- 1Department of Civil Engineering, The University of British Columbia, Vancouver, BC, Canada
- 2Department de Génie de la Construction, École de Technologie Supérieure, Montréal, QC, Canada
- 3Department of Computer Science, The University of British Columbia, Vancouver, BC, Canada
The promise of Building Information Modeling (BIM) for Facilities Management (FM) is based upon building information models as reliable sources of information for decisions during a facility’s life cycle, from the planning to end of life. However, the premise of BIM as an enabler for the delivery of reliable information for FM has numerous challenges. Previous studies have shown that the quality of information provided through current design practices with BIM is inadequate for FM. These information quality (IQ) issues are mostly related to incomplete, inaccurate, inconsistent, and unintelligible facility information that ultimately reduce the usefulness of BIM-based information for FM purposes. In order to support BIM-enabled delivery of useful asset information for FM, certain IQ criteria must be met. Based on three ethnographic case studies, including the analysis of more than two thousand documented BIM for FM-related compliance issues, this research identifies ten key IQ criteria in design BIMs that must be considered to reliably support BIM use for FM, correlates these IQ criteria with key IQ dimensions identified in the literature to reflect their frequency of occurrence, and identifies sources of IQ issues in BIM for FM within design practice. A mixed-method approach for data collection from the case studies is adopted, including document analysis, semi-structured interviews, meeting observation, and a survey. The data collected are analyzed through an iterative coding process, in which the themes emerged are refined and tested as part of a grounded theory approach. This study contributes to the development of the theoretical concept of IQ in BIM for FM that is grounded in data from actual projects with stringent BIM requirements for FM and thorough compliance processes. As a practical contribution, the findings in this study should enable owners and designers to develop a more optimized asset information delivery process, increasing the value of the information in design BIMs for operations with minimal impact on current modeling practices.
1 Introduction
Building Information Modeling (BIM) as an enabler for the delivery of complete and high-quality information for Facilities Management (FM) purposes has yet to be fully realized (Dixit et al., 2019; Liu et al., 2020). This is due to the fact that the current uses of BIM in design and construction practices do not inherently capture the asset information needed for FM activities (Cavka et al., 2018; Motamedi et al., 2018). As Heaton et al. (2019) highlight, there is a lack of a framework that supports the design and development of a BIM for use during the O&M phase. Previous studies have shown that the quality and type of information developed through current BIM-enabled design practices are largely inadequate for FM purposes (Parsanezhad and Dimyadi, 2013; Kiviniemi and Codinhoto, 2014; Lavy and Jawadekar, 2014; Korpela et al., 2015; Pärn et al., 2017; Cavka et al., 2018; Pishdad-Bozorgi et al., 2018; Heaton et al., 2019; Kim et al., 2020; Patacas et al., 2020; Tsay et al., 2022). The information quality issues identified are attributable to incomplete, inaccurate, inconsistent, and unintelligible pieces of facility information, which ultimately reduce the usefulness of BIM-based information for FM purposes (Zadeh et al., 2017).
While there has been significant progress in the field of information quality (IQ) to support BIM for FM (Zadeh et al., 2017; Motamedi et al., 2018; Leygonie et al., 2022), the proposed approaches primarily focus on quality assessment methods and quality controls through checklists, without a deeper understanding of how these IQ issues originate in the first place. It is often assumed that IQ issues originate from poor modeling practices during design and lack of well-defined Information Requirements (IR) (Zadeh et al., 2017; Leygonie et al., 2022). Although the ISO 19650 standard (International Organization for Standardizatio ISO, 2018; International Organization for Standardizatio ISO, 2022a) and BIM guidelines (BuildLACCD.LACCD, 2019; U.S. General Service Administration GSA, 2011; NATSPEC, 2013; National Institute of Building Sciences NIBS, 2015; National Institute of Building Science NIBS, 2017; Australasian BIM Advisory Board ABAB, 2018; Facilities Operations and Development, 2022) have provided significant contributions to facilitate the structured organization and digitization of building information, it remains unclear how different modeling practices employed during model development can lead to IQ issues in practice. As a result, IQ issues continue to persist, even in projects with stringent information requirements and modeling standards established and enforced by the owner (Tsay et al., 2022). Therefore, further investigation into the source of these IQ issues within design practice is necessary to minimize wasted efforts in model rework and information commissioning at handover (Kim et al., 2020).
According to Liu et al. (2017), design firms must restructure their workflows and reconfigure the design process to adopt BIM processes. While ISO 19650 (International Organization for Standardizatio ISO, 2018; International Organization for Standardizatio ISO, 2022a) provides a theoretical framework for this reconfiguration, the owner’s input is still required for the definition of what IQ entails and how to ensure such IQ exists within the models. Research on IQ based on data from actual projects with well-defined information requirements and modeling standards has been limited and mostly theoretical. Hence, it remains unclear in practice what defines IQ in the models from an owner’s perspective and what changes to current BIM-enabled design practices must occur to ensure IQ upon handover.
This research addresses this gap by providing a deeper understanding of the information quality issues that arise on building projects with stringent owner requirements for BIM. This research also provides a unique lens on information quality and compliance with information requirements since it is informed by actual information quality issues encountered on three different BIM for FM projects, including the analysis of over two thousand information compliance issues. Specifically, this research focuses on the following two objectives:
1. Understand what IQ means in BIM for FM in practice and establish a link between the empirical observations and the existing theoretical concept of IQ in BIM. More objectively, this is accomplished by identifying IQ criteria in design BIMs that impact compliance with owner’s information requirements and correlating the IQ criteria with IQ dimensions identified in the literature;
2. Understand why conventional use of BIM during design often fails to meet the proposed IQ criteria in BIM for FM. More objectively, this is accomplished by identifying sources of IQ issues in BIM for FM within design practice.
This research utilized a mixed-methods approach based on three ethnographic case studies that were conducted between 2019 and 2022. Data collection included project documents, semi-structured interviews, meeting observations, and a survey with participants involved in the same three projects. As part of the data collection, the research team benefited from a thorough set of BIM compliance reports that included more than two thousand BIM for FM-related compliance issues documented during design by BIM consultants. The data were collected from different phases in each of the projects covering altogether planning, design, construction, handover, and operations (Succar, 2009). The data collected also included input from different stakeholders in the projects to increase internal validity. Data analysis involved a thematic analysis of the BIM requirements and compliance issues, resulting in the characterization of ten IQ criteria in BIM for FM. The identification of each IQ criterion emerged from the analysis of the information requirements and the IQ issues documented as part of the compliance process in the three case study projects. The ten IQ criteria were then correlated with critical IQ dimensions identified in the literature to reflect their frequency of occurrence. Finally, all data sources available were then parsed to identify design practices that were incompatible with the IQ criteria identified.
This study contributes to the development of the theoretical concept of IQ in BIM for FM through an empirical validation of key IQ issues in BIM-enabled project delivery. This analysis also highlights misalignments between idealized BIM workflows for FM and conventional designer-focused BIM workflows, presenting inherent sources of IQ issues in current design practice. As a practical contribution, this research informs owners and design consultants about: 1) the importance of clearly mapping out modeling practices for the delivery of structured asset information with BIM; 2) the importance of the information requirements, modeling standards, BIM execution plans (BEP), and other such resources for IQ assurance; 3) the potential additional effort involved in the delivery of structured asset information with BIM during design; and 4) some limitations of current design practice in the delivery of BIM for FM. Finally, the findings in this study should enable owners and designers to enable an optimized asset information delivery process, increasing the value of the information in design BIMs for operations with minimal impact on current modeling practices.
The next section presents the research background covering 1) BIM for FM and the importance of IQ, 2), IQ in BIM for FM, and 3) Modeling practices as a factor for IQ. The research methodology applied is then presented in section three, including the different data collection methods and tools employed. In section four, the characterization of ten IQ criteria is introduced and correlated with information quality dimensions, and in section five, the identified sources of IQ issues in BIM for FM are discussed. Finally, section six discusses the findings and conclusions.
2 Literature review
2.1 BIM for FM and the importance of IQ
Considering that BIM can be used for various purposes, supporting different activities performed by different stakeholders, and at different phases of a project, it is crucial to define IQ in BIM based on a specific purpose (Succar et al., 2016; Rojas et al., 2019). Some researchers have explored methods to improve the quality of BIM for architectural design purposes (Choi et al., 2020). Similar work is required for models being used for FM (Leygonie et al., 2022).
Given the potential value that the asset information contained in BIM can bring to building owners (Beatty et al., 2013; Teicholz, 2013), there has been increasing interest in BIM uses in FM (Gao and Pishdad-Bozorgi, 2019). The discipline of FM, however, constitutes an extensive field encompassing multidisciplinary and independent disciplines whose overall purpose is to maximize building functions while ensuring occupants’ wellbeing (Becerik et al., 2012; Atkin and Brooks, 2013). So even within FM, there are many ways in which BIM can be used (Gao and Pishdad-Bozorgi, 2019). A main area of interest in BIM for FM, central to this study, is the handover of asset information for integration with the owner’s Computerized Maintenance Management Systems (CMMS). This asset information delivery process is described in detail by (Tsay et al., 2022) (Figure 1). BIM serves as an initial source of structured information for the development of an asset information database, in which construction teams can then integrate more relevant information for operations and maintenance. Therefore, the importance of IQ in BIM for this process is to ensure that all relevant information can be reliably extracted in an automated manner (indicated by “e” in Figure 1).
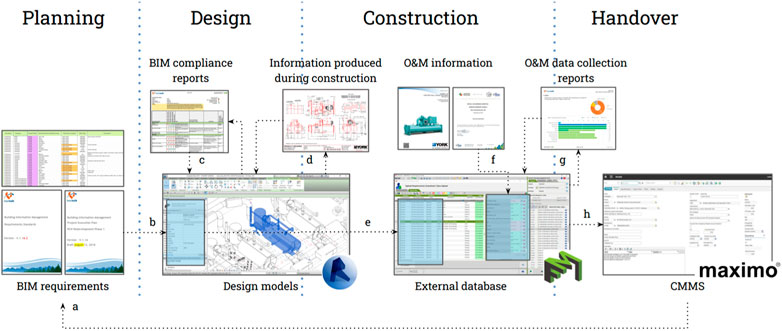
FIGURE 1. Schematic representation of the asset information delivery process in BIM (Tsay et al., 2022). (A) Definition of the information requirements (BIM requirements). (B) Model development observing BIM requirements (creation of digital assets in BIM). (C) Validation of the asset information in BIM (compliance with BIM) requirements). (D) Model updates based on design changes during construction. (E) Data extraction from design models into an external database (creation of an asset registry) (F) Association of asset information from construction phase with respective digital assets in the external database. (G) Validation of the asset information in the external database. (H) Asset information integration into FM systems.
For data extraction to occur successfully, first the BIM data exchange method must be defined, including the mapping between the fields of information in the different databases. Then, the information in the design models must be structured in a compatible way with the defined extraction method. As mentioned by Gholami et al. (2015): “To yield any useful outcomes, the input data provided in the first instance should meet certain quality threshold criteria” (Gholami et al., 2015). Defining data exchange methods and structuring the data in the models are two intrinsic aspects of interoperability, which has been critically recognized as one of the critical pillars for BIM-based design, construction, and facility management (Lee et al., 2021).
Regarding the BIM data exchange method, COBie (National Institute of Building Sciences NIBS, 2015) has emerged as a prominent solution. COBie is an open data transfer specification initially developed by the U.S. Army Corps of Engineers to facilitate the delivery of managed asset information by using low-level formats such as Excel spreadsheets (Sabol, 2008; East B and Carrasquillo-Mangual M, 2022). COBie is built upon the concept of model view definitions (MVD), which are predefined subsets of information contained in an Industry Foundation Class (IFC) file to facilitate BIM data exchanges between specific domains (Lee et al., 2018). In theory many potential benefits have been associated with the use of COBie (Lavy and Jawadekar, 2014; Patacas et al., 2014; Yalcinkaya et al., 2016; Yalcinkaya and Singh, 2019; East B and Carrasquillo-Mangual M, 2022). In practice, however, there are still several challenges that prevent COBie from solving the interoperability issue (Anderson et al., 2012; Patacas et al., 2015; Yalcinkaya et al., 2016; Kumar and Teo, 2020; Abdirad et al., 2021).
While there’s been significant progress in the creation of open standards, tools, and methods for BIM data exchange (Lee et al., 2014; Afsari et al., 2017; Patacas et al., 2020; Lee et al., 2021; Son et al., 2022), challenges have been observed in projects with or without COBie, particularly with how the relevant information for FM is structured in the models in the first place (Parsanezhad and Dimyadi, 2013; Lavy and Jawadekar, 2014; Korpela et al., 2015; Pärn et al., 2017; Pishdad-Bozorgi et al., 2018; Heaton et al., 2019; Patacas et al., 2020; Tsay et al., 2022). Lee et al. (2021) adds that because of error-prone data mapping problems, the adoption of IFC for exchanging and sharing BIM data is frequently limited with severe data integrity issues. Any BIM data exchange method, including COBie, requires the data in BIM to be structured in a specific way in the models, which is not always aligned with the conventional use of BIM in design activities. According to Cavka et al. (2018), it is a given that a project BIM intended for FM use is modeled differently from a design or a construction BIM. This is illustrated in a case study by Heaton et al. (2019) wherein “the asset management team notes that the BIM model is simply not fit for purpose and does not meet their requirements, with often bulk COBie excel sheets handed over with little structure in place”. This frequently observed lack of structure in design models denotes a whole other aspect of the interoperability problem that has not been given sufficient attention in the literature, which will be discussed next in terms of modeling practices and IQ in design models.
2.2 IQ in BIM for FM
According to Becerik et al. (2012), significant amounts of non-geometric data are added to the model during the design phase. However, the information generated merely by the utilization of BIM tools during design is not necessarily adequate for FM. This has been emphasized in several studies. Kim et al. (2020) found in a case study that although BIM was used for the design of a project, a considerable amount of the non-geometric information embedded in the handover model was inaccurate and unnecessary. Different terminologies and varying templates for ordering and representing information from different vendors created interoperability issues that required a significant amount of time to fix. Cavka et al. (2018) investigating a project without predefined BIM requirements found that, from an owner’s perspective, the models can require significant manipulation and preparation to have their data assessed and analyzed. Not only that, but relevant data was often unavailable in the models. For example,: “Undefined relationships between equipment, system, and spaces lead to not being able to identify the location of equipment, the system that equipment belongs to, and which space a system serves”(Cavka et al., 2018). Moreover, Kiviniemi et al. (2014), revealed that “BIM used for design and construction practices does not contain all, or even a significant part of the necessary information for FM practice nor were its assemblies created in a way that would benefit FM”, which was based on the Manchester Town Hall Complex case study.
