- 1Department of Civil and Environmental Engineering, University of Connecticut, Storrs, CT, United States
- 2Department of Civil and Environmental Engineering, Rice University, Houston, TX, United States
This paper is motivated by the unique findings and observations from reconnaissance visits after the earthquake series in Puerto Rico in January 2020. It aims to discuss the potential interactions of Hurricane Maria and 2020 earthquake series and the considerations they underscore for future field reconnaissance missions. Traditionally, post-disaster damage assessment activities focus on one hazard and overlook the potentially cascading effects of multiple hazards on structures and infrastructure. This paper provides case studies showing the possible interaction of multiple hazards and their cascading effects observed in Puerto Rico. Infrastructure surveyed includes port facilities, buildings (particularly historical structures), and bridge structures. The data collected during the reconnaissance missions reveal how the impacts of Hurricane Maria, along with infrastructure aging and delayed repair and recovery activities, may have influenced the damage level and failure modes observed during the earthquake sequence a few years after. These case studies illustrate the nature of multihazard interactions and how these effects should be documented during post-disaster assessments. Beyond the insights gained from the case studies illustrated in this paper, the field survey instrument is provided as a basis for future reconnaissance studies, and the full set of reconnaissance data collected are published on the NSF funded NHERI DesignSafe cyberinfrastructure. As a result, this work not only provides data from Puerto Rico that can inform future damage and recovery modeling efforts, but also offers survey instruments and a field data collection process that is particularly tailored to cases where multihazard effects are at play.
1 Introduction
Regions across the United States and worldwide are generally at risk from more than one natural hazard which vary in spatial and temporal scale, occurrence potential, and intensity. These hazards may include earthquakes, hurricanes, floods, tsunamis, landslides, tornadoes, snowstorms, and fires, among others. Design engineers, infrastructure owners, risk managers, and other stakeholders are challenged to address the potential impacts of one or more natural hazards. Current design philosophies fail to consider the complex and intertwined effects of multiple hazards (Roy and Matsagar, 2021). The development and implementation of effective design and risk mitigation strategies requires an understanding of the performance of structures and infrastructure in a multihazard environment, considering cases of concurrent, non-concurrent, or cascading hazards. Unique engineering challenges are posed by such multihazard problems (Li et al., 2012). Ongoing work in the community ranges from characterizing the multihazard potential (Gill and Malamud, 2014; Hayes et al., 2014), simulating behavior under cascading hazards (Echevarria et al., 2015b; Echevarria et al., 2015; Imani et al., 2015; Keller and Pessiki, 2015), testing structures under concurrent hazard loading (Baheru et al., 2014), to modeling risk and resilience in multihazard settings (Barbato et al., 2013; Kameshwar and Padgett, 2014; Echevarria et al., 2015a; Jaimes et al., 2015; van de Lindt et al., 2015). Unfortunately, the lack of field data to support a variety of multihazard analyses to adequately characterize system failure modes or validate the existing models has slowed the progress in this domain (Bruneau et al., 2017).
In this regard, the cascading effects of two inherently different events that impacted Puerto Rico presented a unique opportunity for researchers to collect invaluable data and provide empirical evidence for potential multihazard interactions. A team of researchers with diverse backgrounds in structural engineering, risk assessment, and risk mitigation for complex and interconnected infrastructure systems conducted reconnaissance missions to document and analyze the damages during the January 2020 earthquake series (Miranda et al., 2020) with linkage to Hurricane Maria, the Category four storm that struck the island in September 2017 (FEMA, 2018). The nomenclature proposed by Zaghi et al. (2016) to discuss multihazard design will be used in the framing of this paper. The case of two subsequent punctuated events, i.e., earthquake series and hurricane, as occurred in Puerto Rico is a classic case of non-concurrent multihazard scenarios. In such a case, degradation of structural capacity or shifts in boundary conditions from the primary event, along with the incremental or incomplete restoration processes prior to subsequent events, can have a significant impact on performance. This premise is illustrated below in Figure 1. Here, it is shown how either a delayed repair or no repair negatively impacts system performance during a second event. For example, for a port structure, gradual decay makes the structure more vulnerable. After an event, the damaged structure could go through a faster decay than before as shown with the larger negative slope. However, this interaction between chronic stressors and multiple hazard events is not well understood, and data is scarce to fill this knowledge gap (Zaghi et al., 2016).
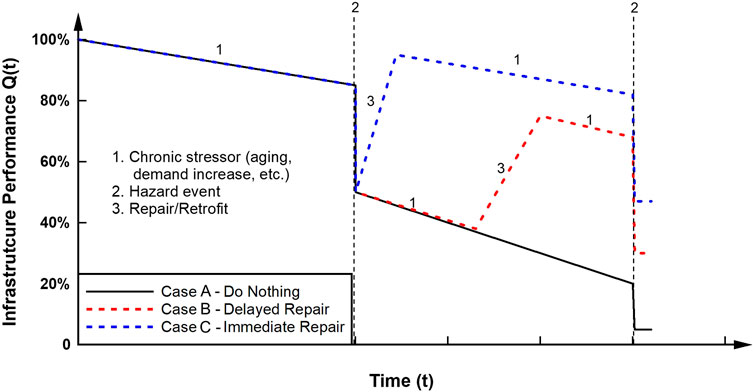
FIGURE 1. Schematic showing change in system performance in subsequent hazards based on repair timeline.
While it is well known that earthquakes can have a different impact on structures that suffer from corrosion damage or scour (Shiraki et al., 2007; Wang et al., 2014; Burke and Bruneau, 2016; Argyroudis et al., 2020), analyses are commonly performed on models of intact structures. This can be detrimental given that structures are rarely hit by an event in their intact form, i.e., new construction. Researchers have studied the combined effects of chronic and extreme stressors on the reliability and resilience of infrastructure systems throughout their lifetime (Ouyang et al., 2012; Yang and Frangopol, 2019; Liu et al., 2020), underscoring the significance of considering the interactions of multiple hazards at different stages in the system’s service life, rather than modeling them independently. For the specific case of bridges (Kumar and Gardoni, 2014; Padgett and Kameshwar, 2016; Vishnu and Padgett, 2020), several studies examined their vulnerability to impacts such as hurricanes and earthquakes, along with gradual stressors such as aging and sea-level rise, while emphasizing the pressing need for multihazard empirical data to support model development and validation. While several researchers have discussed procedures for multihazard risk assessment predicting cascading failures (Eisenberg et al., 2020; Wei et al., 2022), the community lacks empirical data showcasing the impacts of such hazards.
During the investigation in Puerto Rico, it was found that some of the damage scenarios may be explained in a multihazard context that accounts for an interaction of impacts from the recent earthquakes and Hurricane Maria. The earthquakes exposed several unexpected and undocumented vulnerabilities of the infrastructure. As there is limited knowledge on the possible interactions of hurricanes and seismic events, the team investigated conditions that could lead to multihazard damages including: 1) structures and sites that experienced soil erosion during Hurricane Maria, 2) landslide-driven hydrologic effects of Maria that may be worsened during the earthquakes, 3) structural repairs that changed boundary conditions in subsequent events, 4) structures that showed signs of damage after the occurrence of the aftershocks, and 5) structural design concepts, such as elevated construction to avoid flooding, that makes structures more vulnerable to earthquakes.
