- 1Department of Architectural Systems Engineering, October University for Modern Sciences and Arts (MSA University), Greater Cairo, Egypt
- 2Faculty of Engineering, October University for Modern Sciences and Arts (MSA University), Greater Cairo, Egypt
As a response to rising housing prices and the high cost of materials in the building and construction industry, a rural prototype house (the Ecofordable House) was built with alternative technologies. The house is located in the western desert zone of Giza, Egypt, and features enhanced vernacular technologies with local materials. Interlocking compressed stabilized earth brick walls, partially reinforced, jack arch and funicular shell roofs, and date palm midribs were employed in an attempt to reduce the usage of steel, fired bricks, cement, and imported wood. The present research evaluates the house’s construction cost-effectiveness and affordability through detailed real-world data and comparisons of material quantities, labor, and costs with those of conventional methods. The “price-to-income ratio” is used as an indicator of affordability. According to the findings, walls cut costs by half, roofs by a quarter, and midribs by two-thirds; the alternatives combined saved 45%, and the house saved a quarter of the cost after adding common expenses. Moreover, less than one-third of steel, fired bricks, and cement were utilized. In the Egyptian context of government-built houses, the prototype would be affordable for most Egyptian income brackets while the conventional house was expensive for the lowest three. The findings provide empirical support for the economic advantages of enhanced vernacular technologies as alternatives and address residential affordability in similar contexts.
1 Introduction
1.1 Global issues
In 2020, the building and construction sector accounted for 37% of global CO2 emissions (UNEP, 2021), a figure that is rising more rapidly in developing nations than in advanced economies due to rapid urbanization and significant variations in technologies and energy consumptions (Chen et al., 2021). Additionally, worldwide ‘Nationally Determined Contributions’ (NDC) to improve building energy plans often ignore construction materials, including the energy necessary for extraction, manufacture, distribution, and installation, while boosting energy efficiency and renewable post-building energy (UNEP; Zhong et al., 2021), despite the fact that 33% of the total energy consumption over life cycle is caused by the production of materials (Tan et al., 2020). Concrete and steel rebar contributed 62% of manufacturing carbon emissions, whereas masonry’s fired bricks and cement contributed 27% (note the low embodied CO2 of sand despite its weight) (Kumanayake and Luo, 2018). Wood, on the other hand, is an environmentally friendly material with low carbon content because it requires minimal processing and energy from production to disposal. Many countries, however, import wood to meet local demand. When imported, it emits 30% from manufacturing and 70% from its international transport, making it an unsustainable material (Cristea et al., 2013; Li et al., 2018; Xu et al., 2021).
At the same time, global price inflation in the construction materials, rising housing prices (Supplementary Figure S1A), and higher cost of green buildings represent obstacles to housing affordability. When considering “Price-to-income ratio” as an affordability indicator (see Section 2.6), Africa has the highest ratio (Supplementary Figure S1B), Asia is second, followed by America, Europe, and Oceania (Supplementary Figure S1C). (Sims and Fattah, 2016; Adamovic, 2023) However, careful selection and use of appropriate technologies and materials, and a reduction in transportation costs could contribute to overcoming these challenges (Weerasinghe and Ramachandra, 2020; Moghayedi et al., 2022). Due to their environmental and economic benefits, developed countries are reviving vernacular building materials and techniques, including earth. The latter has regained attention as a contemporary construction material that may be less expensive while meeting the same modern requirements. In developing countries, on the other hand, traditional and indigenous materials were replaced by concrete, fired bricks, cement, and steel to increase house durability and quality of life (UN-Habitat, 2009; 2012; Marsh and Kulshreshtha, 2021). According to Marsh and Kulshreshtha (2021)’s survey on earth constructions worldwide, this shift is growing in urban and rural areas and formerly accepted estimates that one-third of the global population resides in earth homes, exceeding 50% in developing countries, are no longer accurate. An updated estimate is 8%–10% worldwide and 20%–25% in developing countries.
Egypt is no exception in this regard. Indigenous technologies such as load-bearing mud-brick walls, domes and vaults were traditionally adopted as they adapt to the hot arid climatic zone of Egypt while offering economic benefits. These methods were almost completely phased out since the early 20th century and currently, reinforced concrete frame buildings with fired and cement brick masonry dominate the country’s construction sector, including rural areas (see Supplementary Figures S2A, B) (Sims and Fattah, 2016). Furthermore, Egypt is fully reliant on raw wood imports to meet its domestic demand (FAO, 2020a; El-Mously, 2020).
Similarly, high housing prices and low incomes are among the factors impeding home affordability in Egypt. The World Bank considers a reasonable price-to-income-ratio to be generally from 3 to 6 (Adabre and Chan, 2019), and UN-Habitat’s report on “Egypt housing profile” says a ratio of 5–7 is affordable in the Egyptian-like contexts. Only informal units had an acceptable ratio of 3.8–7.7, while the ratio was 9.4 in subsidized units, and 25.8 in non-subsidized private sector units, making them all unaffordable when the average annual income is considered (Sims and Fattah, 2016).
1.2 Research aim and contribution
In the desert area of western Giza, Egypt, a rural prototype house (Ecofordable House, EH) that integrates enhanced vernacular technologies recognized for their sustainability (see Section 2.1) was built. Based on the following review, interlocking compressed stabilized earth brick walls (ICSEB), partially reinforced, jack arch/funicular shell roofs, and palm midribs in the openings and pergolas were selected as alternatives. The research seeks to assess their potential construction cost savings and total house price affordability. (Kumanayake and Luo, 2018; Xu et al., 2021; Zhong et al., 2021).
The present research is significant because:
• The combination of walling and roofing alternatives was introduced to Egypt through this house.
• A wood substitute was incorporated in the openings and pergolas in an unconventional way.
• There is a gap in the literature and a scarcity of relevant information regarding the construction cost savings and affordability of the enhanced vernacular technologies adopted in the EH.
• The research offers original real-world data on materials, labor and total costs gathered during the construction process, and compares them to conventional methods.
While the sustainability and affordability of the adopted solutions are often acknowledged and their revival is continuously advocated, the literature focuses on their structural and environmental performance (Cowan, 1981; Venkatarama Reddy and Jagadish, 2003; Venkatarama Reddy, 2004; Vafai et al., 2005; Diodato et al., 2015; Egenti and Khatib, 2016; Touré et al., 2017; Bradley and Gohnert, 2018; González-López et al., 2018; Murmu and Patel, 2018; Fernandes et al., 2019; Moussa et al., 2019; Yang and Wang, 2019; Di Gregorio et al., 2020; Teixeira et al., 2020; Garcia-Castillo et al., 2021; Hanafi, 2021; Hany et al., 2021; Kasinikota and Tripura, 2021; Mahdad et al., 2021; Saari et al., 2021; Wang et al., 2021). A research gap on their construction costs and affordability indicators, which were not well-researched, was found. Experiments on and applications of CSEB exist in most continents, and studies in a number of countries provide precise cost and saving rates; however, the majority analyzes solid non-reinforced CSEB (Adam and Agib, 2001; UN-Habitat, 2009) and are based on feasibility studies (Davis and Maïni, 2017; Kumar et al., 2018) and digitally modelled buildings (Di Gregorio et al., 2020; Nuwagaba, 2020; Unni and Anjali, 2022). Moreover, the literature has scant information about the cost savings associated with masonry jack arch and funicular shell roofs, although historically found in Europe, America, the Middle East, Nepal and India (Keswani, 1997; Rao and Raina, 2005; Kharrufa, 2007; Eslami et al., 2012; Maheri et al., 2012; Khan et al., 2013; Zahrai, 2015; Ozdemir et al., 2017; Shabdin et al., 2020; CSIR and BMTPC, 2021; Motra et al., 2021). Studies on wood products manufactured using date palm leaf midribs (DPLM) offer no cost breakdowns or analyses (Al-Oqla et al., 2015; Metwally and Hamza, 2017; Elseify et al., 2019). This material is available in huge waste quantities across North Africa and the Middle East due to annual pruning, and its craftsmanship has long been found in rural areas (El-Mously, 2018; Midani et al., 2020).
Although the current study was conducted in Egypt and constitutes a case study, it is relevant to a broader geographical region. As previously stated and as will be discussed in the following sections, the utilised technologies have acquired interest in recent years across Europe, Africa, the MENA region, and Asia as sustainable alternatives. Furthermore, the findings could serve as a reliable reference for academics, practitioners, and contractors who are advocating or questioning the economic benefits of enhanced vernacular technologies.
2 Research methods
2.1 Review and selection of alternative technologies
2.1.1 Walling alternative
Because of its availability and suitability to hot, arid climates, earth has been used in construction since ancient times, particularly in desert regions. (Alex, 2018; Fernandes et al., 2019). Earthen houses are those that use unfired earth as a primary component. (Marsh and Kulshreshtha, 2021). They have lower environmental impact, low embodied energy, and improve air quality and thermal comfort (Uzoegbo, 2016; Yang and Wang, 2019; Kasinikota and Tripura, 2021). They require less energy to extract, manufacture, and transport materials, have high load-bearing capacity (UN-Habitat, 2009; Burroughs, 2010; UN-Habitat, 2012; Kulshreshtha et al., 2020), and are fire and insect resistant (Egenti and Khatib, 2016; Yang and Wang, 2019). Despite the advantages of earthen masonry constructions, they were abandoned due to their link with poverty, reduced durability, reduced shear and tensile strengths, and consequent shift to reinforced concrete buildings with fired and cement bricks. In recent decades however, earth techniques have regained popularity due to concerns about the environment and affordability (Burroughs, 2010; Fernandes et al., 2019; Di Gregorio et al., 2020; Kulshreshtha et al., 2020; Marsh and Kulshreshtha, 2021).
The UN-Habitat (UN-Habitat, 2009; UN-Habitat, 2012) and Marsh and Kulshreshtha (2021) have listed several types of earthen masonry depending on the context:
• Compressed stabilized earth bricks or blocks (CEB or CSEB), often known as an enhanced version of adobe bricks, are created by a machine pressing a mixture of soil and a stabilizer.
• Interlocking stabilized soil (earth) bricks or blocks (ISSB/ICSEB), which use the same CEB mixtures but are interlocking.
• Adobe bricks or blocks, which are formed by compacting a clay-straw mixture and have less consistent shapes than CEBs.
• Cob, which are flowing wall and roof shapes formed from clay, sand, and straw.
• Rammed earth, which involves the construction of a mold into which the soil is compacted in layers and allowed to dry.
• Wattle and daub, a type of clay daub strengthened vertically and horizontally by a timber or bamboo frame.
In March 2016, Egypt adopted its first code, “Building with stabilized earth—Part One: Building with compressed stabilized earth units,” highlighting CSEB as a potential alternative to adobe and fired brick masonry units (EG-SE2016). Hanafi (2021)’s investigation on the possibilities of employing CSEB in Upper Egypt’s rural areas discovered that CSEB as a modern version of adobe bricks was the most environmentally, economically, and socially appropriate; also confirmed in other contexts (UN-Habitat, 2009; Di Gregorio et al., 2020; Kasinikota and Tripura, 2021).
CSEB are made by manually or hydraulically compressing soil, water, and a stabilizer. The quality of bricks depends greatly on the soil type, compaction force, type and percentage of stabilizer, production and curing procedures, and weather (Wells, 1993). Cement is the most prevalent stabilizer associated with sandy soil CSEB, and the brick is still considered green with up to 10% by weight because cement contributes to its strength and durability (UN-Habitat, 2009; UN-Habitat, 2012; Bogas et al., 2019; Edris et al., 2021; Kasinikota and Tripura, 2021). Although recent researches has experimented with natural materials, as well as agricultural and industrial waste to minimize or replace cement (fly ash, polymers, geopolymer binders, sugarcane fibers) (Omar Sore et al., 2018; Idriss et al., 2022; Tchouateu Kamwa et al., 2022; Nadia et al., 2023), cement was selected in the production of EH bricks. This is because it is the most tested and utilized stabilizer associated with CSEB production, its commercial availability and lower price in Egypt, higher strength results, and most importantly its suitability to sandy soil and mass-production (UN-Habitat, 2009; UN-Habitat, 2012; Bogas et al., 2019; Edris et al., 2021; Kasinikota and Tripura, 2021).