While it’s been well documented that the information generated in BIM during design is not necessarily adequate for FM, very few studies have attempted to develop a deeper understanding about IQ in BIM for FM. As pointed out before by Poirier et al. (2017) and Zadeh et al. (2017), there is still a need for further research in IQ to establish common ground for mutual understanding and knowledge exchange within the AECOO (architecture, engineering, construction, owner, and operator) domain. Quality is a complex concept that has been studied through different perspectives and has undergone great evolution over time (Donato et al., 2018). According to (Juran and De Feo, 2010), quality can be defined in terms of 1) conformance to the agreed requirements of the customer and 2) a product or service free of deficiencies. In most cases, quality is associated to a product, a service, a project or a process and requires the setting up of a formalized system, quality management, that allows maximum results (effectiveness), using the best human resources, time and economic resources available (efficiency) (International Organization for Standardizatio, 2013).
In computer science research, many IQ dimensions have been proposed to help the assessment of IQ as an initial step towards solving IQ issues as shown by Lee et al. (2002) in a summary of academic research on the multiple dimensions of IQ (Table 1). However, the categorization of IQ dimensions depends on the context of the practitioners (Lee et al., 2002). According to Wang and Strong (1996), the “fitness for use” (or the information usefulness) is the major criterion for IQ; a piece of information is of high quality when it is “useful” for its consumer. Therefore, the identification of consumer’s information needs is an essential step in assessing the quality of information systems like BIMs (Zadeh et al., 2017).
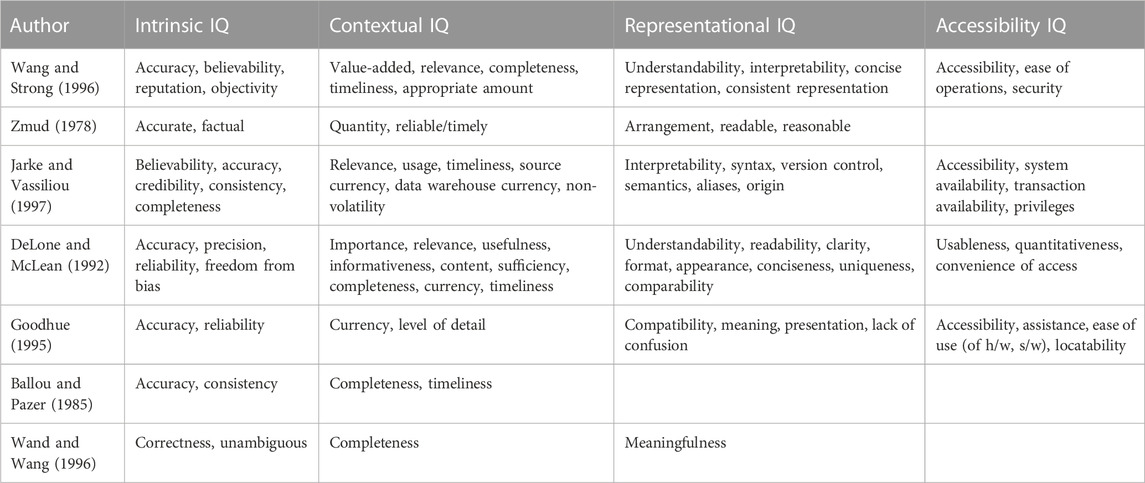
TABLE 1. The academics’ view of information quality (Lee et al. 2002).
In the AECOO domain, considering BIM as the information system, a few studies have been conducted. Berard (2012) presented a framework for assessing design information quality from the builder’s perspective. Du et al. (2014) introduced benchmarking metrics for the IQ Assessment of BIM cloud performances including IQ related metrics, such as model quality, accuracy, and usefulness. Solihin et al. (2015) introduced a testing methodology for validation of IFC files. Lilis et al. (2018) introduced a data quality check procedure in BIM for energy performance simulation, which included three types of information quality criteria: consistency, correctness and completeness checks. They also implemented and tested the checking procedure in two case-study buildings. Donato et al. (2018) assessed the information quality (e.g., adequacy, readability) of BIM models during the architectural design process. Although these studies do not have a specific FM focus, they provide a useful model for developing structured IQ tests and essential IQ criteria, which is utilized in this research.
Another relevant progress towards IQ in AECOO is the publication of ISO 19650 (International Organization for Standardizatio ISO, 2018), which provides a framework for information management using BIM. ISO 19650 prescribes the use of Level of Information need for each information deliverable, which should include the appropriate determination of quality, quantity, and granularity of information. The concept of level of information need is further developed in EN 17412-1 (National Building Specification, 2020) that recently became ISO 7817 (International Organization for Standardizatio ISO, 2022b). In terms of IQ, ISO 19650 emphasizes understandability of the information across stakeholders and mentions the importance of agreement on: information formats; delivery formats; structure of the information model; the means of structuring and classifying information; and attribute names for metadata. Recently, ISO 19650-4 (International Organization for Standardizatio ISO, 2022a) on information exchange was published, providing explicit process and criteria for each individual information exchange. The criteria generally consist of: Common Data Environment (CDE), Conformance, Continuity, Communication, Consistency, Completeness, and other informative criteria.
In the specific context of BIM for FM, Kasprzak et al. (2013) were among the first to discuss IQ. They discussed the procedure developed by Pennsylvania State University to plan the validity and reliability of the model geometry and information for FM. This early research on IQ quality in BIM for FM touches on information requirements for FM, relationships between elements, and risk prioritization. Zadeh et al. (2017) were the first to properly import the theoretical concept of IQ into BIM for FM. They categorized five IQ dimensions based on the research works of Wand and Wang (1996) and Lee et al. (2002) that they translated for AECOO domain according to the FM needs. They also introduce a framework for information quality assessment (IQA) of BIMs for FM uses. The framework is structured based on essential FM subjects: assets, spaces, and systems, and the model characteristics: objects, attributes, relationships, and spatial information. The IQ dimensions categorized in their work (i.e., Completeness, Value Accuracy, Consistency, Well-formedness, and Understandability) are used to correlate the IQ criteria defined in this paper. Motamedi et al. (2018) (4) identified BIM quality assessment criteria considering FM requirements and proposed a practical guideline for the preparation of a FM-BIM. As part of the guideline, they developed a quality control checklist to ensure the usability of the delivered FM-BIM provided. Considering that design and construction models contain information that is not useful in the O&M phase, they also provide a list of items to be purged and cleaned-up from the models. Kumar and Teo (2021) investigated the challenges associated with the COBie datasheet verification and consistency checking process, especially during data drop stages, and developed a solution to mitigate these challenges. Leygonie et al. (2022) developed a comprehensive framework for quality management of BIM models and reported a notable increase in the quality of the deliverables prior to the owner’s final verification through the use of the QC tools and the application of the proposed procedures. Many of these studies propose the use of tools to automate quality checks, such as Dynamo, Revit schedules, Revit Model Review, Revit Model Checker, Navisworks, Solibri Model Checker, COBie QC Reporter, and BIM Assure. Leygonie et al. (2022) however, points out that there are limitations for the use of these individual tools for the breadth and complexity of information quality issues.
While there’s been significant efforts to improve the information quality (IQ) in BIM for FM, most of the proposed approaches are focused on quality assessment methods and quality controls through checklists, without a deeper understanding of how these issues originate in the first place. Even though parts of the quality assessment can be automated, there is still a need to minimize wasted efforts in model rework and information commissioning at handover (Kim et al., 2020). Therefore, further investigation into the source of these issues within design practice is still necessary to mitigate their occurrence.
2.3 Modeling practices as a factor for IQ
Zadeh et al. (2017) highlighted that while any interactions with the model might be a source of IQ problems, including activities related to data exchange between different project participants and the data consumption, modeling practices have the most impact on assuring the IQ of models. The term “modeling practices” will hereinafter refer to a subset of activities within design practices that are related to the creation of content in BIMs. Researchers that investigated how a BIM model should be designed to support its exploitation within the O&M posited that “there is a clear gap of knowledge on how to structure a BIM model that allows its efficient use in the O&M phase” (Heaton et al., 2019). According to Cavka et al. (2018), “ensuring appropriate model structure entails modeling practices that are required for computing required FM information correctly from a BIM, and for enabling information exchange between the model and the owner’s FM applications”. Monteiro and Martins (2013) confirm the importance of modeling practices by demonstrating how specific information input methods are required when creating BIM for different purposes and clients. In their study, they discuss how the modeling practices employed for the design of several construction elements can affect the information output for quantity takeoff purposes. They also report that significant additional work during the modeling phase was required in the design process in ArchiCad to increase the IQ for quantity takeoffs. Liu et al. (2022) also highlights the importance of modeling practices for IQ in BIM and proposes a data-driven framework based on BIM and knowledge graphs to represent unstructured BIM data for automatic inferences of auditing results of BIM model mistakes. Modeling practices are a subject of concern in other industries that also use parametric CAD (Computer Aided Design) (Bodein et al., 2014; Camba et al., 2016). In the automotive industry for example, formal modeling methodologies can offer significant advantages over non-structured approaches, especially when targeting CAD reusability (Bodein et al., 2014).
Because modeling practices can compromise the IQ in BIM, many organizations provide guidelines for modelers to assure the quality of BIMs, such as: The LACCD Building Information Modeling Standards (BuildLACCD.LACCD, 2019), GSA Building Information Modeling Guide Series: 08—GSA BIM Guide for Facility Management (U.S. General Service Administration GSA, 2011), BIM and LOD (NATSPEC, 2013), National BIM Standard United States Version 3, Construction operation building information exchange (COBie) version 2.4 (National Institute of Building Sciences NIBS, 2015), National BIM Guide for Owners (National Institute of Building Science NIBS, 2017), The Asset Information Requirements Guide: Information Required for the Operation and Maintenance of an Asset (Australasian BIM Advisory Board ABAB, 2018), and OSU BIM Project Delivery Standards (Facilities Operations and Development, 2022). Leygonie et al. (2022) (Leygonie et al., 2022) argues that a problem with most of these documents is that they provide generic guidelines for model preparation, often lacking details on how to ensure the ease of use, efficiency, interoperability, and maintainability of FM models. To address this gap, Leygonie et al. (2022) and Motamedi et al. (2018) have proposed comprehensive checklists, covering modeling practices that should be checked to ensure IQ.
Given the importance of modeling practices to ensure high IQ, and considering that modeling best practices can vary according to the defined uses of BIM, it is critical for owners to define and include modeling standards in the IR. This is reinforced by Leygonie et al. (2022), whose main findings were “the importance of working with the owners to develop well-defined IRs and modeling best practices and to include these in the contract. Failure to do so resulted in deliverables lacking relevant information and the need to make major corrections to the models to render them useful for the O&M phase of the facility”. Tauriainen et al. (2016) and Motamedi et al. (2018) (4) add that ideally these modeling standards should be created by the owner of the project in cooperation with design disciplines at the beginning of the project before the modeling process has been initiated. However, despite the availability of modeling guidelines in the industry, very few studies have documented the development of BIM for FM with modeling standards included as part of the IR. Therefore, it is unclear how different modeling practices employed during design can lead to IQ issues in practice. There is still very limited empirical knowledge about the efficiency of having modeling standards as a measure to mitigate IQ issues. Furthermore, since the conventional uses of BIM in design are focused on 3D visualization, automation of documentation, and clash detection (Moreno et al., 2019), the extent to which the modeling practices will have to change in FM-enabled BIMs is not yet clear.
To summarize, the lack of IQ in BIMs has been pointed out as a major challenge in the asset information delivery process, which is a central aspect of BIM for FM. It has also been emphasized that modeling practices employed during design are the main cause of IQ issues. To address this issue, significant effort has been put into defining IQ dimensions and criteria which with two purposes: 1) Improving the assessment of IQ with automated processes (identifying and correcting IQ issues that have already occurred), and 2) Informing changes in modeling practices through well-developed information requirements and inclusion of modeling standards (preventing the occurrence of IQ issues). Despite the advances in the automation of IQ assessment and control, there is very limited empirical knowledge on how to mitigate the occurrence of IQ issues in design. The development of modeling standards and their inclusion in the information requirements has been proposed as a solution. However, it is still unclear how different modeling practices employed during design can lead to IQ issues in practice. Furthermore, the occurrence of IQ issues in design models continue to be a challenge, even in projects with stringent BIM requirements, modeling standards established and rigorous enforcement by the owners (Tsay et al., 2022). To conclude, the main gaps identified in this literature are that, despite the evident importance of IQ in BIM for FM, it remains unclear what defines IQ in the specific context of BIM for FM and how different modeling practices employed during design can lead to IQ issues in practice. Therefore, further investigation into the source of these IQ issues within current design practices is necessary to inform what changes must occur to ensure information quality upon handover.
3 Methodology
This research utilized a mixed-method methodology to address the two objectives that were articulated for this research study: 1) identifying the key IQ criteria in design BIMs and correlating these with IQ dimensions identified in the literature, and 2) identifying the sources of IQ issues in BIM for FM within design practice. The following sections describe the three case study projects and the data collection and analysis methods utilized.
3.1 Case study approach
This research employed a multiple case study approach to provide an in-depth investigation into particular instances related to the research subject (Fellows and Liu, 2022). This strategy was suitable for answering questions related to what is IQ in the given context and how the IQ issues arise during design phase (Yin, 2009). Case studies combine several data collection techniques, including archival research, interviews, questionnaires, and observations (Eisenhardt, 1989), allowing the research team to holistically explain and understand the dynamics of the phenomenon under study (Yin, 2009). The goal of using case studies as the investigation method was to analyze actual project data in its own particular context, without focusing on statistical generalizability. Although case studies cannot be easily generalized, they provide in-depth accounts of phenomena in context and can help practitioners judge whether a specific technology or process can benefit their own organization or projects (Bakis et al., 2006). Three longitudinal ethnographic case studies were conducted, hereinafter referred to as projects A, B, and C (Table 2), in which the research team collaborated closely with the stakeholders during different phases of the projects. The main criteria for the selection of these projects were the owner’s intent of using BIM to support FM, the presence of a thorough and comprehensive set of BIM Requirements established before the design phase, and a well-documented compliance process.
3.2 Data collection
The data collection from projects A, B, and C included semi-structured interviews with key participants and project documents related to the BIM process. The research team also collected data through meetings observations on projects A, and C, and performed surveys with participants from projects A. The survey from project A was conducted as part of a research collaboration with the design company from project A. While limited level of access to data collection with the design teams in projects B, and C did not allow for a survey, similar questions were asked to the design teams on project B, and C through the interviews. A more detailed description of the data collection methods and types of data collected from each project is provided in Table 3.
Forty-eight semi-structured interviews of approximately 1 hour each were conducted with industry experts from different organizations involved in project A. The interviews were recorded and analyzed using the categories that emerged from the document analysis. Open-ended questions were used in the interview to encourage respondents to provide a more detailed response and to build rapport between the respondent and the interviewer (Richards and Morse, 2012). Questions about the BIM process for FM were asked covering the information workflow, scope of each stakeholder, tools, challenges, familiarity with the BIM requirements, perceptions of value, and IQ in the models. Each interview also had a different set of bespoke questions focused on specific aspects of the asset information delivery process pertinent to the interviewee’s area of expertise. The questions were aimed at 1) filling the gaps of information not captured during document analysis and meeting observations, 2), addressing new emerging questions from previously collected data, and 3) capturing individual perceptions of IQ in BIM for FM.
