- 1Department of Architecture, TUM School of Engineering and Design, Technical University of Munich, Munich, Germany
- 2Department of Architecture, IU International University, Munich, Germany
- 3Chair of Building Technology and Climate Responsive Design, Department of Architecture, TUM School of Engineering and Design, Technical University of Munich, Munich, Germany
In the context of dense urban environments and climate change, pedestrians’ thermal experience plays an increasingly significant role in people’s health and well-being. In this research, the authors combine the fields of architecture, climate-responsive design, and robotic fabrication with the goal of investigating strategies to improve outdoor thermal comfort for pedestrians in cities with frequent extreme heat events. Based on a case study in the city of Munich, this paper presents findings into the technological approaches and methods for location-specific climate-resilient brick facades using robotic assembly. To achieve this goal, different bricklaying patterns were investigated to create a self-shading effect and thus reduce solar radiation and ultimately achieve an improved thermal condition for pedestrians moving along urban facades at street level. Using computer-aided microclimate simulation, generic self-shading brick pattern designs were tailored to highly location-specific microclimate requirements. Robotic assembly technology was used to produce such tailored, non-standard brickwork facades. The results of this research led to a data-informed design process with a demonstrator object being realized at 1:1 scale with a height of 2 m and a length of 3 m using a collaborative robot on site. Thermal measurements on the built demonstrator provided indications of reduced surface temperatures despite high solar radiation and thus validated the location-specific self-shading effects according to solar radiation simulation.
1 Introduction
A supremely comfortable urban space can benefit cities in many ways, including encouraging cycling and walking, attracting more people to city comfort zones, increasing the well-being and health of city pedestrians, and being used for commercial and tourist attractions to make regions both climate resilient and economically beneficial (Chokhachian et al., 2020). As an example, the city of Copenhagen uses and documents clear standards for enhancing the quality of life and human well-being in its architecture policy (Architecture Policy for Copenhagen 2017-2025, 2017), thereby promoting the idea of the “city on a human scale” that emphasizes the importance to focus on the eye level at which people sensually experience urban environments. One of the measures to define urban well-being and health is the amount of thermal stress for pedestrians, in which comfortable outdoor thermal conditions are directly related to people’s positive perception of outdoor areas (Antonini et al., 2020; Peng et al., 2021); in temperate climates, the main focus is thus on reducing heat stress during hot summer periods (Rahman et al., 2022). In this context, the increasing occurrence of extreme weather conditions in temperate climates caused by climate change is the reason that exposure to heat stress has become a rising problem for pedestrians in cities (Guide to Climate Change Adaptation in Cities, 2011). In addition, according to the latest forecasts by the World Meteorological Organization, the frequency of such extreme weather events will continue to become more frequent (World Meteorological Organization, 2021).
The challenge of providing comfort to pedestrians in cities can be solved primarily through architecture and urban development. One of the main architectural strategies in temperate climates to decrease heat stress inside cities is to increase the vegetation cover, for example along sidewalks. This has been proven to improve human comfort levels and encourages people to actively use public space (Santamouris, 2014; Negev et al., 2020). In dense urban areas where greenery cannot be increased, other active and passive cooling options can be considered as mitigation strategies; one passive cooling option is to shade building structures or to create surfaces with minimized exposure to solar radiation (Young et al., 2021)—creating cool surfaces to act as cold spots to give citizens comfort outdoor opportunities to recover from increasing heat stress (Klemm et al., 2015). While such options build on the preliminary understanding of local microclimate conditions around buildings (Lenzholzer et al., 2018; Roesler and Kobi, 2018), the potentials and methods for designing spatiotemporal variations of microenvironments for outdoor and public spaces are not yet sufficiently understood (Lau et al., 2022). Moreover, there is a lack of technologies that allow to design, evaluate, and eventually realize highly customized solutions, individually tailored to specific geolocations and microclimatic requirements.
This research thus explores the topic of improving the microclimate in densely populated urban areas in temperate climate zones and tests the proposed strategy with a case study in the city of Munich. By particularly addressing the human thermal exchange with its surrounding surfaces, it investigates location-specific strategies to reduce surface temperatures of building facades, and thereby reduce the radiated heat on people. As such, it explores whether customized self-shading effects on the external facades of buildings tailored to the local situation can reduce exposure to solar radiation and thus decrease solar absorption and heat storage of the surfaces (see Figure 1). More specifically, the investigations focus on an interdisciplinary computational design and robotic brick assembly concept, which has been applied and validated in a real-scale demonstrator object—a south-west-oriented exposed brickwork facade in Munich’s Kreativquartier realized as part of a summer school with students from the Technical University of Munich in August 2020 (see Figure 2).
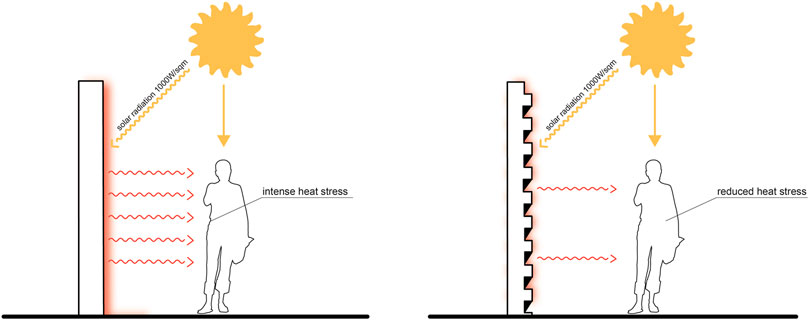
FIGURE 1. Flat surfaces of urban walls that are directly exposed to the sun tend to radiate heat and thus create heat stress for city dwellers. Decreasing the exposure of such facades to solar radiation, e.g., through self-shading effects, can help reduce the heat and thus improve the ambient climate for residents.
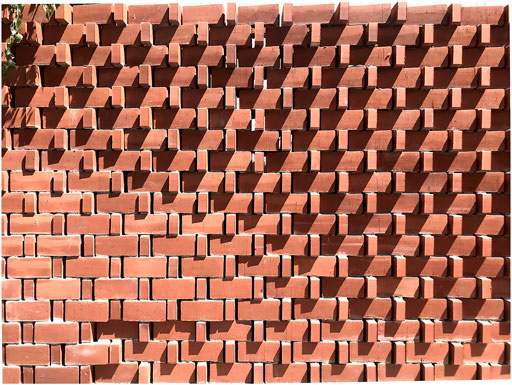
FIGURE 2. Close-up of the self-shading effect of the robotically fabricated facade, shot on 7 August 2020, 13:02.
The paper is organized as follows: Section 2 explores the architectural context and motivation for this research. Section 3 provides an overview of the more recent research in this field and goes into detail on the individual research subjects. The concept and methodology are outlined in Section 4. In Section 5, the results are presented in a case study to illustrate the practical relevance and implementation of the proposed methods. Finally, the results and conclusion of this research are discussed in Section 6.