When interlocking (ICSEB or ISSB), CSEB gains additional benefits because this feature reduces the need for conventional mortar thickness and cement quantity (UN-Habitat, 2009, 2012), provides greater in-plane and out-of-plane shear resistance, reduces skilled labor requirement, enables self-construction, and cuts construction time (Al-Jabri et al., 2005; Uzoegbo, 2016; Ma et al., 2019; Di Gregorio et al., 2020; Wang et al., 2021). When left unfinished, ICSEB walls should be coated with water-based resin or acrylic varnish to withstand moisture and boost durability (Di Gregorio et al., 2020). When reinforced, masonry walls in general gain more resistance to lateral forces. CSEB manufactured with holes allows wall reinforcement by installing rebars and filling them with concrete, giving the structure resilience and reduces its cost (UN-Habitat, 2009; UN-Habitat, 2012; Di Gregorio et al., 2020; Saari et al., 2021). Moreover, they become lighter, allow internal electrical conduits (Di Gregorio et al., 2020), and have improved thermal performance (Uzoegbo, 2016).
In the Ecofordable House, compressed stabilized earth brick walls with interlocking and reinforcing characteristics (hollow ICSEB) and finished with water-based acrylic varnish were used because of these benefits and aspects.
2.1.2 Roof alternatives
Curved roofs under compression, such as vaults, domes, catenaries, and doubly curved roofs (funicular shells), have traditionally been utilized in many buildings, including houses, temples, mausoleums, palaces, churches, and mosques. They were found in the Middle East at a very early date, and were typically made of sun-dried bricks, fired bricks, or stones, depending on the available local materials (Cowan, 1977; Cowan, 1981; Öztürk et al., 2020; Duarte et al., 2021).
According to the UN-HABITAT report on traditional and industrialized building materials, masonry vaults and domes were traditionally common across Egyptian countryside (Sims and Fattah, 2016). Although they have aesthetic, economic, and environmental benefits, they also have certain potential weaknesses such as brittleness, poor tensile strength, and cracking (Bradley et al., 2018). Solutions that might improve their performance include the integration of beams capable of withstanding the lateral force generated by the structure. Hybrid roofs that combine the structural advantage of beams and aesthetic and economic advantages of masonry are found in funicular shell domes and jack-arch vaults (Sivakumar et al., 2015).
“Funicular” means “having the form of or associated with a cord usually under tension” (Merriam-Webster, 2023a). If it is formed like a catenary, the shell is under total tension as a rope, and when turned upside down, it forms a single curve under compression. Funicular shells are doubly-curved (catenary in both axes). These have higher ultimate strength because the dead load is evenly distributed. Funicular shells can cover large areas as a single shell or as waffle slabs. They span square, rectangular, triangular, and non-orthogonal spaces (Cowan, 1977; Jain, 2000; ICAEN, 2004; Kumar and Maheswari, 2019). The largest masonry catenary structure is in the Great Hall of the Palace of Taq Kisra, built around 550A. D. This vault inspired the modern catenary thin concrete shell (Cowan, 1977; Cowan, 1981; Duarte et al., 2021), possibly foreseen by Robert Hooke in 1,675 and influential throughout the 20th century (Cowan, 1981; Jannasch, 2017). A funicular roofing system can be made with concrete shells, bricks, or waste stone/ceramic pieces supported by RC beams. This type provides an improved alternative solution that blends traditional and modern technology, allowing the adoption of natural resources, optimizing the use of steel and cement, adding upper stories, improving aesthetics, and ensuring stability (Keswani, 1997; Jain, 2000; ICAEN, 2004; Kumar and Maheswari, 2019). EH utilized funicular shells made of bricks and waste stone/ceramic, a solution that could significantly contribute to the issue of waste in Egyptian, as well as other countries’, building sector (Daoud et al., 2021). This roof was adopted by several building centers in India (mainly Anangpur Building Center) (Keswani, 1997). A single shell spans 0.8–3.0 m with a rise to span ratio of 1/6, allowing for a second floor (Keswani, 1997; ICAEN, 2004; CSIR and BMTPC, 2021).
A “jack arch” is defined as “a flat arch (as a lintel with a keystone)" (Merriam-Webster, 2023b). The term “Jack” refers to “something that supports or holds in position” as well as “to move or lift” (Merriam-Webster, 2023c). The jack arch roof is a composite roof (Venkatarama Reddy, 2009; Venkatarama Reddy et al., 2014) (also known as a floor) that is an improved version of traditional vaults (ICAEN, 2004). It is made up of brick or concrete vaults that are held up by RC beams or rolling steel joists (I-beams). Single brick arches are placed on edge to make a masonry jack arch using reusable sliding formwork. Beams are typically 1–1.5 m wide and can be prefabricated or cast in place. The rise-to-span ratios of the arches range from 1/8 to 1/12, resulting in a relatively flat roof for intermediate floors. Because the horizontal thrust from the arches cancels each other out, the beams are only intended for vertical loads. However, steel tie rods are transversally installed in the outer bays as arches lack balancing horizontal thrust (Adam and Agib, 2002; BDC 13, 1981; ICAEN, 2004; Khan et al., 2013). Historically, jack arch roofs were made of brick vaults supported by timber joists, which were later replaced by iron as new industrial materials became available. In more recent years, RC beams have been introduced using the same technology (Diodato et al., 2015; Garcia-Castillo et al., 2021). The jack arch originated in Europe, expanded to the Americas, and by the mid-twentieth century was used in East Europe, the Middle East (Maheri et al., 2012; Khan et al., 2013) as seen in Iran (Eslami et al., 2012; Zahrai, 2015; Shabdin et al., 2020), Iraq (Kharrufa, 2007), Turkey (Ozdemir et al., 2017), and Sudan (Mukhtar, 1980; Adam and Agib, 2002), Nepal (Motra et al., 2021), and the Indian subcontinent (Maheri and Rahmani, 2003; Maheri et al., 2012; Khan et al., 2013). It provided span flexibility, upper levels, structural safety, ease of building due to labor and material availability, and good thermal performance (Maheri and Rahmani, 2003; Khan et al., 2013; Leo Samuel et al., 2017). Steel I-beams were one reason the jack arch became socially unpopular and associated with poverty, according to Kharrufa (2007). It caused cracks along the plaster of joists and sometimes at their wall intersections, giving the finish a poor, crackly look. EH incorporated RC joists and lateral beams to address these challenges.
2.1.3 Wood alternative
For over 5,000 years, the Date Palm has been planted throughout the Middle East and North Africa (El-Mously, 2018; Midani et al., 2020). Its Latin name “phoenix dactylifera” comes from “phoenix daktulos,” where “phoenix” refers to the color purple or red in Greek and “daktulos” means finger, pointing to the fruit’s color and form (Zaid and de Wet, 2002; Ghnimi et al., 2017). The Middle East and North Africa alone produce 90% of the world’s date, with Egypt topping the list with 18% in 2020 (FAO, 2020b). The date palm has long been an essential element of the region’s economic and social lives (Agoudjila et al., 2011); it may live for more than 100 years, leaving an average of 13 leaves (per palm) as a huge agricultural waste due to the yearly pruning (Midani et al., 2020). Pruning entails eliminating products that have already served their natural purposes and have become unnecessary to the palm, such as midribs and leaflets (El-Sharbasy, 2018; El-Mously, 2020). The midribs have been traditionally used as a wooden alternative in rural areas for making roofs, fences, furnishings, and boxes, whilst leaflets have been used to create utensils as baskets, mats, hats, brooms (Zaid and de Wet, 2002; El-Mously, 2018). However, the introduction of plastic and a shift in Egyptian consumption patterns caused a drop in date palm-related items since the 1950s (El-Mously, 2018). At the same time, Egypt, like most Arab countries, is dry with little tree cover, making its market fully reliant on imported raw wood. In 2020 for instance, 4.3 million m3 of sawnwood and industrial roundwood were imported to meet the local demand (FAO, 2020a).
Since 1991, researchers at Ain Shams University have experimented with DPLM to evaluate it as a wood substitute. Being able to withstand hot desert storms and millions of loading cycles without breaking due to its unique flexible tissue (Agoudjila et al., 2011; El-Mously, 2018; Midani et al., 2020), the midrib has equivalent physical and mechanical qualities to commercial woods such as beech and spruce (El-Mously, 2018, 2020). Several attempts have created blockboard, particleboard, and blocks with DPLM, but their uses were limited to small scale crafts and industries due to expensive machinery and time-intensive processing (El-Mously, 2018; El-Mously, 2020; El-Mously and Darwish, 2020). The EH have unconventionally reintroduced the DPLM craftsmanship in the manufacture of doors, shutters and pergolas to combine durability while reviving the functions and patterns of wooden mashrabiyyas, a latticework found on the windows of historic Cairo that traditionally ensured privacy and improved indoor thermal comfort (Abdel Gelil, 2006).
2.2 Review on the construction cost of selected technologies
2.2.1 Compressed stabilized earth brick walls (CSEB)
Direct costs of CSEB construction depend on the availability of suitable soil for brick production, current material prices (especially stabilizer), transportation, equipment, wage rates, labor productivity, and construction techniques (Pacheco-Torgal and Jalali, 2012; Uzoegbo, 2016). In the Indian context, a finished solid CSEB masonry is between 15% and 20% less expensive than fired bricks (Singh et al., 2022). A proposed model for alternative multi-story low-cost housing in India suggests that the price of an apartment with masonry CSEB is 13% lower than with reinforced concrete and fired brick walls (Unni and Anjali, 2022). They explained that this reduction is inconsistent with other research studies that suggested savings of up to 40% and that this was justified by the fact that all components, except the CSEB walls, were similar to conventional housing. Using a digital proposal for an Ugandan house, Nuwagaba (2020) found that, while hollow concrete blocks had less embodied energy than fired bricks, they were 36% more expensive per m2 wall, confirming UN-Habitat (2009)’s calculations. CSEB walls were 18% cheaper and had less embodied energy than fired-brick walls (Nuwagaba, 2020). In Sudan, Adam and Agib (2001) revealed that using CSEB blocks in “Al-Haj Yousif experimental prototype school” cut wall costs by 70% when done ‘self-help.’ With Kenaf sand cement corrugated sheets roofing, savings were 40% per m2. In Kenya, CSEB walls cost 20%–70% less than concrete block walls, depending on production.
A feasibility study in Sri Lanka by Davis and Maïni (2017) discovered that CSEB technologies are labor-intensive, with labor costing 60% and materials 40%. According to the report, a 14 cm manually pressed CSEB wall costs 2,007 LKR/m2, a motorized press CSEB wall costs 1,850 LKR/m2, and a 19 cm fired brick wall costs 2,803 LKR/m2. As a result, the first is 28.4% less expensive, while the second is 34% less expensive than fired bricks. When including press, infrastructure, and other costs, CSEB is 26.2% cheaper for manual blocks and 29.9% cheaper for motorized blocks.
Another feasibility study (Kumar et al., 2018) looked into the economic benefits of reinforced interlocking CSEB in the Gulf Coast region of the United States. ICSEB walls, which cost 38,891 $, and CSEB walls, which cost 57,890 $, were 42% and 13.5% less expensive than fired-brick walls, which cost 66,997 $, respectively. A house would cost 104,000 $ if built with reinforced ICSEB, 123,000 $ with CSEB, and 132,107 $ with fired bricks, with the first and second methods being 27% and 18.3% cheaper than fired bricks, respectively.
