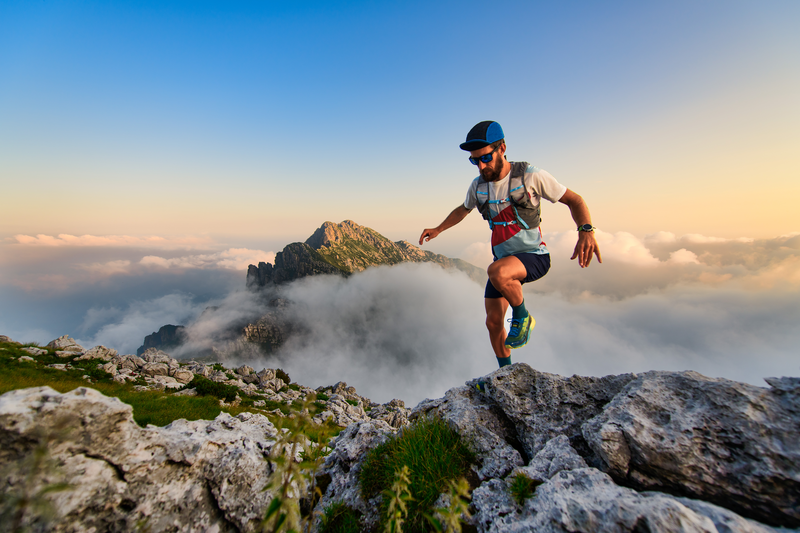
94% of researchers rate our articles as excellent or good
Learn more about the work of our research integrity team to safeguard the quality of each article we publish.
Find out more
REVIEW article
Front. Built Environ. , 28 September 2022
Sec. Construction Materials
Volume 8 - 2022 | https://doi.org/10.3389/fbuil.2022.974005
The historical earthquake activity that involved Andean countries in the last centuries makes the seismic risk evaluation associated to buildings in these areas an issue of crucial concern. Planning seismic risk mitigation policies requires an extensive knowledge of the territorial built environment, of the adopted construction techniques and of the adopted anti-seismic presidiums, in particular when applied during the reconstruction processes carried out after destructive events of the past. In this perspective, this paper contributes to the analysis of adobe vernacular buildings, which represent a spread typology in Latin America and the Caribbean, stressing the past construction practices, their fragilities, and the mitigation measures applied till today. To this purpose, the city center of Cusco, in Peru, where residential buildings are mostly made of adobe, is considered as a meaningful field of observation, because of the long history of the center, which allows to recognize also old construction techniques and the additions/raisings applied during the buildings life, as well as due to the relevant historical seismicity, which permits to appreciate the reconstruction processes implemented after seismic events occurred in the past.
Earthen buildings represent a solution widely used in many areas of the world since ancient times. As a result, nowadays, they host billions of peoples (Vyncke et al., 2018; Blondet et al., 2019) and their safety is a theme of utmost concern (Aroquipa and Hurtado, 2022). Earthen buildings have been conceived according to different construction typologies, often depending on local uses and local building cultures (Houben, 1994; Lourenço et al., 2018), sometimes mixing raw earth with other materials, such as wood and stone or, in more recent times, reinforced concrete and steel.
Among earthen building typologies, adobe solutions, composed of undried earthen blocks that are kept together by a mix of mud and straw, have traditionally been used for housing purposes, especially in Latin America and the Caribbean (LAC), where they represent the most recurrent structural typology in rural territories and urbanized areas. Moreover, adobe constructions are widely present in valuable historical centers, where they represent one of the most important “sign” of the colonial and of the post-colonial periods. The historical center of Cusco in Peru, recognized as UNESCO World Heritage Site in 1983, is one of the outstanding examples from this point of view.
Unfortunately, adobe buildings, commonly considered as “vernacular” constructions, have been often built extemporarily, without any design criterion, but, instead, based on “trial and error” principles (Papanikolaou and Taucer, 2004; Lourenço, 1996). Moreover, they have been often subjected to substantial changes during their life to satisfy residential needs -i.e., added stories, enlargement in plan, etc.- that often entailed a worsening of the structural behavior. As such, adobe buildings usually feature several structural deficiencies that make them highly vulnerable to seismic actions (Kuruscu et al., 2014), (Güney et al., 2016), (Chieffo et al., 2021). Thus, it is crucial to put in the field retrofitting interventions aimed at making them safer and mitigating their seismic risk (Illampas et al., 2015; Tarque et al., 2014; Tarque et al., 2012). From this perspective, it is essential to point out that, although reinforcement techniques have been addressed by several studies in the literature (Vargas-Neumann et al.,, 1986; Blondet et al., 2008a; Blondet et al., 2008b; Vargas-Neumann et al., 2007; Charleson, 2011; Gerardo Arayaet al., 2019), they have been applied seldom. For this reason, multi-level safety assessments are nowadays necessary to evaluate how much retrofitting interventions may potentially reduce the overall seismic vulnerability at both the buildings and the urban scales, so to prioritize the economical resources that have to be put in the field and to succeed in mitigation actions (Bruneau et al., 2003), (Diego et al., 2022). However, in order to carry out multi-level seismic vulnerability assessments, it is necessary to have extensive knowledge of local construction techniques (Tocchi et al., 2022; Chieffo et al., 2022). Several texts describe the construction techniques and properties of the adobe materials, but they do not deepen satisfyingly into the seismic behavior of these buildings. At the same time, studies dealing with the seismic response of adobe buildings often do not deepen how this response is affected by construction techniques.
