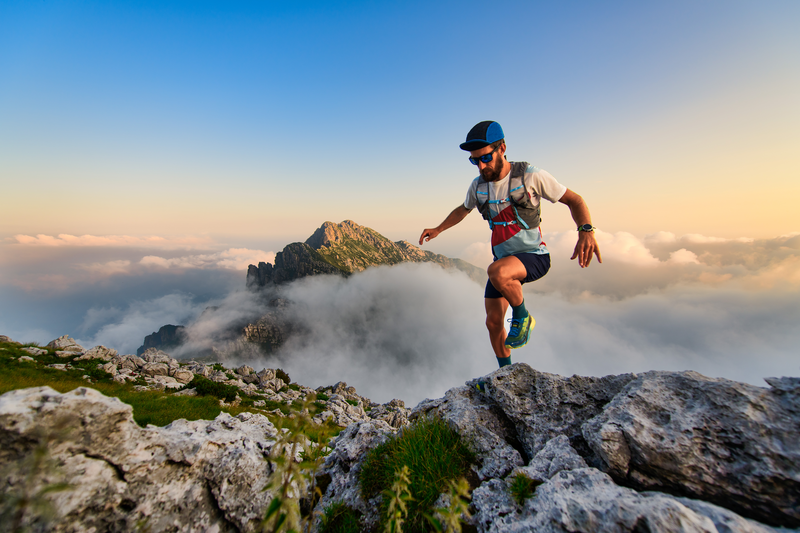
94% of researchers rate our articles as excellent or good
Learn more about the work of our research integrity team to safeguard the quality of each article we publish.
Find out more
ORIGINAL RESEARCH article
Front. Built Environ. , 02 September 2022
Sec. Indoor Environment
Volume 8 - 2022 | https://doi.org/10.3389/fbuil.2022.998872
This article is part of the Research Topic Design of Efficient and Healthy Buildings View all 6 articles
Dubai Municipality is making significant efforts to reduce the concentration of chemical substances in major buildings via Green Building Regulations & Specifications. However, it has limitations to the problem because it simply regulates the indoor air concentration of some harmful substances from building materials. The functional building materials capable of adsorbing and decomposing indoor pollutants such as Formaldehyde (HCHO) and Volatile Organic Compounds (VOCs) are gradually spreading. This paper aims to evaluate the performance of functional building materials and analyze the effect of improving the indoor air environment. As a methodology, the investigation was done to research trends and standards for functional building standards. 20 L small chamber experiment was performed for wallpaper with 0%, 5%, 7%, 10%, and 15% of the ethylene urea (C5H10N2O3), HCHO remover. The result showed standard wallpaper’s adsorption rate on the seventh day was 6.21%. The formaldehyde remover adsorption rate for 7 days was 50.43% when formaldehyde remover was added at a 5 wt% (weight percentage); 60.21% when it was added at 7 wt%; 63.45% when it was added at 10 wt%; and 73.58% when it was added at 15 wt%. The adsorption rate on the seventh day with 7 wt%, 10 wt%, and 15 wt% HCHO remover showed a 60% or more (IS O 16000-24 standard). However, wallpaper with 15 wt%, displayed the highest value, was 5.736 μg/m2, which did not satisfy the IS O 16000-24 standard (6.000 μg/m2). It was statistically proven when the amount of the HCHO remover is increased; the adsorption performance is improved in proportion to the amount added. This study will serve as primary data to prepare UAE standards for the functional building materials with adsorption and decomposition performance of harmful chemicals, moisture absorption and moisture-proof performance, and antibacterial/anti-fungal performance.
Dubai residents in the United Arab Emirates spend more than 90% of their time indoors due to hot arid desert weather (Jung and Awad, 2021a). Interest in Indoor Air Quality (IAQ) is significantly increasing in residential buildings since Sick Building Syndrome (SBS) has been highlighted in UAE (Awad and Jung, 2021a; Jung and Awad, 2021b). According to the analysis of the survey results released by Dubai Municipality in 2013, 29.8% of the respondents had experienced SBS (Jung and Al Qassimi, 2022). It was surveyed that about 7.5% of all residents had ever been to the hospital due to SBS (Arar and Jung, 2021). According to the 2019 UAE National Air Emissions Inventory Project by the Ministry of Climate Change and Environment, 93.5% of residents consider indoor air quality in their housing very important, and 42.9% of respondents were willing to invest up to 100–2,000 AED to improve indoor air quality (The Khaleej Times, 2013). It has been shown that the expert group and the public are very interested in IAQ (Nationals, 2013). Improving the indoor air environment in residential spaces is one of the most critical problems in Dubai (Al Qassimi and Jung, 2021). Factors affecting these indoor air environment problems include harmful chemicals such as formaldehyde (HCHO) and Volatile Organic Compounds (VOCs) such as Benzene (C6H6), Toluene (C7H8), Ethylbenzene (C8H10), Xylene (C8H10), and Styrene (C8H8), emitted from building materials, furniture, home appliances, and indoor household items, airborne bacteria, fungus, and viruses (Arar et al., 2021; Jung and Awad, 2021c). In addition, the causes are very diverse, such as problems with buildings and mechanical facilities, such as a decrease in the opening area due to the high-rise of a residential building, and a lack of ventilation due to high insulation and high airtightness for energy saving of buildings (Ai et al., 2015; Awad and Jung, 2021b).
The use of indoor building materials that emit harmful substances is one of the most influential factors among the various causes that affect indoor air quality deterioration and indoor air environment problems (Tham, 2016). In new buildings, the release of harmful chemicals to the human body such as HCHO and VOCs emitted from adhesives, paints, flooring, and wooden boards used as interior and finishing materials threaten human health. Research to solve and identify these hazards is actively conducted (Jiang et al., 2017).