For the number of respondents required to achieve data saturation, depending on the nature of the study Kvale (2007) recommended 15 ± 10 respondents because of the typical time and resources constraints. Like other studies within the construction industry, the quality of experts (i.e., having the required qualifications in the field under study) is preferred over quantity (Abowitz and Toole, 2010). A total of 20 local experts in BC were interviewed, being all directly involved with projects A,B, and C. Sample size was based on a judgment, in coding and analyzing, of theoretical saturation of categories, which implies that “no new properties emerge and the same properties continually emerge” (Glaser, 1978) and that gaps in major categories were more or less filled (Glaser et al., 1968). It is worth mentioning that saturation is always a subjective judgment and the decision to stop theoretical sampling, using the methodological guidelines, was influenced by the scope of the research project, particularly in terms of time and resources (Breckenridge and Jones, 2009).
The research team members attended 22 meetings in project A that were being held regularly by the owners during the end of construction and handover. During these meetings, the owners, BIM consultants, asset data consultants, and CMMS consultants discussed details of the asset information collected from BIM, and utilization of the asset information for the development of preventive maintenance job plans. The research team also attended 23 meetings that were held regularly by the design team in project C. During these meetings, the BIM consultants, design team, construction managers, and owners discussed details about the implementation of BIM and compliance with the BIM requirements for FM during design. The approach used by the research team during these meetings was participant observation, in which notes were taken and questions were asked only when the principal investigator was invited to do so.
A survey was conducted online in June 2019. The purpose of the survey was to increase the reach of the data collected towards different design team members with different roles considering the limited time and resources for interviews. It comprised 12 multiple-choice and open-ended questions. It received responses from 19 participants from the design firm who were involved in the design phase of project A. Given the small number of participants, the analysis focused on the answers to the open-ended questions about the use of BIM requirements for FM, including challenges, and impacts on their current design processes and workflows. Open-ended questions are useful for surveys that target a small group of people because there is no need for complex statistical analyses, and the qualitative nature of the questions solicits valuable input from each respondent. Anonymity was guaranteed to motivate open feedback about problems and challenges.
The data collected from the interviews, meeting observations and the survey were analyzed through an iterative coding process (Miles et al., 2014), in which themes emerged, were refined, and tested as part of a grounded theory approach (Phelps and Horman, 2010). Two initial categories of codes were used to parse the collected data. The first one being related to different kinds of IQ issues in BIM for FM, and the second about sources of these IQ issues within design practices.
Detailed documentation of the BIM compliance process that happened in projects A, B, and C was a key data source for this study. During design, model audits were performed regularly throughout different stages of the design. The model audits were conducted by BIM consultants hired by the owners to ensure that the models were suitable for the defined goals (More information about this compliance process can be found in Tsay et al. (2022)). As an output of this compliance process, several reports were generated containing several examples of compliance issues in the BIM process that would compromise the data quality and reliability of the models (see Figure 2). Each report contained an overall summary of high-priority noncompliance issues within the models and documents received. Representing the owners, the BIM Consultants reviewed each model’s document structure setup, cross-referenced with guidelines set forth in BIM requirements and the BEP, using Autodesk Revit, Autodesk Navisworks, and proprietary software. In total, 2,177 issues were analyzed. The issues came from model audits on 111 different Revit model files from the three different projects.
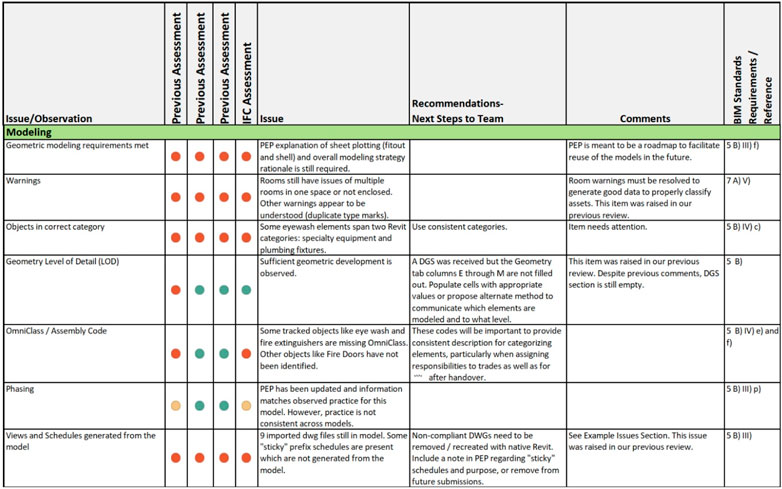
FIGURE 2. Excerpt of a model audit report (Project A) containing a model review matrix with different kinds of compliance issues.
Another key data source for this study was the modeling standards developed by the owners as part of their information requirements. The information requirements in Projects A, B, and C, covered different aspects of how BIM was meant to be used by the delivery team in order to meet the owner’s objectives for FM, such as what information should be present in the model, when, where, by whom, and how. A detailed description of one of these information requirements documents is provided by Tsay et al. (2022). For this study, the data collection on the BIM requirements focused on how the information needed to be structured in the models by the design team, which are hereinafter called modeling requirements [which is similar to model structure requirements defined by Cavka et al. (2018)]. These requirements represent constraints on how the virtual objects and their related information are created in BIM. “Of prime importance for the (Owners) is that the inherent structure of the design models is capable of being the foundation for the collection of additional data and onward mapping to end-use software solutions. This requires that the Design team ensures that those assets tagged as required and tracked are modeled as required.” (BIM Requirements Overview projects A, and B) Given the variety of ways that BIM authoring tools can be used to generate model content, defining lists of parameters/attributes for each category of objects in the BIM authoring tool may not be sufficient. The way in which BIM authoring tools are employed during design has a significant influence on the quality of the asset information extracted from the models. Each step of a stairway, for example, can be modeled as a separate floor object instead of using the stairs function in a BIM authoring tool (Revit in this case). By doing so, the stairs can be perfectly represented geometrically. However, depending on how the asset information is extracted from the models, calculations of floor areas and other parameters associated with stairs will be subject to errors. Similarly, several other aspects of modeling practice need to be considered as part of the BIM requirements to ensure that the information is extracted for the creation of the asset information database without errors. A few examples are presented in Table 4 below.
3.3 Data analysis
This section describes the different types of data analysis conducted for each of the research objectives. Figure 3 provides a graphical overview of the research process including the steps, inputs and outputs of the analysis.
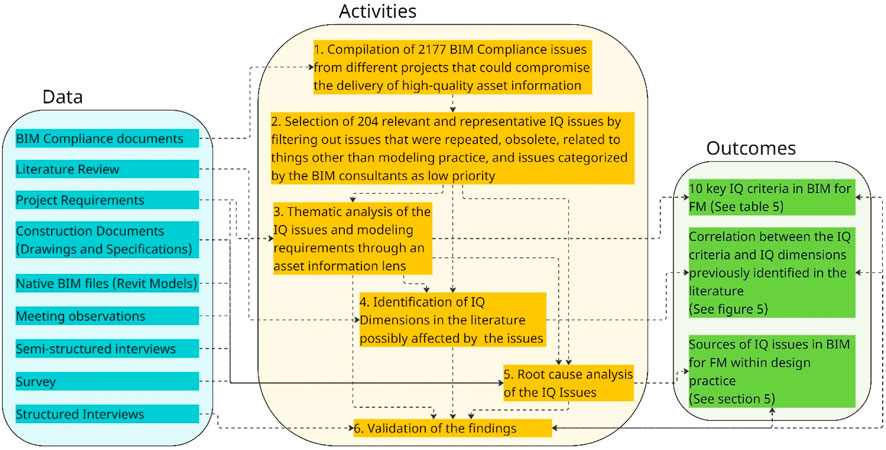
FIGURE 3. Overview of the research approach including different data sources (inputs), activities (steps) and outcomes (outputs).
3.3.1 Data analysis methods used for objective 1 (activities 1-4)
A thematic analysis using “asset information” as a unit of analysis was conducted on BIM requirements and compliance documents. Thematic analysis was employed for its usefulness to capture the complexities of meaning within a textual data set (Guest et al., 2011). Wand and Wang (1996) defined data quality dimensions derived from possible failures of the representation. A similar approach was employed for objective one, in which BIM compliance issues, documented as part of construction projects, were analyzed to elaborate on the concept of IQ in BIM for FM. In other words, the key IQ criteria in BIM for FM were derived from possible failures resulting from model compliance issues. Additionally, each IQ issue was correlated with an IQ dimension defined in the literature (Zadeh et al., 2017).
3.3.2 Data analysis methods used for objective 2 (activity 5)
Objective two involved the analysis of project requirements, Revit models, construction documents, interviews, and surveys with design team members to investigate how or why their conventional use of BIM does not necessarily meet the IQ requirement specified for the delivery of asset information. As a preliminary step to data quality improvement, some frameworks start by performing an analysis of the root causes of data quality issues (Cichy and Rass, 2019). A similar approach was used here, in which the root causes of IQ issues in BIM for FM were investigated by identifying practices that were not compatible with the IQ criteria defined in objective one.
3.4 Validation/verification
Although validation occurs naturally in the ethnographic research process (Abowitz and Toole, 2010), triangulation has also been identified as a strategy for validating data and constructs (Thabet et al., 2016). To increase construct validity and overcome issues of bias (Love et al., 2002), multiple sources of evidence were used, and the data collected from different sources (i.e., interviewees, documents, BIM models/BIM-FM platform) was triangulated. The triangulation also happened across the data collected from three different projects. In addition to triangulation, dedicated meetings were conducted with Architects and BIM experts involved in projects A, B, and C for further validation. During these meetings, the findings of this study were presented to the experts and adjustments were then made to incorporate their feedback. The experts were also asked how the findings from projects A, B, and C compared with their experience in other projects to increase external validity.
4 Key IQ criteria and their correlation with IQ dimensions
This section describes the 10 IQ criteria that were identified based on the BIM for FM requirements established and verified from the owner’s perspective (Table 5). Each IQ criteria emerged from the analysis of the information requirements and the IQ issues documented as part of the compliance process in projects A, B, and C. These criteria translate essential aspects of how asset information should be organized in the models to prevent the occurrence of IQ issues in practice. These key criteria can also be understood as key modeling practices required to ensure IQ in BIM for FM. A name was given to each criterion identified in the data analysis. Although some of the names are similar to the IQ dimensions previously defined in the literature such as “representation” (Wand and Wang, 1996), and “clarity” (DeLone and McLean, 1992), the criteria identified in this study are contextualized in BIM for FM and with the objective of informing modeling practices.
Based on the description of the IQ issues, we then correlated the IQ criteria with the essential IQ dimensions identified in the literature, which specifically included completeness, accuracy, redundancy, well-formedness, and understandability (Zadeh et al., 2017). We then created a Sankey diagram to illustrate the distribution of compliance issues and the relationship between the identified IQ criteria in BIM for FM and IQ dimensions established in the literature (Figure 4). Although this diagram provides a quantitative sense of what are the most common categories of issues in the case studies, it does not take into consideration the potential risk and level of priority in each issue.
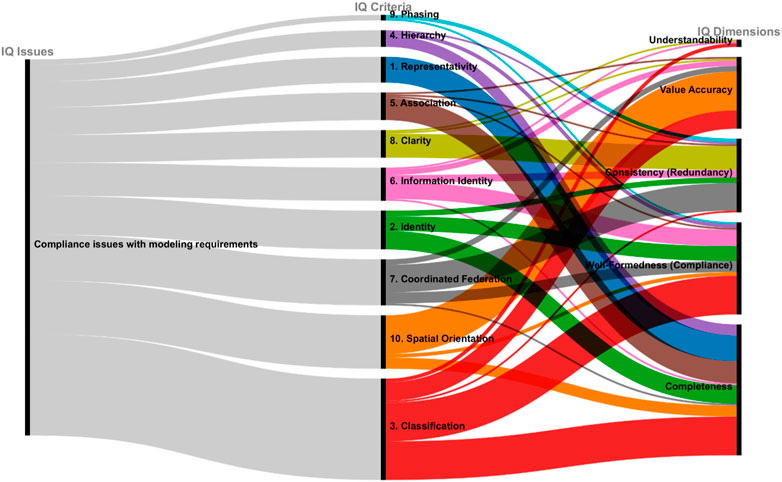
FIGURE 4. Sankey diagram illustrating 1) the distribution of 204 selected IQ Issues (compliance issues with modeling practices) into the 10 key IQ criteria identified on the left side (in grey); and 2) the relationship between identified key IQ criteria and IQ dimensions established in the by Zadeh et al., 2017 (Zadeh et al., 2017) on the right (Multiple colors).
Next, each asset IQ criterion is explained in further detail.
4.1 Representativity
The first criterion identified was related to the digital representation of the assets in the models. It was critical for the owners that all predefined assets were represented in the models using a virtual object as an information container. Although it is a basic premise of BIM, depending on what are the other intended uses of BIM in the project and on the type of assets, this criterion can have less importance for the design consultants. For the production of drawings alone, not representing certain assets with a virtual object can be acceptable as long as they are represented in the drawings. Issues were observed in which relevant assets for the owners were represented by lines and annotation elements in the drawings. Lines and annotations in Revit cannot be used to convey parametric asset information. As an example, the BIM consultants identified “Trench drains shown on sheet A-125a as filled regions rather than modeled elements. This means that they have to be drawn in every view, which is an error-prone and manual process. It would be expected to simply show plumbing fixtures as placed by MEP.” (Model audit review). Another example is shown in Figure 5, in which mechanical assets were found to be represented by annotation tools in Revit.
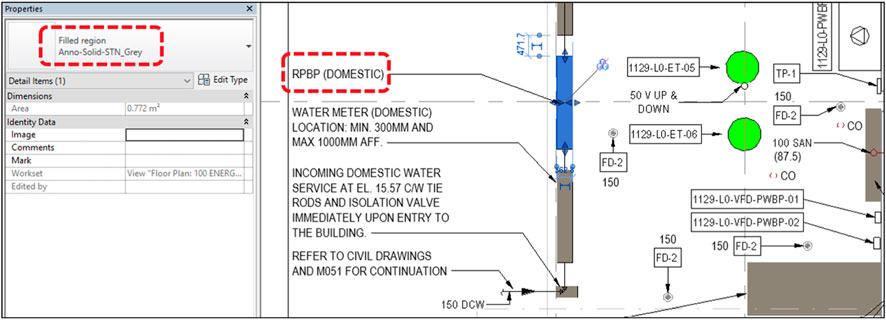
FIGURE 5. Example of issue related to Representativity - Mechanical objects (Reduced Pressure Backflow Preventer) represented in Revit by annotation elements (filled region).
Given that not all objects in the model are necessarily relevant assets for FM, the owners had to specify which assets are going to be tracked, and what naming convention will be used for these assets. In project A, this specification was provided through a spreadsheet called “object list” (Figure 6), in which a list of assets required for FM was provided with a code and description.