Based on this premise, the main aim of this review paper is to analyze the most spread construction techniques used for adobe buildings in South America, starting from a general overview in Latin America and the Caribbean (Section 2) and then focusing the attention on adobe dwellings in Cusco (Section 3), where the authors have already carried out large-scale seismic vulnerability analyses (Brando et al., 2019; Spacone et al., 2019). The city of Cusco was chosen as a meaningful case study because it is rich of residential and religious adobe buildings of utmost architectural and historical value. Indeed, it was recognized a UNESCO World Heritage Site in 1983. Moreover, the high seismic risk of the area and the several earthquakes undergone by the city makes Cusco an interesting “laboratory” for detecting effective retrofitting interventions for mitigation actions to be applied to similar city of South America.
Latin America and the Caribbean (LAC) have been characterized by a relevant seismic activity, mainly concentrated along the Circum-Pacific belt. Strong earthquakes recorded in the past are the results of tectonic movements, and, sometimes, of volcanic eruptions. Indeed, Latin America, with its almost 240 active volcanoes, is the first area of the world in terms of eruptions in the last 10,000 years (Organization of American States. Department of Regional Development and Environment, Organization of American States. Natural Hazards Project, 1990). As such, the entire west coast of South and North America belongs to the so-called “Ring of Fire,” the Pacific area that measures about 40,000 km, characterized by the highest rate of earthquakes and volcanic eruptions. The seismic hazard map of the whole area is shown in Figure 1 and reports the expected Peak Ground Accelerations (PGAs) with a 10% probability of being exceeded in 50 years. It is evident that the Pacific coast is the area with the highest seismic hazard and that, since 2012 till today, the number of earthquakes recorded with a magnitude greater than 7 is relevant. The strongest earthquake ever recorded was the one that occurred in May 1960 in southern Chile, with a magnitude of 9.5 Mw, which caused about 5,700 deaths (Charvériat, 2012).
FIGURE 1. Seismic Hazard Map of Latin America and the Caribbean and distribution of adobe houses. (Source: http://www.seismo.ethz.ch/it/earthquakes/world/90days-mag6plus/).
Although earthquakes represent only 13% of the natural disasters affecting the territory of LAC, also considering the high risk related to hurricanes, floods, and landslides, the mortality linked to seismic events is undoubtedly the highest one, mainly due to the collapse of masonry and, more specifically, of adobe structures (Charvériat, 2012). Figure 1 also shows the territorial area of LAC, presenting a significant diffusion of adobe buildings (dashed black line). The map depicts that these buildings are mainly concentrated in rural and mountain areas, particularly along the Andean chain, from Argentina to Venezuela, as well as in the Caribbean countries, mountain and rural areas between Guatemala, El Salvador, Honduras, and Nicaragua.
Adobe buildings in LAC are mostly not engineered and do not present reinforcements or other anti-seismic details. Thus, they are vulnerable and prone to collapse even under low-intensity earthquakes. For example, during the 1970 earthquake of Peru (7.8 Mw), more than 90% of the damaged buildings were made of adobe, and their collapse provoked more than 40,000 deaths (Kuroiwa et al., 1973). Also, following the 7.5 Mw earthquake recorded in Guatemala in 1976 and the 6.6 Mw Bolivia earthquake (1998), 90% of the recorded fatalities were due to the collapse of adobe houses. In the new millenium, with the 7.6 Mw earthquake of El Salvador (2001), more than 200,000 adobe houses were heavily damaged or collapsed (Papanikolaou and Taucer, 2004).
For adobe buildings in the analyzed area, the adopted construction details vary from one country to another because each community has developed its own techniques and methods based on the type of soil, the climate conditions, and the local building culture. Consulting the World Housing Encyclopedia Report Database (Source: http://www.world-housing.net/), and (Viñuales et al., 2003), it is possible to make a comparison between the architectural and structural features for unreinforced adobe buildings recognized in these countries. Usually, vernacular buildings in adobe are single-family houses. They are composed of at least four rooms, including a living area with kitchen and standard rooms, a sleeping area with two bedrooms, and, in some cases, a commercial space. Moreover, they are single or double-story buildings, with a height varying from 2.00 to 3.00 m and with rectangular or square plans in which the length varies from 6.00 to 30.00 m and the width from 5.00 to 15.00 m. The spacing between the walls ranges from 3.00 to 6.00 m, while the wall thicknesses vary from 0.30 to 1.00 m, depending on the climate zone in which they are located (Figure 2) and the thermal insulation they have to guarantee. Also, the foundation systems vary, in terms of types and geometries, based on local construction practices and materials available on site. They are usually large and well-built stone walls: according to a consolidated “rule of thumbs,” the foundation depth ranges between 0.50 and 0.80 m, while the width is generally equal or slightly larger than the thickness of the supporting walls (Papanikolaou and Taucer, 2004). However, sometimes the foundation system could be absent and the vertical adobe walls of the buildings directly interface with the soil beneath. Atop the foundations, the massive load-bearing walls are made of adobe blocks of several dimensions: 40 × 20 × 10 cm or 40 × 40 × 10 cm blocks are typical in Peru, Argentina, Bolivia, and El Salvador; instead, smaller blocks (30 × 20 × 10 cm) are typical of adobe buildings in Panama, Nicaragua, and Honduras, as shown in, Table 1, where the main structural features of adobe dwellings and the relative Building Code in LAC are reported. Although block sizes can vary, manufacturing techniques are the same for all countries. Blocks are made of a mixture of sand, silt, clay, and water with straw. The differences of the mixture from a country to another relies on the percentages of the component elements and the drying period of the blocks, which depends on the type of soil and of the climatic conditions of the area (Table 1). For instance, the hot climate condition of Argentina and its clayey soil led to the production of adobe blocks with a high percentage of sand and straw, so to avoid the arising of cracks due to shrinkage phenomena. As such, the percentages of component materials (Viñuales et al., 2003) used to produce adobe blocks in this country are characterized by 60% of sand, 27% of silt, 11% of clay, and a significant amount of straw. On the other hand, the cold temperature in Bolivia and the sandier soil did not require these expedients: blocks are characterized by 20% of sand and silt, 60% of clay, and a low amount of straw. Also the drying period of adobe blocks can change from one country to another, still based on climate conditions: blocks were usually left to dry for 2 weeks in Peru, whereas, for the same process, 4 weeks were necessary for El Salvador, where temperatures are less hot. It is also to be pinpointed that the same mixture used for adobe blocks production was usually applied for mortar joints, with thicknesses ranging from 1.00 to 4.00 cm.