Meanwhile, Dubai Municipality is making significant efforts to reduce the concentration of chemical substances in major buildings via Green Building Regulations & Specifications (Municipality Dubai, 2018). However, it has limitations as a fundamental solution to the problem because it simply regulates the indoor air concentration of some harmful substances generated from building materials (Steinemann et al., 2017). As one of several methods to solve this problem, functional building materials capable of adsorbing and decomposing indoor pollutants such as HCHO and VOCs are gradually spreading (Huangfu et al., 2020). Unlike eco-friendly building materials that emit fewer pollutants, functional building materials can objectively verify the specific performance inherent in building materials (Ratera and Veciana, 2012). This refers to building materials that have been proven effective in improving the indoor air environment. Functional building materials include moisture absorptive performance that can adequately control indoor humidity, adsorption, decomposition of harmful chemicals, deodorization, and antibacterial and antifungal performance (Yan, 2013). However, according to a survey by Dubai Municipality, some functional building materials in circulation do not have the effect of adsorbing pollutants (Municipality Dubai, 2020). The average adsorption rate of HCHO was investigated to be less than 40%, so the evaluation of the indoor air environment improvement function of the raw material itself added to these products is insufficient (Lu et al., 2010; Lin et al., 2020).
Considering the health and safety of residents and activating the application of materials with excellent performance and function, this paper aims to evaluate the performance of functional building materials and analyze the effect of improving the indoor air environment (Bribián et al., 2011). In addition, there is an increasing need to prove the objective effect of functional building materials by deriving an accurate performance evaluation method for functional building materials that can be produced, commercialized, and put to practical use from the manufacturing process of functional building materials (Sawada and Serizawa, 2018).
In this study, wallpaper, which occupies the most significant indoor area among interior finishing materials and has the most excellent applicability to actual sites, was mainly targeted. HCHO adsorption performance was evaluated by making functional wallpaper containing HCHO remover using ethylene urea (C5H10N2O3) material (Jin et al., 2017).
The research method is summarized as follows. First, investigate and analyze research trends and standards for functional building materials and related standards. Second, prepare five samples by adding 0% (no additive), 5%, 7%, 10%, and 15% of the C5H10N2O3 remover made of ethylene urea to the weight of the binder, respectively (Hematabadi et al., 2012). Third, by constructing an HCHO adsorption test system using a 20 L small chamber, changes in HCHO concentration over time by the prepared wallpaper samples are collected and analyzed. Fourth, evaluate the adsorption performance of functional wallpaper by calculating the HCHO adsorption rate, adsorption rate, ventilation rate conversion value, and accumulated adsorption amount, respectively (Shim and Choi, 2017).
A total of 153 products, including 117 available materials, 34 paints, and 2 adhesives, are currently distributed in the UAE from 88 companies (University, 2022). The product’s primary functions are indoor humidity control, pollutant adsorption, antibacterial and antifungal, far-infrared radiation, negative ion emission, and deodorization (Chen et al., 2012). Table 1 summarizes the status of functional building materials distributed in the UAE by function.
UAE building material manufacturers develop functional materials that mix natural materials such as loess, charcoal, and diatomaceous earth (Municipality Dubai, 2021). However, the evaluation of pollutant adsorption and moisture absorption and the moisture-proof performance of these raw materials is insufficient (Zou et al., 2021). Although the development and distribution of functional building materials commonly used to improve indoor air quality have increased significantly, these building materials’ objective effects, evaluation methods, and standards have not been established (Lan et al., 2021). In the case of some products, exaggerated advertisements about the adsorption performance of the product are being made, increasing consumer confusion. Some of the products in circulation did not have the effect of adsorbing chemically harmful pollutants (Kim et al., 2011). In particular, in the case of HCHO, the average adsorption rate was found to be less than 40% (Kibanova et al., 2012).
Belakroum et al. (2017) investigated changes in moisture content by experimenting with loess, wood, mortar, and charcoal to confirm the moisture absorption/desorption ability of eco-friendly and functional materials and then investigated the effect on indoor humidity control (Belakroum et al., 2018). Currently, building materials mixed with natural materials such as loess, charcoal, and diatomaceous earth are being developed in the UAE. Still, scientific evidence of the effect of improving the indoor air quality of these materials was reported to be insufficient (Belakroum et al., 2017).
Suresh and Bandosz (2018) developed a functional gypsum board that can reduce the concentration by adsorbing and decomposing HCHO in the indoor air and verified its performance through a real test (Suresh and Bandosz, 2018). As a result of the study, the developed functional gypsum board dropped to the lowest concentration level after 10 days (Blondeau et al., 2014). It showed a relatively significant decrease compared to the general gypsum board. It was found that silk wallpaper with low air permeability harmed the HCHO decomposition and adsorption performance of functional gypsum boards (Yu and Kim, 2013).
Vikrant et al. (2019) measured and analyzed the adsorption performance of HCHO and volatile organic compounds for functional building materials with pollutant adsorption and decomposition performance (Vikrant et al., 2019). The adsorption performance of samples was measured using the adsorption chamber system for ceiling and wall materials made of mineral fiber, loess soil, and charcoal (Zhou et al., 2020). As a result of the measurement, even if the adsorption performance is excellent, if re-release occurs, it cannot be called a building material with adsorption function, so it is suggested that re-release should be considered necessary.
Na et al. (2019) conducted an adsorption performance test focusing on HCHO on four types of functional building materials in the domestic market. As a result, all of the building materials tested showed an adsorption rate of 65% or more, higher than the excellent grade (60% or more) (Lubis et al., 2019).