In South Africa, cement stabilized ICSEB is about half the cost of comparable concrete masonry (Uzoegbo, 2016). In Brazil, the estimated costs of a reinforced ICSEB masonry system is 14% less expensive than traditional fired bricks with RC components, using purchased ICSEB. Making bricks on-site saves 23%, while community engagement in building could save up to 50% of the house’s cost (Di Gregorio et al., 2020).
2.2.2 Hybrid roofs
Several factors make the funicular shell domes economical. First, the amount of steel required for the supporting beams is reduced by 75% (Keswani, 1997). Another study found a 60% save in steel (CSIR and BMTPC, 2021). Second, cement is used only for beams and exterior plastering, saving an average of 35% (CSIR and BMTPC, 2021). Third, free waste materials are used. Fourth, inexperienced workers can build it without centering, unlike masonry domes and vaults (Keswani, 1997; CSIR and BMTPC, 2021). Fifth, in large-scale production, shuttering timber can be replaced with reusable fiberglass molds. Rao and Raina (2005) found that a brick funicular shell roof saves 30% compared to a conventional RC roof. No cost breakdown was found in the literature.
The jack-arch vault, on the other hand, may last for more than 30 years with little or no maintenance with suitable design and finishing (Adam and Agib, 2002). Additionally, it is a less expensive alternative, saving up to 15% of the cost of a typical RC roof, according to Rao and Raina (2005). Again, no analysis was found in the literature to support these figures.
2.2.3 Date palm midrib (DPLM)
In the literature, there was no cost information on DPLM products and very little structured research on raw material costs was found. The raw material was discovered to be 25%–90% less expensive than other natural fiber sources (Al-Oqla et al., 2015; Elseify et al., 2019). A 2015–2017 economic survey on DPLM in Damietta Governorate (Metwally and Hamza, 2017) found that the profitability for investing 1000 DPLM was 35%, depending on the application, which ranged from crates to chairs. 1000 DPLM cost 557 E£; the total is 1453 E£ when adding all expenses, with labor accounting for more than half (52.2%).
2.3 The Ecofordable House description
The design of the constructed model (Figures 1, 2) was awarded first prize in the “Green Urbanism and Innovative Architecture” track (fourth Cairo International Exhibition of Innovation). The alternative technologies were compliant with “Egyptian code for Design and Construction of Concrete Structures” (ECP 203–2018), “Design and Construction of Masonry Works” (ECP 204–2005), and “Building with stabilized earth–part one—2016" (EG-SE2016). The main walling unit is the interlocking compressed stabilized earth brick (ICSEB) (Figure 1C). It is composed of sandy soil, stabilized with Portland cement, and contains two holes that allow reinforcement to act as columns in some locations. The house is roofed with a hybrid system of 15 jack arches (small span vaults 0.8–1 m wide) and six funicular shells (small squared domes 2 m × 2 m), all supported by a grid of RC beams (Figure 1A). Various shells of either fired bricks, marble and granite pieces, limestone, or ceramic pieces were built to compare costs, production, and appearance. The craftsmanship of the date palm leaf midribs (DPLM) was used to make shutters, internal doors, and pergolas. The one-storey house has a porch, an entrance hall, an enclosed living room/reception that can be connected to the entrance hall and kitchen when needed, two bedrooms, one of which can be turned into an extended living room/reception, a kitchen, a bathroom, a roofed terrace, and an external staircase. The internal area without walls is 69.5 m2 and the total house area is 100 m2 (Supplementary Table S1; Figures 1, 2). Supplementary Table S2 provides the specifications for the materials used in the house’s construction (detailed in the next sections).
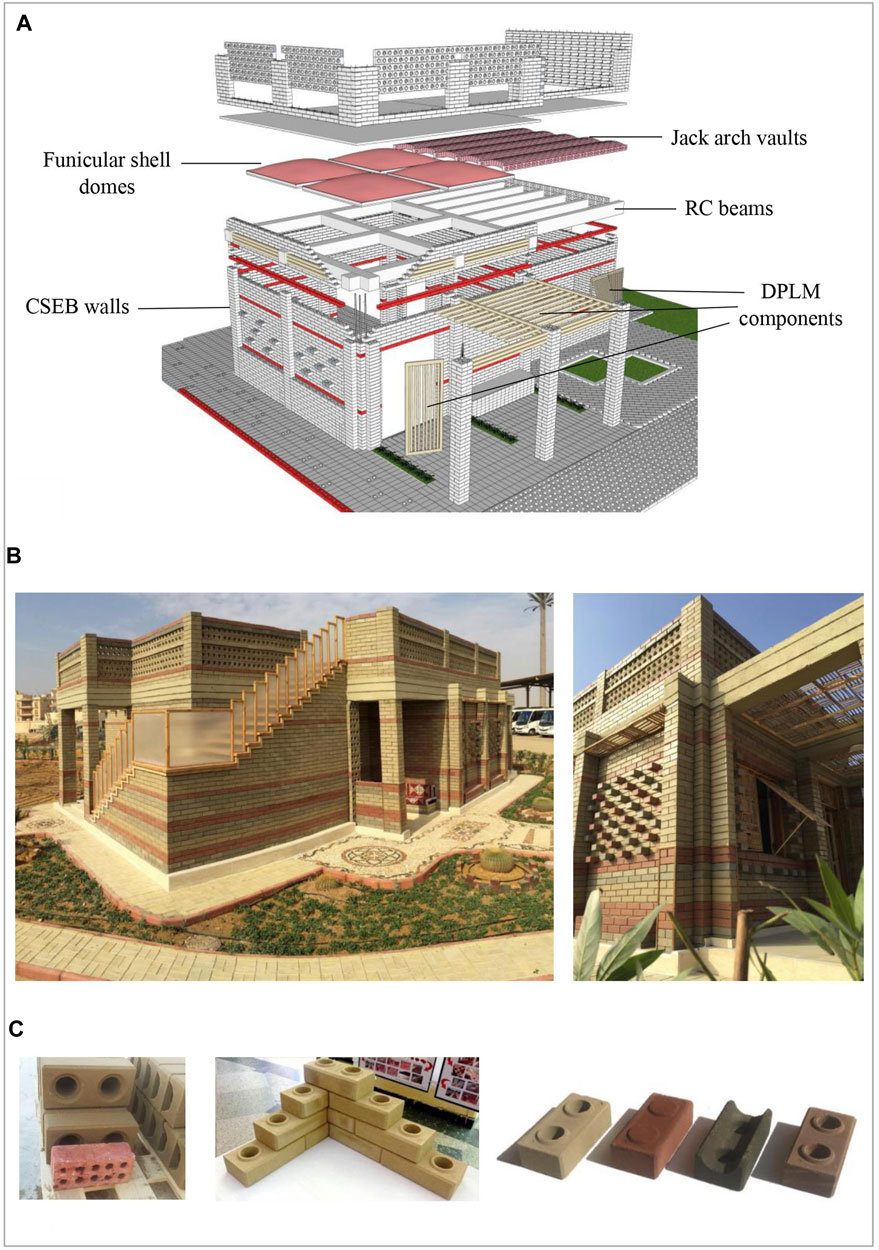
FIGURE 1. The “Ecofordable House” built at MSA University in Egypt (A) initial proposal, (B) the EH exterior, (C) various CSEB produced on campus (Source: authors).
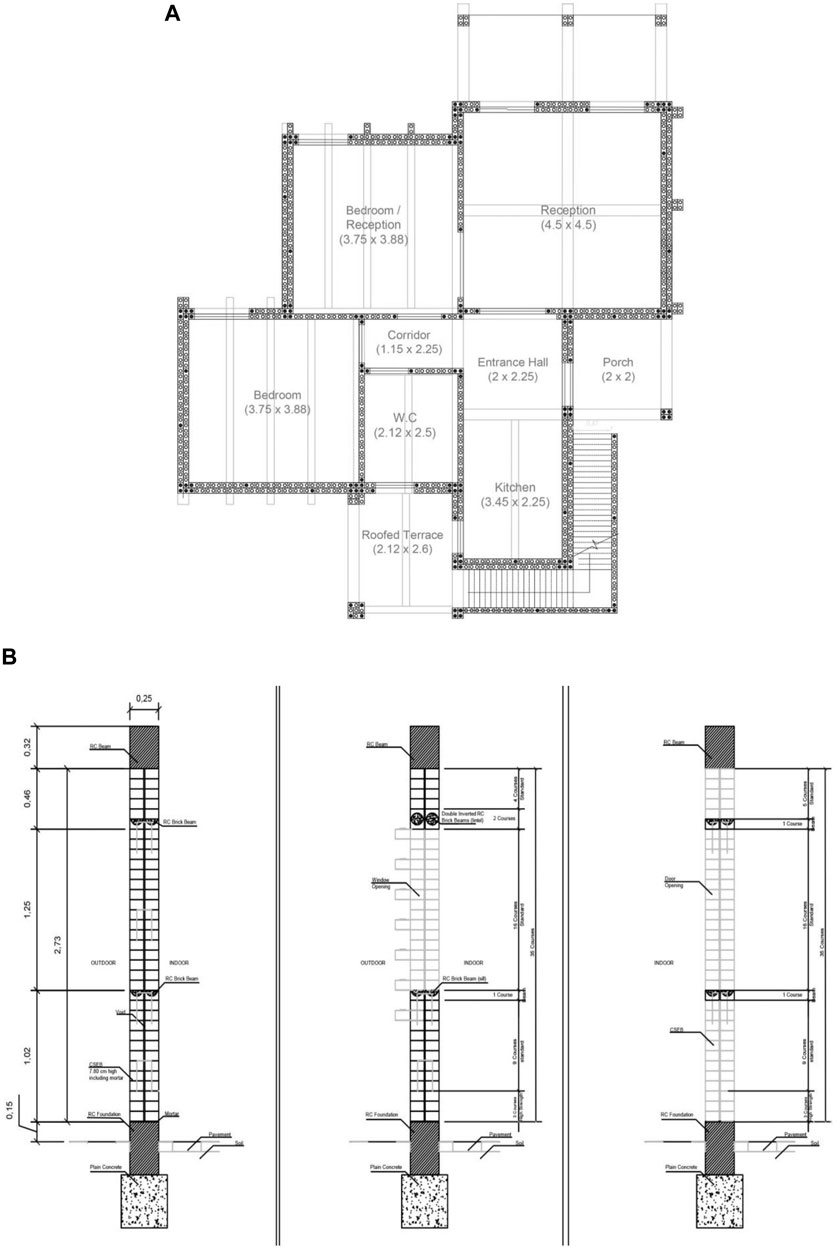
FIGURE 2. EH Masonry: (A) floor plan showing also the reinforced holes and RC beam grid of the roofing system, (B) sections in a solid wall (left), through a window (middle), and through a door (right) (Source: authors).
2.4 Construction techniques of alternative components
2.4.1 Construction of reinforced ICSEB walls
External and internal walls are 25 cm and 12.5 cm thick (as per ECP 204–2005) and reinforced based on the structural design (Figures 2A, B). They are made of 25 × 12.5 × 7.5 cm hollow CSEB. The bricks were manufactured from sandy soil available at the MSA University’s campus (70%–75% sand, 25%–30% fine particles, including 10% clay), and field tests determined their suitability based on EG-SE2016. Standard bricks were made of 90% soil and 10% cement stabilizer by weight (Supplementary Table S3); water was added gradually during mixing, with manual tests to determine the optimal amount. The mixture was then pressed in a semi-hydraulic machine. High strength bricks (HS) for the first three courses and two brick beam courses were made with a 15% cement mixture, with some variations if coloring oxide is added. The bricks were then cured in a humid environment for 28 days. Standard bricks have an average compressive strength of 5.1 N/mm2 and water absorption of 13.6%, while high strength bricks have more than double the strength, 11.4 N/mm2, and lower water absorption, 10.7%. A clear water-based acrylic varnish significantly reduced the standard brick’s water absorption to 6.2%.
The following are brief explanations of the construction steps (see Figure 3).