2 Architectural Context
Qualities of cities in the outdoor pedestrian zones have played an important role since ancient times. Vitruvius already addressed the role of public areas in De Architectura, considered as the “heart of any city plan” to invite residents to linger and recover (Moughtin and Mertens, 2003; Leggero, 2018). For example, the city of Pompeii as well as Florentine architecture still show historical precedents of elaborately integrated benches incorporated into building facades. Their purpose is not to serve as architectural decoration, but engaging the citizens to communicate and actively interact with the outdoor space (Elet, 2002), vividly demonstrated with the design of the Palazzo Rucellai in Florence, one of the first Renaissance facades in Florence that, next to a tailored and highly differentiated façade design, included an integrated bench (c.1455, Leon Battista Alberti, see Figure 3).
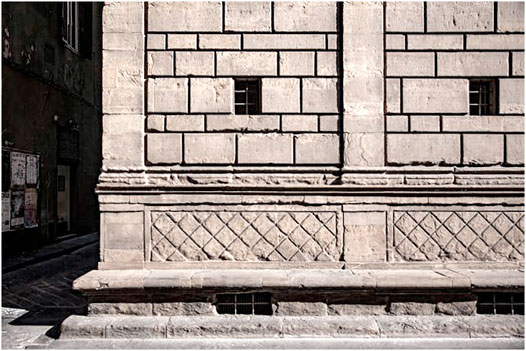
FIGURE 3. The exterior of a building envelope can fulfill various functions, as shown by the integrated bench providing a resting place for passers-by at the Palazzo Rucellai designed by Leon Battista Alberti (15th century) (Alberti, n.d.).
The range of uses of integrated functions in facade design to influence the outdoor space varies depending on the time and place, alternating between representation of power and social control, or simply as a contribution to urban life (Hartnett, 2008). During the Industrial Revolution in the beginning of the 19th century, the notion of urban space changed and lost its initial importance. Technological inventions such as the automobile intensified the trend of outdoor space towards pedestrian-free areas with low amount of comfortable walkable zones. The increase of fast rebuilding after the first and second World War led to further urban densification with disappearing urban recovery spaces for residents (Ascher, 1960) and is still evident until today (Laakkonen, 2020).
This trend from vivid to lifeless urban areas causes dullness, and demands urban stimulation. In the urban context, this stimulation particularly happens due to the unguided and freely circulating, interacting people, whose gathering areas are primarily directed by architecture and urban planning (Gehl, 2011; Church et al., 2012). Based on studies on the demand-driven planning and use of urban spaces with the involvement of citizens, globally active organizations such as the “Gehl Institute” or the “WHO” highlight the need to provide comfort strategies such as shading opportunities on hot summer days for the active use of urban space and recommend the early inclusion of local climate conditions in urban planning to enliven urban life (Gehl and Svarre, 2013; World Health Organization, 2017; Gardner et al., 2018).
3 State of the Art
3.1 The Role of Facade Design on Urban Microclimate
Building envelopes designed to reduce energy consumption for heating as well as cooling are well researched in building design (Tarabieh et al., 2017; Tarabieh et al., 2018; Abdelmohsen et al., 2019). Since the 1980s, energy regulations have pushed research and innovation of building envelopes towards better U-values especially in northern and central Europe, (Wernery et al., 2017), primarily to improve indoor comfort conditions and reduce heating demands. In the last decade, awareness of the impact of building envelopes on outdoor comfort, i.e., the urban microclimate, has grown and its impacts have been studied particularly in the context of climate change and Urban Heat Islands (UHI) (O’Malley et al., 2014; Ningrum, 2018).
In dense urban environments, the absorbed solar radiation is stored and reradiated by urban structures, resulting in decreased comfort conditions and increased energy consumption in summer (Rizwan et al., 2008). Studies show that brick facades with low reflectivity in comparison with heavily insulated envelopes can decrease extreme heat stress for pedestrians by 26% during a hot summer day (Chokhachian et al., 2017). Further research addresses the cooling effects of street level zones by evaporation of brick building envelopes. Molter and Chokhachian proof significant cooling effects for pedestrians in hot urban environments by evaporation cooling of watered brick walls (Molter and Chokhachian, 2021). Han et al. (Han et al., 2017) investigate the effect of two passive cooling systems, water-retaining bricks on roof and radiation shield on roof, concluding that the maximum cooling capability can be achieved through water-retaining bricks on the roof. Another study explores the effects of a moist void-brick wall as a passive microclimatic converter. The results indicate a daily average of 5°C lower surface temperature of the wall compared to the temperature of the ambient air (He and Liu, 2012). Based on these previous research, other authors consider the potentials of geometric configuration with the evaporative cooling potential of brick elements (Molter and Chokhachian, 2021). The results show a relationship between self-shading geometric configuration of the walls and the surface temperatures of the building envelopes. Furthermore, the geometry of the pattern design has a strong impact on thermal performance of the brick facades since the façade surface temperature significantly decreased. This indirect effect on outdoor spaces has a positive effect on the Mean Radiant Temperature (MRT) on a local scale. The MRT describes a measure of an average temperature surrounding a point or mostly a human body with which it will exchange thermal radiation. In terms of solar exposure, the results show the lowest values of solar absorption for the extruded configuration (Molter and Chokhachian, 2021). These assumptions mostly refer to studies on repetitive and regular brick bond patterns, such as the Flemish bond. Yet, there is little research on thermal effects of brick configurations on the process of integrating microclimate simulation into a computational design environment for a site-specific on-site robotic assembly. The proposed research investigates in a demonstrator object that serves to validate the thermal simulations with substantial data of the thermal behavior.
