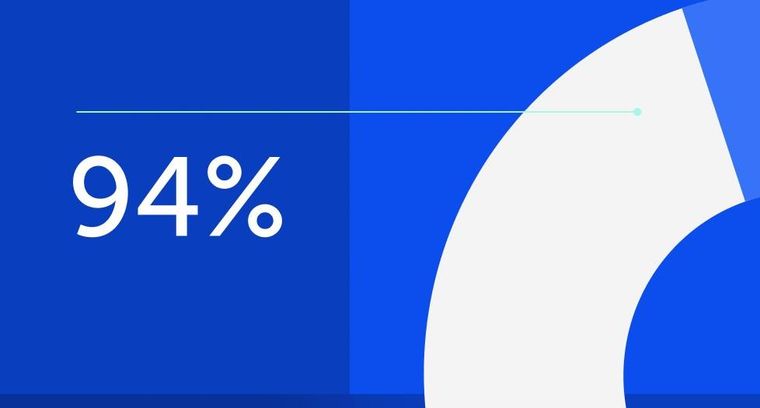
94% of researchers rate our articles as excellent or good
Learn more about the work of our research integrity team to safeguard the quality of each article we publish.
Find out more
ORIGINAL RESEARCH article
Front. Built Environ., 14 June 2022
Sec. Geotechnical Engineering
Volume 8 - 2022 | https://doi.org/10.3389/fbuil.2022.864421
This article is part of the Research TopicCharacterization of expansive soils and mitigation of swelling phenomenaView all 3 articles
Pile foundations are widely used in all types of soils including expansive and collapsible soils that are typically found in nature in a state of unsaturated condition. Opposite trends in volume change behavior are typically observed when water infiltrates into expansive and collapsible soils. The design of pile foundations in these soils is conventionally based on saturated soil mechanics assuming drained conditions ignoring the influence of unsaturated conditions. In this study, infiltration tests were performed on two model piles; one of these piles was tested in an expansive soil and the other in a collapsible soil. The focus of this study was to determine the pile mechanical behavior including the pile head load, displacement response, pile axial force, shaft friction, and pile base pressure taking account of suction changes associated with water infiltration. The pile mechanical behavior is significantly influenced due to changes in the pile shaft friction in both expansive and collapsible soils. The responses of the pile mechanical behavior variations were explained extending the concepts of unsaturated soil mechanics. The results of this study provide valuable information for the practicing engineers to gain a more comprehensive understanding of mechanical behaviors of pile foundations in expansive soils and collapsible soils upon water infiltration.
Expansive soils and collapsible soils are examples of problematic soils that are typically found in a state of unsaturated condition in nature. These soils undergo significant volume changes in response to water content changes. Expansive soils are highly plastic in nature due to the presence of active clay minerals such as the montmorillonite that undergo significant volume expansion when they imbibe water (Shi et al., 2002; Liu and Vanapalli 2021). However, due to the weak bonds between particles, collapsible soils undergo rapid volume reductions when they are inundated with water (Tadepalli and Fredlund 1991; Pereira and Fredlund 2000; Lim and Miller 2004; Noor et al., 2013). Pile foundations are preferred in comparison to other alternatives both in expansive and collapsible soils. Piles that extend beyond weak soil layers and rest on rigid bedrock or a stable soil stratum assure not only a relatively high bearing capacity and stiffness but also limit differential settlements. The use of these foundations also ensures stability to the super structure when the shallow soil layers likely lose most of their shear strength and stiffness associated with water infiltration. Due to these advantages, pile foundations are widely chosen in practical applications to combat the problems associated with the problematic behaviors associated with expansive soils and collapsible soils.