Recently, research on material evaluation for the development of functional materials has been actively conducted. Zhang et al. (2019) evaluated and verified the adsorption performance of BTEXS materials, including total volatile organic compounds (TVOC), using three types of porous natural minerals: diatomaceous earth, zeolite, and volcanic ash for the development of functional building materials. It was confirmed that there is a limit to the adsorption performance using only raw materials.
Nor et al. (2013) confirmed the excellent effect of improving the pollutant removal performance when metal oxides such as copper nitrate are impregnated with natural materials through a study on toluene removal using copper oxide-impregnated volcanic ash. It was also predicted that developing functional building materials using minerals through material modification would be necessary (Hua et al., 2012).
In another study, Park et al. (2015) used CFD analysis to examine the effect of reducing the concentration of contaminants of adsorbed building materials in office spaces. They confirmed that adsorbed building materials removed some toluene (Park et al., 2015; Nakahara et al., 2020).
In major advanced countries, evaluating the amount of pollutant emission has been standardized to manage pollutants in building materials (Du and Li, 2020). According to the pollutant emission characteristics and materials to be assessed, the standards shown in Table 2 have been established. In addition, standards for evaluating the functionality of materials are prepared, and evaluation methods for moisture absorption and moisture-proof function and adsorption function have been developed (Wu et al., 2015).
Currently, the adsorption performance evaluation method of functional building materials is JIS A 1905-11, JIS A 1905-21 of Japan (SAI Global, 2015) (Test method for reducing indoor air pollution source of adsorption building materials by the small chamber method—Part 1, Part 2 February 2007) was enacted. In 2008, JIS A 1961 was passed to target substances from HCHO to volatile organic compounds and other aldehydes (Huang et al., 2019). In addition, ISO 16000-23, an HCHO adsorption performance evaluation standard based on JIS A 1905-1 and JIS A 1906, and ISO 16000-24, a volatile organic compound adsorption performance evaluation standard, were established in March 2008 as DIS status (Chang et al., 2015). It was later adopted as an international standard in December 2009 (Yu and Crump, 2011). In Korea, in 2012, the VOCs and aldehyde reduction performance evaluation method of pollutant-reducing building materials was established as the KS standard (Zheng et al., 2011).
In this study, an HCHO remover was added to the wallpaper manufacturing process, and the removal performance was evaluated according to the amount added (Figure 1) (Ghani et al., 2018). HCHO remover was prepared by mixing 35 wt% of Urea with 2 wt% of glycol to reduce viscosity and water (Jeon et al., 2020). Ethylene urea has the property of removing HCHO through a condensation reaction, so it is widely used as an HCHO remover (Wi et al., 2020). Urea (H2NCONH2) and HCHO produce monomethyl urea (H2NCONHCH2OH) by N-methylenation reaction as in Eq. 1. HCHO is decomposed as in Eq. 2 by the condensation reaction between the produced monomethyl urea and urea (Chen et al., 2017).
FIGURE 1. (A) 20 L Small Chamber when closed during the experiment and (B) Inside the 20 L Small Chamber showing the Wallpaper Sample.
In this study, five types of wallpaper were produced by adding 0%, 5%, 7%, 10%, and 15% of the HCHO remover to the weight of the binder used for wallpaper production, respectively, to comparatively analyze and confirm the adsorption performance of HCHO (Yue et al., 2021).
To evaluate the HCHO adsorption performance of functional wallpaper to which HCHO adsorbent is added, in the measurement method, ISO/DIS 16000-23, JIS 1905-1, JIS A1905-2, and JIS A 1906 (Table 2), the construction of the measurement system and the measurement plan were established and performed (Tiwari et al., 2017).
The flow rate of the 20 L small chamber was controlled to 167,000 μl/min using a mass flow controller (MFC) so that the measurement conditions in the chamber were maintained so that the ventilation rate was 0.5 times/h (Kim et al., 2010). The temperature was measured at 25°C ± 1°C and relative humidity (RH) at 50% ± 3%. HCHO gas having a concentration of 60 ppm was diluted using a pure air generator and then supplied into a small chamber (Figure 1). The feed concentration was 210 μg/m3 (±10%). Figure 2 shows the composition of the measurement sample and the 20 L small chamber where the piece is installed, and the overall measurement system (An et al., 2010).
The HCHO adsorption performance test was conducted for 10 days, and HCHO gas was supplied to the small chamber for 7 days after sample input (Lee and Kim, 2012). Samples were collected on days 1, 3, 5, and 7 using a DNPH-cartridge. The sampling capacity was 5 L (using two sides wallpapers sheets of 7.5 cm × 12 cm on a gypsum board of 6.35 mm) for 38 min at 133.000 μl/min (Park et al., 2011). The collected samples were analyzed using High-Performance Liquid Chromatography (HPLC). The specifications of HPLC used in the study (Table 3) and primary analysis conditions (Table 4) are as follows.
The sample’s adsorption rate and cumulative adsorption amount were calculated using the analyzed inlet and outlet concentrations of the 20 L small chamber. The formula for calculating the adsorption rate is (Eq. 3) (Zhu et al., 2019).
Ce,t: Chamber Inlet Concentration (μg/m3).Cout,t: Chamber Outlet Concentration (μg/m3).
The pollutant adsorption rate (F) indicates the mass of the target material adsorbed per unit for a specified time from the start of the test. It is calculated as follows by the concentration difference between the inlet and outlet of the chamber, the chamber ventilation Qc, and the surface area A of the sample (Eq. 4) (Zhang et al., 2021).
F: Contaminant adsorption rate per unit time and area (μg/m2h).Qc: Amount of Chamber Ventilation (m3/h).A: Surface area of the sample (m2).
The sample’s ventilation amount conversion value (Qeq) is converted into the ventilation amount by the amount of clean air introduced to achieve the same effect as the concentration reduction effect of building materials (Zhu et al., 2022). It is calculated as in Equation 5, and it means that it is constant regardless of the concentration of contaminants under the assumption that the surface concentration of the sample is 0 (Zong et al., 2021).