3.2 Robotic Brick Assembly for Bespoke Designs
The use of robots in the Architecture, Engineering and Construction (AEC) sector has demonstrated the potentials in the production of non-standard designs, offering the opportunity to go beyond the mere technical aspects of construction (Gramazio et al., 2013; Dörfler et al., 2019; Han et al., 2021). Within this context, approaches such as bricklaying robots, concrete printing robots, or timber manufacturing robots, aim to both expand the possibilities of traditional construction methods with the aim to increase productivity and customization, reduce material, costs, and construction time, and minimize risks (Blanco et al., 2020), but further, to form the basis for integrative design and construction approaches in the creation of novel architecture (Craney and Adel, n.d.). By using standardized building components and aligning them spatially within a certain assembly logic, bricklaying robots are one of the most promising developments for both off-site and on-site digital fabrication (Chea et al., 2020; Ambrosino et al., 2021). While custom developed in-situ bricklaying robots such as Hadrian X (Fastbrick Robotics, 2020) and Sam (Bock, 2007) explore the automation of standard brickwork directly on building sites (Shen and He, 2021), robotic bricklaying strategies using industrial robots such as developed by Gramazio Kohler Research have enabled entirely new digital design-to-fabrication workflows for the production of bespoke and non-standard undulated brick structures both in prefabrication (Bonwetsch et al., 2006; Gramazio and Kohler, 2008), as well as for the production of bespoke, non-standard brickwork with mobile robots on site (Dörfler et al., 2016). However, besides its great potential, in situ bricklaying using bricks and mortar using robotic technology directly on construction sites is not yet adopted at scale today. This is partially because traditional construction methods have evolved around human skills and competencies, still posing significant challenges for the automation process (Elhouar et al., 2019; Mitterberger et al., 2020). For the other part, construction sites themselves, which are characterized as poorly structured and dynamic, and inherently subject to change, pose substantial challenges to robot deployment. One possible way to meet this challenge is to use different actors, both humans and robots, to perform heterogeneous tasks in coordinated manner (Angleraud et al., 2021). Therefore, we propose a novel fabrication approach utilizing a collaborative workflow between humans and robots to perform the cooperative task of bespoke bricklaying with mortar on-site. As such, the potential of robotic fabrication technology can be substantially utilized for realizing site-specific and bespoke climate-resilient building structures as proposed by this research directly on site (Chea et al., 2020).
4 Material and Method
This paper discusses an on-site solution for a brick wall size of 3 m by 2 m, concentrating on the human relevant space up to 2-m height, since solar radiation in the space above contributes less to the human thermal perception. Regardless to the human needs, microclimate affects the entire façade and potentially provides further beneficial knowledge to unlock climate-resilient properties on different levels.
The interdisciplinary research presented in this paper applies an experimental and qualitative case study methodology. In this case study, the authors 1) investigated architectural design principles for self-shading brick facades, 2) developed a computational design tool to explore site-specific solutions and optimize the self-shading effect for georeferenced locations using microclimate simulation, 3) developed and explored a collaborative robotic fabrication workflow for bespoke in situ brick and mortar construction, and 4) validated the proposed methods by producing a 1:1 scale demonstrator object in the Kreativquartier in the city of Munich. This demonstrator object allowed the authors to verify whether integrating architecture, computational climate-resilient design and robotic fabrication can unlock the climate-resilient properties of bricks in building facades.
4.1 Material System
Since the designs strategy for this research focused on a solid brick wall for pedestrian street level zones, the authors used a solid brick provided by the brick manufacturer “LeipfingerBader Ziegelwerke” with a standard size of 11.5 × 24 × 5.2 cm, a raw density of 2.0 kg/dm³, and a thermal conductivity of λ = 0.96 W/mK. The chosen red brick color represents a brick type in common use for architectural brick facades. A conventional cement masonry mortar (Maxit mur 920) supported the horizontal mortar joint of the individually placed bricks.
4.2 Design Principles
The entire façade design is based on a regular rat-trap bonded brickwork, a rectangular compound that creates an internal cavity, bridged by two rowlocks, a shiner, and a back brick. The cavity of this the brick compound supports the reduction of heat transmission for solar exposed exterior walls (Marunmale and Attar, 2014). Architect Sir Laurie Baker first introduced this type of building component 1970 in India as an alternative to the conventional Flemish brick masonry to considerably decrease construction cost, time, and labor. In total, this system saves 30–50% of mortar and 20–35% of used bricks while keeping the same wall thickness, compared to conventional Flemish brick masonry (Ullah et al., 2018).
To create the self-shading effect on the proposed rat-trap bond, each compound is stacked with a half shiner length offset to interlock, in which the shiner is capable of changing position by two interacting transformation parameters, rotation and translation. In order not to compromise the general structural behavior of the bond, the two rowlocks and the back brick always remain fixed (see Figure 4).
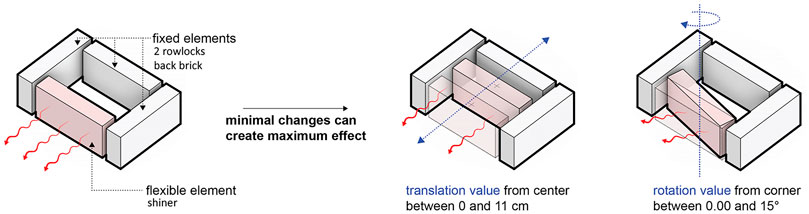
FIGURE 4. While the side headers, the rowlocks and the back brick of the rat-trap bonded brickwork remain fixed, two parameters of the front brick, shiner—rotation and translation—can be modulated to create a self-shading effect and thus reduce the exposure of the entire surface to solar radiation.
4.3 Microclimatic Simulation
Climatic conditions are in general extremely localized and they required simulation methods to completely represent the thermal sensation of a person (Lau et al., 2019). Hence, the integration of environmental modeling workflows in the computational design model supports the decision-making process at a very early design stage. In this study, a custom workflow is generated based on solar exposure of the brick elements and their effect on emitted radiation from the surface. The design studies performed are the result of surface temperature simulations with Ladybug Tools, a software to visualize and analyze weather data in the software Rhino/Grasshopper, provided with detailed climate data for the specific location in Munich using an EnergyPlus Weather File (EPW). The simulations are restricted to the highest solar radiation potential, selected between first of June and 31st of August, daily within the time-period of 11:00 a.m. to 4:00 p.m. with a southwestern exposure (Figure 5).
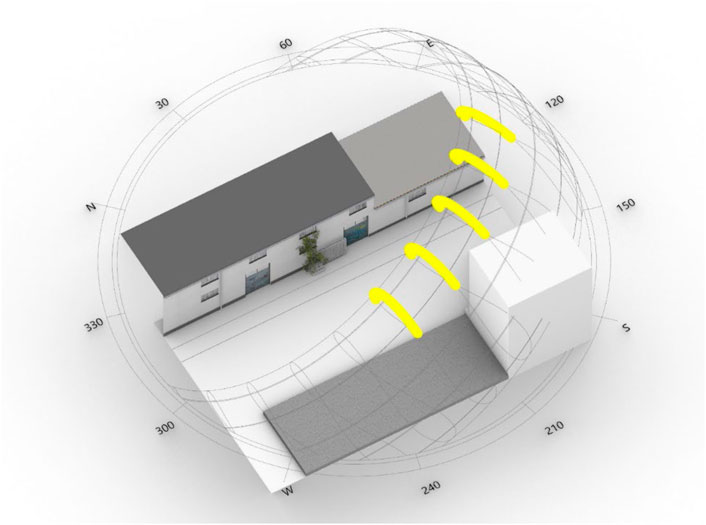
FIGURE 5. Accumulated sun path simulation in Ladybug for the south-west oriented façade between first of June and 31st of August within the time-period of 11.00 a.m. to 4.00 p.m.