In many scenarios, piles are extended below the active zone depth (i.e., the depth of the expansive soil layer in which the moisture content changes is sensitive to environmental factors associated with infiltration and evaporation) with their ends placed on the bedrock. If bedrock is not available at a shallow depth, the pile end is extended into soil layers below the active zone depth with relatively higher stiffness. In other words, mechanical behavior of the soil with the pile end placed on the bedrock is not significantly influenced by the seasonal water content changes. The variations in the load transfer mechanism of piles associated with water infiltration are predominantly in the shaft friction, particularly within the active zone depth. The shaft friction in the active zone is influenced by four key factors that include, net normal stress (lateral earth pressure), suction, interface shear strength properties, and the pile-soil relative displacement.
As shown in Figure 1B, there is significant positive friction that arises along the entire length of the pile to carry the pile head load together with the pile end resistance prior to water infiltration. However, when water infiltrates into the active zone, both suction reductions and suction reduction-induced soil volume changes that occur influence the pile’s mechanical behaviors significantly. For such a scenario, the mobilized lateral swelling pressure acts as an additional increment to the lateral earth pressure, as shown in Figure 1A. The pile-soil shear strength properties at the interface decrease with a decrease in soil suction. The pile-soil relative displacement is likely to uplift the pile due to ground heave. Once the pile is uplifted due to an increase in positive friction in the active zone, negative friction arises in the active zone.
FIGURE 1. Load transfer mechanism variations of piles in unsaturated problem soils with significant volume changes upon infiltration. (A) Expansive soil after infiltration. (B) Prior to infiltration. (C) Collapsible soil after infiltration.
The typical behavior of piles in collapsible soils is shown in Figure 1C. Similar to the behavior of piles in expansive soils, the interface shear strength properties decrease with a reduction in suction associated with water infiltration. The ground settlement due to soil collapse contributes to the downward movement of the soil relative to the pile. Due to this reason, negative friction generates in the active zone. Therefore, both the positive friction in the stable zone and pile base pressure increase to balance the additional load contribution from the negative friction.
In conventional soils, the focus of pile load tests is directed toward the determination of the ultimate bearing capacity and its settlement behavior. However, for pile tests in expansive soils and collapsible soils, more attention is required to understand their variations in the mechanical behavior associated with water infiltration. In other words, the influence of water infiltration on the load transfer mechanisms of piles should be well understood. More attention has been directed in the literature toward understanding the uplift movement of the pile and/or the uplift force generated in the infiltration process under zero pile head load (Overton et al., 2007; Xiao et al., 2011; Wu et al., 2012; Mendoza 2013; Soundara and Robinson 2017). However, there is limited information about the load transfer mechanism in piles during the infiltration process with respect to the pile axial force distributions, pile shaft friction distributions, and pile base pressure.
Various laboratory and field tests performed on piles in unsaturated collapsible soils suggest that the variations of the pile shaft friction are associated with water content changes and soil collapsibility coefficient (Al-Omari et al., 2017; Fattah et al., 2020). The positive friction in the active zone gradually decreases and eventually changes to negative friction as the wetting front moves downward. Such a phenomenon is particularly evident in soils with a high coefficient of collapsibility (Huang et al., 2007; Wang et al., 2010; Noor et al., 2013; Xing and Liu 2018). Few attempts were made to explore the mechanism responsible for such a behavior. The soil suction is a widely accepted key stress state variable that influences the unsaturated soil behavior; however, few studies are reported in the literature that investigate the pile behavior in collapsible soils taking account of the influence of suction (Kakoli 2011; Kakoli et al., 2011). Most of the studies reported in the literature discuss the behavior of soil around the pile in terms of water content variations.
In this study, considering the aforementioned shortcomings, laboratory studies were undertaken in unsaturated expansive and collapsible soils to understand the influence of suction changes associated with water infiltration on the mechanical behavior of model piles. The typically encountered stress and hydraulic boundary conditions during the service stage of the pile were applied while performing the pile infiltration tests. Real-time measurement on pile mechanical behaviors (including the pile axial force distribution and pile head displacement) and soil properties/behaviors (including soil displacement, water content, and suction) were performed for rigorous interpretation of the mechanical behavior variations in pile foundations. The mechanisms responsible for the pile mechanical behavioral changes in both the soils are interpreted extending unsaturated soil mechanics. In addition, experimental results of model pile tests in unsaturated expansive and collapsible soils were compared to illustrate the influence of soil volume deformation around the piles on the load transfer mechanism of piles.