Qeq: Adsorption performance sample ventilation rate conversion value (m3/m2h).
The accumulated adsorption amount Sc was calculated by the ventilation amount Qc of the chamber and the standard gas supply time Te (h) (Chen et al., 2022).
Sc: Accumulated adsorption amount (μg/m3).Te: Standard gas supply time (h).
To evaluate the HCHO adsorption performance of functional wallpaper, the adsorption performance was assessed for 7 days by comparing standard wallpaper without HCHO remover, and four types of functional wallpaper with different amounts of remover added. As evaluation items for quantitative comparison, HCHO concentration at the inlet/outlet for each sample, adsorption rate, ventilation rate conversion value, cumulative adsorption amount, and adsorption rate were selected and compared. Table 5 summarizes the adsorption rate, ventilation rate conversion value, accumulated adsorption amount, and adsorption rate according to the amount of HCHO remover added.
Figure 3 shows the results of the HCHO adsorption experiment for standard wallpaper with the addition of formaldehyde remover at 0 wt%. The traditional wallpaper without adding HCHO remover showed an adsorption rate of 15.93%, with a supply concentration of 214.74 μg/m3 on the first day and an outlet concentration of 180.54 μg/m3 on the first day. On the third day, it slightly increased to 18.94%. After that, the outlet concentration increased, and the adsorption rate decreased, showing an adsorption rate of 6.21% on the seventh day.
Figure 4 shows the results of the HCHO adsorption experiment with 5 wt% added wallpaper. When 5 wt% of HCHO remover was added, the first day supply concentration was 213.74 μg/m3, and the outlet concentration was 73.93 μg/m3, showing the highest adsorption rate of 65.41%. As time passed, the reduction rate of HCHO concentration decreased, showing an adsorption rate of 50.43% on the seventh day. The adsorption rate also reduced from the fifth day, and it was found that the HCHO removal performance gradually decreased. The cumulative adsorption amount for 7 days during the measurement period was 4.310 μg/m2, which did not reach ISO 16000-24 standard for both the adsorption rate and the cumulative adsorption amount.
Figure 5 shows the HCHO adsorption experiment of 7wt% added wallpaper. The first day outlet concentration of wallpaper with 7wt% added was 62.28 μg/m3, which showed an adsorption rate of 71.81% compared to the supply concentration of 220.92 μg/m3. It showed an HCHO removal efficiency of 60.21%. The adsorption rate of 60.21% on the seventh day corresponds to a good grade of 60% or more, but the cumulative adsorption amount was 4.857 μg/m2, which was lower than the ISO 16000-24 standard.
As shown in Figure 6, the results of the adsorption test of wallpaper to which 10 wt% of HCHO remover was added showed an adsorption rate of 74.12% with an inlet concentration of 216.81 μg/m3 and an outlet concentration of 56.12 μg/m3 on the first day. The outlet concentration gradually increased, showing a formaldehyde adsorption rate of 63.45% on the seventh day. In the case of wallpaper with 10 wt% added, the adsorption rate was more than the ISO 16000-24 standard of 60%, but the cumulative adsorption amount was 5.042 μg/m2, which fell short of the standard.
Figure 7 shows the results of the HCHO adsorption experiment of 15wt% added wallpaper. When 15 wt% was added, the adsorption rate was 78.40%, with an outlet concentration of 49.49 μg/m3 on the first day. After that, on the seventh day, the adsorption rate was 73.58%, showing an adsorption rate of 70% during the test period, indicating a relatively small decrease in the adsorption rate compared to wallpaper to which a removal agent of another weight ratio was added. However, even in the case of wallpaper to which 15 wt% of HCHO remover was added, it was only applicable to the ISO 16000-24 standard of 60% or more and did not reach the ISO 16000-24 excellent grade of 85%. The accumulated adsorption amount was 5.736 μg/m2, which did not meet the superb grade standard. There was no significant difference with the wallpaper to which 10 wt% of HCHO remover was added.
As a result of measuring the HCHO adsorption performance of five types of wallpaper prepared according to the conditions for adding the HCHO remover, the adsorption performance increased proportionally as the HCHO remover added increased. When the addition amount of the remover was 7% or more relative to the binder mass, the adsorption rate on the seventh day was 60% or more, which corresponds to the ISO 16000-24 standard. In the case of the accumulated adsorption amount, the maximum of 5.736 μg/m2 for wallpaper with 15 wt% of HCHO remover added the most was lower than the ISO 16000-24 standard of 6.000 μg/m2.
Until now, UAE standards have focused only on the health aspects of domestic building materials that emit less harmful chemicals. However, in Japan and Korea, the government revised the construction of eco-friendly functional building materials, which was recommended in JIS A 1905/1906 and KS I 3546/3547, into a mandatory requirement in 2013 (Shim et al., 2019).
In the UAE, Dubai Municipality is working to improve the IAQ by preparing indoor air quality standards to protect the health and safety of residents from environmental diseases such as SBS and atopy (Business (2022), 2022). Therefore, it is necessary to prepare standards for the functional aspects of building materials such as adsorption and decomposition performance of harmful chemicals, moisture absorption, moisture-proof performance, and antibacterial/anti-fungal performance to activate the application of eco-friendly functional building materials with excellent performance and function.
Further studies are needed regarding more interior finishes materials, especially the ones in popular usage, including full-scale experiments to give precise data for the preparation of the UAE’s IAQ indoor air quality standards to protect the health and safety of its residents.