A south-west oriented regular flat rat-trap bonded brick façade serves as geometrical input. This original flat front surface of the bond is, as any other flat surface, directly exposed to the Sun. On hot days, this direct exposure radiates extreme heat that causes heat stress on passing pedestrians. By simulating solar radiation on to the façade, the surface temperature within its ambient conditions is mapped by a color code for each brick. The color value of each individual brick defines the transformation of the shiner within the predefined parameters of translation and rotation, consequently reducing the surface area exposed to the sunlight rays. An integrated bench in the wall underneath a tree serves as an architectural element for lingering contributing to the outdoor thermal comfort to ensure comfortable sitting; the backrest behind the bench thus remains unaffected by any transformation parameters. Nevertheless, this area leads to a high level of absorption of the incident solar radiation (see Figure 6). The solar exposure has also been visualized in a shadow casting simulation (see Figure 7).
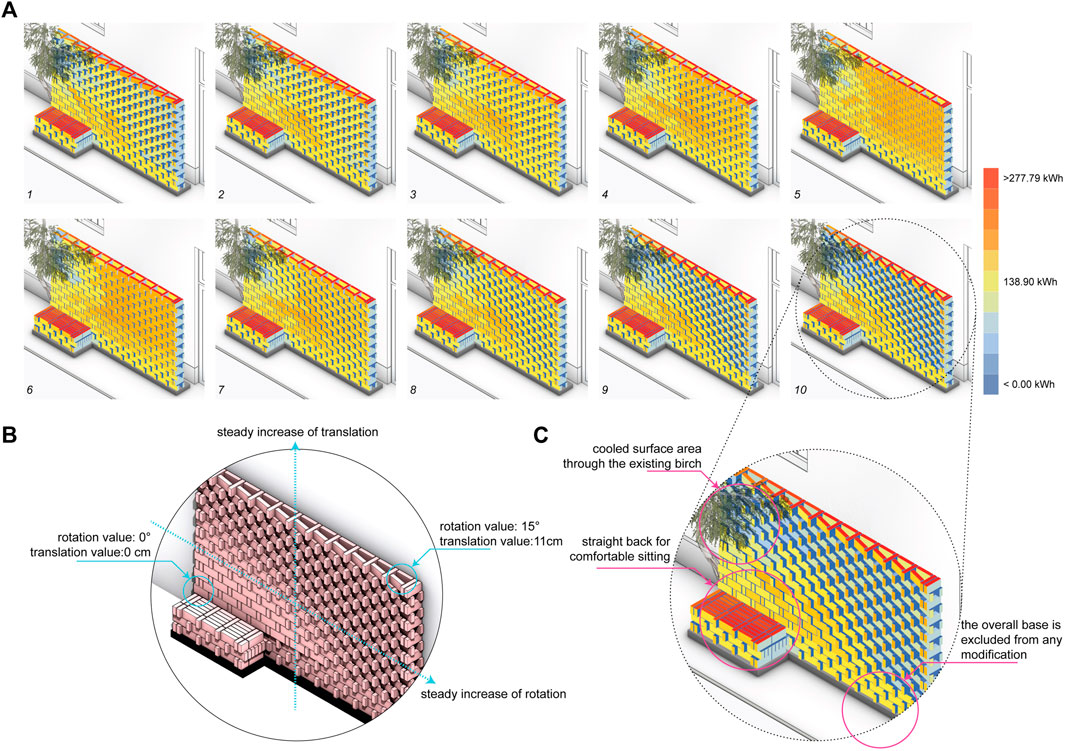
FIGURE 6. (A) Starting from the solar radiation simulation in Ladybug Tools on a regular rat-trap bonded flat brick facade (A5), different configurations based on an altering rotation and translation value of the shiner to the different surface temperatures and analyzed for the average time period extended from 01 June 2020, until 31 August 2020, 11:00–16:00 and visualized in this diagram. The maximum rotation angle ranges from -15° (1) to +15° (10). The results show that the rotation of the shiner from −15° to 0° (1–5), i.e., the rotation towards the sunlight rays cools the surface less than from 0° to 15° (6–10). (B) Design drivers: The rotation and translation values increase from left to right and bottom to top to generate a gradient pattern within the wall. The visualized wall for August 12:30 highlights this within the self-shading effect. (C) Selected design option: The final appearance is limited to certain architectural principles such as the flat back of the bench or the pattern of the base. Natural shading elements such as the birch tree support the shading effect and are therefore less dependent on any pattern modification.
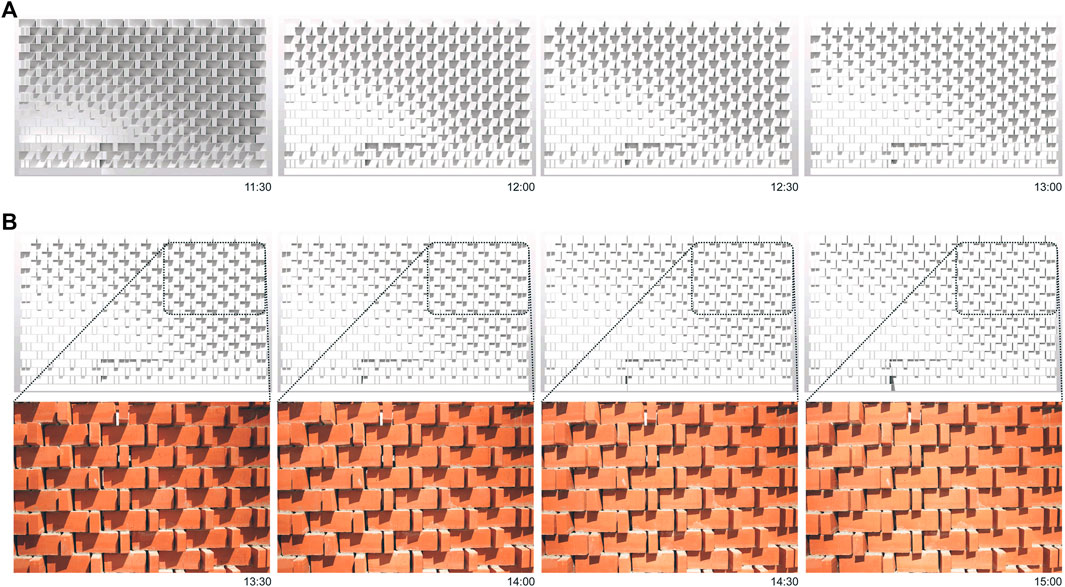
FIGURE 7. Shadow casting simulation of the self-shading effect during a sunny day on August 7th: (A) The orientation of the rotated bricks provides self-shading effects especially in the morning hours and prevent the wall to heat up during day. (B) The afternoon images, taken on 7 August 2020 and the matching visualization for the same times and date proof the assumptions gained from the solar radiation simulation and the casting simulation. The self-shading effect decreases in the afternoon due to the sun’s movement.