• 80 × 40 cm plain concrete foundations were casted.
• Above them, 25 × 60 cm RC strip foundations were cast.
• The first course of bricks was laid to mark the locations of reinforcements per the design.
• 165 cm long, Ø 12 rebars were anchored in specific locations with a depth of 15 cm.
• The first course of high strength (HS) bricks was then built with 1:4 cement mortar (cement:sand, dry), followed by two high strength courses built with slurry mortar as the remaining courses.
• The remainder of the wall was built with standard bricks. To mimic the Islamic ablaq, red or grey ICSEB courses were occasionally added for aesthetic purposes, using a small amount of iron oxide.
• The rebars required for the remaining height were tied to the first ones with a 15–20 cm overlap.
• Every 50 cm, steel stirrups Ø 8 were used to join perpendicular walls (T-shape and corner), and concrete grout 1:2:1 (cement: sand: gravel, dry) was poured in the steel bars holes.
• A course of special beam bricks, horizontally reinforced with Ø 8 steel bars fixed to the vertical rebars, ran twice: at the sill and lintel levels.
• Electrical conduits were easily installed in the brick holes while the walls were being built. After the construction was finished, three layers of water-based acrylic varnish were sprayed on the walls to provide humidity resistance.
2.4.2 Construction of jack arches and funicular shells
Figure 4A shows the completed jack arch and funicular shell roofs, while Figure 4B depicts their construction steps.
• At roof level, reinforced concrete beams were partially cast halfway up. The design determined their cross section and rebar count (Ø 12). The funicular shells were supported by 25 cm × 32 cm beams with shear reinforcement at the edges. Jack arches’ joists were 16 cm × 32 cm (WxH), and their edge beams were identical to funicular shells but without shear reinforcement because the tie rods compensated.
• The mid-heights of the beams serve as the starting point for the funicular shell and jack arches.
• To construct a funicular shell, the curved profile of the shell was created with mud by measuring the rise of the shell at symmetrical places over the span of the shell in both directions.
• A flat formwork similar to that used for casting an RC slab was created to hold the mud mold.
• A small layer of sand was distributed on the shell’s mud mold, and then stones, bricks, or broken tiles (granite, marble, ceramic), etc. were led along the curvature in the desired patterns.
• To protect the finishing materials, a rich cement slurry mortar in a 1:2 ratio was poured over the units.
• When filled with this mortar, the spaces between the pieces act as joints (micro-ribs).
• A second layer of bricks or broken stone is placed on the edges to create an arch action.
• For constructing a jack arch, reusable wooden formwork was pre-prepared according to its shape.
• The fired bricks are then conventionally built into a vault with 1:4 cement mortar.
• Shells and arches were then covered with cement mortar and after 48 h, molds were removed.
• Three transversal Ø 12 tie rods were placed along jack arches with no adjacent arches or shells before casting the remaining height of the beams. The remaining beam height was then casted in-situ.
• Sand was utilized as a roof filler to provide a flat surface; ceramic roof tiles were laid normally.
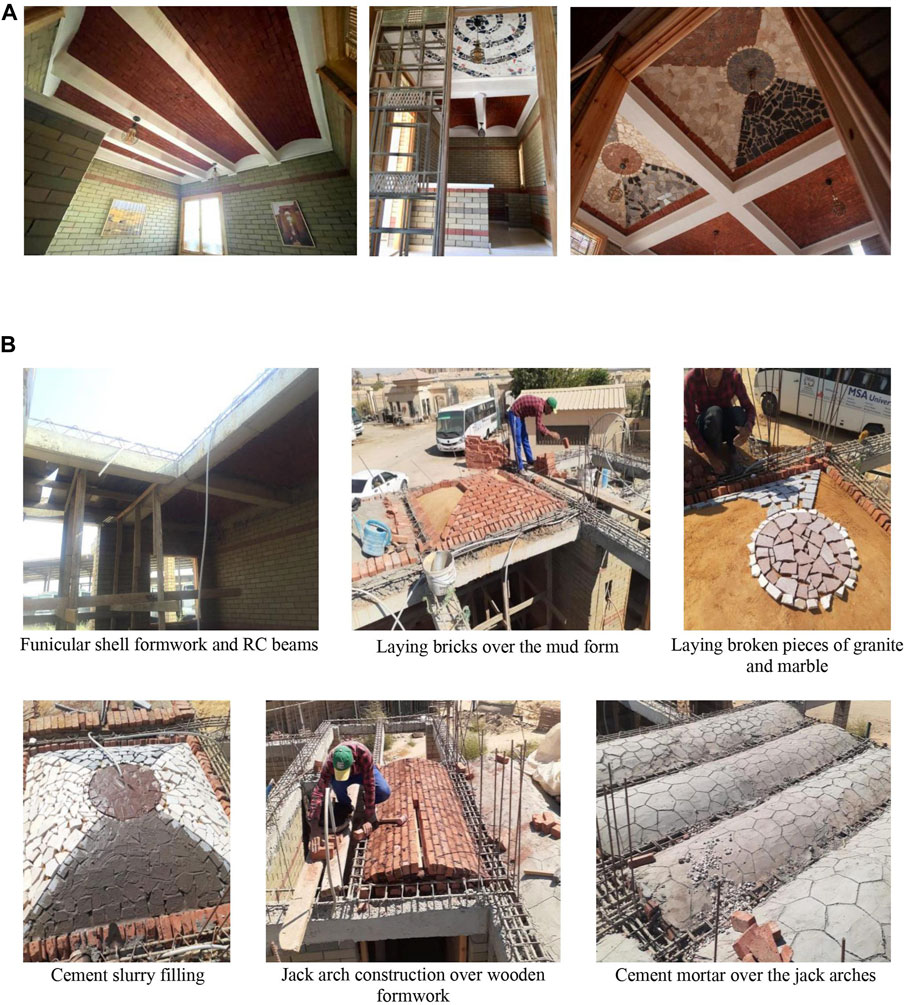
FIGURE 4. EH Alternative roofing: (A) jack arches and ceramic shell in the kitchen and bedrooms (DPLM doors are shown before installing the polycarbonate sheets), and broken marble/granite and brick funicular shells in the LR; (B) parts of the construction of funicular shell and jack arch (Source: authors).
2.4.3 Manufacturing of DPLM components
Palm midribs replaced wood in internal doors, window shutters, and pergolas (Figures 5A, B). The architect communicated the designs to the craftsman, who manufactured them using traditional furniture and crates making techniques (Figure 5A) and hand tools. The midrib is divided into slats, which are then inserted together to create latticework patterns. To protect the midrib from potential mites, a pesticide (Clorzane) was sprayed. Because the DPLM was bent, wooden strips were attached to the vertical edges of the door to secure and straighten the midribs. Furthermore, polycarbonate sheets were installed and secured behind the DPLM patterns of the doors (Figure 5B).
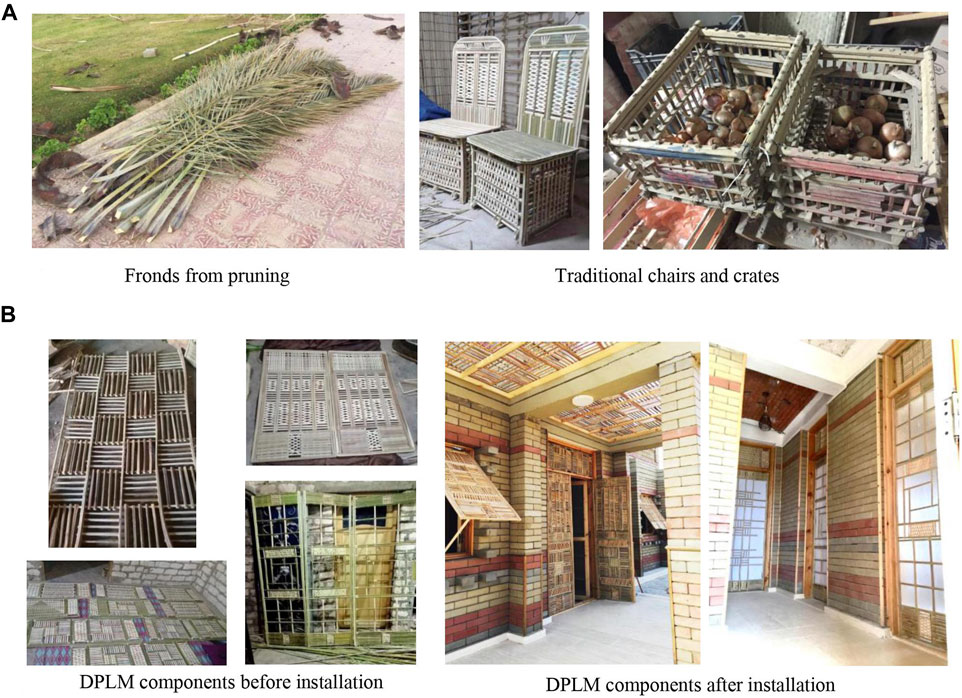
FIGURE 5. Date palm midribs (DPLM) products: (A) fronds from pruning, chairs and crates; (B) the house’s DPLM internal doors, shutters and pergolas (before and after installation) (Source: authors).
2.5 Methodology for determining construction costs
Figure 6 shows the methodology followed to gather data and determine EH construction cost and saving rates. The center responsible for project decided upon required materials, labor, hours, and tools, which the institution’s procurement department followed to purchase materials and tools and pay labor according to agreed-upon wages. Second, engineers and trainees used a daily report form to document construction data. Third, regular meetings reviewed total expenses and price updates. Fourth, alternative system total costs and unit rates were analyzed. Fifth, professional contractors developed a BOQ for building a similar model using conventional techniques. Sixth, the breakdown and overall costs of the two models were compared and savings estimated to assess EH cost-effectiveness. The assumed reduction in the quantities of cement, steel, and fired bricks was also observed.
2.6 Affordability
The home price-to-income ratio (PIR) is frequently used to evaluate affordability and market values due to its ease of calculation based on readily accessible data, such as property price and household income. The World Bank, the UN, the Joint Center for Housing Studies at Harvard University, and other institutions have all used it. (Adabre and Chan, 2019; Leung and Tang, 2023). The following equation is used:
Affordability in Egypt was estimated using the latest CAPMAS (Central Agency for Public Mobilization and Statistics) data on the 10 Egyptian income groups in 2019/2020. (Eldin and Taher, 2020). While The World Bank considers a reasonable price-to-income-ratio to be generally from 3 to 6 (Adabre and Chan, 2019), UN-Habitat’s report on “Egypt housing profile” says a ratio of 5-7 is affordable in the Egyptian-like contexts, which means between 5 and 7 times the annual income. Informal units had an average ratio of 3.8–7.7, the ratio was 9.4 in subsidized units, and 25.8 in non-subsidized private sector units, with only informal units being affordable (Sims and Fattah, 2016).
3 Results
The following sections provide comprehensive construction expenses for EH’s alternative walls, hybrid roof, and DPLM components towards its ultimate cost estimate.
3.1 Reinforced ICSEB walls costs
3.1.1 Cost of bricks
To produce 800 bricks, four non-skilled laborers (trained for 2 days) and one engineer were needed daily (Supplementary Table S4). Labor costed 850 E£, while materials costed 290 E£ (540 E£ for high-strength bricks, hereafter HS) with 74.6% accounting for the labor and 25.4% for the soil and cement, including their transportation (Figure 7). After considering maintenance, water, electricity, tools, and other expenses, the total standard bricks costed 1390 E£ per day. The self-help production (i.e., without manpower, hereafter SHP) costed 540 E£ per day, representing thus a 61% saving. In general, a standard brick costs 1,74 while SHP costs 0.68 E£ based on Eq. 3.