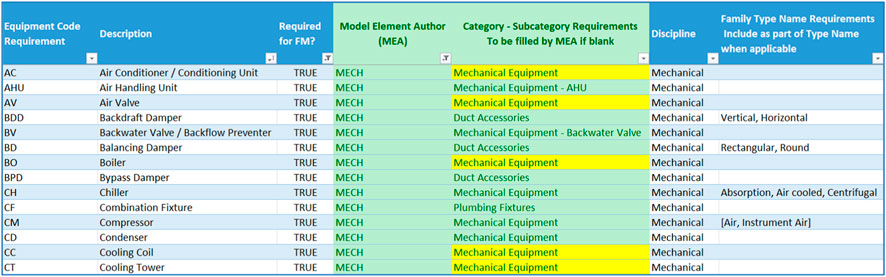
FIGURE 6. Excerpt from the object list spreadsheet provided as part of the modeling standards in project A.
4.2 Identity
The second criterion identified was related to the individual identification of the digital assets in the models. It was critical for the owners that all relevant digital assets could be tracked by a unique ID using a single predefined parameter. This would ensure that all the assets identified in the drawings can be individually tracked in the models and later in the external database. It was observed that in current practice different design teams have different workflows to identify and tag objects in the drawings. For the production of drawings alone, not every virtual object needs a unique ID unless it is shown on a tag or on a schedule. The use of a unique parameter to convey ID also does not necessarily represent an issue when BIM is not intended for FM. This criterion emerged from frequent issues related to failure to provide a consistent and unique ID to virtual objects. As the example below shows (Figure 7), different parameters were used to convey identification information from what was proposed in the requirements.
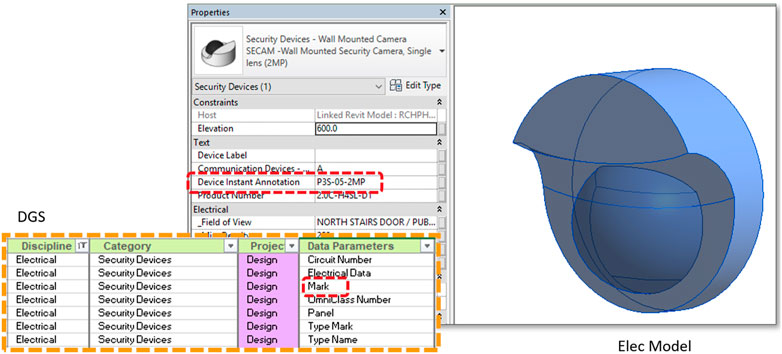
FIGURE 7. Example of issue related to Identity—Alternative parameter being used to convey asset identification information. (On the right, a Revit screenshot with ID information “P35-05-2 MP” under a parameter named “Device Instant Annotation” instead of the parameter “Mark”. On the left, a screenshot of the DGS spreadsheet with the proposed parameter name to be used “Mark”.).
Even though every object created in Revit has an ID number generated automatically, this Revit ID is not usually the number or code displayed in the drawings’ tags or physical tags onsite. Therefore, a specific parameter must be defined to consistently convey this information. Besides, owners often employ specific conventions to create their asset identification number or code, which in this case must be predefined and explained to the designers. As part of the Data and Geometry (DGS) Spreadsheet (see detailed explanation of the DGS as part of the information requirements in Tsay et al. (2022), a specific Revit parameter (i.e., “Mark”) was required for all the tracked assets (Figure 7). It was also mentioned in the BIM compliance documents that “whatever code is shown on tags or schedules should be in either Mark or Type Mark parameters” (Project A- Model audit review).
4.3 Classification
The third criterion identified was related to the classification of digital assets into well-defined groups. It was critical for the owners that the digital assets were following a pre-defined classification system to a predefined level of granularity and naming convention. In order to manage a large number of assets, they have to be categorized into groups. These groups have to be compatible with the groups of assets used in FM systems, so the data can be properly translated between the BIM database and the CMMS database. Revit categories do not offer a sufficient level of granularity for the variety of elements a model may contain. For example, within the Revit category “Mechanical Equipment”, 43 different types of objects were to be tracked in project A. Revit “Family” and ‘type’ names are also usually not reliable. In this case, existing classification systems have to be adopted, such as Omniclass, which is already present in most Revit families. In the current practice of the design firms studied, virtual assets did not follow a specific classification system consistently. The classification of objects into categories and families usually provides a sufficient level of granularity for the internal management of information within the design teams. Furthermore, design team members can create virtual objects under different categories and name families, and object types, according to their own internal naming conventions. Several issues related to inconsistent adoption of classification systems were observed. The use of Revit categories to classify virtual objects also presented issues such as: “Object list suggests Fire Extinguishers would be modeled under specialty equipment but are actually modeled as fire alarm devices.” Figure 8 shows another example with two eyewash stations in the same model, one under the “Plumbing fixture” category and the second under the “Specialty equipment” category. A query based on category would render inaccurate information about the quantity of these assets.
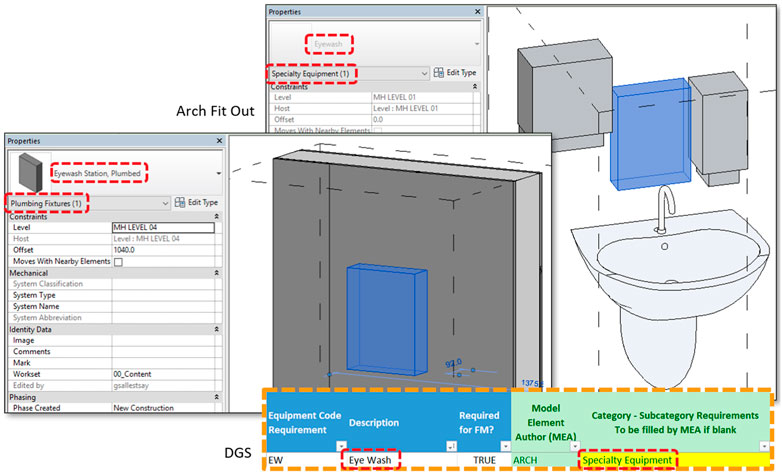
FIGURE 8. Example of Classification issue—Lack of consistent classification and naming (On top and in the middle, Revit screenshots of two eyewash stations with inconsistent naming and classification into categories. At the bottom, a screenshot of the DGS spreadsheet with the proposed naming and category to be used).
The classification of some of the assets as part of specific mechanical, electrical, or plumbing systems is also important for FM. In Project A, a list of system names was provided to be used for each mechanical system, but different system name prefixes were being used (Figure 9). Without a naming convention, queries with minimal spelling divergences can return inaccurate information.
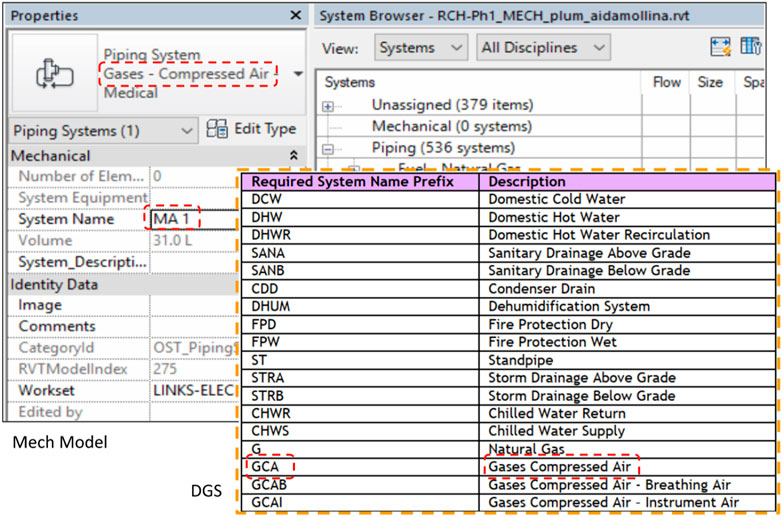
FIGURE 9. Example of classification issue—Mechanical model with mechanical system names differing from the names proposed in the BIM requirements. (Mechanical model behind and a table from the BIM Requirements in front).
Although Omniclass is already present in most Revit families, not all FM teams adopt Omniclass as their classification system. Therefore, a classification of assets in categories and systems must be specifically defined by each owner. The naming convention for the categories and systems must also be defined. In projects A, B, and C, OmniClass was required as a parameter for all required assets in DGS. The Object list (Figure 6) defined under which category every tracked asset should be modeled.
4.4 Hierarchy
The fourth criterion identified was related to the hierarchical relationships between the digital assets. It was critical for the owners that the parent-child relationships between the pre-defined assets were indicated following a pre-defined hierarchical structure. This criterion was important to ensure the identification of the functional relationship between specific assets, namely, parent and child, which is especially important for the maintenance of mechanical equipment. For example, to perform the maintenance of an Exhaust Fan (parent), it is necessary to have specific information about the motor connected to this fan (child). It was observed that, depending on the type of asset, the current practice does not involve providing parent-child relationship between the virtual objects following a specific standard. In many cases, this information is conveyed through schematic drawings and one-line diagrams, which do not translate into parametric information that can be extracted into an external database. This criterion emerged from observed issues in which the BIM consultants related to failure to establish a hierarchical relationship between virtual assets semantically. As an example, “card readers do not contain information on what door it is associated with” (Compliance report, Project A). Although the strategy mentioned in the BEP was that the ‘Mark’ parameter would be used to link the card readers to their respective doors, no parameters connecting to the door were found in the card reader digital assets (Figure 10).
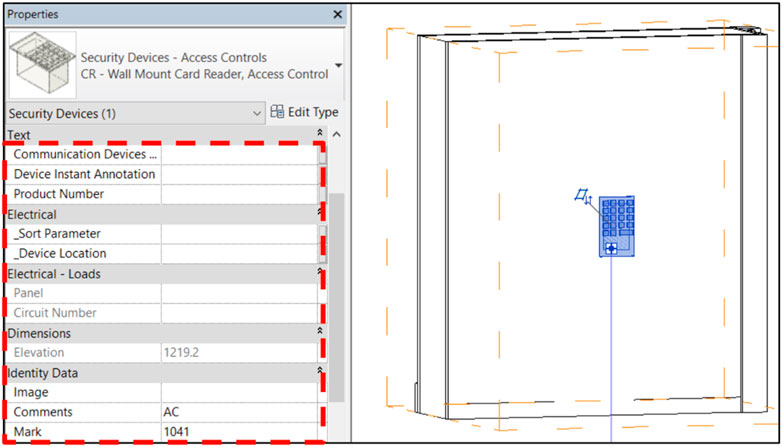
FIGURE 10. Example of issue related to hierarchical asset structure - Electrical model with door security device not associated with any door.
Although the connection of virtual objects through the creation of systems in Revit can automatically provide some functional relationship between assets, this approach was not sufficiently reliable for the BIM Consultants. Since this sort of parent-child relationship is not relevant for all the assets, and can vary between organizations, it is important that the owners provide a definition of an asset hierarchical structure in the IR, along with a definition of what types of assets need parent-child information, and which parameter will be used to convey this information.
4.5 Association
The fifth criterion identified was related to the encapsulation of information using the virtual objects in the model as information containers. “All the information presented in the model views and schedules has to be generated directly from model data so that every piece of information is consistent and alive in the model” (BIM Requirements, project A). It was critical for the owners that every piece of asset information required was associated with a virtual object. This was to ensure that all the relevant asset information in BIM can be queried and exported along with their respective digital asset into an external database.
The current practices observed often involved the employment of different tools, not necessarily interoperable with BIM, for discipline-specific tasks (e.g., energy analysis, structural calculations, calculations of flow rates in mechanical systems). Large organizations can utilize a variety of software packages so as to combine the strengths of different tools. In these cases, the information coming from various external sources are then included in the construction documents not necessarily making their way into BIM. According to one of the BIM Managers in the design team, the conventional workflow was to have “MEP schedules generally built and maintained in Excel, then linked into Revit and onto sheets using a specific plug-in”. This criterion emerged from the observation of issues in which the models contained relevant asset information not linked to their respective digital assets. As an example, cases were observed in which the sheets in the models were empty while the pdf submission of the same sheets contained schedules with relevant information for FM (Figure 11). These schedules were generated outside of Revit and, thus the information contained in these schedules was not associated with any virtual object.
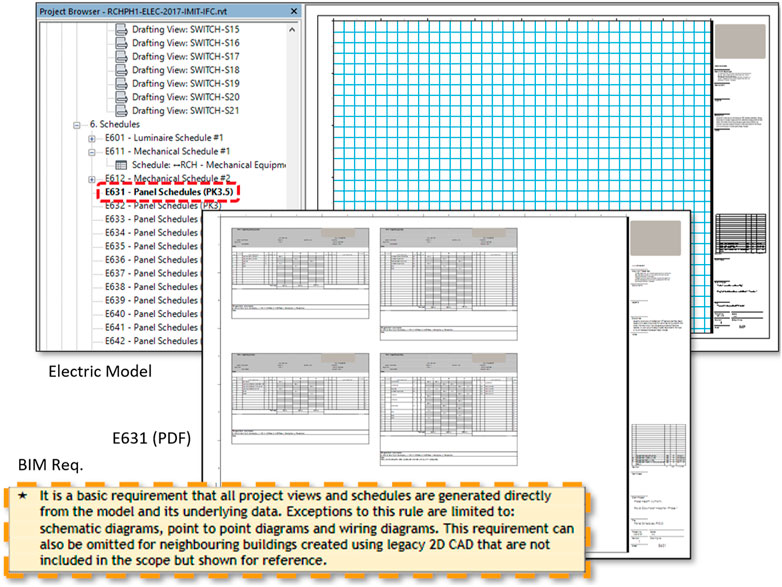
FIGURE 11. Example of Association issue—Schedules brought into Revit Sheets from an external source without connection with Model. (On top, a Revit screenshot with the Sheet E631 empty. In the middle, the pdf submission of the same Sheet E631 with schedules not generated in Revit. At the bottom, an excerpt of the BIM Standard Requirements).
Since not all information generated during design in BIM is relevant for the owners, they need to define what asset information is required for each asset category. This was articulated in the DGS (Figure 12).
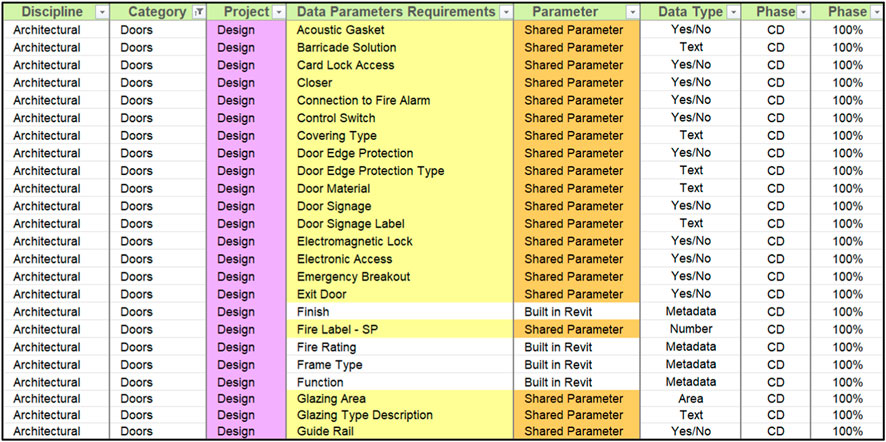
FIGURE 12. Excerpt of DGS spreadsheet with examples of data parameters required for the Revit category ‘Doors’.