This paper intends to present a thought-provoking series of case studies which encourage future reconnaissance missions through the lens of multihazard impacts. The main contributions of the work are: 1) presenting actual field observations and insights on multi-hazard interactions, 2) providing access to the comprehensive set of data collected, curated, and published for reuse on DesignSafe CI, and 3) sharing a field survey instrument and systematic field survey approach for future use in reconnaissance cases with potential multihazard effects. It is important to note that this paper relies heavily on observations, limited publicly available data, and the firsthand accounts of infrastructure owners or operators collected during the field visits. For that reason, significant interpretation was needed by the authors. The authors acknowledge that some of the findings presented may be viewed differently by other experts, but the aim is to share the data and information gleaned from conversations during our multiple visits and post-mission reflections. An overview of the region and susceptibility to hazards is presented first, followed by the details of four case studies of multihazard interactions. This work not only provides data from Puerto Rico that can inform future damage and recovery modeling efforts, but also presents survey instruments and a field data collection process that is particularly tailored to cases where multihazard effects are at play.
2 Overview of location and events
2.1 Location of interest
Ponce, Puerto Rico’s second largest city, is located on the island’s southern coast. During the 1800s, Ponce was an important port for shipping sugar, rum, coffee, and tobacco (Tillman, 2009). As the sugar industry evaporated in the 20th century, the economy experienced a downturn that resulted in an increased reliance on tourism. In addition, the island has grappled with high unemployment and poverty rates, government disinvestment in public services, and crime. Natural hazards in this area expose vulnerabilities from colonial subjugation, economic hardship, environmental injustice, infrastructural neglect, and corruption from the island’s political class (Lloréns, 2018). In the following section, we will provide an overview of the threat from natural disasters and detail the two phenomena that are the focus of this paper: Hurricane Maria and the 2020 earthquake series.
2.2 Overview of hazards
2.2.1 Hurricanes
Puerto Rico is subject to frequent and severe impacts from hurricanes, particularly between June and November (Havidan, 1997; Emery et al., 2004; López-Marrero et al., 2019). Puerto Rico experiences some of the highest hurricane frequency in the North Atlantic basin. Storms typically approach from the east and south-east, and the most intense storms often form on the western coast of Africa and reach Puerto Rico near maximum intensity (Emery et al., 2004). Based on data from 1900 to 2017, a total of 33 hurricanes passed over Puerto Rico or within 86 miles from the coast. Furthermore, 14 of the 33 reached wind velocities of Category three or higher (López-Marrero et al., 2019).
The 2017 Atlantic hurricane season was one of the costliest and most destructive on record. For the first time, three Category four hurricanes made landfall in the US (Blake, 2018). One such event was Hurricane Maria, which devastated Puerto Rico and many islands in the Caribbean. On 20 September 2017, Hurricane Maria’s center crossed the southeast coast of Puerto Rico near Yabucoa around 10:15 UTC. At that time, the maximum wind speed was 155 mph, which is just below Category five intensity. The hurricane’s center crossed the island from southeast to northwest and emerged into the Atlantic around 18:00 UTC (Pasch et al., 2017). The estimated storm surge levels at the coast are shown in Figure 2. The scale of the devastation, costliness of the damage, and delay in releasing federal aid left critical infrastructure unrepaired prior to the earthquake series.
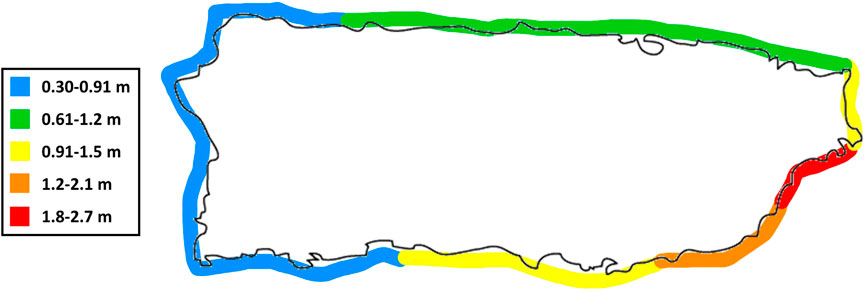
FIGURE 2. Estimated storm surge inundation along the coasts of Puerto Rico adapted from Pasch et al. (2017).
2.2.2 Earthquakes
The island of Puerto Rico is located between the North American and the Caribbean tectonic plates. The boundary is characterized primarily by left-lateral motion along east-west striking faults (Jansma et al., 2000). The Puerto Rico-Virgin Islands microplate is one of three microplates located in this complex plate boundary zone (Raussen and Lykke-Andersen, 2009), and is part of the Caribbean plate. On the northern side of the island, the North American plate is subducting obliquely beneath the Caribbean plate (Vičič et al., 2022). This is bounded by the east-west striking Puerto Rico trench (Jansma et al., 2000). On the southern side of the island, the Muertos trough, an east-west striking fault defines the southern limit of the Puerto Rico-Virgin Islands microplate (Jansma et al., 2000). Seismicity in and around Puerto Rico averages hundreds of earthquakes per year (Jansma et al., 2000).
A series of significant seismic events occurred in the southwestern region of Puerto Rico in early January 2020. The first major event (Mw 5.8) occurred on January 6th at 10:32 a.m. UTC. The second significant event (Mw 6.4) occurred on January 7th at 8:24 a.m. UTC. The third significant event (Mw 5.6) occurred on January 7th at 11:18 a.m. UTC. These earthquakes caused significant damage to many structures near Guayanilla and Ponce, Puerto Rico. The start of the seismic events occurred on 28 December 2019, and the region continued to experience aftershocks as of March 2020 (Miranda et al., 2020). A summary of all magnitude five and larger earthquakes between 29 December 2019 and 17 January 2020 are listed in Table 1 (Van Der Elst et al., 2020). The PGA contours from the Mw 6.4 event are shown in Figure 3.
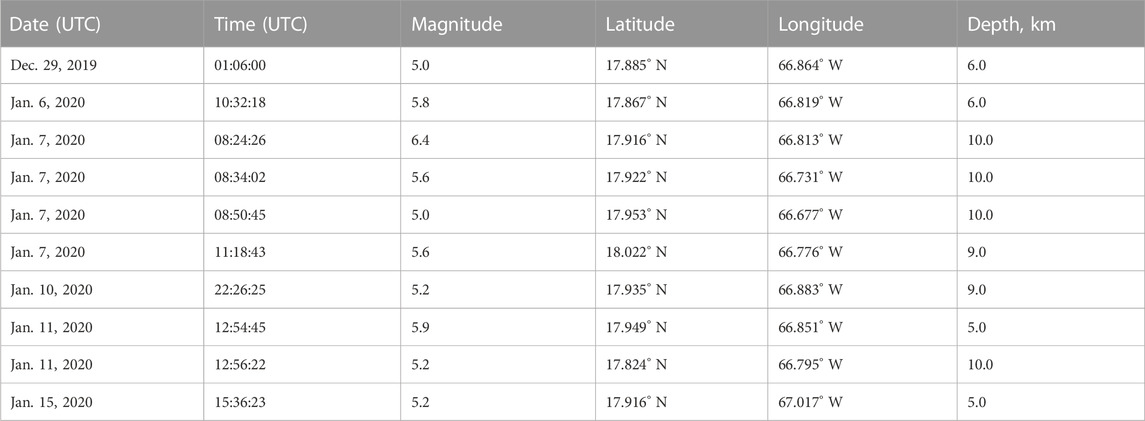
TABLE 1. Summary of magnitude five and larger earthquakes between December 29th, 2019–17 January 2020 (Van Der Elst et al., 2020).