Various design options for a site-specific solution with reduced surface temperature are generated, which negotiate between stability criteria of the rat-trap bond and optimal self-shading effects. By shifting the shiner toward the back, the amount of exposed surface reduces through shading by adjacent protruding bricks. To reduce solar reflection even further, the front brick rotates against the incidence angle of the Sun to deflect the sunlight rays. The wider the angle with respect to the Sun’s location, the lower the value of the absorbed radiation, which results in a further decrease in surface temperature. As design principle, the shiner brick moves a maximum of 11 cm towards the back. In terms of stability, the brick must not exceed half of the total brick length. The rotation angle varies between 0° and 15° and opens facing towards south-west (see also Figure 4). The two parameters allow highly site-specific solutions to lower surface temperatures at the time when it usually reaches a maximum. The outcome is a highly customized rat-trap bonded and self-shading brick wall, in which each shiner is defined with an individual position by the digital model.
4.4 Human-Robot Collaborative Bricklaying Workflow
Previous research has demonstrated robotic systems to be very effective in achieving various differentiated brickwork designs with a high degree of accuracy but also facing limitations such as the on-site mortar use. For this research, a collaborative robot universal Robot (UR10e) operated alongside a group of researchers and students to accomplish the proposed construction tasks in turn-taking actions with humans. This robot was equipped with a vacuum area gripper, that picks up and releases bricks, thus capable of arranging and laying bricks according to its location in the digital model. Mixing, packing, and cleaning the mortar joint is a process that requires a great degree of dexterity and skill. In addition, mortar regarding its setting time reacts to prevailing external conditions—such as humidity, wind, or air temperature in a non-linear way. For this reason, the mortar process was purposely not carried out by a robot, but by a collaborating human. This required a sequential workflow with alternating physical actions between the robot and a human, comprising the computational precision of the robot for the laying of the brick and the realm of manual craft for the mortar jointing (see Figure 8).
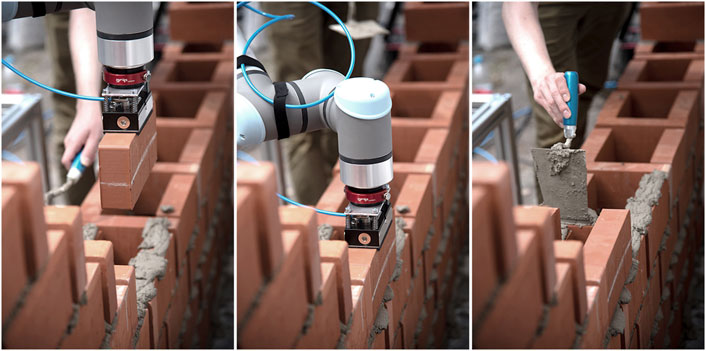
FIGURE 8. Craftitude meets robotic fabrication: While a person mixes and applies the mortar, the collaborative robot lays individual bricks according to the digital model. The consistency of the fresh mortar allows the robot to easily press the brick into place, after which the person can remove any excess mortar and grout the joint.
The digital model of the wall was divided into eleven individual sequences composed of step-like courses of bricks within the portable robot’s static reach of 1.30 m. Each of the pre-defined sequences was then built from a specific robot location. Due to the limited radius of the robot arm, the integrated bench in front of the wall was fabricated as the last sequence. The use of half bricks in alternating courses ensured a straight edge finish of the bench. The computational model utilized survey points to guide the robot’s location, which were prerecorded on-site for estimating each new location by the robot arm relative to its work location. By matching robotically probed points with prerecorded survey points, the robot’s exact current location could be estimated in reference to these points, enabling a seamless alignment of the bricks of each current sequence with the previous one (see Figure 9).
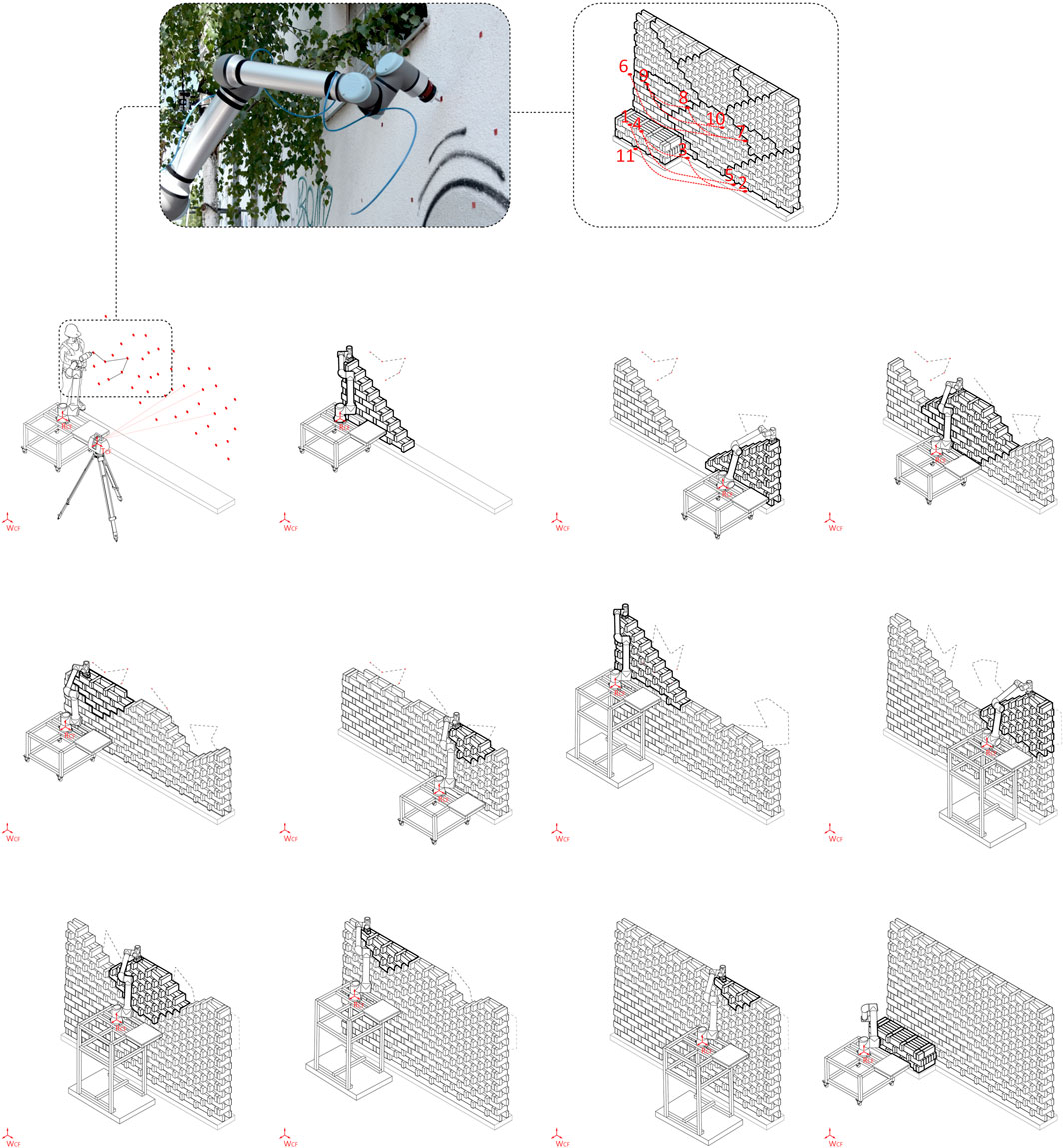
FIGURE 9. The collaborative robot was localized by recording a range of points with the robot arm that had previously been digitized by a robotic total station. This localization procedure was carried out at each of the ten subsequent robot locations during construction.