The experimental settings including geometric dimensions, placement positions of various sensors, stress, and hydraulic boundary conditions of both tests are illustrated in Figure 2.
FIGURE 2. Experimental setup of the pile infiltration test. [(A) Expansive soil. (B) Collapsible soil].
For the pile infiltration test in unsaturated expansive soils, the model pile was made of an aluminum pipe with an outer diameter of 25.4 mm, a wall thickness of 3 mm, and a height of 600 mm. The ratio of the diameter of the testing tank to the diameter of the model pile was designed to be around 12. This ratio is considered satisfactory to alleviate the influence of boundary conditions on the pile load test results based on the pile test results in unsaturated soils (Fan 2007; Han et al., 2016). Pressure cells were located at the top and bottom of the model pile to record the pile head load and pile base pressure. More specifically, the pressure cell at the bottom of the pile is at a depth of around 400 mm. Five strain gauges were set 80 mm intervals from the pile bottom to record the pile axial force in the testing process. A fine sand layer of 150 mm thickness was placed at the bottom test tank. Expansive soil clay sample obtained from Regina, Canada, was mixed with a water content of 27% and compacted in 20 equal layers (each layer with a thickness of 20 mm) to achieve a wet density of 17.36 × 103 kg/m3 (i.e., dry density of 13.67 × 103 kg/m3). After each soil layer was compacted, the surface was scarified using a spatula to achieve a corrugated surface and facilitate in achieving a close contact between adjacent compacted soil layers. During the compaction process of various soil layers, a series of GS-3 volumetric water content sensors and MPS-6 suction sensors were set at various positions, as shown in Figure 2A. In the static loading stage, the ultimate bearing capacity of the model pile was estimated to be 1000 N using Eq. 1 from Chinese Technical Code for Building Pile Foundations (JGJ 94–2008).
where Quk is the ultimate single-pile bearing capacity; Qus is the ultimate pile shaft resistance; Qpk is the ultimate pile base pressure; u is the perimeter of the model pile; qsik is the standard pile shaft friction within the soil layer i; li is the layer thickness of the soil layer i; qpk is the standard pile base stress; and Ap is the pile base area.
Using a factor of a safety value of two, an allowable load of 500 N was applied on the pile head. Manual irrigation was performed for water infiltration into the test tank. It was ensured that the ground water level was a little higher than the top surface of the soil during the testing. Once the soil deformation stabilized and the water content sensor and the suction sensor indicated the soil has been fully saturated, manual irrigation was stopped.
A model test pile made of a hollow aluminum pipe with a diameter of 60mm, a wall thickness of 5 mm, and length 200 mm was used for loading it in unsaturated collapsible soil during the process of water infiltration. Similar to the model pile tested in expansive soil, the ratio of the diameter of the testing tank to the diameter of the model pile was designed to be around 20. Pressure cells were located at the top and bottom of the pile to monitor the pile head load and pile base pressure variations similar to the pile infiltration test performed in expansive soils. A total of 12 strain gauges were used that were at a distance of approximately 100 mm intervals from the bottom to the top of the pile to record the pile axial force during the testing process. Prior to performing the infiltration test, collapsible soil with a water content of 12% was compacted in 17 equal layers (each layer with a thickness of 100 mm) to achieve a wet density of 15.5 × 103 kg/m3 (i.e., dry density of 13.8 × 103 kg/m3). The positions of GS-3 volumetric water sensors and MPS-6 suction sensors buried inside the collapsible soil are given in Figure 2B. In the static loading stage, an allowable load of 800 N was applied on the pile head. The allowable load was determined by the static pile load test, which indicated the ultimate bearing capacity of the pile was 1600 N. Using a factor of a safety value of two, an allowable load of 800 N was determined.