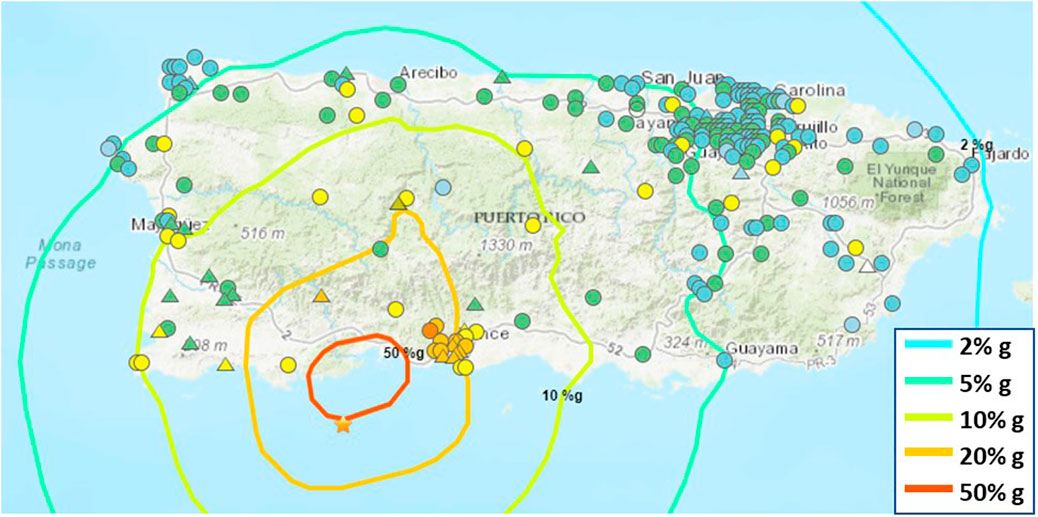
FIGURE 3. PGA contours estimated from ShakeMap for 7 January 2020, Mw 6.4 earthquake (USGS, 2020).
3 Reconnaissance methods
The reconnaissance missions were funded through an NSF RAPID Project. The first visits were conducted January 23rd-24th and February 24th-26th, 2020. The final visit was set to occur in March 2020 but was delayed due to COVID-19 travel restrictions. When travel bans were lifted, the final visit was conducted on April 21st-23rd, 2021. The inspections focused on structures in Southern Puerto Rico that sustained damages during the earthquake series. The damages were compared to previous seismic events to determine if the level of damage was consistent with the magnitude of the earthquake or if additional factors likely contributed to the damage. Traditional post-disaster damage assessment activities focus on one hazard and overlook the potentially destructive cascading effects of multiple hazards on structures and infrastructure. Therefore, the focus of this study was to identify the compounding impacts of Hurricane Maria and the 2020 earthquake series. Through this inspection, a comprehensive data set was collected to understand if the impacts of Hurricane Maria resulted in an increased level of damage or contributed to unexpected modes of failure during the recent earthquake series.
Multiple methods were used to ensure a comprehensive set of data was collected. The data collection methods included a damage assessment survey, multiple image types, images and videos taken with an unmanned aerial system (UAS) operated by a team member, and Lidar scan data. All data from the missions can be found on DesignSafe-CI (Zaghi et al., 2021; Hain et al., 2022). The team made use of the RAPID Facility Field Data Collection App (Rapp) survey application to ensure a systematic approach toward data collection (Berman et al., 2020). The survey included guiding questions to be answered at each site location at the start of data collection. The survey questions are included below in Table 2. The survey is meant to be used in the numerical order shown. The damage assessment survey was completed by 2-3 of the team members to ensure it the findings were consistent.
4 Results from multihazard case studies
4.1 Case 1: Historic buildings in downtown Ponce, PR shock-aftershock
One of the most prevalent examples of multihazard events is the successive nature of the initial earthquake and corresponding aftershocks. While most earthquakes are accompanied by aftershocks, typical designs only consider the maximum magnitude event. As the research team visited Puerto Rico on three occasions after the initial earthquake series, they were able to evaluate the progression of structural damages in the aftershock events.
As the economy of the Ponce region is largely focused on tourism, the team focused on historical structures. These buildings were vulnerable to seismic loads for several reasons, including their age and the brittle nature of the construction materials and structural systems. A sample of the damage progression over time is shown in Figure 4. During the initial events, this building sustained severe damage including shear cracking at the awning and at multiple locations along the roof as shown in Figures 4A, B. At this point, the building was roped off and entry was halted for safety concerns. As the aftershocks continued, the damaged worsened, as shown in Figure 4C. One of the shear cracks along the side of the building expanded and the brick wall collapsed which exposed the structural support system of the roof. It is also important to note that the front of the structure was supported by temporary bracing that was installed after the main shock, which prevented the collapse of this portion. This shows that minimal external bracing/shoring or strengthening is an effective strategy in preventing unrecoverable damage or total collapse during aftershocks. This is particularly important for brittle structures that lack an engineered system to provide ductility and energy dissipation, and those with historic significance. To minimize damage in subsequent events it is critical to first identify the repair priorities and then allocate the resources needed to execute the repairs. When identifying repair priorities, safety, economic impact, and historical significance should be considered.
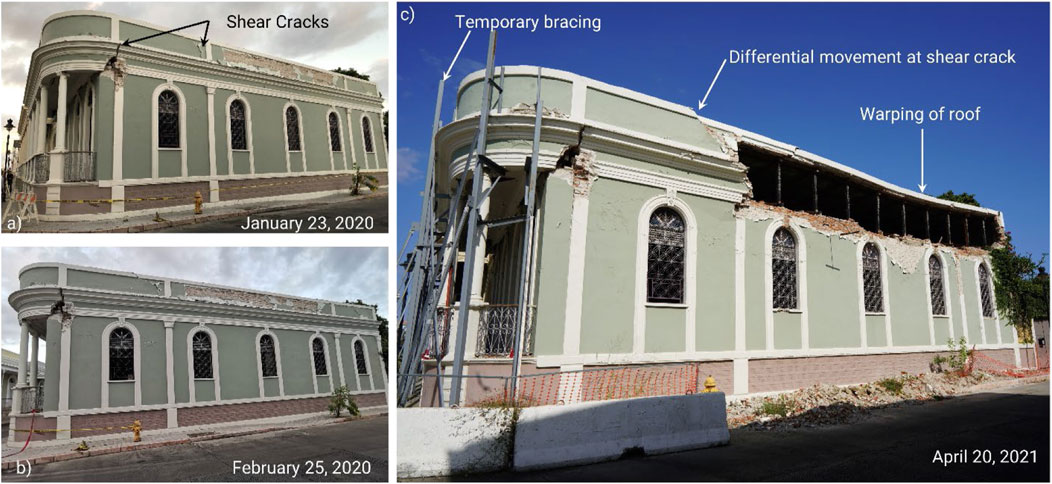
FIGURE 4. Damage progression of sample historical building in downtown Ponce under aftershock events. Images were taken during consecutive visits on (A) 23 January 2020, (B) 25 February 2020, and (C) 20 April 2021.
A second example of a historical building that suffered damage during the initial earthquake series is shown below in Figure 5. In Figure 5A, shear cracks on the unreinforced masonry wall around the window opening are visible. This hotel is located in downtown Ponce. It experienced temporary closures after the damage was discovered from the mainshock. The damage was repaired quickly, and the window was removed to form a more robust lateral load resisting system as shown in Figure 5B. This illustrates how the timely implementation of repairs can protect vulnerable, but significant, structures from increased damage.
