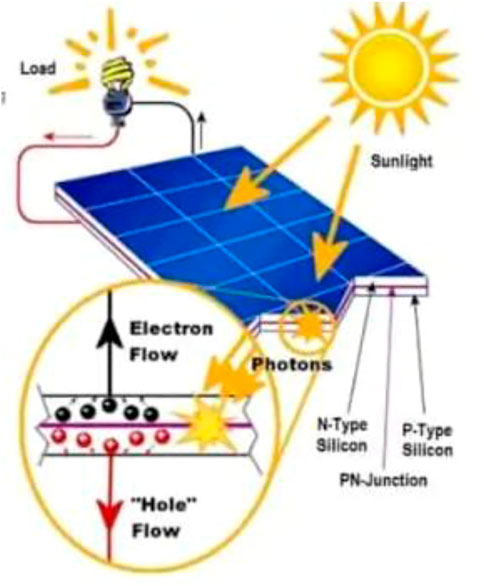
FIGURE 2. Photovoltaic effect. Source: https://www.electricaltechnology.org/2015/06/how-to-make-a-solar-cell-photovoltaic-cell.html.
4 The case study
As residential quarters or neighborhoods have neither a specific population size nor a particular socio-economic class (Choguill, 2008), the research selected an average size residential neighborhood for 6000 residences in the El Banafseg District in New Cairo that is marked in red in Figure 3:1. The selected neighborhood is considered a repetitive pattern in its district, where plots of land follow almost the same plan and layout for sites and services. Hence, the selected residential neighborhood is considered a prototype that can be used in wider areas that share the same climatical conditions.
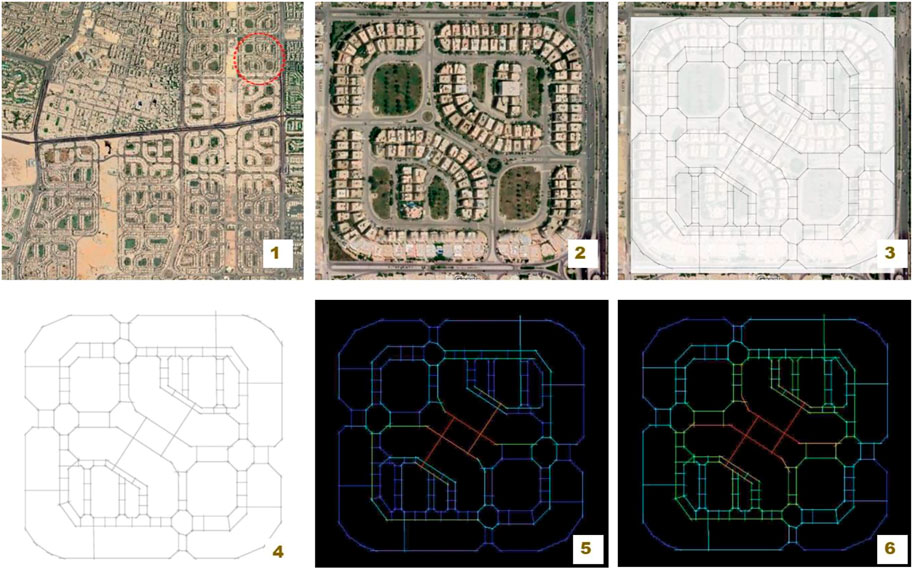
FIGURE 3. Process of the neighborhood selection and analysis of the pedestrian socio-spatial movement using depthMapX software by the authors.
5 Measuring the potential locations of energy harvesting sources
5.1 The method
depthMapX, a software program integrated with the Space syntax theory, was used to measure the flow of residents and patterns of movement in the selected residential neighborhood. Space syntax is a social theory of spaces founded by Bill Hillier and colleagues that uses the spatial system as the “logic of a society” (Karimi, 2018). Bill Hillier explains that “the configuration of the space network is, in and of itself, a primary shaper of the pattern of movement” (Hillier, 2008). Space syntax analyses and measures people’s movement, activities, and usage of a space through a simple geometrical analysis. The theory deals with many spatial factors, such as human behavior, visual perception, and patterns of movement, among others (Hillier et al., 1993). Space syntax holistically reads, gathers, and analyzes spaces to identify the traveling paths, including human movements and pathways. It displays the analyzed information through integrated lines (Seamon, 2015).