In the infiltration stage, manual irrigation was performed, following the procedures summarized for expansive soil for saturating the collapsible soil.
The index properties of expansive soil and collapsible soil used in the pile infiltration tests are summarized in Table 1. The soil–water characteristic curves (SWCCs) of expansive soil and collapsible soil are shown in Figures 3A,B, respectively. More specifically, four methods were combined employed for the measurement of the entire SWCC (i.e., in the suction range of 0–106 kPa), namely, the chilled mirror hygrometer (WP4) method, pressure plate method, filter paper method, and desiccator method, while the SWCC of collapsible soil was collected through the filter paper method. The SWCCs shown in Figures 3A,B were fit using the Fredlund and Xing (1994) equation.
TABLE 1. Index properties of expansive soil and collapsible soil used in the pile infiltration test.
FIGURE 3. SWCC of expansive soil and collapsible soil used in the pile infiltration test. [(A) Expansive soil. (B) Collapsible soil].
A period of 40 h was required to saturate the expansive soil layer in the test tank with a thickness of 400 mm by manual irrigation. The void ratio increases rapidly due to the swelling of expansive soil, which contributes to a significant increase in hydraulic conductivity. By the time the infiltration test was completed, the top layer of expansive soil was close to slurry. Figure 4 shows the variations of the soil and pile head displacement during the pile infiltration test. In the static loading process, piles experienced a settlement of around 3 mm under the applied load of 500 N, while no noticeable soil settlement was detected in this process. After water was added by manual irrigation, expansive soil around the pile showed a gradual but continuous volume expansion in the vertical direction for the 40 h of testing. A total ground heave of 25 mm was measured during this period. However, during this period, the pile head settlement increased from around 3 mm to around 7 mm due to the influence of reduction in soil suction. Since the sand fill at the bottom of the pile was not influenced by water infiltration prior to performing the test, the pile head settlement can be attributed predominantly to the loss of the pile shaft friction.
Figures 5,6, respectively, summarize the variations of the volumetric water content and the suction changes with respect to time during the testing process. A strong correlation can be observed between the volumetric water content and suction changes and the development of the ground heave.
Figure 7 shows the variations of the pile base pressure during the period of testing. The pile base pressure gradually stabilized at a value of 34 N during static loading. However, during the water infiltration process, the pile base pressure grew rapidly to a value of around 183 N within 40 h. The pile base pressure increment suggests that there is loss in the pile shaft friction that can be attributed to the water infiltration process. The slight increment in the pile base pressure can be explained by the soil stress relaxation effect once the expansive soil was saturated.
The pile axial force distribution and pile shaft friction distribution at four specific time intervals (0, 10, 20, and 40 h) are summarized in Figures 8,9, respectively, to understand the pile load transfer mechanism. These four time intervals were specifically selected as significant changes in the volumetric water content and suction values can be observed from Figures 5,6. The experimental results summarized in Figure 8 suggest that during the infiltration process, more pile head load was gradually transferred to a greater depth of the pile and finally carried by the pile base. The reason for such pile load transfer mechanism changes can be explained by Figure 9. From 0 to 20 h, the pile shaft friction within the already saturated zone kept decreasing while the pile shaft friction in the unsaturated zone kept increasing. This behavior can be explained extending the principles of unsaturated soil mechanics, as following: suction reduction induced by water infiltration reduced the contribution of suction to the pile shaft friction. In spite of mobilization of lateral swelling pressure due to the suction reduction and additional normal stress acting on the pile-soil interface, there is a reduction in the pile shaft friction caused by suction reduction, which is more significant. Therefore, loss of the pile shaft friction in the saturated zone resulted in more load carried by the pile in the unsaturated zone and pile base. In addition, pile settlement increased due to the loss of the pile shaft friction that contributed to an increase in the pile-soil relative displacement. The pile-soil relative displacement due to static loading was lower than the critical value with reference to the peak shear strength at the interface prior to water infiltration. The pile-soil relative displacement increment however increases the pile-soil interface shear strength (shaft friction) toward the peak value. This can be a dominant cause for increase in the shaft friction of pile in the unsaturated zone at 10 and 20 h. However, when the entire expansive soil layer in the test tank with the model pile is saturated in 40 h, the contribution of suction to the pile shaft friction is completely lost. Due to this reason, there is a decrease in the peak interface shear strength that contributes to a pile settlement. Apart from the reduction in the peak interface shear strength, a further increase in pile head settlement may cause the pile-soil interface shear strength to reduce from the peak value to the post-peak value. Therefore, pile shaft friction distribution was observed to be a minimum value under saturated conditions.