Adobe structures usually present no more than two stories, with horizontal wooden slabs and openings (at least one door and two windows) occupying approximately 30–40% of the wall length, as shown in Table 1. To ensure a proper bending strength of the spandrels upon the openings, the use of wooden lintels is a frequent practice, as shown in Figure 3.
FIGURE 3. Typical wooden lintels in (A) Guatemala (Source: Dominik et al., 2004), (B) Chile (Source: Velasquez, 2004), and (C) Peru.
Roof systems can be double-pitched or flat, although a slight slope for moving away the rainwater is always present. These roofs generally have a load-bearing structure with wooden or bamboo trussed beams that rest directly on the underlying adobe walls, without the presence of ring beams or other element of support Figures 4A,B. Above them, a wooden plank floor, surmounted by a layer of canes, is always present, with juxtaposed earth and clay tiles or metal sheeting (Figure 4C), as reported in Table 1.
FIGURE 4. Traditional wooden roofs in (A) El Salvador (Source: Lopez et al., 2004), (B) Honduras (Source: French, 2004a), and (C) Nicaragua (Source: French, 2004b).
The lack of efficient connections between orthogonal walls, the absence of ring beams to guarantee solidity to the construction and good loads transmission capacity, the presence of flexible roofs that do not act as rigid diaphragms, are structural peculiarities that detrimentally characterize most of the adobe buildings present in LAC, making them very sensitive to the activation of in-plane and out-of-plane kinematic mechanisms. Buttresses and ties, able to mitigate the vulnerability towards overturning mechanisms, are present seldom. This means that walls are often not adequately resistant to withstand lateral actions. In addition, walls often feature too large openings that do not respect adequate distances with the piers, this lowering the structural strength. Even lintels are often inadequate for their insufficient bonding length that do not ensure loads transmission near the openings.
It must finally pinpoint that the current configuration of most buildings are the result of construction processes carried out without control: many owners modified their dwellings based on their needs, expanding the building in plan or elevation without any preliminary project or prior authorization from the local authorities. Although the structural deficiencies of adobe buildings and their high seismic vulnerability are well recognized, only a few countries in the last 40 years worked to define specific Technical Standard specifically devoted for existing adobe constructions, as shown in Table 1, where the main structural features of adobe dwellings and the relative Building Code in LAC are reported. Among these, the Peruvian “Norma E.080—Diseño y construcción con tierra reforzada” was the first Code that included a chapter concerning adobe buildings. Defined for the first time in 1970, following the Huaraz earthquake, when about 70,000 people living in adobe houses died, it reached the current form after several revisions and, today is the best reference Standard for other countries of LAC (Ministerio de Cultura - Proyecto Qhapaq Ñan Sede Nacional, 2019). However, even in countries where Guidelines for adobe structures are present, the percentage of buildings at risk, due to the lack of adequate details, is still very high. This issue will be discussed in the following Sections with specific reference to one of the most important historical centre of Peru.
In the previous section, an overview of the construction features of adobe buildings in LAC has been given. To provide more in-depth insights, in particular concerning the structural details, the outcomes of an in-field survey carried out in the historical center of Cusco are presented in the following as a meaningful case study. The historical center of Cusco, awarded as UNESCO World Heritage Site in 1983, has been already considered as a case study for large-scale seismic vulnerability assessment carried out by the authors (Brando et al., 2019), through the implementation of an empirical method calibrated on some Italian historic centers (Cocco et al., 2019; Brando et al., 2017). This case study is interesting for its cultural heritage and the identifiable structural typologies, which are the results of the complex urban development of the city (Gutierrez et al., 1981). In Section 3.2, the urban area current layout highlights how a typical valuable historical center made of adobe buildings in a seismic prone area developed during the times. Then, vertical (Section 3.3) and horizontal (Section 3.4) structures are shown and a discussion about their strengths and weaknesses is provided.
The historical evolution of Cusco dates back to the ancient Inca Empire (14th-16th century). It goes through the Spanish colonial (17th-18th century) and the Republican period (19th-20th century) up to the present day (Aedo, 2001). Although the influence of the succeeding masteries strongly influenced the current layout of the city and, in particular, of the historical center, important telluric events that occurred over the years had a relevant impact on how the city developed. One of the most severe events was the 1950 earthquake. A substantial reconstruction plan was drawn up, leading to the demolition of several adobe buildings, which were replaced with reinforced concrete structures (Calvo and Huerta, 2013). On the contrary, buildings with few damage were not interested by the application of structural interventions. Today, these structures feature severe decay signs on load-bearing vertical walls and horizontal diaphragms.
The authors’ urban survey focusing on adobe dwellings showed that these buildings present similar structural configurations. Indeed, the urban plan of Cusco is characterized by several groups of clustered structures forming isolated blocks of buildings surrounded by roads, called “quadra.” The elementary unit of the “quadra” is called “lote,” and it is generally composed of various structural units placed side by side to create an internal courtyards (Figure 5).