5 Results
5.1 Wall Fabrication
A total of 702 bricks were used in the fabrication process. This included 687 whole bricks and 15 half bricks, used for the straight edge finish of the bench. The sequential building process described here required a manual repositioning and referencing of the robot 11 times. While the localization accuracy and the consistency between the final built structure and the initial design was not within scope of the experiment, the accuracy was evaluated by measuring the position of placed bricks relative to previously placed neighboring bricks. These relative measurements were observed to be consistently within 5–8 mm of the value expected from the CAD model. The localization and brick placement errors did not accumulate over the course of the building process, since the robot’s location was estimated each time, 11 times in total, against the initial global reference points recorded by the total station (see Figures 9, 10).
5.2 Validation of the Climatic Simulation
The solar exposure on a warm sunny day reveals the relationship of solar absorption and increased surface temperatures. The results of the on-site measurements show a significant relationship between surface shadowing and surface temperatures of a brick wall (see Figure 11). The results of the measurements of surface temperatures received with the thermal camera on site are not fully comparable with the data generated by the solar radiation simulation in the digital model, that is, since creating such exact boundary conditions for an outdoor space to simulate the actual surface temperature of each brick is very computationally expensive if including all thermal properties and thermal energy transfers between each brick geometry. Furthermore, the simulation mainly highlights the effect of self-shading effects within a building envelope, leading to a significantly reduced lower surface temperature to contribute to an improvement of outdoor comfort. Since the main driver of the surface temperatures on a sunny summer day is the solar gains, this study tries to build a correlation logic between the amount of radiation falling on the wall and the increased surface temperatures demonstrated by the thermal images.
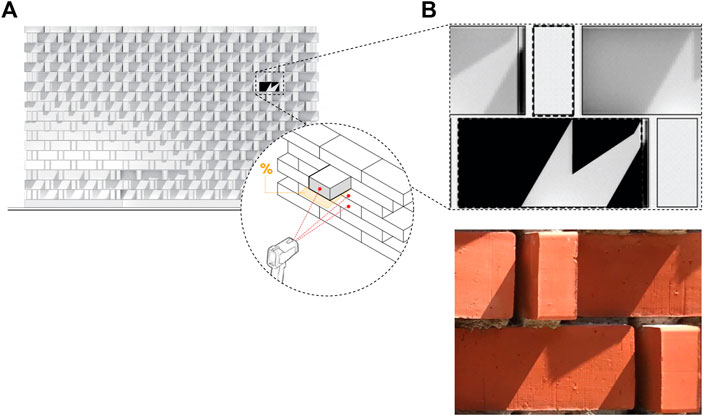
FIGURE 11. comparison of cast shadow: The simulation shows the self-shading effect on protruding geometries in the wall (A) with the identic image (B) on August 7th, 12:30 h. The black colored shiner in (A) shows 53% of shaded surface area for the time with the highest incident of solar radiation which leads to a reduction of solar absorption.
On 7 August 2020, a warm summer day in Munich (temperate climate) the exposed real-scale demonstrator object served for field measurements collected with a thermal camera. The day provided cloudless solar radiation onto the brick wall and the air temperature reached 32° at peak time (2:00 p.m.). At 8.20 a.m., the air temperature showed 21.1°C. The entire wall showed similar surface temperatures since the wall was not exposed to solar radiation during night. During the morning hours, the demonstrator object got exposed to solar radiation. At 12.00 a.m. almost 85% of the shiner brick surfaces were shaded in the right part of the demonstrator object, which resulted in lower solar absorption and therefore lower surface temperatures. At 13.35 h and an air temperature of 31.5°C, the average surface temperature of a brick showed a maximum decrease in surface temperature of 4.7°C between self-shading geometry at the right part and flat geometry on the left part of the wall (see Figure 12). The measurements confirm that slight modifications in geometrical configuration of brick patterns can already decrease surface temperatures of solar exposed brick facades and contribute towards a positive impact on thermal comfort.
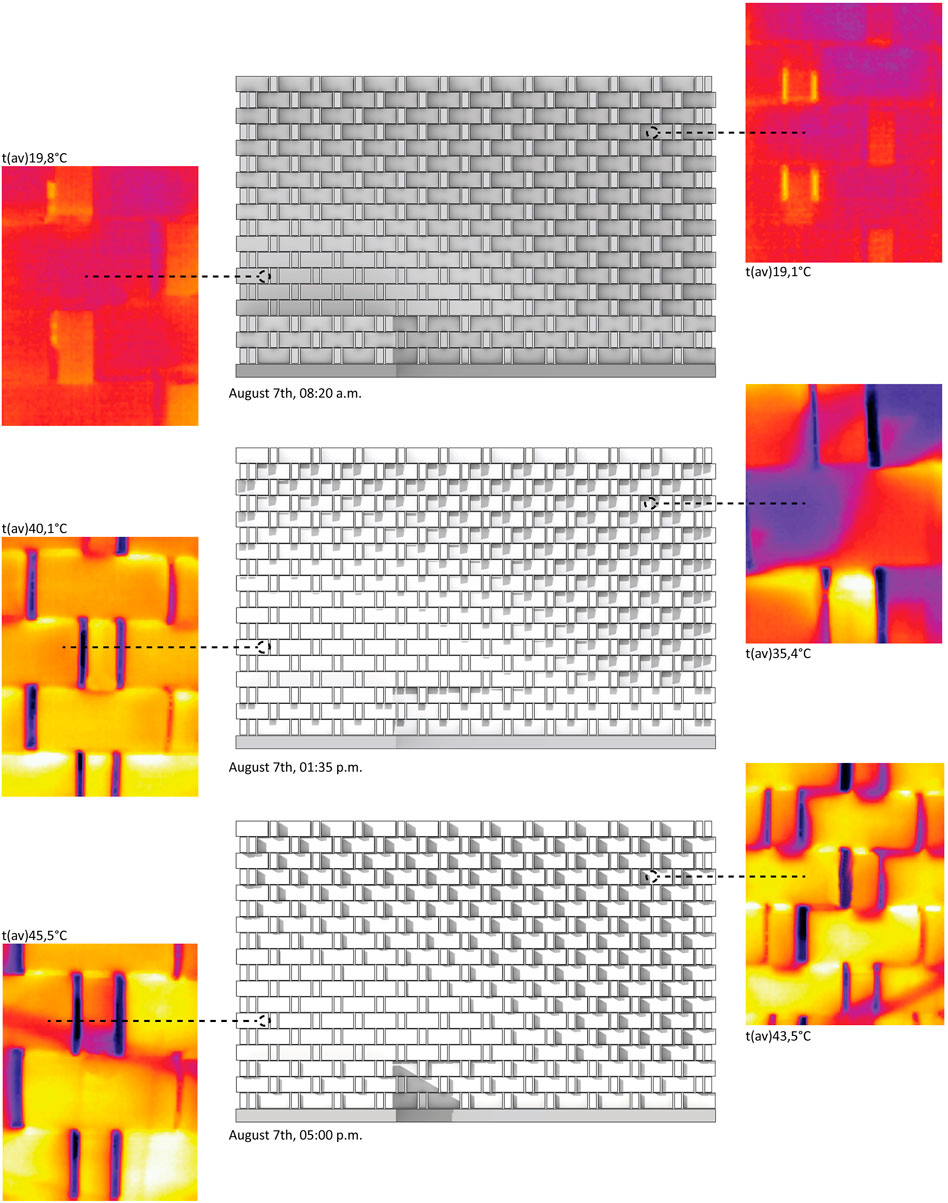
FIGURE 12. Images of the thermal camera at three different times during a hot sunny day (seventh of August) in Munich.