4.6 Information Identity
The sixth criterion identified was related to the address of each piece of asset information within the information containers. It was critical for the owners that all the asset information required was conveyed using predefined parameters. This was to ensure that all the asset information could be mapped and automatically exported from BIM into the external database. The observation of the current practice revealed that different design teams often use different parameters to convey the information needed. Also, the name of the parameters does not necessarily follow a specific naming convention when the models are not deliverables for FM. This criterion emerged from observed issues related to non-standardized use of parameters to associate information with virtual assets. As an example, It was part of the requirements that the design team should create the floor finish as a virtual object, whereas the usual design practice was to represent the floor finish information as a parameter associated with room objects (Figure 13). In such a case, the information was present in the model. However, it would not be easily extracted since the parameter containing information about an asset was associated with a different asset (the room in this case). In other words, the location of the information was unclear.
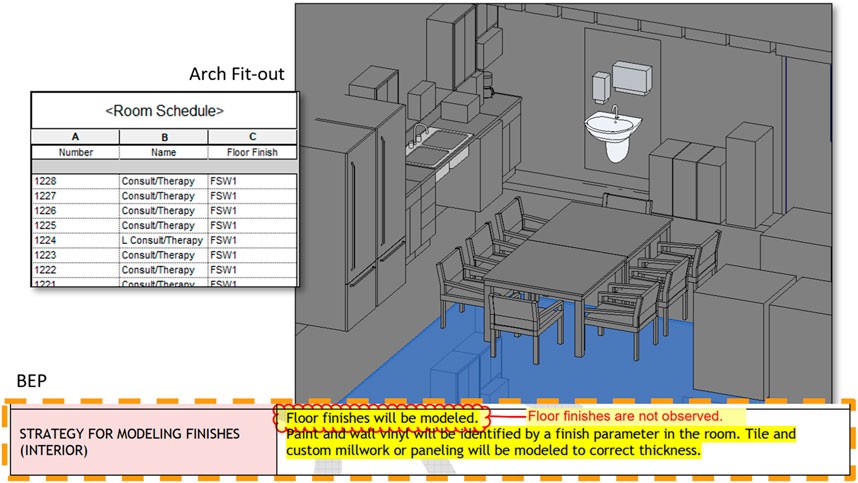
FIGURE 13. Example of issue related to Information Identity—Floor finishes not represented as a virtual object in the architectural model (top right), and floor finish information provided through a parameter associated with the room (top left) not complying with the strategy proposed on the BEP (bottom).
For the owners, any kind of asset information required from a specific type of asset needed to be provided under a specific parameter following a specific naming criteria. This was provided as part of the DGS (Figure 12) in projects A, B, and C. As an example: if the circuit number is a piece of asset information required for all electrical equipment, they all should have a parameter named exactly ‘circuit number’, not ‘circuit #’ or ‘circuit no’. It should be noted that, although COBie was not required in these case studies, the implementation of COBie can be useful to facilitate the mapping between asset information in the BIM database and external databases.
4.7 Coordinated federation
The seventh criterion identified was related to how the digital assets and their information are distributed across different discipline models and worksets. It was critical for the owners that the division of digital assets and their information among separate models and worksets were coordinated between disciplines. This was to ensure that duplications and miscalculations are avoided when asset data extracted from different places are brought together into an external database. Since collaborative design is one of the goals of a BIM process, teams from different disciplines can work simultaneously in their own separate but linked models. However, it is important that the different kinds of assets are assigned to a specific model to facilitate data extraction and avoid duplications or incomplete data. This is also very important for other BIM uses during design, such as virtual coordination. This criterion emerged from issues related to non-standardized division of model content creation among different disciplines and teams. Example: According to the BEP in project A, structural walls and concrete rebar were supposed to be modeled only in the structural model. As the model audits revealed, rebar was found in the architectural model, creating confusion and potential duplication (Figure 14).
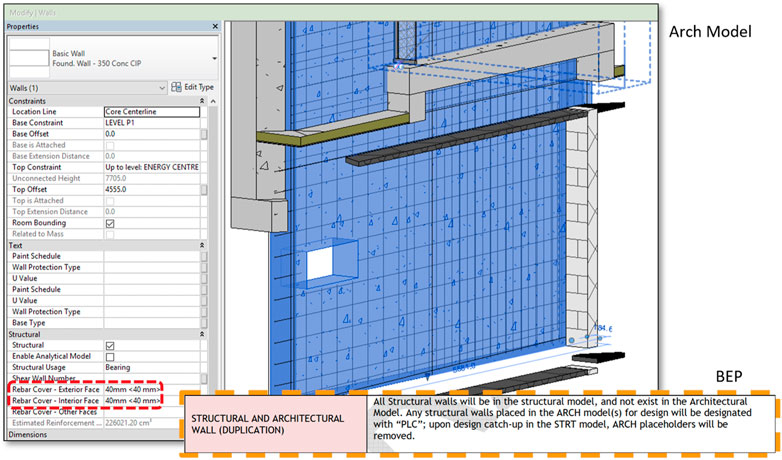
FIGURE 14. Example of issue related to Coordinated Collaboration —Rebar modeled in the architectural model (on top) instead of structural, not complying with the strategy proposed on the BEP (at the bottom).
To ensure that this criterion is met, first the division of digital assets across models and worksets had to be defined and documented as shown in Figure 15.
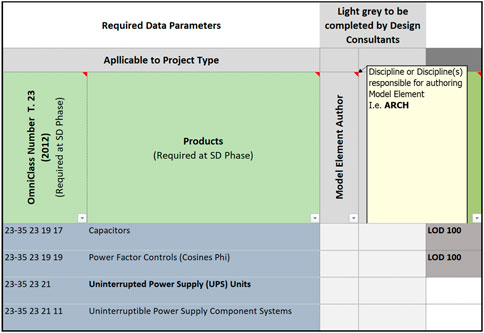
FIGURE 15. Excerpt of Project B’s DGS spreadsheet containing a column for the specification of the discipline model in which the asset will be modeled.
4.8 Clarity
The eighth criterion identified was related to the removal of noise in the asset data within the models. Example: “CD (Construction Documents) and IFC (Issued for Construction) submission models should not contain abandoned designs, testing models, empty worksets, personal views, “Design Options” or unnecessary AutoCAD files. All families not part of the project should be purged.” (BIM Requirements, Project C). It was critical for the owners that the virtual objects that are not representing a required asset were removed or clearly indicated as not for FM. The same applied for asset information parameters. This was to ensure that all the assets and all asset information extracted from the models accurately represents the physical asset, avoiding duplications, miscalculations, and meaningless asset data. It was part of the current design practice to create different design options for the same area, for example. This practice can lead to duplicate assets or errors in asset counts if the digital assets of the secondary design choice are not cleaned out, or clearly indicated as non-existing assets with a predefined parameter. Invisible elements, such as rooms, can also be created and remain invisible in the drawings affecting the asset IQ by inducing errors in the number of rooms or location of assets. This criterion emerged from issues like that, in which duplicated, or unused digital assets and fields of information were found in the models. Figure 16 shows an example of issue related to clarity, in which “some extraneous objects are present away from the building. Presence pollutes database and conflicts with producing an accurate Record Model” (BIM compliance report, Project A).
In order to inform modeling practice and ensure that this criterion was met, the definition of what type of assets are going to be tracked, and their naming convention was needed. These definitions (included as part of the DGS spreadsheet) allowed the design team to ensure the clean-up for these specific asset types.
4.9 Phasing
The ninth criterion identified was related to how the different phases of the project execution are represented in the models. Depending on the project, it becomes crucial to define temporal information for different objects in design models. By temporal information, we mean the point in time of project execution that an object is actually installed or executed onsite. Having temporal information associated with specific objects can inform 4D simulation and is especially important in renovation projects or new construction projects broken down into multiple phases (or scopes). In renovation projects, for example, there must be a way to distinct virtual objects representing existing parts of the building from virtual objects that are part of the new construction. In case of large projects in which the execution has to be broken down in multiple phases, there must be a way to identify in which phase each asset is installed. It was critical for the owners in projects A,B and C that this phase information was indicated consistently in the models. This was to ensure that all the assets and all asset information extracted from the models accurately represents the intended phase of the physical asset, avoiding duplications, miscalculations, and meaningless asset data. Even though there is a built-in parameter in Revit to define the phase in which each object is created or demolished, this parameter was observed to be used differently even between users in the same team. It was observed that some teams also used shared parameters in Revit instead of the built-in parameter. This criterion emerged from this inconsistent use of parameters to convey phasing information. Figure 17 shows an example of an issue related to phasing: “Use of comments for phasing is not documented in the BEP and does not appear consistent across models” (BIM compliance report, Project A).
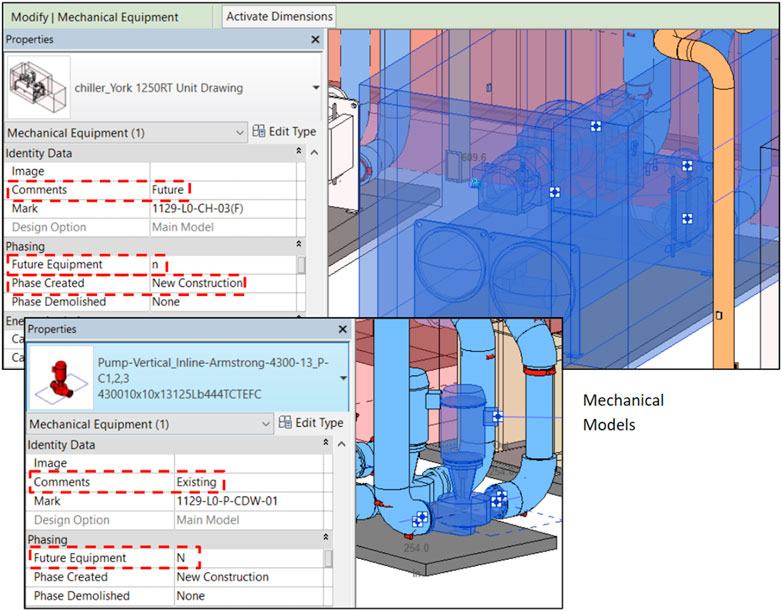
FIGURE 17. Example of issue related Phasing—Inconsistent use of parameters to define asset installation phase.
In order to inform modeling practice and ensure that this criterion was met, clear definition of how phasing information is indicated was required. Example: “Phasing Strategy proposed in the PEP must be followed, and consistency is expected, i.e., no Comments parameter reading ‘existing’ and Revit phase set to ‘New Construction’” (BIM Requirements, Project C).
4.10 Spatial Orientation
The last criterion identified was related to the spatial orientation of the virtual objects. It was critical for the owners that the geometry and location of the digital assets met a predefined minimum criteria. This was to ensure that the information about the location of the assets was accurate enough. Although the geometric information of the assets is not necessarily relevant for FM, there are critical asset information parameters that are calculated based on the defined geometry of the virtual objects (e.g., areas, volume and location). It was observed that the level of geometric information in current practice is often dictated by the use of the models for rendering, and clash detection, which do not prioritize how this information is reflected in parameters. This criterion emerged from issues in which virtual objects were represented with insufficient level of geometric accuracy compromising required asset information. If the geometry of a room is not properly defined, for example, the assets inside might not be properly associated with this room, compromising the parametric information about the location of these assets (Figure 18).
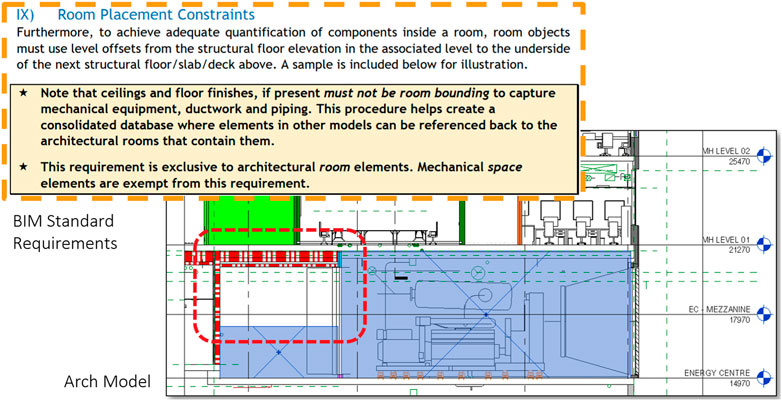
FIGURE 18. Example of issue related to Spatial Orientation - Excerpt from BIM requirements related to room height (on top), and section view of a room created with inadequate height (at the bottom).
In order to inform modeling practice and ensure that this criterion was met, the owners provided a definition of spatial information level of accuracy for each type of asset. In projects A, and B, the criteria adopted to define the minimum levels of geometric information was the AIA BIM Forum LOD (BIM Forum, 2016). For each type of asset, and for each phase of design (i.e., schematic design, design development, construction document development, and issue for construction) an expected LOD was defined (Figure 19).
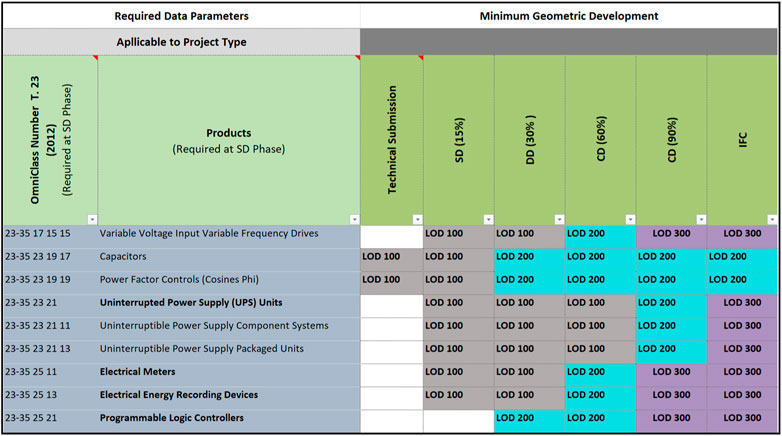
FIGURE 19. Screenshot of Data and Geometry Specification spreadsheet from Project B containing a LOD matrix.
To summarize, the compliance issues analyzed depict a process of adaptation to new modeling practices that are not necessarily required on conventional BIM projects without requirements for FM. BIM authoring tools, such as Revit and ArchiCAD, allow the user to take many different paths to create digital representations of the facility and its construction elements. This provides a degree of freedom to the design team, which can be extremely useful to achieve more complex geometries and to circumvent some software constraints. On the other hand, the reliability of the parametric information extracted from BIMs depends on a certain level of standardization of the modeling practices employed during design. While modeling requirements are fundamental to avoid interoperability issues between different systems and ensure that they can reliably extract data from the models, they also represent constraints for how design teams use BIM as a tool for their main activities (i.e., production of drawings). Thus, it is important to consider how these requirements can disrupt current workflows that are often developed over multiple years and projects inside a design firm, which is discussed in the next section.