Pile infiltration test in collapsible soil (PITC) required 48 h of time to achieve saturated conditions by water infiltration. Figure 10 shows the variations of the soil and pile head displacement during the pile infiltration test. The model test pile experienced a settlement of approximately 3 mm under the applied static load of 800 N. No noticeable soil settlement was detected during this process. The compacted soil in the test tank collapsed and resulted in ground settlement once water started infiltrating into the soil. A total ground settlement of around 57 mm was recorded by the end of the infiltration process. As illustrated in the introduction section, ground settlement contributed to a downward drag of the pile; due to this reason, negative friction developed, as shown in Figure 1C. This part of negative friction acted as an additional load on the pile. Consequently, the pile head settlement increased from 3 mm to around 17 mm during the infiltration process.
The volumetric water content and the soil suction changes during the testing process are summarized in Figures 11,12, respectively. After 20 h of water infiltration, the wetting front reached a depth of 1100 mm, which was the position of the pile base. Within 48 h, the collapsible soil of 1700 mm thickness saturated. Similar to expansive soil, a clear correlation can be observed between the volumetric water content or suction changes and the development of the ground settlement.
The pile base pressure variations are shown in Figure 13. The pile base pressure gradually grew from 100 N to around 700 N within only 48 h of water infiltration. The pile axial force and pile shaft friction distribution at three different time intervals (i.e., 0, 20, and 48 h) are summarized in Figures 14,15, respectively, to analyze the pile load transfer mechanism variations.
From Figure 14, it can be seen that the pile axial force distribution is linearly prior to water infiltration. Such a behavior suggests that a large pile axial force acts at the top compared to the bottom. The collapse deformation with the pile axial force showed close to a slant “D” shape distribution. The maximum pile axial force is approximately at the mid height of the pile. The pile axial force distribution pattern did not change during the infiltration process; however, the pile axial force increased with depth. Meanwhile, the position of the neutral point that indicated the maximum pile axial force shifted to a lower level as the infiltration process continued. In other words, the position of the maximum pile axial force is at a lower level.
Experimental results summarized in Figure 15 offer a better explanation for such a behavior. In the static loading stage, the positive friction was almost linearly distributed along the entire length of the pile. Negative and positive frictions were, respectively, generated in the upper and lower parts of the pile when the collapsible soil was saturated. In other words, more loads were carried by the positive shaft friction in the lower part of the pile and the pile base pressure. Furthermore, the magnitude of the negative friction in the upper part after infiltration is higher than the positive friction prior to infiltration. This is because the ground settlement has significantly increased the pile-soil relative displacement. On the one hand, the peak pile-soil interface shear strength decreased to a certain extent since the part of the pile shaft friction contributed by suction has vanished. On the other hand, the increasing pile-soil relative displacement has contributed to an increase in the pile-soil interface shear strength (pile shaft friction) from the initial value toward the peak value. Apparently, the pile shaft friction increment induced by the pile-soil relative displacement increment is more pronounced. Due to this reason, the magnitude of the negative friction increased compared to the positive friction prior to water infiltration.