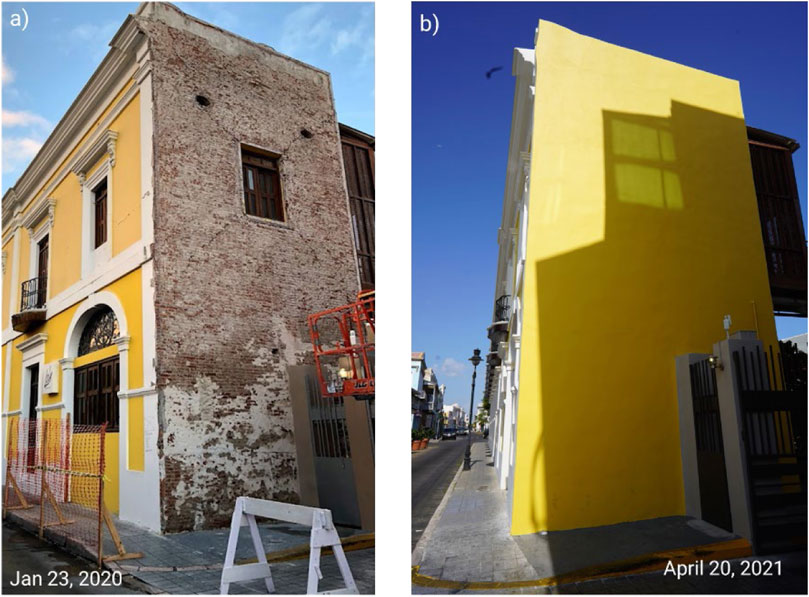
FIGURE 5. Historical hotel in Downtown Ponce showing (A) damage after initial earthquake series and (B) view of repaired wall segment.
In the context of societal and economic impacts, damages after the hurricane and the slow recovery led to mass migration from the affected areas. In the aftermath of the earthquake series, the number of individuals leaving Puerto Rico increased (Sanchez, 2020; Aranda et al., 2022). This included a large number of small business owners supporting the tourism industry. The research team identified that a lack of, or delayed, access to federal funding through FEMA significantly slowed the economic recovery of the region, which adds to the vulnerability. For example, the team noticed that several historic buildings in Ponce that needed restoration after the earthquakes were abandoned by the owners. At the time of the visits, the city officials were in conversation with the historic preservation community to evaluate if some of these buildings have to be demolished because of public safety concerns.
4.2 Case 2: Rafael Cordero Santiago Port of the Americas, Hurricane Maria–earthquake series
This case study details the multihazard impacts from punctuated stressors, i.e., Hurricane Maria and the earthquake series. The extent of damage on ports facilities due to hurricanes is often directly correlated to the proximity of the port to the hurricane landfall. Damages can be attributed to several characteristics of the hurricane, including storm surge, wave heights, wind speed, debris impact, and the duration of exposure. Hurricane damages can include disruptions to key features, such as the navigation channel, or structures, such as piers and wharfs, as well as destruction of ancillary structures, equipment, and cargo throughout the port (Wakeman, 2013). While the port experienced notable damage from Hurricane Maria alone, this section will focus on the physical impacts from the storm that influenced its performance during the earthquake series.
This case highlights one pier that sustained severe damage during the earthquakes, shown below in Figure 6. The level of damage sustained was greater than what is expected from earthquakes of this magnitude, suggesting additional factors contributed to the damage. Major damage types included seaward displacement of panels (Figures 6A, C), bowing of the berth (Figure 7), and collapse of panels (Figures 6A, B). Upon inspection, the team determined the slab failure of the pier that occurred during the earthquake series was caused by compromised support conditions. Key personnel from the site noted washout from the pier was evident after Hurricane Maria. In addition, evidence of coastal erosion in the region was noted in Geotechnical Extreme Events Reconnaissance report following the hurricane (Silva-Tulla et al., 2018). The erosion of the supporting soil fill, as shown in Figure 6B, made the unreinforced slab-on-grade vulnerable to the seismic shaking.
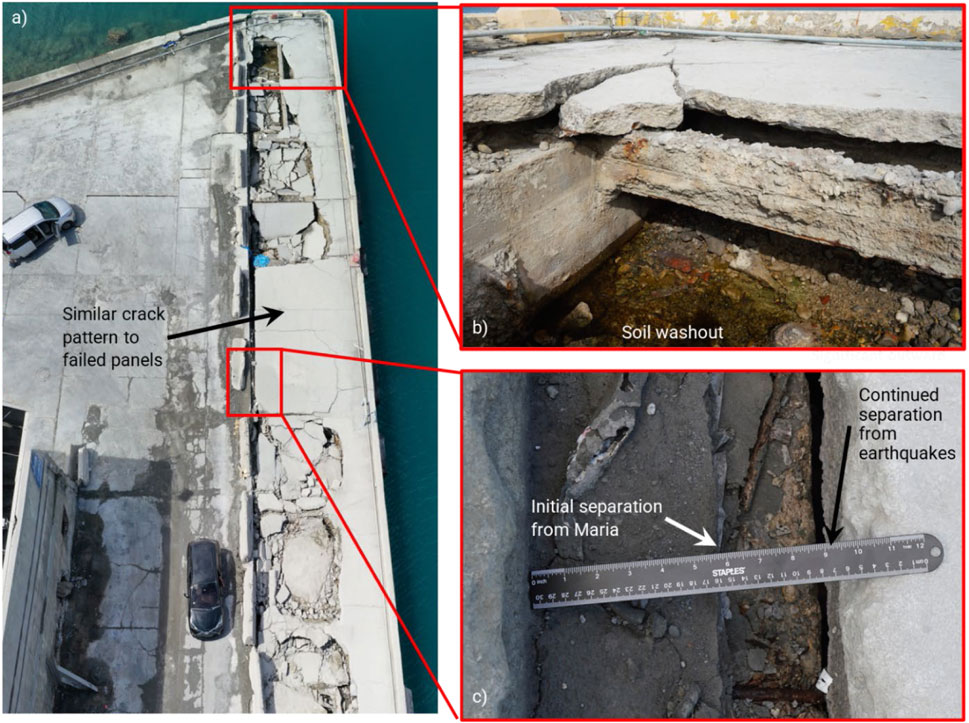
FIGURE 6. Images of damages to Pier following January 2020 earthquake series including (A) an aerial view of the overall damage, (B) washout of soil underneath collapsed panel, and (C) representative image showing separation of main slab and pier.
As shown in Figure 7, there was severe bowing of the berth at the upper region of the pier. This bowing shows an area on the edge of the pier that is experiencing larger outward displacements compared to other areas along the length, which suggests more significant pile damage at this location. It is unlikely the bowing is caused by large soil or water pressure at this location, as washout of the soil fill within the damaged regions was observed.
The port was closed for a short period of time due to damage incurred from the hurricane, yet the port was partially restored due to its critical role. While the port was allocated funds to address damages from Hurricane Maria, at the time of inspection the federal funds still had not been released. Due to this delay, the condition of many structures continued to degrade, worsening the performance in the earthquake series. It is not in the scope of this paper to discuss the reasons for delaying funds, rather we aim to highlight the ramification of such actions in terms of safety and higher overall repair costs following subsequent events. This should be a key consideration for policymakers when responding to and planning for future natural disasters.