5 Sources of IQ issues in BIM for FM within Design practice
Although it has been highlighted that the modeling process has the most impact on assuring the IQ in BIM (Zadeh et al., 2017), and that BIM for FM is modeled differently from BIMs conventionally created during design or construction (Cavka et al., 2018), it remains unclear how and why conventional use of BIM during design often fails to meet the proposed IQ criteria in BIM for FM. Five examples of modeling practices that can compromise the asset IQ were identified and mentioned as part of examples of issues provided in section 4.
• Representation of assets using annotation instead of virtual objects (Figure 5)
• Representation of hierarchical relationship using schematic diagrams disconnected from the virtual objects
• Employment different software and tools to generate asset information disconnected from the models (Figure 11)
• Creation of repeated assets in different discipline models including the use of placeholders (Figure 14)
• Creation of different design options in the same model
• Creation of virtual objects with simplified geometry (Figure 18)
These examples of modeling practices help to explain how design models are not inherently reliable sources of asset information for FM. However, considering that the design teams investigated are proficient in the use of BIM, and have been using BIM in several other projects, why are these practices still common? In the previous section, it was observed that the issues exemplified would not necessarily be characterized as issues in a conventional project, highlighting a misalignment between idealized BIM workflows for FM and conventional design workflows.
In this section, this misalignment is further investigated, and five sources of IQ issues are identified that go beyond modeling practices and speak more to design practices in general. The following five sources of IQ issues will be described in detail in the following subsections.
• The schematic nature of design intent
• Failure to adequately capture information about deviations from design that happen during construction
• Deployment of imported families with generic information
• 2D Drawings as the official means to communicate design intent (3D x 2D)
• Disconnect between asset information in BIM and in the conventional design deliverables (Drawings and Specifications)
5.1 The schematic nature of design intent
Design intent is about how the building and its integrated systems, as a final product, will meet the owners’ needs. However, designers do not always have execution and installation know-how and often rely on general contractors and trades to bridge that knowledge gap during execution. The general contractors, in turn, have more flexibility to choose their best means and methods for the execution. Thus, the design intent is often intentionally represented with incomplete or inaccurate pieces of information, as demonstrated in the following excerpts from the construction documents in Project A:
“The drawings indicate the general location and route to be followed by the piping and ductwork. Where details are not shown on the drawings or only shown diagrammatically, the pipes and ductwork shall be installed in such a way as to conserve head room and interfere as little as possible with the free use of space through which they pass.” (Project A’s Specifications document Division 23)
“Drawings are generally diagrammatic and are intended to indicate the scope and general arrangement of work and are not detailed installation drawings. Do not scale the drawings.” (Project A’s Specifications document Division 23)
“All work shall be in accordance with the PROJECT Drawings and Specifications and their intent, complete with all necessary components, including those (components) not normally shown or specified, but required for a complete installation.” (Project A’s Specifications document Division 23)
Therefore, the schematic nature of design intent can compromise certain aspects of asset IQ expected by the owners, such as accurate spatial information of certain assets as shown in the mechanical equipment represented in the wrong location.
5.2 Failure to adequately capture information about deviations from design that happen during construction
The lack of reliable as-built documents is a well-documented issue in the industry. This retroactive process of incorporating changes in the design (as-built development) is not exclusive to a BIM process; redlines and as-builts are expected in projects regardless of BIM. However, the production of an as-built model is even more laborious and can require more information than as-built drawings. As studies have shown, the creation of an as-built BIM is still a time-consuming, subjective, and error-prone process (Tang et al., 2010).
The design teams in projects A, B, and C were not used to providing an as-built model. Instead, the conventional practice was to incorporate the changes in the drawings only. Challenges related to the production of the as-built model mentioned by the BIM Manager in project A were the insufficient level of detail in the information provided in the redlines, the tardiness in receiving the redlines, and the lack of resources allocated for incorporating the redlines in the models. Figure 20 shows a comparison between site photos and the last design models provided to the owners in project A, which contain inconsistencies not captured, or incorporated, in the models that affect asset IQ. Such inconsistencies were expected to be adjusted during construction, with additional information from fabrication models (models created by GC and trades) or through redlines and updates in the design models (as-built model). However, additional costs and other priorities in the project delivery prevented the complete capture of later changes in the models.
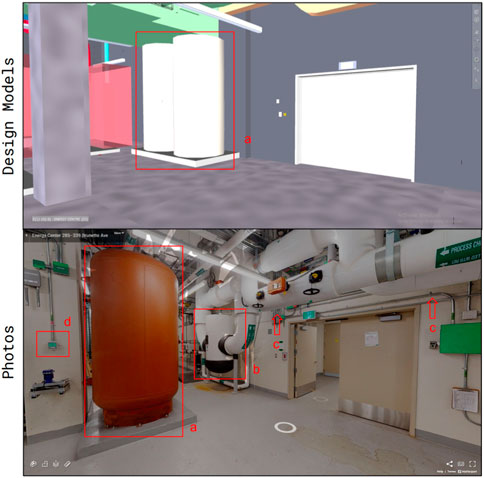
FIGURE 20. Comparison between photos taken on-site and the federated design models in Navisworks (A). Expansion tanks position inconsistent / (B). Air separator not in the model / (C) Pipes and valves not in the models / (D). Thermostat not in the models).
5.3 Deployment of imported families with generic information
A common modeling practice identified was the employment of virtual objects (Revit families) downloaded from external sources (e.g., manufacturer’s website) or imported from other projects without thorough validation of the semantic information brought along with these families. Table 6 shows 74 fields of parametric asset information found in a single virtual object (fan-coil unit) downloaded from a manufacturer website.

TABLE 6. Example of parametric information found for a single asset in the design model of project A.
The issue associated with this practice is that these generic Revit families are often placed in the model containing information that is not necessarily accurate. An assessment of the mechanical, electrical, and specialty equipment objects in Project A revealed an average of 34 fields of asset information per object (Figure 21). From this amount of parametric data found in the design models, only 23% was required by the owners in the DGS. The other 77% of parametric data was generated entirely as a by-product of the conventional design process in BIM. These 77% data parameters had a variety of information that was not necessarily useful or reliable for FM, of which many were brought along with imported families. In these cases, no quality control is performed with the data. As the BIM manager explained during one of the interviews, in a conventional project, it is not the typical workflow to perform quality control over the BIM data that is not shown in the drawings or specifications. Therefore, a significant amount of parametric information in the models is not validated, compromising the reliability of the model as a source of asset information.
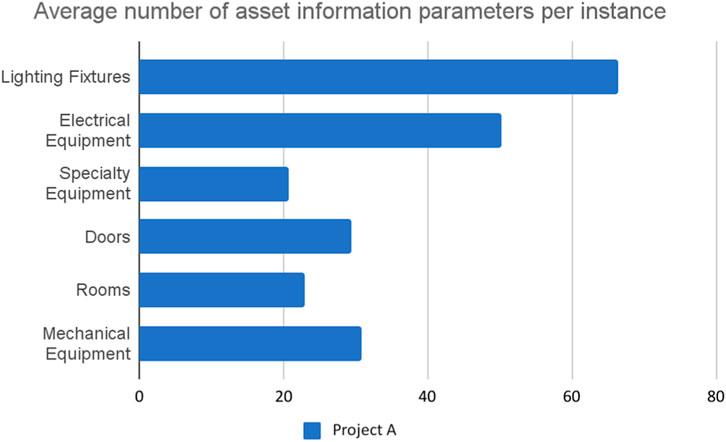
FIGURE 21. Quantitative assessment of information parameters per instance in assets under certain categories in project A.
5.4 2D Drawings as the official means to communicate design intent (3D x 2D)
Project documentation still relies heavily on the production of 2D Drawings even when BIM deliverables are required. 2D drawings are still the most common and reliable way used to convey information. Given the amount of geometric, semantic, and topological information generated throughout the design process in BIM, a complete and thorough quality control over all this information could be counterproductive and expensive. As the Owner’s Senior Design Lead in Project A highlighted, the internal use of BIM in Organization A’a activities was limited to a support role, which he attributed to the lack of contractual power of BIM as a deliverable. “We cannot always trust the information presented in the models. Since the official and contractually required information is the one in the drawings, the information in the models is not fully reliable.”—(Senior Lead, Design Redevelopment & Major Projects). Figure 22 shows a disclaimer provided by the design team in the Revit models of project B, which illustrates the design team’s avoidance of responsibility over the information provided in the design models.
The fact that 2D drawings are still the official way to communicate information for construction has some additional incompatibilities with the ideal BIM process. One of the challenges faced by the design team in project A, especially in mechanical disciplines, was to create a high-fidelity 3D model that could clearly be represented in the drawings. For example, hydraulic pipes vertically superposed might be confusing in a plan view, where only the pipe on top would be visible. In this case, the representation of the pipes side by side would improve design intent clarity (Figure 23).
Following this practice, the drawing does not necessarily represent the exact position of the installed pipes. Still, it provides a better understanding of the system distribution, without the necessity of excessive sections, which is helpful for the installation. Furthermore, considering that 2D drawings can only partially convey the total amount of information created in a model, a BIM workflow with drawings as the main source of information contradicts the need for precise modeling practices, in which higher levels of detail in the model are not perceptible.
5.5 Disconnect between asset information in BIM and the asset information in the conventional design deliverables (Drawings and Specifications)
One of the premises of BIM is its capacity to serve as a central source of information for decision-making. In projects A and B, for example, the owners had “One Version of the Truth” as one of the main BIM goals (Project A’s BEP). To achieve that, it was expected that all the information produced as part of the design process would be created in BIM and associated with virtual objects in the design models. However, the main design deliverables are drawings and specifications (Demkin, 2001) and the information provided in these deliverables is not necessarily consistent one with another or with the information in BIM.
While most drawings are created in BIM, there are some drawings, such as schematic views (Figure 24) and details that are often created in CAD or using 2D tools. In that case, the information, such as parent-child connections with other assets, represented graphically, is not necessarily provided in parameters associated with virtual objects.
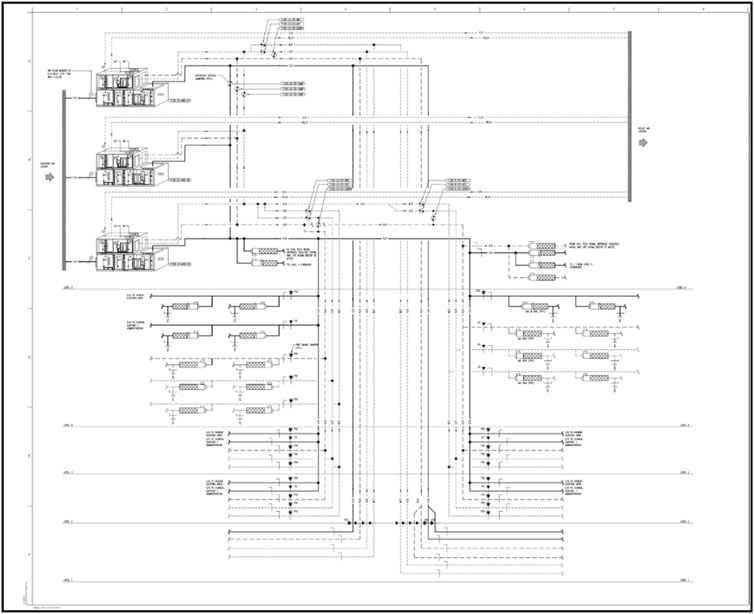
FIGURE 24. Example of a schematic view of an air system in project A with asset information not associated with virtual objects.
Another large set of asset information that is often not associated with any virtual object in BIM is the information provided in the specification document. The specification document comprises written representations of the quality of materials and associated workmanship (Utiome and Drogemuller, 2013). Although the specifications present the asset information structured differently from the models (i.e., not assigned to individual assets), it contains a significant amount of information, including information that can be useful to FM.
Example of asset information in Specifications:
“.5 Access Doors—Non-Patient Areas:
1. constructed of steel, prime coated, except:
2. constructed of stainless steel in areas finished with tile or marble surfaces
3. constructed of stainless steel with neoprene gasketed door in damp and high
Humidity areas
4. flush mounted with 180o opening door, round safety corners, concealed hinges, plaster lock and anchor straps
5. 600 mm × 600 mm (24 in x 24 in) for personnel entry,
6. 300 mm × 450 mm (12 in x 18 in) for hand entry, and
7. generally fitted with screwdriver operated latches, except:
8. in areas subject to security risks (Public Corridors, Psychiatric Patient Areas, Public Washrooms), provide keyed cylinder locks with similar keys.
9. Plaster or wet wall construction: flush with wall or ceiling type with concealed flange.
10. Tile, ceramic tile, marble, terrazzo, plaster or wet wall construction in washrooms and other special areas: flush with wall or ceiling type with concealed flange.
11. Feature wall construction: Recessed wall type that is selected to complement and conform with the architectural module, treatment, or panelling. The size shall conform to adjacent finishes.
12. Access panels in fire separations and fire walls shall have a compatible fire rating and ULC label.
13. Access doors are not required in removable ceilings. Provide coloured marking devices after completion of ceilings, at four corners of each panel below point requiring access. Colour code markers to show service or device above.
14. At time of instruction of owners operating staff, hand-over and obtain signed receipt for 4 sets of each type of key used to lock access doors.” (Project A’s Specifications Document Division 23)
As observed in projects A, B, and C, the information in the specifications document is generated in parallel, without any association with the virtual objects in BIM. Furthermore, it is not guaranteed that the information in the specifications is also in the drawings, or even consistent with the information in the drawings as shown in the following excerpts:
“Drawings and specifications are complementary in nature and combined, create a complete set of construction documents. Any item called for by one and not by the other shall be interpreted as being called for by both.” (Project A’s Specifications Document Division 23)
“Should inconsistencies exist such as the drawings disagreeing within themselves or with the specifications, the better quality and/or greater quantity of work or materials shall be estimated upon, performed and furnished unless otherwise ordered by the Consultant in writing during the bidding period.” (Project A’s Specifications Document Division 23)
Therefore, there is no guarantee that the information provided in the specifications is in BIM or consistent with the information in BIM.
6 Discussion and conclusion
Based on the analysis of the data from the compliance process in three projects with well defined information requirements including modeling standards, 10 key IQ criteria were identified in BIM for FM from the owner’s perspective. According to these key criteria, in order to enable design models to reliably support FM, the asset information in these models needs to be structured in such a way that ensures the following.
1. All predefined building assets are digitally represented in the models by a virtual object
2. Digital assets are trackable by a unique ID using a predefined attribute
3. Digital assets follow a specific classification and naming convention predefined by the owner
4. Parent-child relationships between digital assets are defined in the models following a hierarchical structure predefined by the owner
5. All asset information developed during the design is associated with a digital asset within the models
6. Required asset information is conveyed using predefined attributes
7. Division of digital assets and their information among separate models and worksets is coordinated between disciplines
8. Virtual objects that are not representing a required building asset are removed or clearly and uniformly indicated
9. In multiphase projects, the phase information is indicated uniformly
10. Spatial information of the digital assets meets the predefined minimum criteria
These identified asset IQ criteria are specific to projects with BIM Requirements for FM and can be counter-productive in projects without FM requirements, which will depend on each design team’s conventional uses of BIM. Furthermore, the concept of IQ in BIM for FM is directly dependent on predefined information requirements. In other words, it is not possible for a design team to ensure IQ for FM without the owner’s predefined information requirements.