From comparisons between the experimental results related to the variations of the load transfer mechanism of piles in unsaturated expansive and collapsible soils during the infiltration process, it can be summarized that suction variations and suction variation-induced volume changes are the two main reasons responsible for these changes. Immediately after the soil around the pile is saturated, the pile shaft friction would decrease from a theoretical stand point of view since there is no contribution arising from suction to the pile shaft friction. However, the suction variation–induced volume changes of expansive and collapsible soils would have different proportions of contributions toward the pile shaft friction arising from the other two influencing factors, namely, the net normal stress and pile-soil relative displacement. For expansive soil, swelling in the horizontal direction restricted by piles turns into lateral swelling pressure. The mobilized lateral swelling pressure adds an additional stress to the lateral earth pressure, which further increases the pile shaft friction. For both expansive and collapsible soils, soil volume changes in the vertical direction, which fundamentally change the magnitude and even directions of pile shaft friction due to the pile-soil relative displacement. Therefore, for piles in unsaturated expansive soils, upon infiltration the pile shaft friction in the active zone may either increase or decrease. While for piles in unsaturated collapsible soils, negative friction generates in the active zone due to water infiltration. As the pile shaft friction in the active zone changes, the pile load transfer mechanism would change correspondingly. For the two tests presented in this study, the soil volume change has a more predominant influence in comparison to the suction change. In other words, volume change behavior is a key parameter in influencing the pile mechanical behaviors in expansive and collapsible soils.
Load transfer mechanisms of piles in unsaturated soils with noticeable volume deformations (i.e., expansive soil and collapsible soil) would be significantly influenced by the water content or suction changes. In order to understand the mechanical behavioral changes in unsaturated expansive soils and collapsible soils that are typically encountered under in situ conditions, model pile tests were performed by simulating the conditions of water infiltration in a laboratory environment. Experimental results from this study are presented and analyzed in this article, extending the mechanics of unsaturated soils. The following conclusions derived are summarized as follows.
1) Pile shaft friction and the pile load transfer mechanisms are combined influenced by the suction variations and suction variation-induced volume changes of soils around the pile. For a pile in expansive soil with a service load, water infiltration causes a reduction in the pile shaft frictions due to two reasons. The pile shaft friction reduces due to the loss of suction associated with water infiltration, which is significant than the pile shaft friction increment associated with the mobilization of lateral swelling pressure. In addition, the pile-soil relative displacement increment increases the pile-soil interface shear strength (shaft friction) toward the peak value at a greater depth. However, pile-soil interface shear strength at shallow depth reduces from a peak value to the post-peak value. Due to this reason, more pile head load is gradually transferred to the pile at a greater depth, resulting in the increment of the pile base pressure and pile head settlement.
2) For a pile in collapsible soil with a service load, water infiltration contributes to the development of negative friction in the active zone. This is because the ground settlement due to soil collapse causes the soil to move downward relative to the pile. In spite of losing the contribution of suction to the pile-soil interface shear strength (shaft friction), the increasing pile-soil relative displacement significantly increases the magnitude of the negative friction. In other words, the increasing pile-soil relative displacement has increased the pile shaft friction from the initial value to the peak value even though the direction of the pile shaft friction has reversed. Therefore, more loads will be carried by the positive friction in the lower part of the pile and pile base pressure.
3) Volume deformation of expansive and collapsible soils can significantly influence the pile mechanical behaviors due to the changes in the net normal stress in the horizontal direction and pile-soil relative displacement in the vertical direction. In the two tests presented in this study, the influences of suction reduction–induced soil volume changes have a significant influence compared to suction reduction. In other words, the volume change behavior is a key factor in the pile load transfer mechanisms.
The raw data supporting the conclusion of this article will be made available by the authors, without undue reservation.