This is the typical configuration of the so-called “casa cusqueña,” dating back to the Spanish colonial period, which recalls the ancient kancha incaica of the previous historical period of the Inca empire. There are many similarities between the kancha of the Inca period (Figure 6A) and the casa cusqueña of the Spanish period (Figure 6B) (Gutierrez et al., 1981). Both are characterized by three or four buildings with a rectangular plan gathered around a central square-shaped patio. Apart from allowing the entry of air and light, it represented a space for social integration. The only difference between the kancha incaica and the casa cusqueña is the relationship between the various structural units: in the kancha incaica, houses were not contiguous to each other, as shown in (Figure 6A); while in the casa cusqueña of the Spanish period, the continuity between one house and another leads to the creation of a closed core from the outside, which opens to the internal patio through a single entrance (Figure 6B). In both cases, an alternation between empty and full spaces is created. Although these buildings may seem like a single structural body, they result from an assembly between different structural units, each characterized by a structural behavior that can be considered independent from the others, at least for gravity loads, and presenting a simple rectangular plan with internal orthogonal walls to divide different living spaces.
FIGURE 6. The adobe building organization of Cusco (A) during the Inca Empire (kancha incaica) (Source: Aedo 2001), and (B) during the Spanish colonization (casa cusqueña).
About the construction system, the Incas and the Spanish colonists made extensive use of stone and adobe blocks, which are, still today, the most used materials in Cusco, as shown in Figures 7A,B with a thematic maps created through the Software QGis 2.18.13 (Qgis User Guide, 2019). The data shown in Figure 7A were collected during a survey campaign carried out by the authors and confined to the core of the historic center, that is, included in the Cusco UNESCO World Heritage Site. As shown in Figure 7B, out of a total of 840 dwellings, 72% of the buildings in the historic center of Cusco are vernacular adobe buildings.
FIGURE 7. (A) Territorial distribution of the main structural typologies in the historical center of Cusco and (B) its frequencies.
Nowadays, Peruvian adobe buildings should feature limit of the height depending on the seismic zones (Reglamento Nacional de Edificaciones (RNE) del Ministerio de Vivienda Construcción y saneamiento, 2006): for buildings belonging to the historic center of Cusco, located in the seismic zone 2, a maximum of two floors is prescribed. Most of the observed buildings complies with this limitation, as shown in the thematic map created with the software QGis 2.18.13 (Qgis User Guide, 2019) and the relative frequencies reported in Figures 8A,B, respectively. However, sometimes, three stories buildings can be observed since the City Master Plan contemplates the possibility of having the third floor for a maximum building height of 10.00 m (Municipalidad del Cusco and Instituto Nacional de Cultura, 2018).
FIGURE 8. (A) Territorial distribution of the number of stories and (B) relative frequencies in the historical center of Cusco.
As observed in Section 2 for a general building in LAC, also in Cusco the first floor hosts a living area, with a kitchen, dining room, and, sometimes, a small shop, while the upper floors host the sleeping area, accessible by external wooden stairs (Aedo, 2001).
Vertical adobe walls feature a minimum thickness of 40cm, corresponding to the maximum dimension of the molds commonly used to produce single adobe blocks. In particular, the most used molds in Peru present a parallelepiped shape, with dimensions 43 × 43 × 11 cm (called Full adobe) or 43 × 22 × 11 (called ½ adobe). These molds are used to produce blocks that, once dried, become smaller: 40 × 40 × 10 cm and 40 × 20 × 10 cm, respectively. The blocks assembly varies according to the type of intersection between the orthogonal walls, which can be L, T, or cross-shaped intersections, as shown in Figure 9. To create these joints square blocks were mainly used, with rectangular blocks for the corners (Neumann and Blondet, 2010). In case of earthquake, these connections, if well realized, should a mitigation of the overturning mechanisms. However, some vertical cracks detected on some adobe structures in Cusco (Figure 10A) highlighted that these connections are often ineffective and that walls have been built detached, one adjacent to the other.
FIGURE 10. Deficiencies of adobe walls. (A) Typical cracks located at the corners of an adobe wall in Cusco due to the lack of connections between transversal walls. (B) An inappropriate mortar application in a typical adobe wall.
For adobe constructions, mortar should be wrapped as a plastic compound, easily spreadable, to ensure homogeneity to the wall. Therefore, the use of a wet mortar should be avoided because it would cause shrinkage in the drying phase with the consequent loss of friction between blocks, this leading to a detrimental effect on both the in-plane strength and stability of the entire wall. For adobe masonry, the clay-based mortar, made of the same compound used for the adobe blocks, is considered the most suitable and the most affordable from the economic point of view. It usually has physical and mechanical properties like compressive strength, shrinkage, adhesion, and erosion equal to those of adobe blocks. Its use allows avoiding issues related to the lack of compatibility between materials, which usually is one of the causes of the wall deterioration for masonry structures. However, for adobe buildings, to avoid cracks due to shrinkage, a certain amount of straw should be added to the mortar mix, and joints with a thickness ranging from 1.00 to 2.00 cm should be usually realized (Aedo, 2003). These recommendations have been rarely applied in Cusco, as shown in Figure 10B, where a recurring situation for a typical adobe wall, made of blocks assembled with very thin layers of mortar, moreover only in the horizontal direction, is depicted. The insufficient quantity of mortar led to the formation of voids with consequent blocks cracking.
During the construction of an adobe building, the position of openings should be known in advance and any subsequent changes should be avoided. Furthermore, some general rules should be followed to avoid a consistent decrement of the wall strength (Houben, 1994): the openings must be distributed as much as possible, avoiding their concentration in a single area; they must not be too wide (less than 1.20 m), and they must be located on the façade, observing the correct distances with the piers of the wall and with other openings. In addition to these general rules of good construction, the Peruvian Code provides more detailed descriptions shown in (Figure 11A): the maximum admissible width 1) for doors and windows must be 1/3 of the wall length (L), and their distance from the piers of the buildings at least three times the wall thickness (e).
FIGURE 11. (A) Recommendations of the Peruvian Code (Ministerio de Vivienda, Construcción y Saneamiento, 2017) for the correct position of openings, in comparison with (B) the distribution of the openings on the façade of a typical adobe dwelling in Cusco.