3.1.2 Cost of soil-cement mortar
CSEB mortar is also made from soil (for detailed expenses see Supplementary Table S5). A 10 m2 half-brick (12.5 cm) wall required 70 L of dry soil-cement, which included 60 L soil, 9 L cement (12.5 kg), and 0.5 L of white glue for adhesive. A more expensive option is pure white glue. Water is added to form a soft paste. 1 m2 costed 2.9 E£ (7 L), compared to 38 E£ for conventional mortar (25 L), representing a 92.4% saving. The former required 1.25 kg of cement per m2 while the latter required 7.5 kg, saving 83% by weight. The unit rate of 1 L soil-cement mortar is 0.41 E£ based on Eq. 3.
where 1 m2 wall requires 7 L dry mortar.
3.1.3 Cost of the overall ICSEB masonry without reinforcement
1 m2 wall needed 54 bricks and 7 L (dry) of soil-cement mortar (Table 1); a mason and helper built 10 m2 per day. 12.5 cm wall without finishing costed 137 E£ and 79.6 E£ (SHP) per m2; 25 cm wall costed double these values. Acrylic coating per 1 m2 increased the cost of a 1 m2 wall to be 166 E£ and 108.6 E£ (SHP) for a 12.5 cm wall and 303 E£ and 188.2 E£ (SHP) for a 25 cm wall. Without reinforcement, materials and labor accounted for 70% and 30% of the total cost, respectively (Figure 7). In the case of SHP brick walls, the breakdown is 54% and 46%. These percentages are comparable to those in Sri Lanka’s feasibility study (Davis and Maïni, 2017). When using SHP CSEB instead of labor produced, a saving of 34.5% (12.5 cm wall) and 37.9% (25 cm wall) was found, compared to 70% saving in Sudan back in 2001 (Adam and Agib, 2001).
3.1.4 Cost of RC microcolumns and RC brick beam
141 holes were reinforced according to the design. Rebar, stirrups, plain concrete 1:2:1 (cement: sand: gravel), and epoxy mortar (to secure the rebars in the foundation) were used (Table 1). The holes needed 1 m3 of plain concrete. Three workers anchored the rebar into the foundation, 7 workers cut and fixed rebars and stirrups, and 6 workers and 6 helpers poured the concrete. After adding the overall labor cost (4,600 E£), the microcolumns costed 12,486 E£ (without the bricks, which are considered in the masonry). The cost per microcolumn was 88.55 E£, with materials accounting for 63.2% and labor for 36.8% (Figure 7). The brick beam course was filled with 0.4 m3 concrete (1:2:1) costing 136.5 E£, and 47.5 kg stirrups costing 711 E£ (Table 1). One worker per beam was needed to cut, shape, and fix the stirrups, while 2 workers and 2 helpers poured the plain concrete, costing 1,050 E£. The beam costed 1,897.5 E£ (without bricks), with materials accounting for 55.3%, labor 44.7% (Figure 7) and a rate of 13.18 E£ per m long.
3.1.5 Total cost of walling system and unit rate
EH has a masonry area of 167 m2, including 92 m2 external, 52 m2 internal, 14.5 m2 external HS and 8.5 m2 internal HS (first 3 courses and 2 beam courses). When masonry, microcolumns, brick beams, finishing, and first course cement mortar expenses were added (Table 2), the overall cost was 59,201.4 E£ and 43,630 E£ (SHP), or 354 and 261 (SHP) per m2 wall. The SHP-brick saved 26.3% in walls.
3.2 Jack arches and funicular shells roofing cost
3.2.1 Cost of a jack arch and funicular shell roof without the RC beams
350 fired bricks were needed to build a 1 m × 4 m2 jack arch, as well as 166 L of dry cement mortar (1:4) for the mortar and plastering the vault from the outside, as shown in Table 3. The materials per m2 costed 124.25 E£ and the labor, which included two masons and two helpers, costed 200 E£ per m2. When the average tie rod expense and the average formwork wood expense were considered, the material costs were 139.85 E£ per m2, representing 41%, while the labor costed 201.56 E£, representing 59%. The 15 jack arches (42.73 m2) costed 14,588 E£, i.e., 341.4 E£ per m2. A 2 m × 2 m fired-brick funicular shell required 470 bricks and 100 L of dry rich cement slurry mortar (1:2), as well as 66 L of cement mortar (1:4) for outside plastering. When the shell was built using fired-bricks, broken marble and granite or broken ceramic tiles, or limestone tiles, the materials per m2 costed 166.5 E£, 134.25 E£, and 184.25 E£, respectively, where the second and third types had the same material cost. The labor, which included two masons and two helpers per shell, costed 200 E£ per m2 without the formwork labor cost. The formwork was completed for each funicular shell in the manner described in the construction process; two workers were required per funicular shell, at a cost of 300 E£ (75 E£/m2). When the average formwork wood cost per 1 m2 was considered, the total material costs became 171.2 E£, 138.95 E£, 188.95 E£, representing 38.4%, 33.6%, and 40.7%, respectively, while total labor costed 275 E£, representing 61.6%, 66.4%, and 59.3% for fired-bricks, broken marble and granite or broken ceramic tiles, and limestone tile shells, respectively.
3.2.2 Cost of hybrid roofs including the RC beams
The volume of the RC beams is 6.3 m3, accounting for 25.3 m2 of the roof. Their 66 m2 sides needed plastering and painting (Table 3). The cost of alternative roofing system ranged between 53,806–55,147 E£, including beams. Despite the beam cost (18,610 E£) being higher than the masonry work (14,576 E£) of the jack arch, this system was the cheapest, 558.32 E£/m2, with the beam representing 56% of its cost (Figure 8). It was followed by broken marble, granite and ceramic shells, 582.6 E£ (beam 46%) then fired bricks, 607.1 E£ (beam 44%), and finally limestone, 620.6 E£ (beam 43%). The difference between the jack arch system and the limestone shell system is 10%. A removable PVC formwork would have reduced the cost of the funicular shell (more cost-effective for mass construction). The jack arch was 23.6% cheaper than the fired brick shell without beams (341.4 E£ vs. 446.25 E£, but just 8% cheaper when they are included.
3.3 DPLM openings and pergolas cost
One thousand and sixty palm midribs were used to make 28.5 m2 of doors, shutters, and pergolas. One craftsman can make one door (1.8 m2), two windows (2 m2), or 2 m2 pergolas daily (Table 4). DPLM for windows and pergolas costed 50 E£ and the craftsman’s fee was 100 E£, for a total of 150 E£ per m2 representing 33.3% and 66.7%, respectively (without the common items such as the wooden frames, accessories, and hinges, which will be added in a later section). The door materials’ cost, including the polycarbonate sheets and the two vertical wooden strips, was 180 E£, while the craftsman costed 200 E£. In total, the door costed 380 E£, or 211 E£ per m2, representing 47.4% for and 52.6% respectively because of the polycarbonate and wood. Table 4 illustrates also the entire cost of the DPLM components.
4 Discussion
4.1 Construction cost savings of the EH
Based on the previous sections, Figure 9A provides the saving rates in each EH component assuming the conventional house cost is 100% (a detailed comparison of the costs of EH and a comparable conventional house is provided in Supplementary Table S6). The conventional walling system includes RC columns, fired brick masonry, plastering, and painting while the alternative walling system features ICSEB walls with RC components (microcolumns and RC brick beams) and 3 layers of water-based acrylic coating. The conventional roof is a 20 cm thick RC flat slab, whereas the alternative roofing consists of 15 fired brick jack arches and six funicular shells, all assumed of fired bricks as suggested by HBRC to comply with ECP 204–2005. DPLM and polycarbonate sheets replaced wooden paneled doors (whitewood slats covered on both sides with plywood sheets), DPLM latticework shutters replaced Egyptian sheesh (Venetian shutters with horizontal spruce slats), and DPLM pergolas replaced spruce pergolas.
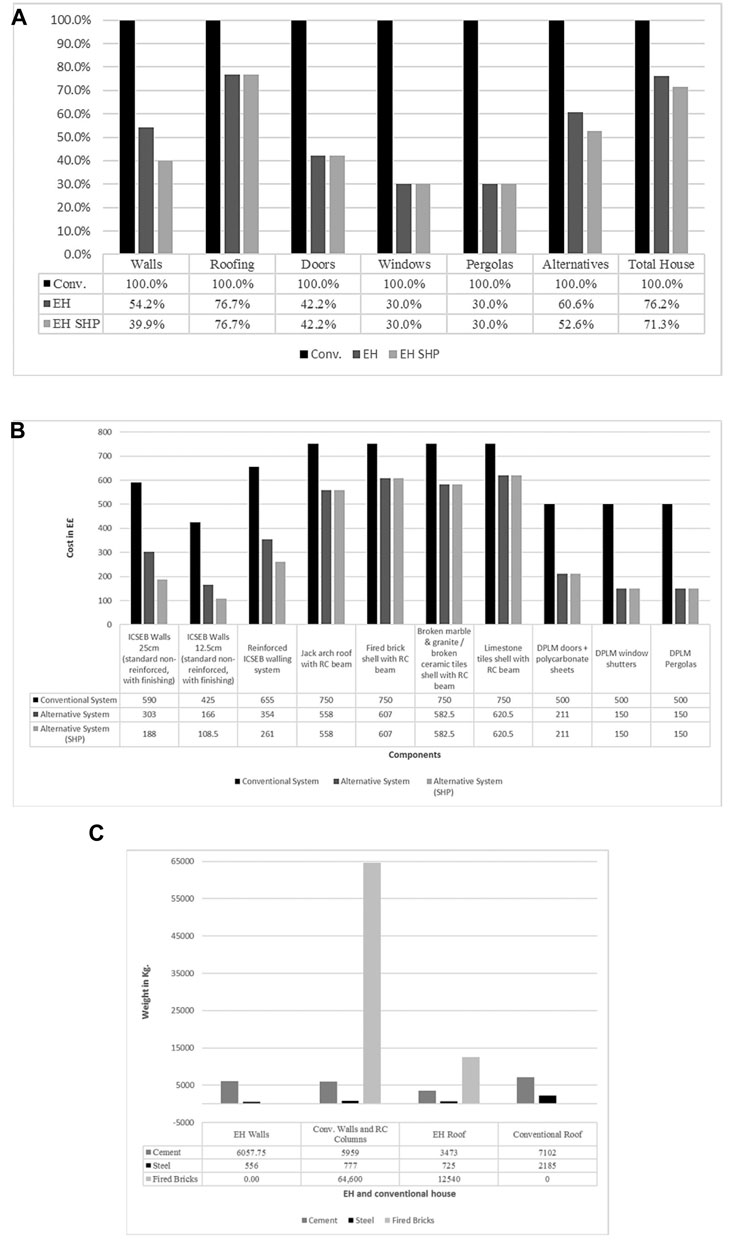
FIGURE 9. Savings in the alternative components: (A) saving rates of standard EH and with self-help produced bricks compared to a conventional house, (B) unit rates comparison between CH, EH and EH SHP, (C) comparison of utilized quantities of materials in alternative and conventional technologies by weight.