Regarding the source of compliance issues, some reasons why conventional design practices do not necessarily meet these criteria were identified. Some of the observed sources of IQ issues are related to how BIM is used.
• Representation of assets using annotation instead of virtual objects
• Deployment of imported families with generic information without validation
• Representation of hierarchical relationship using schematic diagrams disconnected from the virtual objects
• Employment different tools to generate asset information outside of the models
• Creation of repeated assets in different discipline models
• Creating different design options in the same model
• Creating virtual objects with simplified geometry
Some of the observed sources of the IQ issues identified go beyond the use of BIM during design. These other sources are related to the incompatibilities between the proposed modeling practices for the delivery of asset information and general aspects of current design practice.
• The schematic nature of design intent compromises the precision of asset information
• The failure to adequately capture information about deviations from design that happen during construction.
• Designers do not take responsibility for all the information provided in the models and 2D drawings as the official means to communicate design intent (3D x 2D).
• Disconnect between asset information in BIM and the asset information in the conventional design deliverables (Drawings and Specifications)
Although many studies have indicated that issues in design models prevent adequate delivery of asset information (Parsanezhad and Dimyadi, 2013; Lavy and Jawadekar, 2014; Korpela et al., 2015; Pärn et al., 2017; Pishdad-Bozorgi et al., 2018; Heaton et al., 2019; Patacas et al., 2020), the existence of these issues has not been properly addressed or studied in depth in the literature. The findings in this study indicate that these issues are not trivial design errors, as often assumed, but a consequence of bespoke design practices to use BIM for specific purposes (i.e., articulate the development of design intent and production of drawings). Meeting the BIM IQ criteria for FM implies increases in the scope of design activities. Considering that projects with BIM requirements for FM are still a minority for most design firms, as owners move forward to require BIM for the delivery of asset information, proper considerations about incentives should be made for this additional scope. Another relevant aspect of project delivery for IQ in BIM for FM is the delivery method employed. Although exploring the effect of different delivery methods was out of the scope of this research, it is important to mention that Integrated Project Delivery can provide a more collaborative environment for the implementation of innovation (Jones, 2014), in which early input from different stakeholders can help manage potential IQ issues.
Ultimately, the prevailing idea of a BIM for FM based on the asset information generated in BIM as a by-product of the design process needs revision. The proposition of BIM for FM has been developed upon the idea that digital workflows can prevent the loss of information that happens between the different project phases (Figure 25). However, this study shows that the IQ losses between the design and the end of construction phase cannot be fully prevented by the current digital workflows. In practice, conventional design processes still have systemic limitations for using BIM to deliver high-quality building asset information and that need to be further considered.
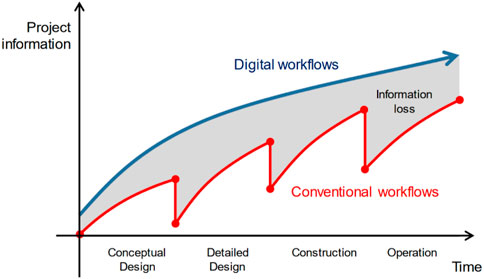
FIGURE 25. Loss of information caused by disruptions in the digital information flow [(Borrmann et al., 2018) based on Eastman et al. (2011)].
Regarding the asset information created in BIM, it is often assumed that “compared to two-dimensional drawings and specifications, BIM Models contain a tremendous amount of electronic information that can be transmitted quickly, efficiently, and can be easily extracted and reused in whole or in part. In particular, the final BIM Model may have a significant value for owners. It can be used to enhance facilities management in the entire project lifecycle.” (Porwal and Hewage, 2013). In this study, some of these underlying assumptions underpinning the proposed process of asset information delivery are challenged.
• All the information in the models is accurate/useful - Not all of the parametric information generated as a by-product of the design process is ready to be used as reliable asset information. A significant amount of information generated in the models during design is not validated. To demand validation of all the information generated during design could be counterproductive for design teams.
• All the information in the construction documents can be generated in BIM and consistent with it - Although it was an expectation for projects A, B, and C that all the information delivered would be available digitally in the models, the information in the specifications document and some schematic drawings and details were not originated from, or associated, with BIM. That would require changes to existing design workflows that none of the design teams were willing to embrace.
It is worth mentioning that different approaches have emerged to circumvent the challenge of validating every single piece of asset information generated in the models as by-product of the design process. The use of MVDs (Model View Definitions) for example, allow the data exchange of a subset of the overall IFC schema for a specific use or workflow, narrowing the scope depending on the need of the receiver (COBie in the case of FM). The concept of Information Delivery Specification (IDS) has emerged over the past few years, piloted by buildingSMART International, to define the requirements supporting an information exchange in both a human and a machine-readable format. An IDS extends the concept of MVD by providing a more customizable way to specify data that is required to support a specific model use to attain a purpose. The IDS allows users to specify properties, materials, types, etc. related to specific use cases, and then automating the checking of models against these specifications. It is believed that providing a robust set of information requirements, articulated in a clear and repeatable manner through and IDS, will greatly improve the information exchange process. There is ongoing work within buildingSMART to further develop this.
A few authors, such as Dossick et al. (2019) and Bonanomi (2019), have pointed out a lack of balance between three areas of the multi-pronged effort required for the delivery of structured asset Information (adoption of new technologies, organizational reconfigurations, and establishment of efficient processes). A proclivity towards the adoption of technologies seems to foster the appearance of standards and solutions that underestimates the underlying reconfiguration required of organizational structures, internal roles, as well as regulative institutions (Zomer et al., 2022). Digital technologies, such as BIM, are disruptively changing the way professionals get their work done, how they relate to each other, and the way they set their working context (Bonanomi, 2019). For designers, the proposed BIM-based handover process involves disruptions in current practices that are not well understood given the novelty of the process. The lack of understanding about the process and its implications in current practices prevents adequate preparation and management of the process. Therefore, identifying and discussing the process changes brought by BIM is seen as a fundamental gap and promising future research direction (Gao and Pishdad-Bozorgi, 2019).
While Monteiro and Martins (2013) have demonstrated how modeling practices can influence the capacity of using BIM for specific purposes, such as cost estimating, very few authors have explored the relationship between modeling practices during design and IQ in BIM for FM. One of the few studies addressing this gap was Zadeh et al. (2017) study on IQ assessment for FM. Their study, however, was limited by the lack of real owner-defined information requirements and a well-defined asset information delivery process. According to Wang and Strong (1996), quality can only be measured once the data consumer necessities are defined. Therefore, the quality of the information in BIM for FM can only be properly assessed with clear information requirements defined by the owners based on their internal processes. Compared to the findings in Zadeh et al. (2017), this study used three projects with real owner-defined information requirements to expand on the definition of IQ in BIM for FM considering its usefulness not only to the end-users in FM, but as a basis for the process of gathering and delivering the structured asset information. As a result, key quality criteria for the asset information in design BIMs and the main barriers for compliance were identified and underpinned in a way that can inform the use of BIM for FM increasing the value of the information in design BIMs for operations with minimized impact on the design consultants’ current practices.
With regards to limitations, this research studied IQ in BIM for FM based on three projects with very similar proposed processes for asset information delivery process. More case studies similar to this one are necessary to capture possible variations in different contexts (e.g., use of COBie, use of different BIM authoring tools, different project delivery methods). For future research, the development and comparison of what IQ in BIM means to different BIM uses (e.g., cost estimation, permit approvals, clash detection, asset information delivery, energy analysis), and assessment of how the modeling practices required in each case converge or diverge could help inform BIM users on how to approach BIM implementation on different projects.
Data availability statement
The datasets presented in this article are not readily available because privacy of the companies involved in the case studies. Requests to access the datasets should be directed to gtsay@mail.ubc.ca.
Ethics statement
The studies involving human participants were reviewed and approved by UBC Office of Research Ethics. The patients/participants provided their written informed consent to participate in this study.
Author contributions
All authors listed have made a substantial, direct, and intellectual contribution to the work and approved it for publication. All authors contributed to the article and approved the submitted version.
Funding
This research was funded by Natural Sciences and Engineering Research Council of Canada (NSERC), and Fraser Health Authority, grant numbers F18-05090 and F19-04525.
Acknowledgments
This article was developed as a part of the UBC BIM Topics Lab in collaboration with industry partners. The authors would like to thank the owners in projects A, B, and C, the design team in project A, and all other participants involved in this research for sharing their knowledge to their best and for fostering genuine interest in scientific research.
Conflict of interest
The authors declare that the research was conducted in the absence of any commercial or financial relationships that could be construed as a potential conflict of interest.
Publisher’s note
All claims expressed in this article are solely those of the authors and do not necessarily represent those of their affiliated organizations, or those of the publisher, the editors and the reviewers. Any product that may be evaluated in this article, or claim that may be made by its manufacturer, is not guaranteed or endorsed by the publisher.
References
Abdirad, H., Dossick, C. S., Johnson, B. R., and Migliaccio, G. (2021). Disruptive information exchange requirements in construction projects: Perception and response patterns. Build. Res. Inf. 49 (2), 161–178. doi:10.1080/09613218.2020.1750939
Abowitz, D., and Toole, T. (2010). Mixed method research: Fundamental issues of design, validity, and reliability in construction research. J. Constr. Eng. Management-asce - J CONSTR ENG MANAGE-ASCE 136, 108–116. doi:10.1061/(asce)co.1943-7862.0000026
Afsari, K., Eastman, C., and Shelden, D. (2017). Building information modeling data interoperability for cloud-based collaboration: Limitations and opportunities. Int. J. Archit. Comput. 15 (3), 187–202. doi:10.1177/1478077117731174
Anderson, A., Marsters, A., Dossick, C. S., and Neff, G. (2012). Construction to operations exchange: Challenges of implementing COBie and BIM in a large owner organization. Reston, VA: American Society of Civil Engineers (ASCE, 688–697.
Australasian BIM Advisory Board (ABAB). Asset information requirements guide: Information required for the operation and maintenance of an asset [Internet]. 2018. Available from: https://www.abab.net.au/wp-content/uploads/2018/12/ABAB_AIR_Guide_FINAL_07-12-2018.pdf.
Bakis, N., Kagioglou, M., and Aouad, G. (2006). Evaluating the business benefits of information systems.
Ballou, D. P., and Pazer, H. L. (1985). Modeling data and process quality in multi-input, multi-output information systems. Manag. Sci. 31 (2), 150–162. doi:10.1287/mnsc.31.2.150
Beatty, R., Eastman, C., Kim, K., and Fang, Y. (2013). Case study 2: Texas A&M health science center-a case study of BIM and COBie for facility management. BIM Facil. Manag., 164–184.
Becerik, G. B., Farrokh, J., Nan, L., and Gulben, C. (2012). Application areas and data requirements for BIM-enabled facilities management. J. Constr. Eng. Manag. 138 (3), 431–442. doi:10.1061/(asce)co.1943-7862.0000433
Berard, O. B. (2012). Building information modeling for managing design and construction: Assessing design information quality. Technical University of Denmark.
Bodein, Y., Rose, B., and Caillaud, E. (2014). Explicit reference modeling methodology in parametric CAD system. Comput. Industry 65 (1), 136–147. doi:10.1016/j.compind.2013.08.004
Bonanomi, M. M. (2019). Digital transformation of multidisciplinary design firms: A systematic analysis-based methodology for organizational change management. Springer.
Borrmann, A., König, M., Koch, C., and Beetz, J. (2018). “Building information modeling: Why? What? How?,” in Building information modeling (Springer), 1–24.
Breckenridge, J., and Jones, D. (2009). Demystifying theoretical sampling in grounded theory research. Grounded Theory Rev. 8 (2).
BuildLACCD.LACCD (2019). Building information modeling standards version 4.3 [internet]. [cited 2022 Dec 5]. Available from: http://az776130.vo.msecnd.net/media/docs/default-source/contractors-and-bidders-library/standards-guidelines/bim/laccd_bims-4-3_dbb.pdf?sfvrsn=0.
Camba, J. D., Contero, M., and Company, P. (2016). Parametric CAD modeling: An analysis of strategies for design reusability. Computer-Aided Des. 74, 18–31. doi:10.1016/j.cad.2016.01.003
Cavka, H. B., Staub-French, S., and Poirier, E. A. (2018). Levels of BIM compliance for model handover. J. Inf. Technol. Constr. (ITcon). 23 (12), 243–258.
Choi, J., Lee, S., and Kim, I. (2020). Development of quality control requirements for improving the quality of architectural design based on BIM. Appl. Sci. 10 (20), 7074. doi:10.3390/app10207074
Cichy, C., and Rass, S. (2019). An overview of data quality frameworks. IEEE Access 7, 24634–24648. doi:10.1109/access.2019.2899751
DeLone, W. H., and McLean, E. R. (1992). Information systems success: The quest for the dependent variable. Inf. Syst. Res. 3 (1), 60–95. doi:10.1287/isre.3.1.60
Dixit, M. K., Venkatraj, V., Ostadalimakhmalbaf, M., Pariafsai, F., and Lavy, S. (2019). Integration of facility management and building information modeling (BIM): A review of key issues and challenges. Facilities 37 (7/8), 455–483. doi:10.1108/f-03-2018-0043
Donato, V., Lo Turco, M., and Bocconcino, M. M. (2018). BIM-QA/QC in the architectural design process. Archit. Eng. Des. Manag. 14 (3), 239–254. doi:10.1080/17452007.2017.1370995
Dossick, C., Osburn, L., and Neff, G. (2019). Innovation through practice: The messy work of making technology useful for architecture, engineering and construction teams.Eng. Constr. Archit. Manag.
Du, J., Liu, R., and Issa, R. R. (2014). BIM cloud score: Benchmarking BIM performance. J. Constr. Eng. Manag. 140 (11), 04014054. doi:10.1061/(asce)co.1943-7862.0000891
East B, Carrasquillo-Mangual M (2022). The COBie guide. [Internet]. [cited 2022 Sep 2]. Available from: https://portal.nibs.org/files/wl/?id=GvMvniYRQOUN77pPyhtx2QXVqOgNmpie.
Eastman, C. M., Eastman, C., Teicholz, P., Sacks, R., and Liston, K. (2011). BIM handbook: A guide to building information modeling for owners, managers, designers, engineers and contractors. John Wiley & Sons.
Eisenhardt, K. M. (1989). Building theories from case study research. Acad. Manag. Rev. 14 (4), 532–550. doi:10.2307/258557
Facilities Operations and Development (2022). The Ohio state university (OSU). Building information modeling (BIM) project delivery standards version 2022 [internet]. Available from: https://fod.osu.edu/sites/default/files/ohio-state_bim_pds_v2022.pdf.
Fellows, R., and Liu, A. (2022). Research methods for construction. [Internet]. Wiley; [cited 2022 Sep 2]. Available from: https://www.wiley.com/en-us/Research+Methods+for+Construction%2C+5th+Edition-p-9781119814733.