Figure 3:3 shows the tracing of all possible pedestrian movement trails within the territory of the selected neighborhood, drawn as vector lines using AutoCAD software. The movement trails are analyzed by the depthMapX software that uses the socio-spatial aspect to indicate various levels of pedestrian movements, from heavy movement to medium-high, medium-low, and low movements. Figures 3:5 and 6 show the diagram of all potential pedestrian sidewalks in the selected neighborhood analyzed and categorized into high, medium-high, medium-low, and low intensities of movement. Pedestrian movement levels are indicated in different color codes by the software. The research numerical simulation part is guided by this coding.
5.2 Intensity of users for the trails provided with PZT tiles
The studied neighborhood of El Banafseg comprises 200 building blocks. Each block is G+2 with two residential units per floor. As a moderate-class inhabitant area, it is assumed that, on average, one family of five people occupies each unit, which makes the total number of inhabitants around 6000 people. As a new settlement, it is expected that the occupants are both children and people of middle age. Consequently, it is supposed that most inhabitants will walk in the area.
According to the diagram exported from depthMapX, three traffic zones could be implemented for kinetic energy harvesting technology using PZT tiles. As shown in the integrated map (Figure 3:6), three zones of high to medium traffic capacity are selected to be implemented in the study: the red, orange, and yellow zones. The high flow of pedestrians is in red, the medium-high flow is in orange, and the medium-low traffic is in yellow. The research postulates that the intensity of the inhabitants who will use the high-traffic trails is 90%, and intensities of 80% and 40% are assigned for the medium-high flow trails and for the medium-low trails, respectively. To calculate the energy harvested from the tiles, the total length of each path category is calculated using the Integration Map (Figure 3:6).
The lengths of the three trail categories are as follows:
5.3 Kinetic energy harvesting using PZT tiles
The energy harvesting tiles employed in this study are the “Waynergy Floors” produced by the Waydip Company. This type is selected as it is suitable for applications in outdoor areas such as crosswalks and sidewalks, and it has the ability to sell the generated electrical power to the governmental grid (Elhalwagy et al., 2017). The usual application of this type of tile is for illuminating roads and traffic light control. The dimensions of the tiles are 40 × 40 cm. Therefore, 10 tiles can be installed for every 2 lineal meters of path. Each tile generates ≈4 W per step in a pulse that takes 0.18 s for a pedestrian with an average weight of 75 kg and normal pressure, as shown in Figure 4 (Duarte et al., 2013).
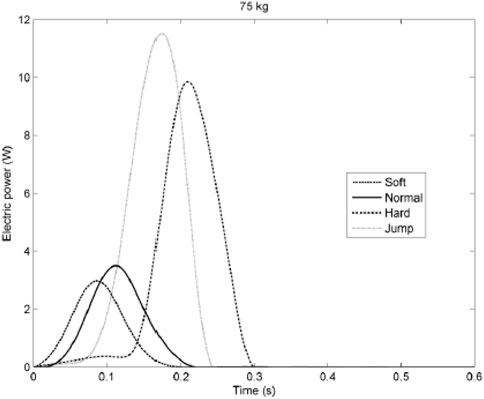
FIGURE 4. Electric power harvested by a 75 kg person using Waynergy tiles (Duarte et al., 2013).
It is assumed that the tiles should be installed on the centerline of the path to ensure pressure during the walking activity.
For conservative calculations, it is assumed that the pedestrian will walk in a linear manner such that a tile may not be pressed twice at a certain instance. Furthermore, the human step (stride) is about 45 cm (≈1.5 ft), and the human activity will last for 10 h per day. To calculate the energy harvested from human strides on these tiles, the following equation is derived by the authors:
Energy harvested per kWh/day = number of pedestrians × number of steps per 1 m of trail per person × number of tiles per meter × energy harvested per step × time of one step × length of the trail × number of hours/(3600*1000).
Considering that
Therefore, the energy harvested from pedestrians during walking activity per day in all zones is calculated as follows.