The damages caused significant delays and postponement of the expansion plans, which were to transform the port into an integrated transport center and logistics platform for international trade in the Caribbean region. The goal of this development strategy was to convert the facility into a third-generation, multi-cargo port, in order to meet the growing needs of the container ship sector and the demands of globalization. This delay has exacerbated the economic impact of the disaster to the affected region, as the lack of capacity to manage the increased cargo volume has led to further economic losses.
4.3 Case 3: Rafael Cordero Santiago Port of the Americas, chronic stressors–earthquake series
Ports are located in highly-corrosive coastal environments. The two most commonly used structural materials, steel and reinforced concrete, are both vulnerable to corrosion deterioration (Maniglio et al., 2021). As such, ports are at a higher risk of accelerated damage due to corrosion compared to other structures. Design considerations, such as increasing the thickness of the cover concrete, using corrosion resistant reinforcements such as epoxy coated or stainless-steel rebar, or including sacrificial thickness or a concrete coating for steel piles, can mitigate the adverse impacts of corrosion damage. However, implementing such strategies often results in increased costs and not all options were available during the construction of older port structures. For reinforced concrete elements, such as beams, slabs, and piles, corrosion of reinforcement is likely to occur if widespread cracking or insufficient cover is present. Corrosion of the reinforcement leads to the spalling of cover concrete, which then further exposes reinforcement. This corrosion weakens the structural elements and may lead to widespread damage under extreme loadings, such as hurricanes and earthquakes.
The majority of the earthquake damage at the ports inspected was exacerbated by underlying corrosion of the structure. This was likely worsened or initiated in Hurricane Maria due to acceleration of corrosion damage in structural components due to increased exposure to sea water. The high level of corrosion present contributed to the spalling and failure of elements in the seismic events. Piers had substantial corrosion in the superstructure. This included the beams (Figure 8A), support structures for slabs (Figure 8B) and the concrete barrier surrounding the edge of the piers (Figure 8C). In many cases, the corrosion damage was not observable prior to the earthquakes when the concrete spalled, but the level of corrosion in the exposed elements indicates that corrosion has been occurring for some time. This makes it challenging to identify corrosion in inspections and, therefore, unlikely to be repaired until it reaches a critical level. Understanding the progression of corrosion and how this deterioration impacts structural behavior under extreme events is critical for predicting performance of aging structures and should be considered in design.
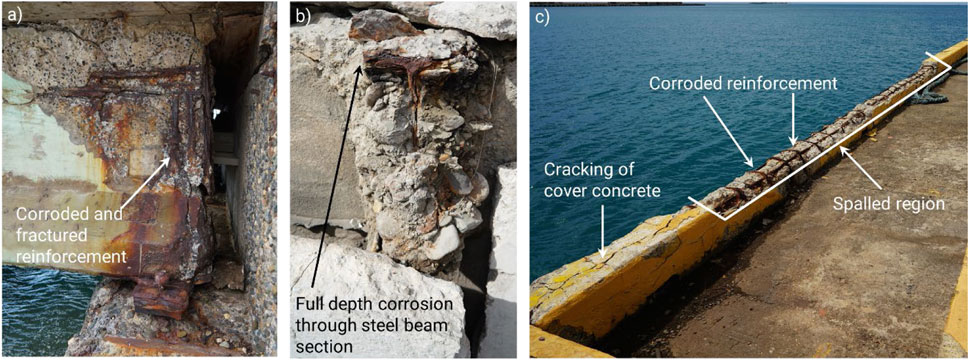
FIGURE 8. Elements at the ports inspected had severe corrosion damage that was observable after the earthquake series. Sample views of different elements with visible deterioration from corrosion include (A) beams, (B) support structures for slabs, and (C) concrete barrier surrounding the edge of the piers.
While the economic impact of this multihazard scenario is not immediately evident, it can be viewed from the perspective of the expansion plans discussed earlier. Any expansion requires repair and replacement of the existing corrosion damaged elements. This could potentially lead to delays and increased costs due to the need to repair or remove the existing structures.
4.4 Case 4: Bridge in Guayanilla, Hurricane Maria—earthquake series
During Hurricane Maria, the Guayanilla River flooded, which resulted in washout and debris impacts. This was observed on the bridge carrying PR-127, which spans the river. The washout was most severe at the abutment, where exposed piles can be seen on the left of Figure 9A. The bridge was closed and required repair, including backfilling behind the abutment and fill work in front of the abutment (Maria, 2017). This bridge was not visited in the missions in 2020, but after seeing the drone footage from after Hurricane Maria, was added to the inspection list for the 2021 mission. Following the earthquake series and as of April 2021, the bridge remained open to traffic. A panoramic view of the bridge taken with a drone is shown in Figure 9B. Upon further inspection, the research team found several examples of seismic damage, including cracking at the abutments from pounding, bent anchor bolts at the bearings, and spalling at the top of the columns, as shown in Figures 9D, E. There was also evidence of geotechnical damage, as shown in Figure 9C, but it was not clear if this was left over from hurricane Maria, or a result of the earthquakes.
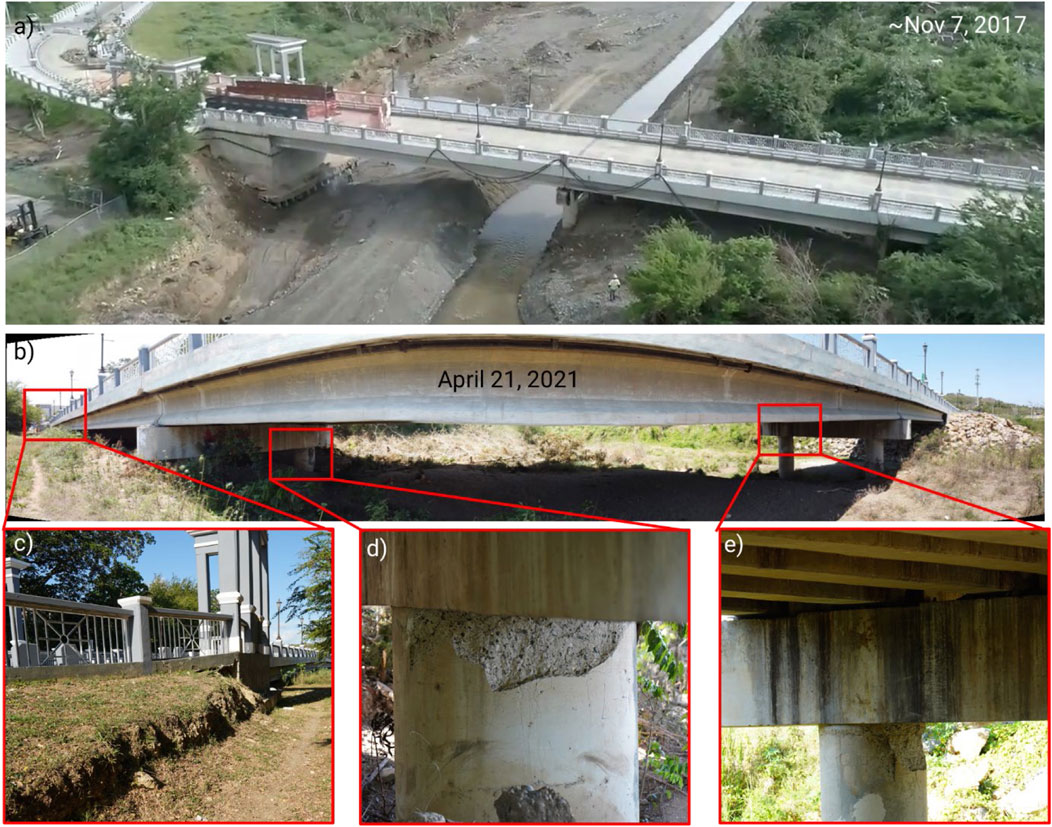
FIGURE 9. Bridge in Guayanilla, PR over the Guayanilla River. These photos include (A) a screenshot from a drone flyover taken 50 days after hurricane Maria (Maria, 2017), (B) an overall view of the bridge taken on the final reconnaissance mission, (C) the geotechnical impacts observed at the abutment, (D) cracking on support column on Pier 1, and (E) cracking of support column on Pier 2.