In Cusco, façades with not centered openings are recurrent. Moreover, the distances between the openings and the piers of the building are often not sufficient and not proportional to the wall thickness. Figure 11B, showing a building in the historic center of Cusco, highlights how the openings are all concentrated on two areas of the façade, and they are scattered along with the building height. Furthermore, considering that the wall thickness (e) is about 0.60 m, the opening in the upper right should be placed at least 1.80 m (3e) away from the pier, but it is clear that this limit is not complied with. On the contrary, the minimum distance of 1.00 m between one opening and the others is respected. Irregularity in the openings arrangement and their poor spacing from the piers can led to the formation of important cracks due to the activation of in-plane shear mechanisms, as shown in Figure 12.
To avoid the wall overturning under the action of external forces, the Peruvian Code recalls the importance of buttresses that act as vertical reinforcement elements. They must have a thickness (e0) bigger than that of the wall (e) (Figure 11A). In Cusco, these structural elements are common and applied at a large extent; however, they do not have a well-defined geometry. As shown in Figure 13A, they consist of a simple enlargement of the wall near the base to ensure more excellent stability to the structure.
FIGURE 13. Construction details in Cusco. (A) Traditional enlargement of the stone wall at the bottom to act as a buttress and (B) Typical wooden lintels.
Even if there are no specific recommendations in the Peruvian Code, good construction practice involves the use wooden frames and wooden lintels (Houben, 1994), as widely observed in Cusco. The choice of using wood is linked to the thermal expansion coefficient of this material, which is similar to that one of the earth used for the masonry. Metal elements would cause, on the contrary, different thermal expansion coefficients and, most importantly, would be prone to corrosion problems due to the moisture in the adobe blocks. As for the wooden lintels, they usually present a rectangular cross section (0.20 × 0.10 m) and have been conceived for allowing the load transmission near openings. For this purpose, it is essential that both the ends penetrate inside the wall for a length of at least 0.25 m. However, the most suitable material for these structural elements would be stone. However, since it is not readily available and expensive, it is reserved only for public or prestigious buildings. It is replaced by wood for ordinary buildings, as shown in Figure 13B.
Generally, adobe walls are susceptible to capillary rising damp and, for this reason, the foundation systems extend beyond the street level. This extension is known as “sobrecimiento,” and it shows the material composition of foundations, mainly made by cyclopean concrete (Figure 14A) or stones assembled with cement mortar (Figure 14B).
FIGURE 14. Traditional foundation system in Cusco, made with (A) cyclopean concrete or with (B) stone masonry and cement mortar.
The Peruvian Code (Ministerio de Vivienda, Construcción y Saneamiento, 2017) recommends foundations with a depth and a minimum width of 0.60 cm and extensions above the street level for at least 0.30 m (Figure 15A). Foundation systems observed on adobe buildings in Cusco comply with these requirements. Most of them presents stone foundation systems, with a minimum thickness of 0.60 m and an elevation above the street level ranging from 1.00 to 2.00 m (Figure 15B). Of course, the elevation of the “sobrecimiento” varies proportionally to the inter-story height: buildings with an inter-story height from 2.00 to 3.00 m present foundations 1.00 m high; buildings with an inter-story height greater than 3.50 m present foundations rising to 2.00 m above street level.
FIGURE 15. Foundation systems: (A) Requirement of the Peruvian Code (Ministerio de Vivienda, Construcción y Saneamiento, 2017); (B) A typical foundation system of an adobe structure in Cusco.
The Peruvian Code recommends wooden horizontal floors for adobe buildings in seismic areas. They shall be well anchored to the wall using ring beams to distribute the load on the supporting walls, avoiding concentrated forces.
These ring beams, named “viga collar,” should be made of materials compatible with the earth-based walls, like eucalyptus or wood, and all floors and roofs supporting beams should converge on them. In adobe buildings, floor slabs can span 3.00–4.00 m, and they can be composed of circular or squared wooden beams. Circular beams may have a diameter ranging from 0.17 to 0.20 m, while the squared ones have usually sections 0.20 x 0.20 m (Asociación Columbiana de Ingeniería Sísmica, 2004). They generally have a spacing of about 0.50 m. The planking level may be composed of overlapping bamboo canes and earth or simple wooden planks studded to the underlying beams (Figure 16A). Indeed, in Cusco, floor slabs are generally composed of circular beams characterized by an irregular shape, placed at a distance of about 0.40 m from each other and with wooden planks 3—5 cm thick, as shown in Figure 16B. However, in most cases, these circular beams come out from the adobe walls and are visible on the façade. Most likely, this is a constructive expedient to make the beams able to work as tie rods, but the lack of anchor plates allows them to slip off from the wall when severe earthquakes occur. Furthermore, this constructive expedient suggests that no ring beams are present to restrain the beam at the end.
FIGURE 16. Typical wooden floors of adobe buildings. (A) Construction details for a correct arrangement of the floor beams compared to (B) the typical wooden floor configuration in Cusco.
Adobe building roofs can have different configurations: flat or sloping, with one or two pitches and a supporting structure made of eucalyptus or wooden beams, assembled by nails and ropes. The most common type of roof for multi-story adobe buildings is the so-called “cubierta par y nudillo.” This roof configuration takes its name from its components: the “par” are the two slope beams that act as struts and give the proper inclination to the roof. The “nudillo” is the horizontal element, placed under the mid-section of the sloped beams, with the aim of mitigating their bending under the roof weight. The struts converge on the “viga hilera” in the upper part and on the “viga collar” at the bottom. Both are beams placed in the longitudinal direction to the trusses, and they represent the ridge beam and the ring beam of the roof, respectively. Below them, there are the so-called “viga tirante” that can keep together the structural roof system acting as tie rod (Figure 17A) (Argumedo et al., 1992).