• Red zone harvested kinetic energy = 0.9 × 6000 × (1/0.45) × (1/0.4) × 4 × 0.18 × 534.8 × 10/(3600 × 1000) = 32.088 kWh/day
• Orange zone harvested kinetic energy = 0.8 × 6000 × (1/0.45) × (1/0.4) × 4 × 0.18 × 241.885 × 10/1000 = 12.9 kWh/day
• Yellow zone harvested kinetic energy = 0.4 × 6000 × (1/0.45) × (1/0.4) × 4 × 0.18 × 3838/1000 = 10.23 kWh/day
The total energy harvested from pedestrians during the daily walking activity in all zones = 55.218 kW h/day.
6 Energy required to illuminate the walking trails
There is a relationship between the height of street lighting poles and the width of the street. Furthermore, the distance between street lighting poles is typically calculated based on lighting pole height and is often 2.5–3 times that height. As per the Global Street Design Guide, a pedestrian walkway should have a pedestrian light that is between 4.5 and 6 m in height. Hence, the average distance between pedestrian lighting poles should be 13 m. As shown in Figure 5, the height and spacing of light poles depend on the street width.
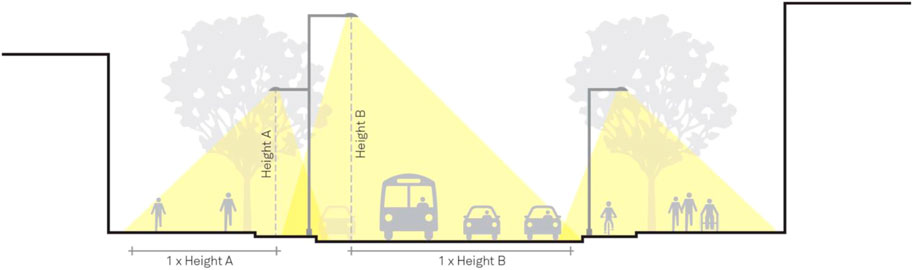
FIGURE 5. Relationship between street lighting poles and the width of the street. Source: Global Street Design Guide.1
A 30 W lamp pole spaced every 13 m can be used to calculate the energy required to illuminate the walking trails. The working hours are 12 h. First, the number of poles in the neighborhood is calculated as follows:
The energy harvested by the PZT tiles can provide about 38% of the energy required to illuminate the walking trails, as shown in Figure 6.
7 Generating energy using PV cells
The other renewable green energy resource implemented in this case study is photovoltaic solar panels. Per local regulations, 50% of the roof area is reserved for constructing a light structure. Furthermore, about 5 m × 2.5 m are reserved for staircase covering. Taking into account the shadow area of both the lightweight structure and the staircase covering, the allowable area for installation of solar panels is 8 m × 14 m, which yields about 112 m2. To calculate the energy harvested from the installation of the PV cells, the online tool developed by the Center for Emerged Learning Technologies (CELT) hosted by the British University in Egypt is implemented. The tool adopts the climate condition of Cairo and assumes that the installed PV system is a grid-connected system. The tool is available at the following link: https://www.celt-bue.com/apps/#/pvSizingOnlineTool. The tool reports that for a residential building in Cairo, each 10 m2 of roof can produce 1 kWh if all solar panels are facing south at a 30° inclination. The sun’s illumination distribution follows a Gaussian distribution where the illumination starts weak and increases as the sun becomes brighter and then fades out at sunset (Han et al., 2015). For calculations, it is assumed that this Gaussian distribution can be replaced by a uniform sun illumination referred to as peak sun hour (PSH). In Egypt, the PSH value is 5 h.
For buildings that have roofs aligned in a purely north-south direction, the amount of energy produced from the available area for PV cells is as follows:
For other buildings that make angles with north, the area available for the installation of the PV cells is governed by the angle of the building to north. Referring to Figure 3:2, the average angle of these buildings with north is 32°. Therefore, the area available for the PV cells for this group of buildings can be calculated as follows:
Referring to Figure 3:2, the number of buildings in the studied neighborhood is 200; 132 buildings are aligned in the north-south direction, and 68 buildings make an average angle of 32° with north. Therefore, the total energy harvested from the PV cells installed on the roofs of the whole neighborhood building blocks can be calculated as follows:
Total energy harvested using the PV cells installed on the roofs = 56 × 132 + 40.27 × 68 = 10,130.36 kWh/day. The harvested energy can be sold to the governmental network to avoid the installation of storage batteries. The efficiency of the batteries usually affects the amount of useful energy.