While conventional walling costed 109,297 E£, the alternative costed 59,201.5 E£ with labor-produced bricks and 43,630 E£ with SHP bricks, saving 46% and 60%. Despite the findings of Jarkas (2016) that a slab supported by two-way beams negatively affects labor productivity, time and cost compared to flat roofs, the EH roofing saved 23.3% in average. The hybrid alternative roofing costed 54,671 E£, with 50% accounting for the plastered and painted RC beams, compared to 71,250 E£ for the flat slab finished roof. The volume of the reinforced concrete in the hybrid roof was 6.3 m3, compared to 19 m3 in the flat slab, resulting in a 67% reduction. This value is consistent with the findings of Keswani (1997) and CSIR and BMTPC (2021), which mentioned savings in steel between 60% and 75%. Four single and two sliding doors made of DPLM and polycarbonate costed 3,481.5 E£ instead of 8,250 E£ for wooden paneled doors, a 58% reduction. The three DPLM window shutters costed 600 E£ instead of the 2000 E£ for the sheesh, signifying a 70% reduction. A total area of 8 m2 DPLM pergolas costed 1200 E£ instead of 4,000 E£ for spruce, saving also 70%. The costs of alternative systems combined were 117,954 E£ and 102,382.5 E£ (SHP) versus 194,797 E£ for conventional systems, representing a 39.5% and 47.5% saving respectively. After considering the costs of the common components in both systems (127,765 E£), such as plain and RC foundations, plain concrete beneath flooring, flooring tiles, electrical and plumbing installations, ceramic tiling of the toilet walls, door and window frames, glass panels, accessories, and entrance stairs, Ecofordable House’s total cost became 245,719 E£ and 230,147.5 E£ (SHP), while its conventional counterpart costs 322,562 E£, saving 23.8% and 28.7%. Although the savings rate for the entire house is lower than for the alternative systems alone, the cost difference is substantial when constructing multiple houses, given their lower environmental impact. In addition to summarizing the unit rates and final savings calculated in previous sections, Table 5; Figure 9B illustrate the breakdowns for each technology separately. Standard non-reinforced 25 cm ICSEB walls saved 48.7% and 68% (SHP). A 12.5 cm ICSEB wall saved 61% and 74.5% (SPH). The savings were considerably aided by the finishing.
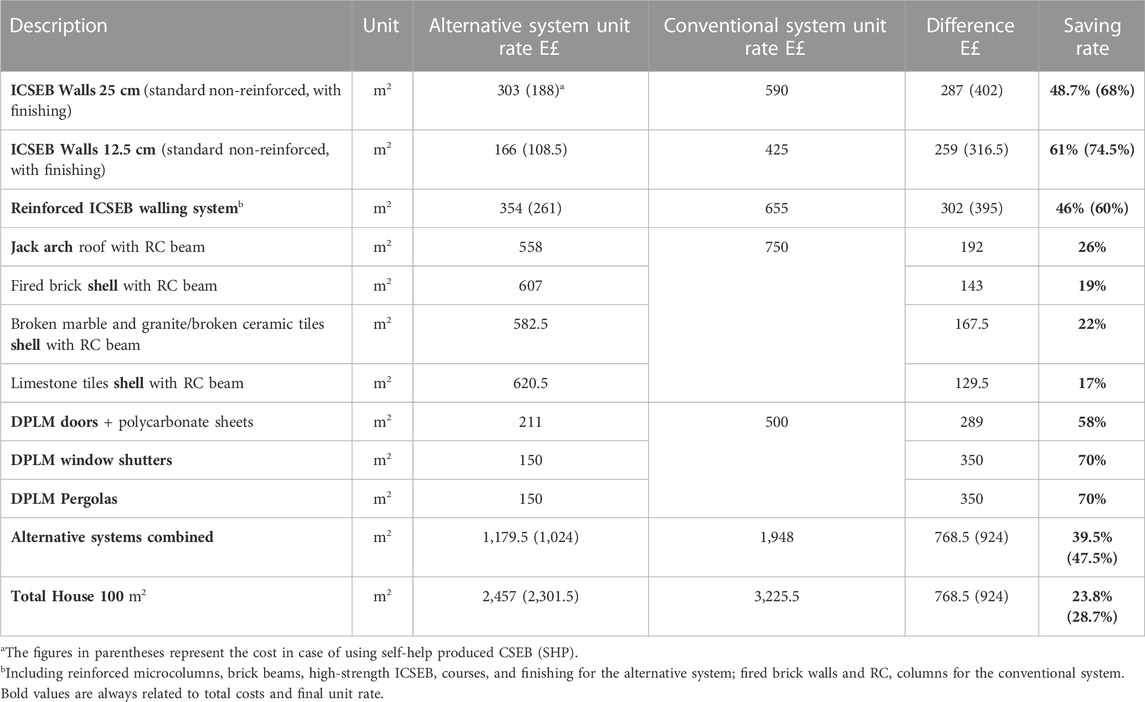
TABLE 5. Summary of cost comparisons and saving rates of the ecofordable house’s alternative technologies.
This study found that ICSEB is more beneficial than in Uganda. Nuwagaba (2020) estimated that non-reinforced CSEB walls in an Ugandan house (digital model) is 18% cheaper than fired bricks. Furthermore, compared to a 2001 study in Kenya, which estimated CSEB walls cost 20%–70% of concrete block walls (Adam and Agib, 2001), this study provides more accurate figures. The feasibility study by Davis and Maïni (2017) in Sri Lanka suggested that 14-cm-thick CSEB walls are 26.2%–29.9% cheaper than 19-cm-thick fired brick walls. Comparing different wall thicknesses was challenging because it was unclear if they were both loadbearing. Recent research by Singh et al. (2022) in India mentioned a 15%–20% savings in finished CSEB masonry compared to fired bricks without providing detailed cost analyses. The feasibility study by Kumar et al. (2018) in the US Gulf Coast found similar EH savings rates, where reinforced ICSEB walls were 42% cheaper than fired brick walls. In Brazil, Di Gregorio et al. (2020) estimated that a reinforced ICSEB system saves 23% compared to conventional fired bricks and RC columns (Di Gregorio et al., 2020), while EH saved the double, 46% and 60% (SHP). The same study by Kumar et al. (2018) suggested that, when adding common expenses, a reinforced ICSEB house is 27% cheaper than fired-bricks’, close to EH saving rate (23.8% and 28.7 SHP). Finished jack arch roof saves 26%, while funicular shells save between 17% and 22%, according to the material. These figures are not consistent with Rao and Raina (2005) suggestions, which mentioned that jack arch and funicular shell roofing saves 15% and 30% compared to RC slab.
4.2 Quantities of materials with high environmental impact
Figure 9C compares cement, steel, and fired brick contents in alternative and conventional systems (detailed quantities are available in Supplementary Table S7). The weight of cement, including 10% CSEB stabilization, was 9,530.75 kg vs. 13,061 kg; steel was 1,281 kg vs. 3,962 kg; and fired bricks were 6.6 m3 vs. 34 m3. Quantity reductions in the proposed house were 27% in cement, 68% in steel, and 80% in fired bricks, even when assuming only fired bricks were used in the hybrid roof (ECP 204–2005). With an average fired brick density of 1,900 kg/m3, the total weight of these materials combined is 23,352 kg instead of 81,623 kg in conventional systems, utilizing only 28.6% by weight and saving thus 71.4% and implying considerably lower embodied energy. More research is needed to investigate this topic.
4.3 EH affordability
As per the latest CAPMAS report of 2019/2020 (Eldin and Taher, 2020), the average annual household income in Egypt ranged between 34,200 E£ and 95,400 E£ in a scale of 10 income brackets, as shown in Table 6. Without taking into account the land price and profit, i.e., government-built houses, the price-to-income-ratio of the 100 m2 Ecofordable House with SHP bricks (EH SPH) ranged between 2.4 and 6.7, indicating that it would be affordable for all income brackets, and even considered cheap for seven of them as the ratio was below the UN-Habitat affordability range (5-7 in contexts similar to Egypt, (Sims and Fattah, 2016). The standard Ecofordable House ratio was between 2.6 and 7.2, making it cheap for six income brackets, affordable for three, and expensive, but on the borderline of affordability, for the lowest bracket. The conventional house (CH) ratio, on the other hand, ranged between 3.4 and 9.4, making it cheap for the highest income bracket only, affordable for six, and expensive for the lowest three brackets for which government-built housing is significant. The affordability will improve in case of smaller house area.
5 Conclusion
A rural prototype house (Ecofordable House, EH) that combines improved vernacular technologies was built in the desert area of western Giza, Egypt, in response to increasing housing prices and the prohibitive cost of materials in the construction sector. Based on a review of the literature, interlocking compressed stabilized earth brick walls (partially reinforced), jack arch and funicular shell roofs, and date palm midribs were selected as alternatives to minimize the use of steel, fired bricks, cement, and imported wood. The study aimed to evaluate their potential construction cost savings and affordability using real data rather than feasibility studies or digital and economic models. Although the revival of the employed solutions is continuously advocated, the literature focuses mostly on structural and environmental performance, with little information on economic advantages. A research gap on their construction cost effectiveness and affordability was found.
The paper began by examining relevant technologies and discussing the reasons for selecting EH solutions before delving into economic data available in the literature. Then, it presented a description of the house and detailed the construction process of alternate components. It provided original information on construction steps, materials, and labor costs collected during the construction process, calculated overall costs, and compared them to conventional methods. Finally, price-to-income ratios were calculated to estimate the affordability of the house for various income brackets in Egypt.
Compared to fired brick walls and RC columns combined, the alternative walling system (partially reinforced ICSEB varnished with acrylic) reduced wall costs by nearly half (46%), with savings increasing to 60% when self-help produced ICSEB (SHP) was used. The cost of jack arch and funicular shell hybrid roofs was 23.3% less than that of 20 cm RC flat slabs. Midribs of date palm leaves (DPLM) and polycarbonate doors were 58% less expensive than wooden paneled doors, and DPLM shutters and pergolas were 70% less expensive than whitewood (spruce). Alternative technologies saved 39.5%, while using SHP bricks saved 47.5%. EH saved 23.8% after including shared components, and 28.7% when using SHP bricks. Furthermore, the suggested technologies saved 71.4% of the weight of cement, steel, and fired bricks, implying a lower environmental impact. In Egypt, EH with SHP bricks would be affordable in government-built houses for all 10 income brackets (cheap for seven). The standard EH would be affordable for 9 brackets (cheap for 3) and expensive for the lowest bracket (on the borderline of affordability). The conventional house is affordable for 7 brackets (cheap for the highest bracket only), and expensive for the lowest 3, for which public housing is being built.
Although the current study was undertaken in Egypt and is a case study, it is applicable to a broader geographical region because the utilized technologies have attracted interest as sustainable alternatives in recent years throughout Europe, Africa, the MENA region, and Asia. In addition, the findings could serve as a resource for academics, practitioners, and contractors that advocate or doubt the economic benefits of enhanced vernacular technologies. An important limitation of this study is that it only examines the initial building costs. Additional research utilizing life cycle costing (LCC) and life cycle assessment (LCA) is necessary to thoroughly analyze the economic performance and environmental impact of the Ecofordable House.
Data availability statement
The original contributions presented in the study are included in the article/Supplementary Material, further inquiries can be directed to the corresponding author.
Author contributions
NA: Project initiator, house design, site supervision, construction data documentation, coordination with procurement department, handling raw material, data analysis, scheduling, BOQ, writing, photographing, formatting, referencing. IA: Handling raw material, data analysis, scheduling, BOQ, photographing, formatting, referencing.
Funding
The “Ecofordable House” was funded by Dr. Nawal El-Degwi, MSA University head of the board of trustees.
Conflict of interest
The authors declare that the research was conducted in the absence of any commercial or financial relationships that could be construed as a potential conflict of interest.
Publisher’s note
All claims expressed in this article are solely those of the authors and do not necessarily represent those of their affiliated organizations, or those of the publisher, the editors and the reviewers. Any product that may be evaluated in this article, or claim that may be made by its manufacturer, is not guaranteed or endorsed by the publisher.
Supplementary material
The Supplementary Material for this article can be found online at: https://www.frontiersin.org/articles/10.3389/fbuil.2023.1058782/full#supplementary-material
References
Abdel Gelil, M. N. (2006). A new mashrabiyya for contemporary Cairo: Integrating traditional latticework from Islamic and Japanese cultures. J. Asian Archit. Build. Eng. 5 (1), 37–44. doi:10.3130/jaabe.5.37
Adabre, M. A., and Chan, A. P. C. (2019). The ends required to justify the means for sustainable affordable housing: A review on critical success criteria. Sustain. Dev. 27 (4), sd.1919–794. doi:10.1002/sd.1919
Adam, E. A., and Agib, A. R. A. (2001). Compressed stabilised earth block manufacture in Sudan. Paris, France: Division for Educational Policies and Strategies, UNESCO.