This case study was included to show an example of where the multihazard effect was not clear. While there was no direct evidence, the soil washout that occurred at the abutment and piers may have contributed to more extensive damage to the bridge columns than was observed at other bridges in the area. In addition, if this spalling is not repaired in a timely fashion, the column reinforcement at the critical plastic hinge zone will be subjected to premature corrosion damage, which will further weaken the structure.
The restoration of transportation infrastructure was prioritized in the aftermath of the hurricane, and the bridge structures visited were found to be in a relatively good condition. This was an important factor in avoiding any disruptive failure of the bridges after the earthquakes, thus ensuring continued access to the impacted areas.
5 Discussion
The cases presented illustrate multihazard impacts observed on different structure types and under different hazards. Multihazard reconnaissance is significantly more challenging because the state of the structure is not always documented after the first event, or through time. In addition, a consistent data collection approach is needed to ensure that the data collected at different times are comparable in terms of location and focus. The founding of the Structural Extreme Events Reconnaissance (StEER) Network in 2018 was a notable first step in this direction. However, we need to promote the use of a multihazard approach in reconnaissance. The traditional approach has been focused on documenting damage from a single event and ensuring meaningful information can be extracted from the observation data. It is important to evaluate the damages in the context of the condition of the structure and the potential interaction of multiple hazards, otherwise the findings may be misleading. Findings from reconnaissance are used to inform changes to design codes, thus it is critical to make sure the correct conclusions are drawn. For example, if we see failure of concrete elements without paying attention to level of corrosion or a change in the support conditions, we may misattribute the cause. While the data in this paper was not directly used to assess safety levels of the structures noted, it is our hope that the data presented will encourage the multihazard perspective in future reconnaissance missions. The safety assessment process requires well-calibrated and generalizable analytical models of structural types and systems, which is a major undertaking that was outside of the scope of this effort. However, as an intermediate approach, additional questions can be added to surveys in current field safety evaluation protocols and manuals to note potential evidence of prior damages due to other hazards or aging as well as the interaction of effects from multiple hazards.
Another consideration for the future of multihazard design in regions such as Puerto Rico is addressing conflicting design objectives. The design of buildings in Puerto Rico is largely dependent on the location of the structure. Most buildings in coastal areas of the island are concrete structures. These buildings resist high winds, but their large mass makes them vulnerable to the earthquakes. In addition, elevated construction is used for the buildings to prevent water damage due to storm surge. However, this system is vulnerable to earthquakes because of the soft story behavior as shown in Figure 10A. As additional regions become exposed to new and more extreme natural hazards due to climate change, the research on multihazard damages and design will need to be implemented in design codes.
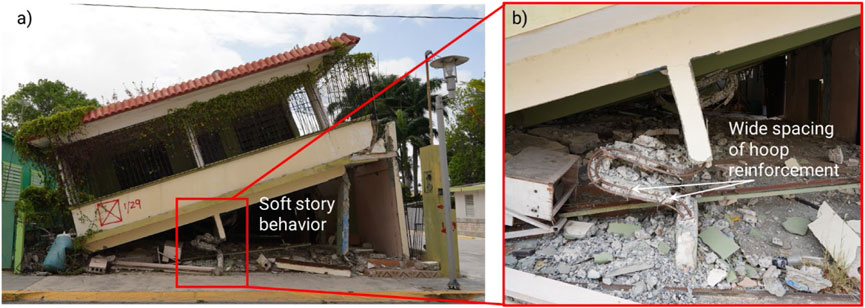
FIGURE 10. Damage of building in Guánica, PR following earthquake series showing (A) overall damage and soft-story failure mode and (B) close up of support column collapse.
We would be remiss not to note the importance of contractors and inspectors adhering to designs. During the reconnaissance mission, the team overserved many buildings that appeared to have construction flaws such as poor-quality concrete or insufficient steel reinforcement (Figure 10B). Due to the limited information available to the team, it is unclear if the issues were caused in the design or construction phase. While these findings do not fall under the purview of multihazard design or inspection per se, they are critical components of forensic engineering to ascertain failure patterns.
6 Conclusion
This paper presents a series of case studies from multiple reconnaissance missions in the region surrounding Ponce, PR, analyzing the damages from the January 2020 earthquake series with linkage to Hurricane Maria, the Category four storm that struck the island in September 2017. In cases of subsequent hazards, degradation of structural capacity or shifts in boundary conditions from the primary event, along with the incremental or incomplete restoration processes prior to subsequent events, can have a significant impact on performance. The cascading effects of the two inherently different events that impacted Puerto Rico presented a unique opportunity for researchers to collect invaluable data and provide empirical evidence to characterize multihazard interactions.
Beyond the key insights gained from investigation of the case studies described in this paper, this study introduced a new field survey instrument. This instrument emphasized unique considerations for future field reconnaissance missions to systematically consider the potential for multiple event effects over short and long time periods. Moreover, the full dataset from multiple waves of reconnaissance was published on NHERI’s DesignSafe cyberinfrastructure platform to allow sharing and data reuse. Such data could provide a future opportunity to verify and validate structural system level damage and restoration models, particularly for the understudied area of life-cycle resilience in which incomplete recovery or damage accumulation affects subsequent event performance.
Data availability statement
The datasets presented in this study can be found in online repositories. The names of the repository/repositories and accession number(s) can be found in the article/supplementary material.
Author contributions
AH, AZ, and JP contributed to conception and design of the study, AT organized the database, AH, AZ, AT, and JP interpreted the results. AH wrote the first draft of the manuscript. AZ, AT, and JP wrote sections of the manuscript. All authors contributed to manuscript revision, read, and approved the submitted version.
Funding
This paper presents an overview of the reconnaissance missions in Ponce, PR and the surrounding region to document multihazard impacts from Hurricane Maria and the January 2020 earthquake series. The missions, data processing and curation were conducted under NSF Awards #2022390 and #2022427.
Acknowledgments
The authors would like to acknowledge the support and guidance of the RAPID Facility at the University of Washington, particularity Dr. Michael Grilliot who assisted with data collection and processing from the first field visit. In addition, the project would not have been possible without Angela Lanning, who supported the field missions and lead the archiving of the data.
Conflict of interest
The authors declare that the research was conducted in the absence of any commercial or financial relationships that could be construed as a potential conflict of interest.
Publisher’s note
All claims expressed in this article are solely those of the authors and do not necessarily represent those of their affiliated organizations, or those of the publisher, the editors and the reviewers. Any product that may be evaluated in this article, or claim that may be made by its manufacturer, is not guaranteed or endorsed by the publisher.