In this study, to improve the HCHO adsorption performance of wallpaper, which has a relatively large indoor area among functional materials, in UAE, and has the most significant practical applicability, five samples were prepared by adding an HCHO remover made of ethylene urea material, and the adsorption performance was evaluated. Worth mentioning that the wallpaper quality used in UAE is considered from the high-quality types in terms of durability. Additionally, UAE’s users may change their mood frequently (6 months to 1-year range) and therefore wallpapers are the best choice (Business (2022), 2022).
Functional wallpaper with HCHO remover added demonstrated HCHO removal effectiveness over a specified threshold, as opposed to conventional wallpaper. In the case of standard wallpaper, the adsorption rate on the seventh day was 6.21%, and the HCHO removal performance showed a difference in the adsorption rate and the cumulative adsorption amount according to the amount of the remover added. When the adsorption rate on the seventh day was compared, 5 wt% added resulted in 50.43%, 7 wt% in 60.21%, 10 wt% in 63.45%, and 15 wt% in 73.58%. The adsorption rate grade according to the ISO 16000-24 standard showed an excellent grade level of 60% or more when 7 wt%, 10 wt%, and 15 wt% were added based on the seventh day result. Therefore, the experiment was stopped on day 7, although it is recommended in the UNE 16000-9 are 28 days.
The cumulative adsorption amount also increased as the HCHO remover added increased. However, wallpaper added with 15 wt%, which showed the highest value, was 5.736 μg/m2, which did not satisfy the excellent grade of 6.000 μg/m2 of the ISO 16000-24 standard. The 15 wt% added wallpaper helps the superb quality with an adsorption rate of 70% or more, but the cumulative adsorption amount did not meet the standard.
When the amount of the HCHO remover is increased, the adsorption performance is judged to be improved in proportion to the amount added. It is believed that as the amount of additives other than binders used in the wallpaper process increases, the basic physical properties of the wallpaper may be weakened in proportion to this.
In addition, although a relatively large amount of 15% of the total binder mass was added, the results did not reach a good grade. Accordingly, it is judged that research on the development of functional materials with improved HCHO removal performance using various materials and the study of changes in the physical properties of wallpaper according to the number of additives in the wallpaper manufacturing process should be carried out together.
The original contributions presented in the study are included in the article/Supplementary Material, further inquiries can be directed to the corresponding author.
All authors contributed significantly to this study. CJ and GE, NA and GE identified and secured the example buildings used in the study. The data acquisition system and installation of sensors were designed and installed by CJ and NA. NA and GE was responsible for data collection. CJ and NA performed data analysis. The manuscript was compiled by CJ and GE and reviewed by NA. All authors have read and agreed to the published version of the manuscript.
The authors would like to express their gratitude to Ajman University for the generous support in publishing this paper.
The authors declare that the research was conducted in the absence of any commercial or financial relationships that could be construed as a potential conflict of interest.
All claims expressed in this article are solely those of the authors and do not necessarily represent those of their affiliated organizations, or those of the publisher, the editors and the reviewers. Any product that may be evaluated in this article, or claim that may be made by its manufacturer, is not guaranteed or endorsed by the publisher.
Ai, Z. T., Mak, C. M., and Cui, D. J. (2015). On-site measurements of ventilation performance and indoor air quality in naturally ventilated high-rise residential buildings in Hong Kong. Indoor Built Environ. 24 (2), 214–224. doi:10.1177/1420326x13508566
Al Qassimi, N., and Jung, C. (2021). The effect of air purifying plants on the reduction of volatile organic compounds (VOCs) during the four seasons in UAE. Front. Built Environ., 188.
An, J. Y., Kim, S., Kim, H. J., and Seo, J. (2010). Emission behavior of formaldehyde and TVOC from engineered flooring in under heating and air circulation systems. Build. Environ. 45 (8), 1826–1833. doi:10.1016/j.buildenv.2010.02.012
Arar, M., and Jung, C. (2021). Improving the indoor air quality in nursery buildings in United Arab Emirates. Int. J. Environ. Res. Public Health 18 (22), 12091. doi:10.3390/ijerph182212091
Arar, M., Jung, C., and Qassimi, N. A. (2021). Investigating the influence of the building material on the indoor air quality in apartment in Dubai. Front. Built Environ. 7, 804216. doi:10.3389/fbuil.2021.804216
Awad, J., and Jung, C. (2021a). Evaluating the indoor air quality after renovation at the greens in Dubai, United Arab Emirates. Buildings 11 (8), 353. doi:10.3390/buildings11080353
Awad, J., and Jung, C. (2021b). The evaluation of residents' health in high-rise residential buildings in Dubai, United Arab Emirates. Front. Built Environ. 145. doi:10.3389/fbuil.2021.766057
Belakroum, R., Gherfi, A., Bouchema, K., Gharbi, A., Kerboua, Y., Kadja, M., et al. (2017). Hygric buffer and acoustic absorption of new building insulation materials based on date palm fibers. J. Build. Eng. 12, 132–139. doi:10.1016/j.jobe.2017.05.011
Belakroum, R., Gherfi, A., Kadja, M., Maalouf, C., Lachi, M., El Wakil, N., et al. (2018). Design and properties of a new sustainable construction material based on date palm fibers and lime. Constr. Build. Mater. 184, 330–343. doi:10.1016/j.conbuildmat.2018.06.196
Blondeau, P., Séguy, C., Nicolle, J., and Abadie, M. O. (2014). Experimental characterization and modeling of a functional wall covering removing formaldehyde from the indoor air. La Rochelle: Indoor Air.
Bribián, I. Z., Capilla, A. V., and Usón, A. A. (2011). Life cycle assessment of building materials: Comparative analysis of energy and environmental impacts and evaluation of the eco-efficiency improvement potential. Build. Environ. 46 (5), 1133–1140. doi:10.1016/j.buildenv.2010.12.002
Business (2022), Gulf Why UAE residents must pay closer attention to their indoor air quality. Retrieved from https://gulfbusiness.com/why-uae-residents-must-pay-closer-attention-to-their-indoor-air-quality/.