6 Conclusion and Discussion
This paper showed the workflow to use algorithmically optimized designs of a brick façade, tailored to a site-specific solar radiation profile of a wall, and consequent robotic assembly of the individualized, hyperlocal design in a human-robot collaboration process. The authors prove the relationship of digital design methods integrating simulation methods to optimize geometries and thermal performances and validated the approach experimentally with a real-scale demonstrator object. As such, this research contributes to existing methodologies by integrating fabrication criteria with solar radiation analysis within a single digital form-finding process and a fully digitally supported design-to-fabrication workflow, solving for multiple objectives of fabricatability and thermal performance.
Simulation environments such as solar radiation models reveal the potential to render microclimatic data at various levels of resolution. The combination of digital architectural models associated to microclimate simulations as an algorithmic input rule for design adaptations can thus adjust to the prevailing ambient conditions on a very local scale. A resulting continuous feedback loop enables the digital design to be enhanced with adaptable models and the superimposition of microclimate inputs. This approach allows assumptions to be made on perceived human comfort through decreasing solar radiation during warm summer days on a hyperlocal scale.
The research further confirms the potential for reducing solar radiation through self-shading bricks by real-world thermal measurements, thus proving the potentials of linked digital processes from design to fabrication. Nonetheless, to contribute to sustainable urban living and site-specific urban transformations, such research approaches must be made available for designers and planners to foster a sensitivity on the impact of façade designs and provide tools for their future implementation. Easy accessibility of these tools will lead to a broader integration of linked digital design intelligence at very early design stages, eventually enabling novel performance-oriented and functionally integrated architectural designs.
Due to the size of the demonstrator object, the introduced human-robot collaboration process facilitates the iterative planning as well as simulation design process and the individual placing of bricks compared to the conventional brick laying can’t be accomplished with such high precision. This proposed workflow of microclimatically driven, customized design towards fabrication is a process leading to a potentially integration into prefabrication.
Data Availability Statement
Publicly available datasets were analyzed in this study. This data can be found here: https://github.com/augmentedfabricationlab/climate_active_bricks https://www.ladybug.tools/epwmap/.
Author Contributions
JF, PM, AC, and KD jointly planned the research design and developed the method. AC provided the method with the microclimatic simulation, JF prepared with KD the design-to-fabrication workflow implementing the microclimatic simulation for the design feedback as well as its real-scale fabrication as proof of concept. PM carried out the thermal measurements on the final demonstrator object to validate the microclimatic simulations of AC. JF compiled the manuscript with feedback from KD, PM, and AC. All authors edited and approved the final manuscript.
Conflict of Interest
The authors declare that the research was conducted in the absence of any commercial or financial relationships that could be construed as a potential conflict of interest.
Publisher’s Note
All claims expressed in this article are solely those of the authors and do not necessarily represent those of their affiliated organizations, or those of the publisher, the editors and the reviewers. Any product that may be evaluated in this article, or claim that may be made by its manufacturer, is not guaranteed or endorsed by the publisher.
Acknowledgments
The realization of this project and of the research was only feasibly through the support of the Technical University of Munich, Department of Architecture and of the industry partners involved. The acknowledgement goes to Auer (Chair of Building Technology and Climate Responsive Design, TUM Department of Architecture), Wolfgang Wiedemann (The Chair of Geodesy, TUM Department of Aerospace and Geodesy), Valentin Heizinger (Leipfinger Bader Ziegelwerke GmBH) as well as Corbinian Böhm and Michael Gruber (Empfangshalle). The authors acknowledge the students who participated during the summer school in August 2021: Daria Alekseeva, Maurice Demeyer, Arvand Vaghari Fard, Robin Feys, Georgy Frolov, Arno Gabriel Goedefroo, Iuliia Larikova, Shiran Potié, Mr. Sébastien Wilwers.
References
Abdelmohsen, S. M., Tarabieh, K. A., Salem, I. I., El-Ghazi, Y. S., El-Dabaa, R. B., and Hassan, A. G. (2019). Coupling Parametric Design and Robotic Assembly Simulation to Generate Thermally Responsive Brick Walls. Build. Simul. Conf. Proc. 5 (September), 3006–3013. doi:10.26868/25222708.2019.210904
Alberti, L. B. (n.d.) “Palazzo Rucellai (Florence),” in wikimapia.org. Availble at: http://wikimapia.org/1899376/Palazzo-Rucellai#/photo/7214194.
Ambrosino, M., Delens, P., and Garone, E. (2021). “Modeling and Control of Multi-Units Robotic System: Boom Crane and Robotic Arm,” In Proceedings of the 38th International Symposium on Automation and Robotics in Construction (ISARC), Dubai, UAE. doi:10.22260/ISARC2021/0107
Angleraud, A., Sefat, A. M., Netzev, M., and Pieters, R. (2021). Coordinating Shared Tasks in Human-Robot Collaboration by Commands. Front. Robot. AI 8 (October), 734548. doi:10.3389/frobt.2021.734548
Antonini, E., Vodola, V., Gaspari, J., and De Giglio, M. (2020). Outdoor Wellbeing and Quality of Life: A Scientific Literature Review on Thermal Comfort. Energies 13 (8), 2079. doi:10.3390/en13082079
Architecture Policy for Copenhagen 2017-2025 (2017). in 76. Copenhagen: The City of Copenhagen, Technology and Environment Department. Available at: https://kk.sites.itera.dk/apps/kk_pub2/pdf/1904_4b203fafa9a8.pdf.