References
Aranda, E., Blackwell, R., Escue, M., and Rosa, A. (2022). Cascading disasters: The impact of hurricane Maria and Covid-19 on post-disaster Puerto Rican migrants’ adaptation and integration in Florida. Lat. Stud. 2022, 1–24. doi:10.1057/s41276-022-00390-3
Argyroudis, S. A., Mitoulis, S. A., Hofer, L., Zanini, M. A., Tubaldi, E., and Frangopol, D. M. (2020). Resilience assessment framework for critical infrastructure in a multi-hazard environment: Case study on transport assets. Sci. Total Environ. 714, 136854. doi:10.1016/j.scitotenv.2020.136854
Baheru, T., Chowdhury, A. G., Bitsuamlak, G., Masters, F. J., and Tokay, A. (2014). Simulation of wind-driven rain associated with tropical storms and hurricanes using the 12-fan Wall of Wind. Build. Environ. 76, 18–29. doi:10.1016/j.buildenv.2014.03.002
Barbato, M., Petrini, F., Unnikrishnan, V. U., and Ciampoli, M. (2013). Performance-based hurricane engineering (PBHE) framework. Struct. Saf. 45, 24–35. doi:10.1016/j.strusafe.2013.07.002
Berman, J. W., Wartman, J., Olsen, M., Irish, J. L., Miles, S. B., Tanner, T., et al. (2020). Natural hazards reconnaissance with the NHERI RAPID facility. Front. Built Environ. 6, 573067. doi:10.3389/fbuil.2020.573067
Blake, E. S. (2018). The 2017 atlantic hurricane season: Catastrophic losses and costs. Weatherwise 71 (3), 28–37. doi:10.1080/00431672.2018.1448147
Bruneau, M., Barbato, M., Padgett, J. E., Zaghi, A. E., Mitrani-Reiser, J., and Li, Y. (2017). State of the art of multihazard design. Virginia, United States: American Society of Civil Engineers.
Burke, S., and Bruneau, M. (2016). Effect of surface roughness on cyclic ductility of corroded steel. J. Struct. Eng. 142 (6), 04016014. doi:10.1061/(asce)st.1943-541x.0001425
Echevarria, A., Zaghi, A. E., Chiarito, V., Christenson, R., and Woodson, S. (2015). Experimental comparison of the performance and residual capacity of CFFT and RC bridge columns subjected to blasts. J. Bridge Eng. 21 (1), 04015026. doi:10.1061/(asce)be.1943-5592.0000762
Echevarria, A., Zaghi, A. E., Christenson, R., and Accorsi, M. (2015a). CFFT bridge columns for multihazard resilience. J. Struct. Eng. 2015, C4015002.
Echevarria, A., Zaghi, A. E., Christenson, R., and Plank, R. (2015b). Residual axial capacity comparison of CFFT and RC bridge columns after fire. Polymers 7 (5), 876–895. doi:10.3390/polym7050876
Eisenberg, D. A., Park, J., and Seager, T. P. (2020). Linking cascading failure models and organizational networks to manage large-scale blackouts in South Korea. J. Manag. Eng. 36 (5), 04020067. doi:10.1061/(asce)me.1943-5479.0000820
Emery, R., Serrano, M. I., and Foster, D. R. (2004). Landscape and regional impacts of hurricanes in Puerto Rico. Ecol. Monogr. 74 (2), 335–352. doi:10.1890/02-4057
FEMA, (2018). 2017 hurricane season FEMA after-action report. Washington, DC: Federal Emergency Management Agency.
Gill, J. C., and Malamud, B. D. (2014). Reviewing and visualizing the interactions of natural hazards. Rev. Geophys. 52 (4), 680–722. doi:10.1002/2013rg000445
Hain, A., Zaghi, A., and Padgett, J. (2022). “Data collection of damage to Puerto Rico's infrastructure due to Hurricane Maria and the 2019-2020 Puerto Rico earthquakes - mission 2,” in RAPID: Multi-hazard damage to Puerto Rico’s infrastructure - investigation of the interaction of hurricane and earthquakes. version 1 (Austin, TX: Natural Hazards Engineering Research Infrastructure: Cyberinfrastructure (DesignSafe) 2020–2025). Available at: https://www.nsf.gov/awardsearch/showAward?AWD_ID=2022469. doi:10.17603/ds2-1mny-a490
Havidan, R. (1997). A socioeconomic analysis of hurricanes in Puerto Rico: An overview of disaster mitigation and preparedness. Hurricanes 1997, 121–143. doi:10.1007/978-3-642-60672-4_7
Hayes, G. P., McNamara, D. E., Seidman, L., and Roger, J. (2014). Quantifying potential earthquake and tsunami hazard in the Lesser Antilles subduction zone of the Caribbean region. Geophys. J. Int. 196 (1), 510–521. doi:10.1093/gji/ggt385
Imani, R., Mosqueda, G., and Bruneau, M. (2015). Finite element simulation of concrete-filled double-skin tube columns subjected to postearthquake fires. J. Struct. Eng. 141 (12), 04015055. doi:10.1061/(asce)st.1943-541x.0001301
Jaimes, M. A., Reinoso, E., and Esteva, L. (2015). Risk analysis for structures exposed to several multi-hazard sources. J. Earthq. Eng. 19 (2), 297–312. doi:10.1080/13632469.2014.962673
Jansma, P. E., Mattioli, G. S., Lopez, A., DeMets, C., Dixon, T. H., Mann, P., et al. (2000). Neotectonics of Puerto Rico and the Virgin Islands, northeastern Caribbean, from GPS geodesy. Tectonics 19 (6), 1021–1037. doi:10.1029/1999tc001170
Kameshwar, S., and Padgett, J. E. (2014). Multi-hazard risk assessment of highway bridges subjected to earthquake and hurricane hazards. Eng. Struct. 78, 154–166. doi:10.1016/j.engstruct.2014.05.016
Keller, W. J., and Pessiki, S. (2015). Effect of earthquake-induced damage on the sidesway response of steel moment-frame buildings during fire exposure. Earthq. Spectra 31 (1), 273–292. doi:10.1193/123112eqs362m
Kumar, R., and Gardoni, P. (2014). Effect of seismic degradation on the fragility of reinforced concrete bridges. Eng. Struct. 79, 267–275. doi:10.1016/j.engstruct.2014.08.019
Li, Y., Ahuja, A., and Padgett, J. E. (2012). Review of methods to assess, design for, and mitigate multiple hazards. J. Perform. Constr. Facil. 26 (1), 104–117. doi:10.1061/(asce)cf.1943-5509.0000279
Liu, W., Song, Z., and Ouyang, M. (2020). Lifecycle operational resilience assessment of urban water distribution networks. Reliab. Eng. Syst. Saf. 198, 106859. doi:10.1016/j.ress.2020.106859Get
Lloréns, H. (2018). Ruin Nation: In Puerto Rico, Hurricane Maria laid bare the results of a long-term crisis created by dispossession, migration, and economic predation. NACLA Rep. Am. 50 (2), 154–159. doi:10.1080/10714839.2018.1479468
López-Marrero, T., Heartsill-Scalley, T., Carlos, F-L-G-R., Escalera-Garcia, I. A., and Echevarria-Ramos, M. (2019). Broadening our understanding of hurricanes and forests on the caribbean island of Puerto Rico: Where and what should we study now? Forests 10 (9), 710. doi:10.3390/f10090710
Maniglio, M., Balomenos, G. P., Padgett, J. E., and Cimellaro, G. P. (2021). Parameterized coastal fragilities and their application to aging port structures subjected to surge and wave. Eng. Struct. 237, 112235. doi:10.1016/j.engstruct.2021.112235
Maria, H. (2017). PhotoSkyView. Hurricane Maria - the main bridge of Guayanilla after 50 Days (huracán Maria) - phantom 4Pro. https://www.youtube.com/watch?v=XHTrmqMBkDk&list=PLLvcsDpvAIDjPaWbKuWRKRFtYGtPqlILy&index=7.