YL performed the pile infiltration tests, collected and analyzed the data, and developed the manuscript draft. SV provided conceptual advice, insights, and recommendations for the experimental program. He also edited the manuscript and contributed in the analyses. SJ assisted in the test program and in editing the tables and figures involved in the text.
The authors declare that the research was conducted in the absence of any commercial or financial relationships that could be construed as a potential conflict of interest.
All claims expressed in this article are solely those of the authors and do not necessarily represent those of their affiliated organizations, or those of the publisher, the editors, and the reviewers. Any product that may be evaluated in this article, or claim that may be made by its manufacturer, is not guaranteed or endorsed by the publisher.
The authors gratefully acknowledge the research funding from the Natural Sciences and Engineering Research Council of Canada (NSERC) and National Natural Science Foundation of China (Grant Number: 42107196).
Al-Omari, R. R., Fattah, M. Y., and Fadhil, S. H. (2017). “Adhesion Factor of Piles Embedded in Unsaturated Swelling Soil,” in 19th International Conference on Soil Mechanics and Geotechnical Engineering, September 2017 (Seoul Korea: Stress transfer), 2703–2706.
Fan, Z. H. (2007). Research on Swelling-Shrinkage Characteristic and Pile-Soil Interaction of Expansive Soil Foundation. Changsha, China: Central South University. Ph.D thesis.
Fattah, M. Y., Al-Omari, R. R., and Fadhil, S. H. (2020). Load Sharing and Behavior of Single Pile Embedded in Unsaturated Swelling Soil. Eur. J. Environ. Civ. Eng. 24 (12), 1967–1992. doi:10.1080/19648189.2018.1495105
Fredlund, D. G., and Xing, A. (1994). Equations for the Soil-Water Characteristic Curve. Can. Geotech. J. 31 (4), 521–532. doi:10.1139/t94-061
Han, Z., Vanapalli, S. K., and Kutlu, Z. N. (2016). Modeling Behavior of Friction Pile in Compacted Glacial till. Int. J. Geomechanics 16 (6), D4016009. doi:10.1061/(asce)gm.1943-5622.0000659
Huang, X. F., Chen, Z. H., Ha, S., Xue, S. G., and Sun, S. X. (2007). Research on Bearing Behaviors and Negative Friction Force for Filling Piles in the Site of Collapsible Loess with Big Thickness. Chin. J. Geotechnical Eng. 29 (3), 338–346. doi:10.3321/j.issn:1000-4548.2007.10.031
Kakoli, S. T. N., Hanna, A. M., and Ayadat, T. (2011). “Simulation of Collapsible Soils Subjected to Inundation,” in Proceedings of the 17th International Conference on Soil Mechanics and Geotechnical Engineering, 5-9 October 2009, (Alexandria, Egypt: IOS Press), 3431–3434.
Kakoli, S. T. N. (2011). Negative Skin Friction Induced on Piles in Collapsible Soils Due to Inundation. Montreal, Canada: Concordia University. Ph.D thesis.
Lim, Y. Y., and Miller, G. A. (2004). Wetting-Induced Compression of Compacted Oklahoma Soils. J. Geotech. Geoenviron. Eng. 130 (10), 1014–1023. doi:10.1061/(asce)1090-0241(2004)130:10(1014)
Liu, Y., and Vanapalli, S. K. (2021). Mechanical Behavior of a Floating Model Pile in Unsaturated Expansive Soil Associated with Water Infiltration: Laboratory Investigations and Numerical Simulations. Soils Found. 61 (4), 929–943. doi:10.1016/j.sandf.2021.06.004
Mendoza, C. (2013). “Measurement of Friction on Piles over Neutral Plane in Expansive Soils,” in Advances in unsaturated soils-Caicedo et al (London: Taylor & Francis Group).