Gao, X., and Pishdad-Bozorgi, P. (2019). BIM-enabled facilities operation and maintenance: A review. Adv. Eng. Inf. 39, 227–247. doi:10.1016/j.aei.2019.01.005
Gholami, E., Kiviniemi, A., and Sharples, S. (2015). Implementing building information modelling (BIM) in energy-efficient domestic retrofit: Quality checking of BIM model.
Glaser, B. G., Strauss, A. L., and Strutzel, E. (1968). The discovery of grounded theory; strategies for qualitative research. Nurs. Res. 17 (4), 364. doi:10.1097/00006199-196807000-00014
Glaser, B. G. (1978). Theoretical sensitivity. University of California. [cited 2022 Sep 2]. Available from: https://eduq.info/xmlui/handle/11515/17665.
Goodhue, D. L. (1995). Understanding user evaluations of information systems. Manag. Sci. 41 (12), 1827–1844. doi:10.1287/mnsc.41.12.1827
Heaton, J., Parlikad, A. K., and Schooling, J. (2019). Design and development of BIM models to support operations and maintenance. Comput. Industry 111, 172–186. doi:10.1016/j.compind.2019.08.001
International Organization for Standardizatio (2013). ISO 9000:2015 Quality management systems — fundamentals and vocabulary. 2015 [cited 2022 Sep 2]. Available from: https://www.iso.org/standard/45481.html.
International Organization for Standardizatio (ISO) (2018). ISO 19650-1:2018 Organization and digitization of information about buildings and civil engineering works, including building information modelling (BIM) — information management using building information modelling — Part 1: Concepts and principles [Internet]. [cited 2022 Sep 2]. Available from: https://www.iso.org/standard/68078.html.
International Organization for Standardizatio (ISO) (2022). ISO 19650-4:2022 Organization and digitization of information about buildings and civil engineering works, including building information modelling (BIM) — information management using building information modelling — Part 4: Information Exchange [Internet]. [cited 2022 Sep 2]. Available from: https://www.iso.org/standard/78246.html.
International Organization for Standardizatio (ISO) (2022). ISO/DIS 7817 Building information modelling - level of information need - concepts and principles [Internet]. 2022 [cited 2022 Mar 4]. Available from: https://www.iso.org/standard/82914.html.
Jones, B. (2014). Integrated project delivery (IPD) for maximizing design and construction considerations regarding sustainability. Procedia Eng. 95, 528–538. doi:10.1016/j.proeng.2014.12.214
Juran, J. M., and De Feo, J. A. (2010). Juran’s quality handbook: The complete guide to performance excellence. McGraw-Hill Education.
Kasprzak, C., Ramesh, A., and Dubler, C. (2013). Developing standards to assess the quality of BIM criteria for facilities management. Build. Solutions Archit. Eng., 680–690.
Kim, S., Poirier, E. A., and Staub-French, S. (2020). Information commissioning: Bridging the gap between digital and physical built assets. J. Facil. Manag. 18 (3), 231–245. doi:10.1108/jfm-04-2020-0024
Kiviniemi, A., and Codinhoto, R. (2014). Challenges in the implementation of BIM for FM—case manchester Town Hall complex. Comput. Civ. Build. Eng.
Korpela, J., Miettinen, R., Salmikivi, T., and Ihalainen, J. (2015). The challenges and potentials of utilizing building information modelling in facility management: The case of the center for properties and facilities of the university of helsinki. Constr. Manag. Econ. 33 (1), 3–17. doi:10.1080/01446193.2015.1016540
Kumar, V., and Teo, A. L. E. (2021). Development of a rule-based system to enhance the data consistency and usability of COBie datasheets. J. Comput. Des. Eng. 8 (1), 343–361. doi:10.1093/jcde/qwaa083
Kumar, V., and Teo, E. A. L. (2020). Perceived benefits and issues associated with COBie datasheet handling in the construction industry.Facilities
Kvale, S. (2007). Planning an interview study: Doing interviews [internet]. 1 oliver’s yard, 55 city road. London England EC1Y 1SP United Kingdom: SAGE Publications, Ltd. [cited 2022 Sep 2]. Available from: https://methods.sagepub.com/book/doing-interviews.
Lavy, S., and Jawadekar, S. (2014). A case study of using BIM and COBie for facility management. Int. J. Facil. Manag. 5 (2).
Lee, G., Jeong, J., Won, J., Cho, C., Youjoon, S., Ham, S., et al. (2014). Query performance of the IFC model server using an object-relational database approach and a traditional relational database approach. J. Comput. Civ. Eng. 28 (2), 210–222. doi:10.1061/(asce)cp.1943-5487.0000256
Lee, Y. C., Eastman, C. M., and Solihin, W. (2018). Logic for ensuring the data exchange integrity of building information models. Automation Constr. 85, 388–401. doi:10.1016/j.autcon.2018.06.002
Lee, Y. C., Eastman, C. M., and Solihin, W. (2021). Rules and validation processes for interoperable BIM data exchange. J. Comput. Des. Eng. 8 (1), 97–114. doi:10.1093/jcde/qwaa064
Lee, Y. W., Strong, D. M., Kahn, B. K., and Wang, R. Y. (2002). Aimq: A methodology for information quality assessment. Inf. Manag. 40 (2), 133–146. doi:10.1016/s0378-7206(02)00043-5
Leygonie, R., Motamedi, A., and Iordanova, I. (2022). Development of quality improvement procedures and tools for facility management BIM. Developments in the Built Environment, 100075.
Lilis, G., Giannakis, G., Katsigarakis, K., and Rovas, D. (2018). “A tool for IFC building energy performance simulation suitability checking,” in eWork and eBusiness in architecture, engineering and construction (CRC Press), 57–64.
Liu, H., Abudayyeh, O., and Liou, W. (2020). BIM-based smart facility management: A review of present research status, challenges, and future needs, 1087–1095.
Liu, H., Cheng, J. C., Gan, V. J., and Zhou, S. (2022). A novel Data-Driven framework based on BIM and knowledge graph for automatic model auditing and Quantity Take-off. Adv. Eng. Inf. 54, 101757. doi:10.1016/j.aei.2022.101757
Liu, Y., van Nederveen, S., and Hertogh, M. (2017). Understanding effects of BIM on collaborative design and construction: An empirical study in China. Int. J. Proj. Manag. 35 (4), 686–698. doi:10.1016/j.ijproman.2016.06.007
Love, P., Holt, G., and Li, H. (2002). Triangulation in construction management research. Research outputs pre 2011 [Internet]. 2002 Jan 1; Available from: https://ro.ecu.edu.au/ecuworks/3875.
Miles, M. B., Huberman, A. M., and Saldana, J. (2014). Qualitative data analysis: A methods sourcebook. Third Edition. SAGE Publications Ltd. SAGE Publications.
Monteiro, A., and Martins, J. P. (2013). A survey on modeling guidelines for quantity takeoff-oriented BIM-based design. Automation Constr. 35, 238–253. doi:10.1016/j.autcon.2013.05.005
Moreno, C., Olbina, S., and Issa, R. R. (2019). BIM use by architecture, engineering, and construction (AEC) industry in educational facility projects. Adv. Civ. Eng. 2019, 1–19. doi:10.1155/2019/1392684
Motamedi, A., Iordanova, I., and Forgues, D. (2018). FM-BIM preparation method and quality assessment measures.
National Building Specification (2020). BS EN 17412-1:2020 Building information modelling - level of information need. Concepts and principles. British Standards Institution. [cited 2022 Mar 4]. Available from: https://www.thenbs.com/PublicationIndex/documents/details?Pub=BSI&DocID=330918.
National Institute of Building Science (NIBS) (2017). National BIM guide for owners [internet]. National Institute of Building Sciences Washington, DC. Available from: https://www.nibs.org/files/pdfs/NIBS_BIMC_NationalBIMGuide.pdf.
National Institute of Building Sciences (NIBS) (2015). Construction operation building information exchange (COBie) – version 2.4 [internet]. Available from: https://www.nationalbimstandard.org/files/NBIMS-US_V3_4.2_COBie.pdf.
NATSPEC (2013). BIM and LOD - building information modelling and level of development [internet]. Construction information systems limited ABN 20 117 574 606. Available from: https://bim.natspec.org/images/NATSPEC_Documents/NATSPEC_BIM_LOD_Paper_131115.pdf.
Pärn, E. A., Edwards, D. J., and Sing, M. C. P. (2017). The building information modelling trajectory in facilities management: A review. Automation Constr. 75, 45–55. doi:10.1016/j.autcon.2016.12.003
Parsanezhad, P., and Dimyadi, J. (2013). Effective facility management and operations via a BIM-based integrated information system. [cited 2022 Sep 2]; Available from: https://www.researchbank.ac.nz/handle/10652/3034.
Patacas, J., Dawood, N., and Kassem, M. (2020). BIM for facilities management: A framework and a common data environment using open standards. Automation Constr. 120, 103366. doi:10.1016/j.autcon.2020.103366
Patacas, J., Dawood, N., Vukovic, V., and Kassem, M. (2015). BIM for facilities management: Evaluating BIM standards in asset register creation and service life planning. J. Inf. Technol. Constr. 20 (10), 313–318.
Patacas, J. M. D. L., Dawood, N., and Kassem, M. (2014). Evaluation of IFC and COBie as data sources for asset register creation and service life planning.
Phelps, A. F., and Horman, M. J. (2010). Ethnographic theory-building research in construction. J. Constr. Eng. Manag. 136 (1), 58–65. doi:10.1061/(asce)co.1943-7862.0000104
Pishdad-Bozorgi, P., Gao, X., Eastman, C., and Self, A. P. (2018). Planning and developing facility management-enabled building information model (FM-enabled BIM). Automation Constr. 87, 22–38. doi:10.1016/j.autcon.2017.12.004
Poirier, E. A., Forgues, D., and Staub-French, S. (2017). Understanding the impact of BIM on collaboration: A Canadian case study. Build. Res. Inf. 45 (6), 681–695. doi:10.1080/09613218.2017.1324724
Porwal, A., and Hewage, K. N. (2013). Building Information Modeling (BIM) partnering framework for public construction projects. Automation Constr. 31, 204–214. doi:10.1016/j.autcon.2012.12.004
Richards, L., and Morse, J. M. (2012). README FIRST for a user’s guide to qualitative methods. SAGE, 337.
Rojas, M. J., Herrera, R. F., Mourgues, C., Ponz-Tienda, J. L., Alarcon, L. F., and Pellicer, E. (2019). BIM use assessment (BUA) tool for characterizing the application levels of BIM uses for the planning and design of construction projects. Adv. Civ. Eng. 2019, 1–9. doi:10.1155/2019/9094254
Solihin, W., Eastman, C., and Lee, Y. C. (2015). Toward robust and quantifiable automated IFC quality validation. Adv. Eng. Inf. 29 (3), 739–756. doi:10.1016/j.aei.2015.07.006
Son, S., Lee, G., Jung, J., Kim, J., and Jeon, K. (2022). Automated generation of a model view definition from an information delivery manual using idmXSD and buildingSMART data dictionary. Adv. Eng. Inf. 54, 101731. doi:10.1016/j.aei.2022.101731
Succar, B., Saleeb, N., and Sher, W. (2016). Model uses: Foundations for a modular requirements clarification language. Australasian Universities Building Education, 1–12.
Succar, B. (2009). Building information modelling framework: A research and delivery foundation for industry stakeholders. Automation Constr. 18 (3), 357–375. doi:10.1016/j.autcon.2008.10.003
Tang, P., Huber, D., Akinci, B., Lipman, R., and Lytle, A. (2010). Automatic reconstruction of as-built building information models from laser-scanned point clouds: A review of related techniques. Automation Constr. 19 (7), 829–843. doi:10.1016/j.autcon.2010.06.007
Tauriainen, M., Marttinen, P., Dave, B., and Koskela, L. (2016). The effects of BIM and lean construction on design management practices. Procedia Eng. 164, 567–574. doi:10.1016/j.proeng.2016.11.659
Thabet, W., Lucas, J., and Johnston, S. (2016). A case study for improving BIM-FM handover for a large educational institution, 2177–2186.
Tsay, G. S., Staub-French, S., and Poirier, É. (2022). BIM for facilities management: An investigation into the asset information delivery process and the associated challenges. Appl. Sci. 12 (19), 9542. doi:10.3390/app12199542
U.S. General Service Administration (GSA) (2011). GSA building information modeling guide 08 - BIM guide for facility management [internet]. Available from: https://www.gsa.gov/real-estate/design-excellence-program/3d4d-building-information-modeling/bim-guides/bim-guide-08-facility-management.
Utiome, E., and Drogemuller, R. (2013). An approach for extending Building Information Models (BIM) to specifications. Tsinghua University Press, 290–299.
Wand, Y., and Wang, R. Y. (1996). Anchoring data quality dimensions in ontological foundations. Commun. ACM 39 (11), 86–95. doi:10.1145/240455.240479
Wang, R. Y., and Strong, D. M. (1996). Beyond accuracy: What data quality means to data consumers. J. Manag. Inf. Syst. 12 (4), 5–33. doi:10.1080/07421222.1996.11518099
Yalcinkaya, M., Singh, V., Nenonen, S., and Junnonen, J. M. (2016). Evaluating the usability aspects of construction operation building information exchange (COBie) standard. TUT–Tampere University of Technology.
Yalcinkaya, M., and Singh, V. (2019). VisualCOBie for facilities management: A BIM integrated, visual search and information management platform for COBie extension. Facilities 37 (7/8), 502–524. doi:10.1108/f-01-2018-0011
Zadeh, P. A., Wang, G., Cavka, H. B., Staub-French, S., and Pottinger, R. (2017). Information quality assessment for facility management. Adv. Eng. Inf. 33, 181–205. doi:10.1016/j.aei.2017.06.003
Zmud, R. W. (1978). An empirical investigation of the dimensionality of the concept of information. Decis. Sci. 9 (2), 187–195. doi:10.1111/j.1540-5915.1978.tb01378.x
Keywords: information quality (IQ), building information model (BIM), facilities management (FM), modeling practices, BIM for FM, model-based project delivery, COBie, ISO 19650
Citation: Tsay GS, Staub-French S, Poirier E, Zadeh P and Pottinger R (2023) BIM for FM: understanding information quality issues in terms of compliance with owner’s Building Information Modeling Requirements. Front. Built Environ. 9:1117066. doi: 10.3389/fbuil.2023.1117066
Received: 06 December 2022; Accepted: 24 April 2023;
Published: 11 May 2023.
Edited by:
Ha Tran, The University of Melbourne, AustraliaReviewed by:
Pedro Mêda, University of Porto, PortugalMehran Oraee, The University of Melbourne, Australiadsul
Copyright © 2023 Tsay, Staub-French, Poirier, Zadeh and Pottinger. This is an open-access article distributed under the terms of the Creative Commons Attribution License (CC BY). The use, distribution or reproduction in other forums is permitted, provided the original author(s) and the copyright owner(s) are credited and that the original publication in this journal is cited, in accordance with accepted academic practice. No use, distribution or reproduction is permitted which does not comply with these terms.
*Correspondence: Gustavo S. Tsay, gtsay@mail.ubc.ca; Sheryl Staub-French, sherylsf@civil.ubc.ca