FIGURE 17. Typical roof system “par y nudillo.” (A) Structural components of the considered roof system (Source: Glosario Ilustrado de arte arquitectónico, https://www.glosarioarquitectonico.com/glossary/par-y-nudillo/) and (B) its actual configuration on an adobe structure in Cusco.
In Cusco, the most common roof configuration is the gabled one, with a sloping ranging from 22° to 25°, as recommended by the City Master Plan (Municipalidad del Cusco and Instituto Nacional de Cultura, 2018). Its most recurrent load-bearing structure has the configuration mentioned above, created with circular wooden beams. Trusses are located every 0.50 m from each other, and a lower tie beam transversally connects the two opposite walls of the building every 2.00 m (Figure 17B). Furthermore, according to the traditional construction practice (Figure 18A), as observed during thesurvey carried out in Cusco (Figure 18B), the roofing system is composed of different layers: the first layer of bamboo canes called “tendido de caña”, placed transversally to the trusses, a 5–10 cm thick second layer composed by rammed earth, able to isolate the roof from water infiltrations, and a final layer with roof tiles. The lightness of these horizontal wooden slabs and their low in-plane stiffness certainly increase the structural vulnerability of Peruvian adobe buildings. Anyway, Figure 18B shows that one of the main problem is the low bending stiffness of the plank, which provokes undesired flexural deformation of the covering system.
FIGURE 18. The most common roofing system in adobe buildings: (A) description of its main components and (B) a representative example found in Cusco.
This review paper aimed at deepening the knowledge on construction techniques of Peruvian residential buildings in adobe, in order to provide information to be spent for vulnerability analyses at the urban scale. After an overview on most used adobe construction techniques in Latin America and the Caribbean, the attention was focused on the Peruvian city of Cusco, whose historic center was entirely investigated by the authors.
The main conclusions of the study can be outlined as follows:
⁃ Adobe buildings are widely present in many parts of the world, especially in areas with a high seismic risk, where seismic risk mitigation actions are urgent to avoid irremediable losses;
⁃ Although some countries have new Building Codes to design earthquake-resistant adobe buildings and there have been numerous studies to define sustainable intervention techniques, the number of unreinforced adobe buildings is still very high. Most of them are non-engineered structures erected without any preliminary design and based on the construction knowledge of the local inhabitants.
⁃ Regardless of the geographical area in which they are located, the structural deficiencies they present are very similar: the lack of ring beams that guarantee a correct distribution of horizontal forces between the walls, the lack of good connections between orthogonal walls, the presence of openings not correctly distributed along the façades, are just some of the main problems observed;
⁃ Although adobe is a low-cost and sustainable material, it has often been replaced by reinforced concrete in recent years. Some countries have even banned the construction of adobe buildings due to their high vulnerability. Therefore, unless the vulnerability of these structures is reduced and the population is made aware of the importance of their conservation, these structures will disappear.
All these considerations highlight the urgency of adopting seismic risk mitigation measures. The structural deficiencies highlighted and the construction techniques studied in this paper represent the starting point for multi-level seismic vulnerability analyses on adobe residential buildings, which the authors are carrying out. These analyses will define those structural interventions that, if implemented on a large scale, will allow significant seismic risk mitigation of historical centers in LAC presenting features that are similar to the ones observed in the city of Cusco.
All authors listed have made a substantial, direct and intellectual contribution to the work, and approved it for publication.
The authors declare that the research was conducted in the absence of any commercial or financial relationships that could be construed as a potential conflict of interest.
All claims expressed in this article are solely those of the authors and do not necessarily represent those of their affiliated organizations, or those of the publisher, the editors and the reviewers. Any product that may be evaluated in this article, or claim that may be made by its manufacturer, is not guaranteed or endorsed by the publisher.
The Supplementary Material for this article can be found online at: https://www.frontiersin.org/articles/10.3389/fbuil.2022.974005/full#supplementary-material
Aedo, W. C. (2001). Vivienda urbana popular de adobe en el Cusco, Perú. Asentam. humanos medio Sociocult. 50, 48.
Aedo, W. C. (2003). Anti-seismic construction handbook. Villefontaine Cedex, France: CRATerre, Maison Levrat, Parc Fallavier.
Alemán, A. (2017). Manual para la construcción y mantenimiento de vivienda. Editor A. Stein Heinemann, S. Bartels, and E. Gaviria (Tegucigalpa, Honduras).
Argumedo, R. S., Ochoa, J. A. F., and Arce, E. K. (1992). La casa de la calle tigre : Recuperación y puesta en valor de una casa del Cusco. Cusco: Universidad Nacional de San Antonio Abad del Cusco.
Aroquipa, H., and Hurtado, A. (2022). Seismic resilience assessment of buildings: A simplified methodological approach through conventional seismic risk assessment. Int. J. Disaster Risk Reduct. 77, 103047. doi:10.1016/j.ijdrr.2022.103047
Asociación Columbiana de Ingeniería Sísmica (2004). Manual para la rehabilitación de viviendas construidas en adobe y tapia pisada.
Blondet, M., Vargas, J., and Tarque, N. (2008a). “Available low-cost technologies to improve the seismic performance of earthen houses in developing countries,” in Proceedings, 14th World Conf. Earthq. Eng. Available at: ftp://jetty.ecn.purdue.edu/spujol/Andres/files/09-02-0001.PDF.
Blondet, M., Vargas, J., and Tarque, N. (2008b). “Low-cost reinforcement of earthen houses in seismic areas,” in Proc. 14th World Conf. Earthq. Eng, Beijing, China. Available at: c:%5CUsers%5CUsuario%5CDesktop%5CPhD%5CPapers%5CRetrofitting%5CBlondet M. et al. - Low-cost reinforcement of earthen houses in seismic areas.PDF.