Miranda, E., Acosta Vera, A., Aponte, L., Archbold, J., Cortes, M., Du, A., et al. StEER - 7 jan. 2020 Puerto Rico Mw 6.4 earthquake: Preliminary virtual reconnaissance report (PVRR). 2020.
Ouyang, M., Dueñas-Osorio, L., and Min, X. (2012). A three-stage resilience analysis framework for urban infrastructure systems. Struct. Saf. 36, 23–31. doi:10.1016/j.strusafe.2011.12.004
Padgett, J. E., and Kameshwar, S. (2016). “Supporting life cycle management of bridges through multi-hazard reliability and risk assessment,” in Multi-hazard approaches to civil infrastructure engineering (Berlin, Germany: Springer), 41–58.
Pasch, R. J., Penny, A. B., and Berg, R. (2017). Hurricane Maria (AL152017). United States: National Hurricane Center.
Rathje, E., Dawson, C., Padgett, J. E., Pinelli, J-P., Stanzione, D., Adair, A., et al. (2017). DesignSafe: A new cyberinfrastructure for natural hazards engineering. Reston, VA: American Society of Civil Engineers.
Raussen, S., and Lykke-Andersen, H. (2009). “Micro plate tectonics in the Virgin Islands Basin, north eastern Caribbean,” in AGU Fall Meeting Abstracts, Chicago, IL, 12-16, December, 2022.
Roy, T., and Matsagar, V. (2021). Multi-hazard analysis and design of structures: Status and research trends. Struct. Infrastructure Eng. 2021, 1–30. doi:10.1080/15732479.2021.1987481
Sanchez, R. (2020). For Puerto Rico’s earthquake families, trauma continues thousands of miles from home. Georgia, United States: CNN.
Shiraki, N., Shinozuka, M., Moore, J. E., Chang, S. E., Kameda, H., and Tanaka, S. (2007). System risk curves: Probabilistic performance scenarios for highway networks subject to earthquake damage. J. Infrastructure Syst. 13 (1), 43–54. doi:10.1061/(asce)1076-0342(2007)13:1(43)
Silva-Tulla, F., Pando, M., Soto, A., Morales, A., Pradel, D., Inci, G., et al. (2018). Geotechnical impacts of hurricane Maria in Puerto Rico. Virginia, United States: ASCE. Report No GEER-057.
Tillman, B. F. (2009). Measuring globalization's influence on the cultural landscape: Spatial succession in the plaza of Ponce, Puerto Rico. Southeast. Geogr. 49 (4), 340–353. doi:10.1353/sgo.0.0056
USGS, M. (2020). 6.4 - 8km S of indios, Puerto Rico. Available at: https://earthquake.usgs.gov/earthquakes/eventpage/us70006vll/map?shakemap-code=us70006vll&shakemap-source=us&shakemap-pga=true&shakemap-stations=true&shakemap-mmi-contours=false.
van de Lindt, J. W., Ellingwood, B. R., McAllister, T., Gardoni, P., Cox, D. T., Cutler, H., et al. (2015). “Computational environment for modeling and enhancing community resilience: Introducing the center for risk-based community resilience planning,” in Second International Conference on Performance-based and Lifecycle Structural Engineering (PLSE 2015), Brisbane, AU, December 9-11, 2015.
Van Der Elst, N., Hardebeck, J. L., and Michael, A. J. (2020). Potential duration of aftershocks of the 2020 southwestern Puerto Rico earthquake. US Geological Survey: US Geological Survey, 2331–1258.
Vičič, B., Momeni, S., Borghi, A., Lomax, A., and Aoudia, A. (2022). The 2019–2020 Southwest Puerto Rico earthquake sequence: Seismicity and faulting. Seismol. Soc. Am. 93 (2A), 533–543. doi:10.1785/0220210113
Vishnu, N., and Padgett, J. E. (2020). Interaction of life-cycle phases in a probabilistic life-cycle framework for civil infrastructure system sustainability. Sustain. Resilient Infrastructure 5 (5), 289–310. doi:10.1080/23789689.2019.1574514
Wakeman, T. H. (2013). University Transportation Research Center—Region 2. Washington, DC: University Transportation Research Center, US Department of Transportation. 49997-56-24. Available at: https://rosap.ntl.bts.gov/view/dot/27004.
Wang, Z., Dueñas-Osorio, L., and Padgett, J. E. (2014). Influence of scour effects on the seismic response of reinforced concrete bridges. Eng. Struct. 76, 202–214. doi:10.1016/j.engstruct.2014.06.026
Wei, L., Hu, K., Hu, X., Wu, C., and Zhang, X. (2022). Quantitative multi-hazard risk assessment to buildings in the Jiuzhaigou valley, a world natural heritage site in Western China. Geomatics, Nat. Hazards Risk 13 (1), 193–221. doi:10.1080/19475705.2021.2004244
Yang, D. Y., and Frangopol, D. M. (2019). Life-cycle management of deteriorating civil infrastructure considering resilience to lifetime hazards: A general approach based on renewal-reward processes. Reliab. Eng. Syst. Saf. 183, 197–212. doi:10.1016/j.ress.2018.11.016
Zaghi, A. E., Padgett, J. E., Bruneau, M., Barbato, M., Li, Y., Mitrani-Reiser, J., et al. (2016). Establishing common nomenclature, characterizing the problem, and identifying future opportunities in multihazard design. J. Struct. Eng. 142 (12), H2516001. doi:10.1061/(asce)st.1943-541x.0001586
Zaghi, A. P., Hain, J., Tafur, A., Lanning, A., and Grilliot, A., (2021). “Data collection of damage to Puerto Rico's infrastructure due to Hurricane Maria and the 2019-2020 Puerto Rico earthquakes,” in RAPID: Multi-hazard damage to Puerto Rico’s infrastructure - investigation of the interaction of hurricane and earthquakes. (Austin, TX: Natural Hazards Engineering Research Infrastructure: Cyberinfrastructure (DesignSafe) 2020–2025). Available at: https://www.nsf.gov/awardsearch/showAward?AWD_ID=2022469. version 1. doi:10.17603/ds2-jj5g-yg64
Keywords: multihazard, reconnaissance, earthquakes, Hurricane Maria, cascading effects
Citation: Hain A, Zaghi AE, Padgett JE and Tafur A (2023) Case studies of multihazard damage: Investigation of the interaction of Hurricane Maria and the January 2020 earthquake sequence in Puerto Rico. Front. Built Environ. 9:1128573. doi: 10.3389/fbuil.2023.1128573
Received: 20 December 2022; Accepted: 27 January 2023;
Published: 13 February 2023.
Edited by:
Cheryl Ann Blain, United States Naval Research Laboratory, United StatesReviewed by:
Behrouz Behnam, Amirkabir University of Technology, IranPaolo Castaldo, Polytechnic University of Turin, Italy
Copyright © 2023 Hain, Zaghi, Padgett and Tafur. This is an open-access article distributed under the terms of the Creative Commons Attribution License (CC BY). The use, distribution or reproduction in other forums is permitted, provided the original author(s) and the copyright owner(s) are credited and that the original publication in this journal is cited, in accordance with accepted academic practice. No use, distribution or reproduction is permitted which does not comply with these terms.
*Correspondence: Alexandra Hain, YWxleGFuZHJhLmhhaW5AdWNvbm4uZWR1