7.1 Energy consumption in a typical residential neighborhood
The energy consumed by a residential neighborhood depends on many factors, such as its social class, which identifies the number of family members in each unit and, consequently, the amount of energy consumed.
According to the Annual Report of the Electricity Company in Egypt 2020/2021 posted on the official website of the Egyptian Ministry of Electricity and Renewable Energy,3 the energy consumed per person per year on average is 2000 kWh (Figure 7).
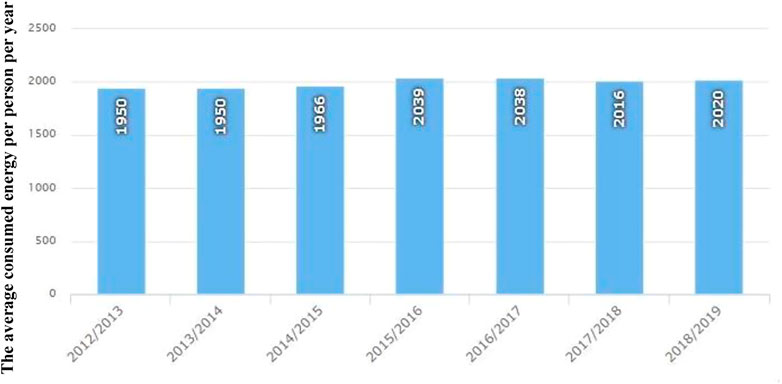
FIGURE 7. Average consumed energy per person per year in Egypt. Source: The Annual Report of the Electricity Company in Egypt 2020/2021.2
Therefore, the consumption of the selected neighborhood in El Banafseg per day is 2000 kWh/365 day × 6000 residences = 32,876.7 kW h/day.
Therefore, the energy harvested by the PV cells can provide about 30% of the energy consumed by all of the neighborhood residences (Figure 8).
8 Conclusion
The research focused on the human dimension, the main driver of any residential neighborhood, as a source of energy generation in relation to energy consumption. It utilized the qualities and components of typical residential neighborhoods as energy generators. Thus, neighborhoods’ physical aspects, namely, rooftops of building blocks and walkways, as well as their non-physical aspects, such as residents’ socio-spatial activities, were employed as potentials for energy harvesting.
The research used software that links human activities with the spatial dimension of places to predict the flow capacity as a guiding tool to calculate the potential trails for the kinetic energy.
The research exploited two types of renewable energy resources, kinetic and solar energy, to create a green neighborhood. Piezoelectric tiles installed in the busiest walking trails can generate energy that can illuminate neighborhood lighting poles. Rooftop photovoltaic electric cells can be implemented to generate electricity from solar energy. The research shows that about 38% of the power required by neighborhood lighting poles can be generated using kinetic energy, while 30% of the energy consumed by the neighborhood residences could be generated from solar energy collected from rooftop PV systems.
Data availability statement
The original contributions presented in the study are included in the article/Supplementary Material; further inquiries can be directed to the corresponding author.
Author contributions
AM and MH contributed to the conception, structure, and design of the research. AM contributed to the energy harvesting sections, including calculations. MH developed the urban design sections, including the spatial analysis. All authors listed have made a substantial, direct, and intellectual contribution to the work and approved it for publication.
Funding
The funding of this research paper was partially provided by the British University in Egypt (up to $1000) upon final acceptance of the manuscript.
Acknowledgments
The authors acknowledge the effort and the expertise of Professor Husam Bakr at the Architecture Department, The British University in Egypt, in the work carried out using depthMapX software. The completion of the spatial analytical part of the research would not have been possible without his support.
Conflict of interest
The authors declare that the research was conducted in the absence of any commercial or financial relationships that could be construed as a potential conflict of interest.