Blondet, M., Svetlana, B., and Loaiza, C. (2019). “El portal de la Enciclopedia Mundial de las construcciones de vivienda en zonas sismicas,” in XIV congreso nacional de Ingenieria civil - iquitos.
Brando, G., De Matteis, G., and Spacone, E. (2017). Predictive model for the seismic vulnerability assessment of small historic centres: Application to the inner Abruzzi Region in Italy. Eng. Struct. 153, 81–96. doi:10.1016/j.engstruct.2017.10.013
Brando, G., Cocco, G., Mazzanti, C., Peruch, M., Spacone, E., Alfaro, C., et al. (2019). Structural survey and empirical seismic vulnerability assessment of dwellings in the historical centre of Cusco, Peru. Int. J. Archit. Herit. 15, 1395–1423. doi:10.1080/15583058.2019.1685022
Bruneau, M., Chang, S. E., Eguchi, R. T., Lee, G. C., O’Rourke, T. D., Reinhorn, A. M., et al. (2003). A framework to quantitatively assess and enhance the seismic resilience of communities. Earthq. Spectra 19, 733–752. doi:10.1193/1.1623497
Calvo, R., and Huerta, R. (2013). El Centro Historico del Cusco: Consideraciones para la renovación de su gestión. Cult: Cusco Minist.
Charleson, A. (2011). Seismic strengthening of earthen houses using straps cut from used car tires: A construction guide. Oakland, CA: Earthquake Engineering Research Institute.
Charvériat, C. (2012). Natural disasters in Latin America and the caribbean: An overview of risk. SSRN J. 434. doi:10.2139/ssrn.1817233
Chieffo, N., Mosoarca, M., Formisano, A., Lourenço, P. B., and Milani, G. (2021). The effect of ground motion vertical component on the seismic response of historical masonry buildings: The case study of the Banloc Castle in Romania. Eng. Struct. 249, 113346. doi:10.1016/j.engstruct.2021.113346
Chieffo, N., Formisano, A., Landolfo, R., and Milani, G. (2022). A vulnerability index based-approach for the historical centre of the city of Latronico (Potenza, Southern Italy). Eng. Fail. Anal. 136, 106207–106219. doi:10.1016/j.engfailanal.2022.106207
Cocco, G., D’Aloisio, A., Spacone, E., and Brando, G. (2019). Seismic vulnerability of buildings in historic centers: From the “urban” to the “aggregate” scale. Front. Built Environ. 5, 1–14. doi:10.3389/fbuil.2019.00078
Comités Nacionales de Reglamentación Técnica Reglamento técnico Salvadoreño (2020). Urbanismo y construcciòn en lo relativo al uso del sistema constructivo de adobe para viviendas de un nivel. San Salvador, El Salvador: Organismo Salvadoreño de Reglamentación Técnica.
Despang, H. M. (2014). Manual de diseño sismo-resistente simplificado para Guatemala. Guatemala: AGIES Asociación Guatemalteca de Ingeniería Estructural y Sísmica, 1–158.
Diego, L., Migoya, D., and Beltetón, O. F. (2022). A first approach to the estimation of seismic damage in Guatemala city : A seismic exposure model Bullettin of Earthquake Engineering, 1–25.
Dominik, L., Holliday, L., Omar, G., and Beleton, F. (2004). World housing Encyclopedia. Guatemala: World Hous.
Gerardo Araya, L., Duy, E., Reidel, Ú., Kunze, S., Burbano, C., and Saavedra, E. (2019). “Bloques de adobe reforzados transversalmente con plàstico de bottellas recicladas,” in 15° congreso internacional de Patologìay recuperaciòn de Estructuras Salta, Argentina.
Güney, D., Kuruşcu, A. O., and Arun, G. (2016). Damage evaluation of masonry buildings after van earthquakes in 2011. Int. J. Archit. Herit. 10, 269–280. doi:10.1080/15583058.2015.1113343
Gutierrez, R., De Azevedo, P., Viñuales, G. M., Azevedo, E. D., and Vallin, R. (1981). La casa cusqueña. Argentina: Departamento de Historia de la Arquitectura Universidad Nacional de Nordeste.
Houben, H. (1994). Earth construction. A comprehensive guide. London: Intermediate Technology Publications.
Illampas, R., Charmpis, D. C., and Ioannou, I. (2015). Non-linear dynamic finite element analysis of adobe masonry structures with various roof diaphragm configurations. Seismic Assess. Behav. Retrofit Herit. Build. Monum., 475–487. doi:10.1007/978-3-319-16130-3
INPRES INTI and CIRSOC (2018). Reglamento Argentino para construcciones sismorresistentes, Parte III, Construcciones de Mamposterìa. Available at: http://library1.nida.ac.th/termpaper6/sd/2554/19755.pdf.
Instituto Uruguayo de Normas Tecnicas (2012). Proyectos de construcciòn- desarrollo del proyecto de arquitectura. Montevideo, Uruguay: Instituto Uruguayo de Normas Tècnica.
Kuroiwa, J., Deza, E., and Jaen, H. (1973). “Investigations of the Peruvian earthquake of may 31, 1970,” in Proceedings, Fifth World Conference on Earthquake Engineering Rome, 447–457.
Kuruscu, A. O., Güney, D., and Arun, G. (2014). Seismic behaviour of vernacular masonry buildings during 2010 and 2011 earthquakes in Turkey. Int. Mason. Conf., 1–10.
Lopez, M. A., Bommer, J., and Benavidez, G. (2004). World housing Encyclopedia, El Salvador. World Hous. Encycl.
Lourenço, P. B., Silva, R. A., and Oliveira, D. V. (2018). Historic and modern earthen structures: Challenges and recent research. Proc. Int. Mason. Soc. Conf. 0, 1–28.