Adam, E. A., and Agib, A. R. A. (2002). Roofing systems in Sudan. Paris, France: Division for Educational Policies and Strategies, UNESCO.
Adamovic, M. (2023). Property prices. Available at: https://www.numbeo.com/property-investment/ (Retrieved January 6, 2023).
Agoudjila, B., Benchabane, A., Boudenne, A., Ibos, L., and Fois, M. (2011). Renewable materials to reduce building heat loss: Characterization of date palm wood. Energy Build. 43 (2), 491–497. doi:10.1016/j.enbuild.2010.10.014
Alex, D. (2018). Recognition of a heritage in danger: Rammed-Earth architecture in lyon city, France. IOP Conf. Ser. Earth Environ. Sci. 143, 012054. doi:10.1088/1755-1315/143/1/012054
Al-Jabri, K. S., Hago, A. W., Al-Nuaimi, A. S., and Al-Saidy, A. H. (2005). Concrete blocks for thermal insulation in hot climate. Cem. Concr. Res. 35 (8), 1472–1479. doi:10.1016/j.cemconres.2004.08.018
Al-Oqla, F. M., Mohd Sapuan, S., Mohamad Ridzwan, I., and Nuraini Abdul, A. (2015). Selecting natural fibers for bio-based materials with conflicting criteria. Am. J. Appl. Sci. 12 (1), 64–71. doi:10.3844/ajassp.2015.64.71
BDC 13 (1981). Code of practice for construction of jack-arch type of built-up floor or roof. New Delhi: Indian Standards Institution.
Bogas, J. A., Silva, M., and Gomes, M. G. (2019). Unstabilized and stabilized compressed Earth blocks with partial incorporation of recycled aggregates. Int. J. Archit. Herit. 13 (4), 569–584. doi:10.1080/15583058.2018.1442891
Bradley, R. A., and Gohnert, M. (2018). Compressed stabilized earth block shell housing: Performance considerations. Pract. Periodical Struct. Des. Constr. 23 (3), 04018009. doi:10.1061/(ASCE)SC.1943-5576.0000373
Bradley, R., Gohnert, M., and Fitchett, A. (2018). Guidelines to mitigate cracking in compressed stabilized earth brick shells. J. Perform. Constr. Facil. 32 (3), 1–19. doi:10.1061/(ASCE)CF.1943-5509.0001170
Burroughs, S. (2010). Recommendations for the selection, stabilization, and compaction of soil for rammed Earth wall construction. J. Green Build. 5 (1), 101–114. doi:10.3992/jgb.5.1.101
Chen, J., Wang, Y., Shi, Q., Peng, X., and Zheng, J. (2021). An international comparison analysis of CO 2 emissions in the construction industry. Sustain. Dev. 29 (4), 754–767. doi:10.1002/sd.2172
Cowan, H. J. (1977). A history of masonry and concrete domes in building construction. Build. Environ. 12 (1), 1–24. doi:10.1016/0360-1323(77)90002-6
Cowan, H. J. (1981). Some observations on the structural design of masonry arches and domes before the age of structural mechanics. Archit. Sci. Rev. 24 (4), 98–102. doi:10.1080/00038628.1981.9696481
Cristea, A., Hummels, D., Puzzello, L., and Avetisyan, M. (2013). Trade and the greenhouse gas emissions from international freight transport. J. Environ. Econ. Manag. 65 (1), 153–173. doi:10.1016/j.jeem.2012.06.002
CSIRBMTPC (2021). Compendium of building technologies. India: Ministry of Science and Technology-Ministry of Housing & Urban Affairs.
Daoud, A. O., Othman, A. A. E., Ebohon, O. J., and Bayyati, A. (2021). Quantifying materials waste in the Egyptian construction industry: A critical analysis of rates and factors. Ain Shams Eng. J. 12 (4), 4275–4289. doi:10.1016/j.asej.2021.02.039
Davis, L. K., and Maïni, S. (2017). Feasibility report for compressed stabilised earth block (CSEB) production and use in the north and east of Sri Lanka. Luxembourg: Publications Office of the European Union.
Di Gregorio, L., Guimarães, G., Tenório, M., Lima, D., Haddad, A., Danziger, F., et al. (2020). The potential of CEB reinforced masonry technology for (Re)construction in the context of disasters. Materials 13 (17), 3861. doi:10.3390/ma13173861
Diodato, M., Macchioni, N., Brunetti, M., Pizzo, B., Nocetti, M., Burato, P., et al. (2015). Understanding Spanish timber jack arch floors: Examples of assessment and conservation issues. Int. J. Archit. Herit. 9 (6), 641–654. doi:10.1080/15583058.2015.1041193
Duarte, G., Brown, N., Memari, A., and Duarte, J. P. (2021). Learning from historical structures under compression for concrete 3D printing construction. J. Build. Eng. 43, 103009. doi:10.1016/j.jobe.2021.103009
Edris, W., Matalkah, F., Rbabah, B., AbuSbaih, A., and Hailat, R. (2021). Characteristics of hollow compressed earth block stabilized using cement, lime, and sodium silicate. Civ. Environ. Eng. 17 (1), 200–208. doi:10.2478/cee-2021-0021
Egenti, C., and Khatib, J. M. (2016). “13 - sustainability of compressed Earth as a construction material,” in Sustainability of construction materials. Editor J. M. Khatib (Sawston, United Kingdom: Woodhead Publishing), 309–341.
Eldin, A. S., and Taher, K. M. (2020). The most important indicators of income, expenditure and consumption research 2019-2020. Retrieved from Cairo: https://www.capmas.gov.eg/Pages/StaticPages.aspx?page_id=7183.
El-Mously, H., and Darwish, E. A. (2020). “Date palm byproducts: History of utilization and technical heritage,” in Date palm fiber composites: Processing, properties and applications. Editors M. Midani, N. Saba, and O. Y. Alothman (Singapore: Springer Singapore), 3–71.
El-Mously, H. (2018). Innovating green products as a mean to alleviate poverty in Upper Egypt. Ain Shams Eng. J. 9 (4), 2039–2056. doi:10.1016/j.asej.2017.02.001
El-Mously, H. (2020). “Date palm fiber composites as wood substitutes,” in Date palm fiber composites: Processing, properties and applications. Editors M. Midani, N. Saba, and O. Alothman (Singapore: Springer), 357–386.
Elseify, L. A., Midani, M., Shihata, L. A., and El-Mously, H. (2019). Review on cellulosic fibers extracted from date palms (Phoenix Dactylifera L.) and their applications. Cellulose 26 (4), 2209–2232. doi:10.1007/s10570-019-02259-6
El-Sharbasy, S. (2018). Illustrated Guide in the cultivation and service of date palm. Egypt: FAO Publications.
Eslami, A., Ronagh, H. R., Mahini, S. S., and Morshed, R. (2012). Experimental investigation and nonlinear FE analysis of historical masonry buildings – a case study. Constr. Build. Mater 35, 251–260. doi:10.1016/j.conbuildmat.2012.04.002
FAO (2020a). Forestry production and trade. Available at: https://www.fao.org/faostat/en/#data/FO.
FAO (2020b). Crops and livestock products. Available at: https://www.fao.org/faostat/en/#data/QCL.
Fernandes, J., Peixoto, M., Mateus, R., and Gervásio, H. (2019). Life cycle analysis of environmental impacts of earthen materials in the Portuguese context: Rammed Earth and compressed Earth blocks. J. Clean. Prod. 241, 118286. doi:10.1016/j.jclepro.2019.118286
Garcia-Castillo, E., Paya-Zaforteza, I., and Hospitaler, A. (2021). Analysis of the fire resistance of timber jack arch flooring systems used in historical buildings. Eng. Struct. 243, 112679. doi:10.1016/j.engstruct.2021.112679
Ghnimi, S., Umer, S., Karim, A., and Kamal-Eldin, A. (2017). Date fruit (Phoenix dactylifera L.): An underutilized food seeking industrial valorization. NFS J. 6, 1–10. doi:10.1016/j.nfs.2016.12.001
González-López, J. R., Juárez-Alvarado, C. A., Ayub-Francis, B., and Mendoza-Rangel, J. M. (2018). Compaction effect on the compressive strength and durability of stabilized Earth blocks. Constr. Build. Mater 163, 179–188. doi:10.1016/j.conbuildmat.2017.12.074
Hanafi, W. H. H. (2021). Compressed stabilized Earth block: Environmentally sustainable alternative for villages housing. J. Eng. Appl. Sci. 68 (1), 20. doi:10.1186/s44147-021-00017-9
Hany, E., Fouad, N., Abdel-Wahab, M., and Sadek, E. (2021). Investigating the mechanical and thermal properties of compressed Earth bricks made by eco-friendly stabilization materials as partial or full replacement of cement. Constr. Build. Mater 281, 122535. doi:10.1016/j.conbuildmat.2021.122535
Idriss, E., Tome, S., Rolande Aurelie, T. K., Nana, A., Nemaleu, J. G. D., Judicaёl, C., et al. (2022). Engineering and structural properties of compressed Earth blocks (CEB) stabilized with a calcined clay-based alkali-activated binder. Innov. Infrastruct. Solutions 7 (2), 157. doi:10.1007/s41062-022-00760-9
Jain, A. (2000). Slum rehabilitation and housing the poor. Soc. Change 30 (1-2), 139–152. doi:10.1177/004908570003000210
Jannasch, E. (2017). Fit forms and free forms of the masonry dome. Nexus Netw. J. 19 (3), 599–617. doi:10.1007/s00004-016-0319-3
Jarkas, A. M. (2016). Effect of buildability on labor productivity: A practical quantification approach. J. Constr. Eng. Manage. 142 (2), 06015002. doi:10.1061/(ASCE)CO.1943-7862.0001062
Kasinikota, P., and Tripura, D. D. (2021). Evaluation of compressed stabilized Earth block properties using crushed brick waste. Constr. Build. Mater 280, 122520. doi:10.1016/j.conbuildmat.2021.122520
Keswani, K. (1997). The contribution of building centres to low-cost housing in India. Build. Res. Inf. 25 (1), 50–64. doi:10.1080/096132197370606
Khan, A., Arif, S., Nadeem, O., and Anwer, A. (2013). Jack arch: The back-bone of British colonial residential buildings in India. Pak. J. Sci. 65 (3), 352–356.
Kharrufa, S. (2007). Reduction of building waste in Baghdad Iraq. Build. Environ. 42 (5), 2053–2061. doi:10.1016/j.buildenv.2006.03.011
Kulshreshtha, Y., Mota, N. J. A., Jagadish, K. S., Bredenoord, J., Vardon, P. J., van Loosdrecht, M. C. M., et al. (2020). The potential and current status of earthen material for low-cost housing in rural India. Constr. Build. Mater 247, 118615. doi:10.1016/j.conbuildmat.2020.118615
Kumanayake, R., and Luo, H. (2018). Life cycle carbon emission assessment of a multi-purpose University building: A case study of Sri Lanka. Front. Eng. Manag. 5 (3)–393. doi:10.15302/j-fem-2018055
Kumar, V. D. S., and Maheswari, A. U. (2019). Analytical study of funicular and flat slabs. Int. J. Sci. Technol. Eng. 5 (8), 46–50. http://www.ijste.org/articles/IJSTEV5I8014.pdf.