Chang, S. J., Wi, S., Jeong, S. G., and Kim, S. (2015). Evaluation of the adsorption performance and sustainability of exfoliated graphite nanoplatelets (xGnP) for VOCs. Materials 8 (11), 7615–7621. doi:10.3390/ma8115412
Chen, L. Y., Lin, M. W., and Chuah, Y. K. (2017). Investigation of a potted plant (Hedera helix) with photo-regulation to remove volatile formaldehyde for improving indoor air quality. Aerosol Air Qual. Res. 17 (10), 2543–2554. doi:10.4209/aaqr.2017.04.0145
Chen, P. Y., McKittrick, J., and Meyers, M. A. (2012). Biological materials: Functional adaptations and bioinspired designs. Prog. Mater. Sci. 57 (8), 1492–1704. doi:10.1016/j.pmatsci.2012.03.001
Chen, Q., Tian, E., Luo, Z., and Mo, J. (2022). Adsorption film with sub-milli-interface morphologies via direct ink writing for indoor formaldehyde removal. J. Hazard. Mater. 427, 128190. doi:10.1016/j.jhazmat.2021.128190
Du, W., and Li, M. (2020). Assessing the impact of environmental regulation on pollution abatement and collaborative emissions reduction: Micro-evidence from Chinese industrial enterprises. Environ. Impact Assess. Rev. 82, 106382. doi:10.1016/j.eiar.2020.106382
Ghani, A., Ashaari, Z., Bawon, P., and Lee, S. H. (2018). Reducing formaldehyde emission of urea formaldehyde-bonded particleboard by addition of amines as formaldehyde scavenger. Build. Environ. 142, 188–194. doi:10.1016/j.buildenv.2018.06.020
Hematabadi, H., Behrooz, R., Shakibi, A., and Arabi, M. (2012). The reduction of indoor air formaldehyde from wood based composites using urea treatment for building materials. Constr. Build. Mater. 28 (1), 743–746. doi:10.1016/j.conbuildmat.2011.09.018
Hua, M., Zhang, S., Pan, B., Zhang, W., Lv, L., and Zhang, Q. (2012). Heavy metal removal from water/wastewater by nanosized metal oxides: A review. J. Hazard. Mater. 211, 317–331. doi:10.1016/j.jhazmat.2011.10.016
Huang, K. C., Tsay, Y. S., Lin, F. M., Lee, C. C., and Chang, J. W. (2019). Efficiency and performance tests of the sorptive building materials that reduce indoor formaldehyde concentrations. PloS one 14 (1), e0210416. doi:10.1371/journal.pone.0210416
Huangfu, Y., Lima, N. M., O’Keeffe, P. T., Kirk, W. M., Lamb, B. K., Walden, V. P., et al. (2020). Whole-house emission rates and loss coefficients of formaldehyde and other volatile organic compounds as a function of the air change rate. Environ. Sci. Technol. 54 (4), 2143–2151. doi:10.1021/acs.est.9b05594
Jeon, J., Park, J. H., Wi, S., Yun, B. Y., Kim, T., and Kim, S. (2020). Field study on the improvement of indoor air quality with toluene adsorption finishing materials in an urban residential apartment. Environ. Pollut. 261, 114137. doi:10.1016/j.envpol.2020.114137
Jiang, C., Li, D., Zhang, P., Li, J., Wang, J., and Yu, J. (2017). Formaldehyde and volatile organic compound (VOC) emissions from particleboard: Identification of odorous compounds and effects of heat treatment. Build. Environ. 117, 118–126. doi:10.1016/j.buildenv.2017.03.004
Jin, S. J., Khan, Y., Maeng, J. H., Kim, Y. J., Hwang, J., Cheong, M., et al. (2017). Efficient catalytic systems for the carboxylation of diamines to cyclic ureas using ethylene urea as a promoter. Appl. Catal. B Environ. 209, 139–145. doi:10.1016/j.apcatb.2017.02.079
Jung, C., and Al Qassimi, N. (2022). Investigating the emission of hazardous chemical substances from mashrabiya used for indoor air quality in hot desert climate. Sustainability 14 (5), 2842. doi:10.3390/su14052842
Jung, C., and Awad, J. (2021a). The improvement of indoor air quality in residential buildings in Dubai, UAE. Buildings 11 (6), 250. doi:10.3390/buildings11060250
Jung, C., and Awad, J. (2021b). The analysis of indoor air pollutants emission from new apartments at business bay in Dubai, UAE. Front. Built Environ. 152. doi:10.3389/fbuil.2021.765689
Jung, C., and Awad, J. (2021c). Improving the IAQ for learning efficiency with indoor plants in university classrooms in ajman, United Arab Emirates. Buildings 11 (7), 289. doi:10.3390/buildings11070289
Kibanova, D., Sleiman, M., Cervini-Silva, J., and Destaillats, H. (2012). Adsorption and photocatalytic oxidation of formaldehyde on a clay-TiO2 composite. J. Hazard. Mater. 211, 233–239. doi:10.1016/j.jhazmat.2011.12.008
Kim, K. H., Jahan, S. A., and Lee, J. T. (2011). Exposure to formaldehyde and its potential human health hazards. J. Environ. Sci. Health, Part C 29 (4), 277–299. doi:10.1080/10590501.2011.629972
Kim, K. W., Kim, S., Kim, H. J., and Park, J. C. (2010). Formaldehyde and TVOC emission behaviors according to finishing treatment with surface materials using 20 L chamber and FLEC. J. Hazard. Mater. 177 (1-3), 90–94. doi:10.1016/j.jhazmat.2009.09.060
Lan, H., Zhang, Y., Cheng, M., Li, Y., and Jing, Z. (2021). An intelligent humidity regulation material hydrothermally synthesized from ceramic waste. J. Build. Eng. 40, 102336. doi:10.1016/j.jobe.2021.102336
Lee, J. H., and Kim, S. (2012). The determination of the adsorption performance of graphite for VOCs and formaldehyde. Energy Build. 46, 56–61. doi:10.1016/j.enbuild.2011.10.046
Lin, Z., Shen, W., Roux, J. C., and Xi, H. (2020). Photo-catalytic degradation of mixed gaseous HCHO and C6H6 in paper mills: Experimental and theoretical study on the adsorption behavior simulation and catalytic reaction mechanism. J. Hazard. Mater. 388, 121779. doi:10.1016/j.jhazmat.2019.121779
Lu, Y., Wang, D., Ma, C., and Yang, H. (2010). The effect of activated carbon adsorption on the photocatalytic removal of formaldehyde. Build. Environ. 45 (3), 615–621. doi:10.1016/j.buildenv.2009.07.019
Lubis, M. A. R., Park, B. D., and Lee, S. M. (2019). Performance of hybrid adhesives of blocked-pMDI/melamine-urea-formaldehyde resins for the surface lamination on plywood. J. Korean Wood Sci. Technol. 47 (2), 200–209. doi:10.5658/wood.2019.47.2.200
Municipality Dubai (2018). Green building Regulations & specifications. Retrieved from: https://www.dm.gov.ae/wp-content/uploads/2018/01/05_ENG_DCL_LawsLegislation_EngineeringSection_GreenBuildingRegulation.pdf.