Lourenco, P. B. (1996). Computational strategy for masonry structures. Delft, Netherlands: Delf University Press.
Ministerio de Cultura - Proyecto Qhapaq Ñan Sede Nacional (2019). Guía metodológica de conservación 1 - materiales de Construccion y Morteros. Lima, Peru: Ministero de Cultura del Perù.
Ministerio de obras publicas (2014). Reglamento estructural de Panamà. Available at: https://www.gacetaoficial.gob.pa/pdfTemp/28090_A/GacetaNo_28090a_20160805.pdf.
Ministerio de Transporte e Infraestructura (2015). Nueva cartilla de la construcciòn. Available at: http://library1.nida.ac.th/termpaper6/sd/2554/19755.pdf.
Ministerio de Vivienda, Construcción y Saneamiento (2017). NORMA E.080 Diseño y construcción con tierra reforzada. El Peru, 29.
Ministero de desarrollo economico viceministerio de desarrollo (2019). Normas Tecnicas de vivienda Republica de Bolivia.
Municipalidad del Cusco and Instituto Nacional de Cultura (2018). Plan Maestro del centro histórico del Cusco. Cusco, Peru: Gobierno Municipal del Cusco, Gerencia de centro historico.
Neumann, J., and Blondet, M. (2010). Casas sismorresisitentes Y saludables de adobe reforzado con cuerdad. Lima, Peru: Pontificia Universidad Católica del Perú PUCP and Servicio Nacional de capacitation para la industria de la construccion (SENCICO), 64.
Organization of American States. Department of Regional Development and Environment, Organization of American States. Natural Hazards Project (1990). Disaster, planning and development: Managing natural hazards to reduce loss, United States. Agency for international development. Office of foreign disaster assistance. U. S. A. for I. D. O. of F. D. A. Washington, DC: The Department United States.
Papanikolaou, A., and Taucer, F. (2004). Review of non-engineered houses in Latin America with reference to building practices and self-construction projects, 228. Available at: http://bookshop.europa.eu/en/review-of-non-engineered-houses-in-latin-america-with-reference-to-building-practices-and-self-construction-projects-pbLBNA21190/.
Reglamento Nacional de Edificaciones RNE del Ministerio de Vivienda Construcción y saneamiento (2006). Ministerio de Vivienda. Construcción Saneam. 53, 1689–1699. doi:10.1017/CBO9781107415324.004
Spacone, E., Brando, G., Peruch, M., Mazzanti, C., Sovero, K., and Tarque, N. (2019). An extensive survey of the historic center of Cusco for its seismic vulnerability assessment. RILEM Bookseries 18, 1257–1267. doi:10.1007/978-3-319-99441-3_135
Tarque, N., Crowley, H., Pinho, R., and Varum, H. (2012). Displacement-based fragility curves for seismic assessment of adobe buildings in Cusco, Peru. Earthq. Spectra 28, 759–794. doi:10.1193/1.4000001
Tarque, N., Camata, G., Spacone, E., Varum, H., and Blondet, M. (2014). Nonlinear dynamic analysis of a full scale unreinforced adobe model. Earthq. Spectra 30, 1643–1661. doi:10.1193/022512EQS053M
Tocchi, G., Polese, M., Di Ludovico, M., and Prota, A. (2022). Regional based exposure models to account for local building typologies. Netherlands: Springer.
Vargas-Neumann, J., Torrealva, D., and Blondet, M. (2007). Building hygienic and earthquake-resistant adobe houses using geomesh reinforcement. Available at: http://www.world-housing.net/wp-content/uploads/2011/06/Adobe-Geomesh-Arid_Tutorial_English_Blondet.pdf.1
Vargas, J., Bariola, J., Blondet, M., and Mehta, P. K. (1986). Seismic strength of adobe masonry. Mater. Struct. 19 (4), 253–258.
Velasquez, C. A. (2004). Matias hube ginestar, felipe rivera jofre, hernan santa maria oyandenel, and david hernandez JaraWorld housing Encyclopedia, Chile. World Hous. Encycl. 1–21.
Viñuales, G. M., Martins Neves, C. M., Flores, M. o., and Ríos, L. S. (2003). Arquitecturas de Tierra en Iberoamérica. Available at: http://www.caminosostenible.org/wp-content/uploads/BIBLIOTECA/Arquitectura de Tierra en Iberoamerica.pdf%0Ahttps://www.scribd.com/document/227291144/Arquitectura-de-Tierra-en-Iberoamerica-pdf.
Keywords: seismic vulnerability, adobe, construction techniques, cusco, vernacular dwellings
Citation: Cocco G, Brando G and Spacone E (2022) A review of local construction practices applied on unreinforced adobe buildings in South America. Front. Built Environ. 8:974005. doi: 10.3389/fbuil.2022.974005
Received: 20 June 2022; Accepted: 16 August 2022;
Published: 28 September 2022.
Edited by:
Ana Mafalda Matos, University of Porto, PortugalReviewed by:
Deniz Güney, San Diego State University, United StatesCopyright © 2022 Cocco, Brando and Spacone. This is an open-access article distributed under the terms of the Creative Commons Attribution License (CC BY). The use, distribution or reproduction in other forums is permitted, provided the original author(s) and the copyright owner(s) are credited and that the original publication in this journal is cited, in accordance with accepted academic practice. No use, distribution or reproduction is permitted which does not comply with these terms.
*Correspondence: Giulia Cocco, Z2l1bGlhLmNvY2NvQHVuaWNoLml0
Disclaimer: All claims expressed in this article are solely those of the authors and do not necessarily represent those of their affiliated organizations, or those of the publisher, the editors and the reviewers. Any product that may be evaluated in this article or claim that may be made by its manufacturer is not guaranteed or endorsed by the publisher.
Research integrity at Frontiers
Learn more about the work of our research integrity team to safeguard the quality of each article we publish.