Noor, S. T., Hanna, A., and Mashhour, I. (2013). Numerical Modeling of Piles in Collapsible Soil Subjected to Inundation. Int. J. Geomech. 13 (5), 514–526. doi:10.1061/(asce)gm.1943-5622.0000235
Overton, D. D., Chao, K. C., and Nelson, J. D. (2007). “Heave Distress of a Manufacturing Building,” in Proceeding of Geo-Denver 2007: Contemporary Issues in Deep Foundations, October 2007 (Reston, VA: ASCE). doi:10.1061/40902(221)23
People's Republic of China Ministry of Construction (2008). Technical Code for Building Pile Foundations (JGJ 94- 2008). Beijing, China: China Architecture & Building Press.
Pereira, J. H. F., and Fredlund, D. G. (2000). Volume Change Behavior of Collapsible Compacted Gneiss Soil. J. Geotech. Geoenviron. Eng. 126 (10), 907–916. doi:10.1061/(asce)1090-0241(2000)126:10(907)
Shi, B., Jiang, H., Liu, Z., and Fang, H. Y. (2002). Engineering Geological Characteristics of Expansive Soils in China. Eng. Geol. 67 (1-2), 63–71. doi:10.1016/s0013-7952(02)00145-x
Soundara, B., and Robinson, R. G. (2017). Hyperbolic Model to Evaluate Uplift Force on Pile in Expansive Soils. KSCE J. Civ. Eng. 21 (3), 746–751. doi:10.1007/s12205-016-1001-8
Tadepalli, R., and Fredlund, D. G. (1991). The Collapse Behavior of a Compacted Soil during Inundation. Can. Geotech. J. 28 (4), 477–488. doi:10.1139/t91-065
Wang, C., Wang, X., Zhou, S., and Wang, B. (2010). Centrifugal Model Tests on Self-Weight Collapsible Loess and Negative Skin Friction of Pile Foundations. Chin. J. Rock Mech. Eng. 29 (S1), 3101–3107.
Wu, Z. W., Song, H. Z., Wang, H. Y., Liu, C. J., and Jiang, Y. (2012). Prototype Experimental Study of Expansive Force of Pile Embedded in Expansive Soil. Rock Soil Mech. 33 (S2), 173–177.
Xiao, H.-b., Zhang, C.-s., Wang, Y.-h., and Fan, Z.-h. (2011). Pile-Soil Interaction in Expansive Soil Foundation: Analytical Solution and Numerical Simulation. Int. J. Geomech. 11 (3), 159–166. doi:10.1061/(asce)gm.1943-5622.0000046
Keywords: model piles, expansive soils, collapsible soils, soil suction, infiltration, pile–soil interaction
Citation: Liu Y, Vanapalli SK and Jiang S (2022) Mechanical Behavior of Piles in Typical Unsaturated Expansive and Collapsible Soils Upon Water Infiltration. Front. Built Environ. 8:864421. doi: 10.3389/fbuil.2022.864421
Received: 28 January 2022; Accepted: 25 April 2022;
Published: 14 June 2022.
Edited by:
Mounir Bouassida, Tunis El Manar University, TunisiaReviewed by:
Mohammed Y. Fattah, University of Technology, IraqCopyright © 2022 Liu, Vanapalli and Jiang. This is an open-access article distributed under the terms of the Creative Commons Attribution License (CC BY). The use, distribution or reproduction in other forums is permitted, provided the original author(s) and the copyright owner(s) are credited and that the original publication in this journal is cited, in accordance with accepted academic practice. No use, distribution or reproduction is permitted which does not comply with these terms.
*Correspondence: Sai K. Vanapalli, dmFuYXBhbGxAZW5nLnVvdHRhd2EuY2E=
Disclaimer: All claims expressed in this article are solely those of the authors and do not necessarily represent those of their affiliated organizations, or those of the publisher, the editors and the reviewers. Any product that may be evaluated in this article or claim that may be made by its manufacturer is not guaranteed or endorsed by the publisher.
Research integrity at Frontiers
Learn more about the work of our research integrity team to safeguard the quality of each article we publish.