Municipality Dubai (2020). Green buildings in Dubai. Retrieved from: https://www.dm.gov.ae/municipality-business/al-safat-dubai-green-building-system/green-building-in-dubai/.
Municipality, Dubai (2021). Practice guide Al safat Regulations rev 01. Retrieved from: https://www.dm.gov.ae/wp-content/uploads/2021/01/Practice-Guide-Al-Safat-Regulations-Rev-01.pdf.
Na, C. J., Yoo, M. J., Tsang, D. C., Kim, H. W., and Kim, K. H. (2019). High-performance materials for effective sorptive removal of formaldehyde in air. J. Hazard. Mater. 366, 452–465. doi:10.1016/j.jhazmat.2018.12.011
Nakahara, K., Muttakin, M., Yamamoto, K., and Ito, K. (2020). Computational fluid dynamics modelling of the visible light photocatalytic oxidation process of toluene for indoor building materials with locally doped titanium dioxide. Indoor Built Environ. 29 (2), 163–179. doi:10.1177/1420326x19854499
Nationals, (2013). Indoor air quality in Dubai to be tested. Retrieved from: https://www.thenationalnews.com/uae/indoor-air-quality-in-dubai-to-be-tested-1.285677.
Nor, N. M., Lau, L. C., Lee, K. T., and Mohamed, A. R. (2013). Synthesis of activated carbon from lignocellulosic biomass and its applications in air pollution control—A review. J. Environ. Chem. Eng. 1 (4), 658–666. doi:10.1016/j.jece.2013.09.017
Park, J. C., Kwon, Y. C., and Jun, H. D. (2011). A study on the improvement of indoor air quality of newly-built apartment houses using low emission building materials. J. Asian Archit. Build. Eng. 10 (1), 235–240. doi:10.3130/jaabe.10.235
Park, S., Seo, J., and Kim, J. T. (2015). A study on the application of sorptive building materials to reduce the concentration and volume of contaminants inhaled by occupants in office areas. Energy Build. 98, 10–18. doi:10.1016/j.enbuild.2014.12.056
Ratera, I., and Veciana, J. (2012). Playing with organic radicals as building blocks for functional molecular materials. Chem. Soc. Rev. 41 (1), 303–349. doi:10.1039/c1cs15165g
Sai Global, (2015). Jis a 1905-1:2015. Retrieved from: https://infostore.saiglobal.com/en-us/Standards/JIS-A-1905-1-2015-624945_SAIG_JSA_JSA_1435108/.
Sawada, T., and Serizawa, T. (2018). Filamentous viruses as building blocks for hierarchical self-assembly toward functional soft materials. Bull. Chem. Soc. Jpn. 91 (3), 455–466. doi:10.1246/bcsj.20170428
Shim, H., and Choi, G. (2017). Study of construction convergence technology for performance improvement in functional building materials. J. Build. Eng. 11, 108–114. doi:10.1016/j.jobe.2017.04.008
Shim, H., Kim, T., and Choi, G. (2019). Technology roadmap for eco-friendly building materials industry. Energies 12 (5), 804. doi:10.3390/en12050804
Steinemann, A., Wargocki, P., and Rismanchi, B. (2017). Ten questions concerning green buildings and indoor air quality. Build. Environ. 112, 351–358. doi:10.1016/j.buildenv.2016.11.010
Suresh, S., and Bandosz, T. J. (2018). Removal of formaldehyde on carbon-based materials: A review of the recent approaches and findings. Carbon 137, 207–221. doi:10.1016/j.carbon.2018.05.023
Tham, K. W. (2016). Indoor air quality and its effects on humans—a review of challenges and developments in the last 30 years. Energy Build. 130, 637–650. doi:10.1016/j.enbuild.2016.08.071
The Khaleej Times (2013). Dubai Municipality to assess the quality of indoor air in public buildings. Retrieved from: https://www.khaleejtimes.com/article/dubai-municipality-to-assess-quality-of-indoor-air-in-public-buildings.