Publisher’s note
All claims expressed in this article are solely those of the authors and do not necessarily represent those of their affiliated organizations, or those of the publisher, the editors, and the reviewers. Any product that may be evaluated in this article, or claim that may be made by its manufacturer, is not guaranteed or endorsed by the publisher.
Footnotes
1Global Street Design Guide: Can be downloaded from https://globaldesigningcities.org/publication/global-street-design-guide/utilities-and-infrastructure/lighting-and-technology/lighting-design-guidance/
2Annual Report of the Electricity Company in Egypt 2020/2021 http://www.moee.gov.e.g.,/test_new/PDFReports/2020-2021-AR.pdf
3The official website of the Egyptian Ministry of Electricity and Renewable Energy. http://www.moee.gov.eg/test_new/ST_consumption.aspx
References
Allen, N. (2018). Concepts of neighbourhood: A review of the literature building better homes towns and cities national science challenge working paper 18-02 title concepts of neighbourhood: A review of the literature working paper 18-02. Technical report. doi:10.13140/RG.2.2.36482.61123
Alluri, N. R., Maria Joseph Raj, N. P., Khandelwal, G., Vivekananthan, V., and Kim, S. (2020b). Aloe vera: A tropical desert plant to harness the mechanical energy by triboelectric and piezoelectric approaches. N Energy 73, 104767. doi:10.1016/j.nanoen.2020.104767
Alluri, N. R., Raj, N., Khandelwal, G., Vivekananthan, V., and Kim, S. (2020a). “Triboelectric nanogenerators: Design, fabrication, energy harvesting, and portable-wearable applications,” in Nanogenerators. Editors S. Jae Kim, A. Chandrasekhar, and N. Rao Alluri (IntechOpen). doi:10.5772/intechopen.78915
Anjum, M. U., Fida, A., Ahmad, I., and Iftikhar, A. (2018). A broadband electromagnetic type energy harvester for smart sensor devices in biomedical applications. Sensors Actuators, A Phys. 277, 52–59. doi:10.1016/j.sna.2018.05.001
Azzam, A. (2017). Socially sustainable neighborhoods: Special reference to the Egyptian context. Master’s thesis. AUC Knowledge Fountain. https://fount.aucegypt.edu/etds/182.
Ben-Joseph, E. (1995). Residential street standards and neighborhood traffic control. Berkeley, CA: University of California.
Carmona, M., Heath, T., Oc, T., and Tiesdell, S. (2003). Urban spaces-public places: The dimensions of urban design. Oxford, UK: Architectural Press.
Chaskin, R. (1997). Perspectives on neighborhood and community: A review of the literature. Soc. Serv. Rev. 71 (4), 521–547. doi:10.1086/604277
Choguill, C. L. (2008). Developing sustainable neighbourhoods. Habitat Int. 32, 41–48. doi:10.1016/j.habitatint.2007.06.007
Duarte, F., Casimiro, F., Correia, D., Mendes, R., and Ferreira, A. (2013). “A new pavement energy harvest system,” in 2013 International Renewable and Sustainable Energy Conference (IRSEC), Ouarzazate, Morocco, Ouarzazate, March 7-9, 2013, 408–413.
Elhalwagy, A. A., Ghoneem, M. Y. M., and Elhadidi, M. (2017). Feasibility study for using piezoelectric energy harvesting floor in buildings’ interior spaces. Energy Procedia 115, 114–126. doi:10.1016/j.egypro.2017.05.012
Ghonimi, I. G. (2021). The role of urban space configuration in maintaining social inclusion: A comparison of four types of neighborhoods in greater cairo region-egypt. J. Urban Res. 39 (1), 75–104. doi:10.21608/jur.2021.30232.1002
Ghonimi, I., and Awaad, A. (2018). Socially sustainable neighborhoods in Egypt: Assessing social capital for different neighborhood models in Greater Cairo Region. JES. J. Eng. Sci. 46 (2), 160–180. doi:10.21608/jesaun.2018.114463
Habitat, U.N. (2014). A new strategy of sustainable neighborhood planning: Five principles. Available at: https://mirror.unhabitat.org/pmss/listItemDetails.aspx?publicationID=3518 (Accessed December 12, 2022).