Kumar, N., Barbato, M., and Holton, R. (2018). Feasibility study of affordable earth masonry housing in the U.S. Gulf Coast region. J. Archit. Eng. 24 (2), 04018009. doi:10.1061/(ASCE)AE.1943-5568.0000311
Leo Samuel, D. G., Dharmasastha, K., Shiva Nagendra, S. M., and Maiya, M. P. (2017). Thermal comfort in traditional buildings composed of local and modern construction materials. Int. J. Sustain. Built Environ. 6 (2), 463–475. doi:10.1016/j.ijsbe.2017.08.001
Leung, C. K. Y., and Tang, E. C. H. (2023). The dynamics of the house price-to-income ratio: Theory and evidence. Contemp. Econ. Policy 41 (1), 61–78. doi:10.1111/coep.12538
Li, S., Wu, H., and Ding, Z. (2018). Identifying sustainable wood sources for the construction industry: A case study. Sustainability 10 (1), 139. doi:10.3390/su10010139
Ma, H., Ma, Q., and Gaire, P. (2019). Development and mechanical evaluation of a new interlocking Earth masonry block. Adv. Struct. Eng. 23 (2), 234–247. doi:10.1177/1369433219868931
Mahdad, M. H., Benidir, A., and Brara, A. (2021). Experimental assessment of mechanical behavior of a compressed stabilized Earth blocks (CSEB) and walls. J. Mater. Eng. Struct. 8, 95–110.
Maheri, M. R., and Rahmani, H. (2003). Static and seismic design of one-way and two-way jack arch masonry slabs. Eng. Struct. 25 (13), 1639–1654. doi:10.1016/S0141-0296(03)00143-3
Maheri, M. R., Pourfallah, S., and Azarm, R. (2012). Seismic retrofitting methods for the jack arch masonry slabs. Eng. Struct. 36, 49–60. doi:10.1016/j.engstruct.2011.11.018
Marsh, A. T. M., and Kulshreshtha, Y. (2021). The state of earthen housing worldwide: How development affects attitudes and adoption. Build. Res. Inf. 50, 485–501. doi:10.1080/09613218.2021.1953369
Merriam-Webster (2023a). funicular. Merriam-Webster.com. Retrieved from: https://www.merriam-webster.com/dictionary/funicular.
Merriam-Webster.(2023b). Jack arch. Merriam-Webster.com. Retrieved from: https://www.merriam-webster.com/dictionary/jack%20arch
Merriam-Webster.(2023c). jack. Merriam-Webster.com. Retrieved from: https://www.merriam-webster.com/dictionary/jack#h1
Metwally, S. A., and Hamza, Y. T. A. (2017). The agricultural investments role in the progress of some small projects in Damietta. Jornal Agric. Econ. Soc. Sci. 8 (2), 105–112. doi:10.21608/jaess.2017.36453
M. Midani, N. Saba, and O. Y. Alothman (Editors) (2020). Date palm fiber composites (Singapore: Springer).
Moghayedi, A., Awuzie, B., Omotayo, T., Le Jeune, K., and Massyn, M. (2022). Appraising the nexus between influencers and sustainability-oriented innovation adoption in affordable housing projects. Sustain. Dev. 30, 1117–1134. doi:10.1002/sd.2306
Motra, G. B., Sah, B. K., and Jha, P. (2021). Structural condition assessment and retrofitting of Shital Niwas building (presidential palace). Prog. Disaster Sci. 10, 100174. doi:10.1016/j.pdisas.2021.100174
Moussa, H. S., Nshimiyimana, P., Hema, C., Ousmane, Z., Messan, A., and Courard, L. (2019). Comparative study of thermal comfort induced from masonry made of stabilized compressed earth block vs conventional cementitious material. J. Minerals Mater. Charact. Eng. 07, 385–403. doi:10.4236/jmmce.2019.76026
Mukhtar, Y. A. (1980). Roofs in northern Sudan—their thermal performance and methods of improvement. Archit. Sci. Rev. 23 (2), 51–54. doi:10.1080/00038628.1980.9696441
Murmu, A. L., and Patel, A. (2018). Towards sustainable bricks production: An overview. Constr. Build. Mater 165, 112–125. doi:10.1016/j.conbuildmat.2018.01.038
Nadia, B., Fatma, K., and Nasser, C. (2023). Mechanical, thermal and durability investigation of compressed Earth bricks stabilized with wood biomass ash. Constr. Build. Mater. 364, 129874. doi:10.1016/j.conbuildmat.2022.129874
Nuwagaba, H. M. (2020). Opportunities for affordable construction in Uganda using locally available materials. Massachusetts, USA: Master of Engineering in Civil And Environmental Engineering, Massachusetts Institute of Technology.
Omar Sore, S., Messan, A., Prud'homme, E., Escadeillas, G., and Tsobnang, F. (2018). Stabilization of compressed Earth blocks (CEBs) by geopolymer binder based on local materials from Burkina Faso. Constr. Build. Mater. 165, 333–345. doi:10.1016/j.conbuildmat.2018.01.051
Ozdemir, M. A., Kaya, E. S., Aksar, B., Seker, B., Cakir, F., Uckan, E., et al. (2017). Seismic vulnerability of masonry Jack arch slabs. Eng. Fail. Anal. 77, 146–159. doi:10.1016/j.engfailanal.2017.02.008
Öztürk, Ş., Bayraktar, A., Hökelekli, E., and Ashour, A. (2020). Nonlinear structural performance of a historical brick masonry inverted dome. Int. J. Archit. Herit. 14 (8), 1161–1179. doi:10.1080/15583058.2019.1592265
Pacheco-Torgal, F., and Jalali, S. (2012). Earth construction: Lessons from the past for future eco-efficient construction. Constr. Build. Mater 29, 512–519. doi:10.1016/j.conbuildmat.2011.10.054
Rao, A. N. V., and Raina, S. (2005). Energy independence in buildings”: Why and how? Natl. Counc. Cem. Build. Mater. 4, 8–11.
Saari, S., AbuBakar, B. H., and Surip, N. A. (2021). Factors of non-uniform properties of interlocking compressed Earth brick units. Dev. Built Environ. 5, 100042. doi:10.1016/j.dibe.2021.100042
Shabdin, M., Attari, K. A. N., and Zargaran, M. (2020). Shaking table study on the seismic performance of an Iranian traditional Un-Reinforced Masonry (URM) building. Structures 27, 424–439. doi:10.1016/j.istruc.2020.06.002
Singh, S., Chohan, J. S., Kumar, R., and Gupta, P. K. (2022). Stability of compressed Earth blocks using sugarcane bagasse ash and wheat straw. Mater. Today Proc. 51, 993–997. doi:10.1016/j.matpr.2021.07.023
Sivakumar, P., Manjunatha, K., and Harish, A. B. (2015). Experimental and FE analysis of funicular shells. Int. J. Eng. Innovative Technol. 4 (9), 178–186.
Tan, Y., Shuai, C., Shen, L., Hou, L., and Zhang, G. (2020). A study of sustainable practices in the sustainability leadership of international contractors. Sustain. Dev. 28 (4), 697–710. doi:10.1002/sd.2020
Tchouateu Kamwa, R. A., Tome, S., Chongouang, J., Eguekeng, I., Spieß, A., Fetzer, M. N. A., et al. (2022). Stabilization of compressed Earth blocks (CEB) by pozzolana based phosphate geopolymer binder: Physico-mechanical and microstructural investigations. Clean. Mater. 4, 100062. doi:10.1016/j.clema.2022.100062
Teixeira, E. R., Machado, G., Junior, P., Guarnier, C., Fernandes, J., Silva, S. M., et al. (2020). Mechanical and thermal performance characterisation of compressed earth blocks. Energies 13 (11), 2978. doi:10.3390/en13112978
Touré, P. M., Sambou, V., Faye, M., Thiam, A., Adj, M., and Azilinon, D. (2017). Mechanical and hygrothermal properties of compressed stabilized Earth bricks (CSEB). J. Build. Eng. 13, 266–271. doi:10.1016/j.jobe.2017.08.012
UNEP (2021). Global status report for buildings and construction. Nairobi: UN Environment Programme, 2021.
Un-Habitat, (2009). Interlocking stabilised soil blocks: Appropriate earth technologies in Uganda. Uganda: UN-Habitat.
Un-Habitat, (2012). Going green, A handbook of sustainable housing practices in developing countries. Nairobi: United Nations Human Settlement Programme.
Unni, A., and Anjali, G. (2022). Cost-benefit analysis of conventional and modern building materials for sustainable development of social housing. Mater. Today Proc. 51, 1649–1657. doi:10.1016/j.matpr.2021.12.113
Uzoegbo, H. C. (2016). “Dry-stack and compressed stabilised Earth-block construction,” in Nonconventional and vernacular construction materials. Editors K. A. Harries, and B. Sharma (Sawston, United Kingdom: Woodhead Publishing), 205–249.
Vafai, A., Estekanchi, H. E., and Javidruzi, M. (2005). Experimental study of prefabricated funicular shell units. Struct. Build. 158 (3), 167–174. doi:10.1680/stbu.2005.158.3.167
Venkatarama Reddy, B. V., and Jagadish, K. S. (2003). Embodied energy of common and alternative building materials and technologies. Energy Build. 35 (2), 129–137. doi:10.1016/S0378-7788(01)00141-4
Venkatarama Reddy, B. V., Leuzinger, G., and Sreeram, V. S. (2014). Low embodied energy cement stabilised rammed Earth building—a case study. Energy Build. 68, 541–546. doi:10.1016/j.enbuild.2013.09.051
Venkatarama Reddy, B. V. (2009). Sustainable materials for low carbon buildings. Int. J. Low-Carbon Technol. 4 (3), 175–181. doi:10.1093/ijlct/ctp025
Wang, T., Wang, Y., Zeng, G., Zhang, J., and Shi, D. (2021). Stability coefficient of interlocking compressed Earth block masonry under axial compression. Can. J. Civ. Eng. 48 (4), 389–398. doi:10.1139/cjce-2019-0281
Weerasinghe, A. S., and Ramachandra, T. (2020). Implications of sustainable features on life-cycle costs of green buildings. Sustain. Dev. 28 (5), 1136–1147. doi:10.1002/sd.2064
Wells, J. (1993). Appropriate building technologies: An appraisal based on case studies of building projects in Senegal and Kenya. Constr. Manag. Econ. 11 (3), 203–216. doi:10.1080/01446199300000020
Xu, A., Tresa, E., Bacchetta, M., Bellelli, F., and Monteiro, J. A. (2021). Trade and climate change, information brief N°4: Carbon content of international trade.
Yang, X., and Wang, H. (2019). Strength of hollow compressed stabilized earth-block masonry prisms. Adv. Civ. Eng. 2019, 1–8. doi:10.1155/2019/7854721
Zahrai, S. M. (2015). Experimental study of typical and retrofitted jack arch slabs in a single story 3D steel building. Int. J. Civ. Eng. 13 (3), 278–288. doi:10.22068/IJCE.13.3.278
Zaid, A., and de Wet, P. F. (2002). “Botanical and systematic description of the date palm,” in Date palm cultivation. Editor A. Zaid (Rome: Food and Agricultural Organization of the United Nations).
Keywords: alternative building technologies, cost-effectiveness, affordability, interlocking compressed stabilised earth bricks, jack arch, funicular shell, date palm midrib
Citation: Abdel Gelil Mohamed N and Abo Eldardaa Mahmoud I (2023) Cost-effectiveness and affordability evaluation of a residential prototype built with compressed earth bricks, hybrid roofs and palm midribs. Front. Built Environ. 9:1058782. doi: 10.3389/fbuil.2023.1058782
Received: 30 September 2022; Accepted: 13 March 2023;
Published: 27 March 2023.
Edited by:
Ana Mafalda Matos, University of Porto, PortugalReviewed by:
Inês Flores-Colen, University of Lisbon, PortugalMahendra Gooroochurn, University of Mauritius, Mauritius
Copyright © 2023 Abdel Gelil Mohamed and Abo Eldardaa Mahmoud. This is an open-access article distributed under the terms of the Creative Commons Attribution License (CC BY). The use, distribution or reproduction in other forums is permitted, provided the original author(s) and the copyright owner(s) are credited and that the original publication in this journal is cited, in accordance with accepted academic practice. No use, distribution or reproduction is permitted which does not comply with these terms.
*Correspondence: Nermine Abdel Gelil Mohamed, bmdlbGlsQG1zYS5lZHUuZWc=