Tiwari, D., Goel, C., Bhunia, H., and Bajpai, P. K. (2017). Melamine-formaldehyde derived porous carbons for adsorption of CO2 capture. J. Environ. Manag. 197, 415–427. doi:10.1016/j.jenvman.2017.04.013
University, K, (2022). Design and development of smart functional materials for efficient water purification. Retrieved from: https://www.ku.ac.ae/visiting-undergraduate-research-program/design-and-development-of-smart-functional-materials-for-efficient-water-purification.
Vikrant, K., Cho, M., Khan, A., Kim, K. H., Ahn, W. S., and Kwon, E. E. (2019). Adsorption properties of advanced functional materials against gaseous formaldehyde. Environ. Res. 178, 108672. doi:10.1016/j.envres.2019.108672
Wi, S., Kim, M. G., Myung, S. W., Baik, Y. K., Lee, K. B., Song, H. S., et al. (2020). Evaluation and analysis of volatile organic compounds and formaldehyde emission of building products in accordance with legal standards: A statistical experimental study. J. Hazard. Mater. 393, 122381. doi:10.1016/j.jhazmat.2020.122381
Wu, Y., Gong, G., Yu, C. W., and Huang, Z. (2015). Proposing ultimate moisture buffering value (UMBV) for characterization of composite porous mortars. Constr. Build. Mater. 82, 81–88. doi:10.1016/j.conbuildmat.2015.02.058
Yan, Z. G. (2013). A review of aerogels and their application as a multi-functional building material. Appl. Mech. Mater. 253, 564–567. doi:10.4028/www.scientific.net/amm.253-255.564
Yu, C., and Crump, D. (2011). Standards for evaluating indoor air. Indoor Built Environ. 20 (4), 389–392. doi:10.1177/1420326x11417330
Yu, C. W., and Kim, J. T. (2013). Photocatalytic oxidation for maintenance of indoor environmental quality. Indoor Built Environ. 22 (1), 39–51. doi:10.1177/1420326x12470282
Yue, X., Ma, N. L., Sonne, C., Guan, R., Lam, S. S., Van Le, Q., et al. (2021). Mitigation of indoor air pollution: A review of recent advances in adsorption materials and catalytic oxidation. J. Hazard. Mater. 405, 124138. doi:10.1016/j.jhazmat.2020.124138
Zhang, G., Liu, Y., Zheng, S., and Hashisho, Z. (2019). Adsorption of volatile organic compounds onto natural porous minerals. J. Hazard. Mater. 364, 317–324. doi:10.1016/j.jhazmat.2018.10.031
Zhang, S., Xing, J., Ling, J., and Yang, H. (2021). Experimental study on performance and influencing factors of chitosan-based nonwoven on gaseous HCHO removal. Build. Environ. 190, 107565. doi:10.1016/j.buildenv.2020.107565
Zheng, Q., Lee, D., Lee, S., Kim, J. T., and Kim, S. (2011). A health performance evaluation model of apartment building indoor air quality. Indoor Built Environ. 20 (1), 26–35. doi:10.1177/1420326x10393719
Zhou, J., Liu, G., Jiang, Q., Zhao, W., Ao, Z., and An, T. (2020). Density functional theory calculations on single atomic catalysis: Ti-Decorated Ti3C2O2 monolayer (MXene) for HCHO oxidation. Chin. J. Catal. 41 (10), 1633–1644. doi:10.1016/s1872-2067(20)63571-9
Zhu, J., Lou, X., Wang, Y., Xiong, Z., Chen, J., and Yan, W. (2022). Conjugated microporous poly (aniline) s for removal of low-concentration formaldehyde. Chem. Eng. Sci. 248, 117119. doi:10.1016/j.ces.2021.117119
Zhu, X., Lv, M., and Yang, X. (2019). A test-based method for estimating the service life of adsorptive portable air cleaners in removing indoor formaldehyde. Build. Environ. 154, 89–96. doi:10.1016/j.buildenv.2019.03.018
Zong, Z., Chen, D., Zhao, C., Tang, G., Ji, Y., Zhang, H., et al. (2021). Photocatalytic degradation performance of gaseous formaldehyde by Ce-Eu/TiO2 hollow microspheres: From experimental evaluation to simulation. Environ. Sci. Pollut. Res. 28 (26), 34762–34775. doi:10.1007/s11356-021-13112-4
Keywords: indoor air quality, formaldehyde (HCHO), HCHO remover, eco-friendly functional building material, HCHO adsorption
Citation: Jung C, Alqassimi N and El Samanoudy G (2022) Evaluating the adsorption performance of functional building material with HCHO remover. Front. Built Environ. 8:998872. doi: 10.3389/fbuil.2022.998872
Received: 20 July 2022; Accepted: 10 August 2022;
Published: 02 September 2022.
Edited by:
Roberto Alonso González-Lezcano, CEU San Pablo University, SpainReviewed by:
Daniel Arranz Paraíso, Universidad San Pablo CEU, SpainCopyright © 2022 Jung, Alqassimi and El Samanoudy. This is an open-access article distributed under the terms of the Creative Commons Attribution License (CC BY). The use, distribution or reproduction in other forums is permitted, provided the original author(s) and the copyright owner(s) are credited and that the original publication in this journal is cited, in accordance with accepted academic practice. No use, distribution or reproduction is permitted which does not comply with these terms.
*Correspondence: Chuloh Jung, Yy5qdW5nQGFqbWFuLmFjLmFl
Disclaimer: All claims expressed in this article are solely those of the authors and do not necessarily represent those of their affiliated organizations, or those of the publisher, the editors and the reviewers. Any product that may be evaluated in this article or claim that may be made by its manufacturer is not guaranteed or endorsed by the publisher.
Research integrity at Frontiers
Learn more about the work of our research integrity team to safeguard the quality of each article we publish.