Han, X., Xu, C., Ju, X., Du, X., and Yang, Y. (2015). Energy analysis of a hybrid solar concentrating photovoltaic/concentrating solar power (CPV/CSP) system. Sci. Bull. 60, 460–469. doi:10.1007/s11434-015-0738-7
Hillier, B., Penn, A., Hanson, J., Grajewski, T., Xu, J., et al. (1993). Natural movement: Configuration and attraction in urban pedestrian movement. Environ. Plan. B Plan. Des. 20, 29–66. doi:10.1068/b200029
Hillier, B. (2008). “The new science of space and the art of place,” in New urbanism and beyond. Editor T. Haas (New York, NY: Rizzoli).
Hu, H. B., Hou, Z. H., Huang, C. H., LaMonte, M. J., Wang, M., and Lu, B. (2022). Associations of exposure to residential green space and neighborhood walkability with coronary atherosclerosis in Chinese adults. Environ. Pollut. 292, 118347. doi:10.1016/j.envpol.2021.118347
Invernizzi, F., Dulio, S., Patrini, M., Guizzetti, G., and Mustarelli, P. (2016). Energy harvesting from human motion: Materials and techniques. Chem. Soc. Rev. 45 (20), 5455–5473. doi:10.1039/C5CS00812C
Kallus, R., and Law-Yone, H. (1997). Neighbourhood – the metamorphosis of an idea. J. Archit. Plan. Res. 14 (2), 107–125.
Karimi, K. (2018). Space syntax: Consolidation and transformation of an urban research field. J. Urban Des. 23 (1), 1–4. doi:10.1080/13574809.2018.1403177
Khalid, S., Raouf, I., Khan, A., Kim, N., and Kim, H. S. (2019). A review of human-powered energy harvesting for smart electronics: Recent progress and challenges. Int. J. Precis. Eng. Manufacturing-Green Technol. 6, 821–851. doi:10.1007/s40684-019-00144-y
Mowafi, M., Khadr, Z., Bennett, G., Hill, A., Kawachi, I., and Subramanian, S. V. (2012). Is access to neighborhood green space associated with bmi among Egyptians? A multilevel study of Cairo neighborhoods. Health and place 18 (2), 385–390. doi:10.1016/j.healthplace.2011.12.002
Royal, A. M. (1985). Male/female pharyngealization patterns in Cairo Arabic: A sociolinguistic study of two neighborhoods (Egypt, acoustics, women, ethnography). University of Texas at Austin.
Seamon, D. (2015). Understanding place holistically: Cities, synergistic relationality, and space syntax. Joss, Bartlett 6 (1), 19–33.
Talen, E. (2000). The problem with community in planning. J. Plan. Literature 15 (2), 171–183. doi:10.1177/08854120022092971
Visconti, P., Bagordo, L., Velázquez, R., Cafagna, D., and De Fazio, R. (2022). Available technologies and commercial devices to harvest energy by human trampling in smart flooring systems: A review. Energies 15, 432. doi:10.3390/en15020432
Keywords: residential neighborhood, renewable energy, energy harvesting technologies, photovoltaic cells, piezoelectric tiles
Citation: Helmy M and Micheal A (2023) The neighborhood as an energy-generating community: the case of the El Banafseg residential neighborhood, East Cairo. Front. Built Environ. 9:1146471. doi: 10.3389/fbuil.2023.1146471
Received: 17 January 2023; Accepted: 02 May 2023;
Published: 18 May 2023.
Edited by:
Ayman Mahmoud, Cairo University, EgyptReviewed by:
Bekkay Hajji, Mohamed Premier University, MoroccoAndrea Frattolillo, University of Cagliari, Italy
Copyright © 2023 Helmy and Micheal. This is an open-access article distributed under the terms of the Creative Commons Attribution License (CC BY). The use, distribution or reproduction in other forums is permitted, provided the original author(s) and the copyright owner(s) are credited and that the original publication in this journal is cited, in accordance with accepted academic practice. No use, distribution or reproduction is permitted which does not comply with these terms.
*Correspondence: Mona Helmy, bW9uYS5oZWxteUBidWUuZWR1LmVn