Ascher, C. S. (1960). PAUL ZUCKER. Town and Square: From the Agora to the Village Green. Pp. Xxiii, 287. New York: Columbia University Press, 1959. $15.00. Ann. Am. Acad. Political Soc. Sci. 329 (1), 188. doi:10.1177/000271626032900157
Blanco, J. L., Mischke, J., Ribeirinho, M. J., Rockhill, D., Sjödin, E., Strube, G., et al. (2020). The Next Normal in Construction. Mckinsey & Company. Available at: https://www.mckinsey.com/∼/media/McKinsey/Industries/Capital Projects and Infrastructure/Our Insights/The next normal in construction/The-next-normal-in-construction.pdf.
Bock, T. (2007). Construction Robotics. Auton. Robot. 22 (3), 201–209. doi:10.1007/s10514-006-9008-5
Bonwetsch, T., Kobel, D., Gramazio, F., and Kohler, M. (2006). “The Informed Wall: Applying Additive Digital Fabrication Techniques on Architecture.” in Synthetic Landscapes - ACADIA 2006 International Conference. 489–495.
Chea, C. P., Bai, Y., Pan, X., Arashpour, M., and Xie, Y. (2020). An Integrated Review of Automation and Robotic Technologies for Structural Prefabrication and Construction. Transp. Saf. Environ. 2 (2), 81–96. doi:10.1093/tse/tdaa007
Chokhachian, A., Perini, K., Giulini, S., and Auer, T. (2020). Urban Performance and Density: Generative Study on Interdependencies of Urban Form and Environmental Measures. Sustain. Cities Soc. 53 (February), 101952. doi:10.1016/j.scs.2019.101952
Chokhachian, A., Perini, K., Dong, S., and Auer, T. (2017). “How Material Performance of Building Facade Affect Urban Microclimate,” in Powerskin Conference 2017, Munich, Germany, 13. Available at: https://www.ar.tum.de/fileadmin/w00bfl/klima/Publikationen/Fachartikel/Powerskin2017_Material-Performance-of-Building.pdf.
Church, E., Miranda, C., Szibbo, N., Elliott, R., and Cranz, G. (2012). Cities and People Project: A White Paper on Human Interaction with the Built Environment Institute for Environmental Entrepreneurship We Are Thankful to Arup Global Research. Available at: https://doi.org/10.13140/RG.2.1.4062.0889.
Craney, R., and Adel, A. (2020). “Engrained Performance,” in ACADIA 2020: Distributed Proximities/Volume I: Technical Papers. Editors B. Slocum, V. Ago, S. Doyle, A. Marcus, M. Yablonina, and M. del Campo, 604–613. Available at: http://papers.cumincad.org/data/works/att/acadia20_604.pdf.
Dörfler, K., Hack, N., Sandy, T., Giftthaler, M., Lussi, M., Walzer, A. N., et al. (2019). Mobile Robotic Fabrication beyond Factory Conditions: Case Study Mesh Mould Wall of the DFAB HOUSE. Construction Robotics 3 (1–4) 53–67. doi:10.1007/s41693-019-00020-w
Dörfler, K., Sandy, T., Giftthaler, M., Gramazio, F., Kohler, M., and Buchli, J. (2016). “Mobile Robotic Brickwork,” in Robotic Fabrication in Architecture, Art and Design 2016 (Cham: Springer International Publishing), 204–217. doi:10.1007/978-3-319-26378-6_15
Elet, Y. (2002). Seats of Power: The Outdoor Benches of Early Modern Florence. J. Soc. Archit. Hist. 61 (4), 444–469. doi:10.2307/991868
Elhouar, S., Alzarrad, M. A., and Elhouar, S. (2019). “A Synopsis of 3D Printing and Robotics Applications in Construction,” in Proceedings of the Creative Construction Conference 2019, Budapest, June 2019 (Budapest University of Technology and Economics), 769–775. doi:10.3311/CCC2019-105
Fastbrick Robotics (2020). FBR (Fastbrick Robotics) | Industrial Automation Technology. Available at: https://www.fbr.com.au/ (Accessed April 15, 2022).
Gardner, J., Marpillero-Colomina, A., and Begault, L. (2018). “Inclusive Healthy Places - A Guide to Inclusion and Health in Public Space: Learning Globally to Transform Locally,” 75. Available at: https://gehlinstitute.org/wp-content/uploads/2018/07/Inclusive-Healthy-Places_Gehl-Institute.pdf%0Ahttps://gehlinstitute.org/work/inclusive-healthy-places/.
Gramazio, F., Kohler, M., and Willmann, J. (2013). The Robotic Touch: How Robots Change Architecture. Zurich: Park Books.
Gramazio, F., and Kohler, M. (2008). “Digital Materiality in Architecture: Bridging the Realms of the Virtual and Physical,” in Explorations in Architecture : Teaching, Design, Research,. Editor R. Geiser (Basel: Birkhäuser), 179–199.
Guide to Climate Change Adaptation in Cities (2011). The International Bank for Reconstruction and Development. Washington D.C: The World Bank. Available at: https://openknowledge.worldbank.org/handle/10986/27396.
Han, I. X., Meggers, F., and Parascho, S. (2021). Bridging the Collectives: A Review of Collective Human-Robot Construction. Int. J. Archit. Comput. 19 (4), 512–531. doi:10.1177/14780771211025153
Han, R., Xu, Z., and Qing, Y. (2017). Study of Passive Evaporative Cooling Technique on Water-Retaining Roof Brick. Procedia Eng. 180, 986–992. doi:10.1016/j.proeng.2017.04.258
Hartnett, J. (2008). Si Quis Hic Sederit: Streetside Benches and Urban Society in Pompeii. Am. J. Archaeol. 112 (1), 91–119. doi:10.3764/aja.112.1.91
He, J., and Liu, K. Q. (2012). Numerical Analysis of Passive Microclimatic-Modifying Effects of a Moist Void-Brick Wall. Amm 193-194 (August), 1156–1164. doi:10.4028/www.scientific.net/AMM.193-194.1156
Klemm, W., Heusinkveld, B. G., Lenzholzer, S., Jacobs, M. H., and Van Hove, B. (2015). Psychological and Physical Impact of Urban Green Spaces on Outdoor Thermal Comfort during Summertime in The Netherlands. Build. Environ. 83, 120–128. doi:10.1016/j.buildenv.2014.05.013
Laakkonen, S. (2020). Urban Resilience and Warfare: How Did the Second World War Affect the Urban Environment? City Environ. Interact. 5 (March), 100035. doi:10.1016/j.cacint.2020.100035
Lau, K. K.-L., Shi, Y., and Ng, E. Y.-Y. (2019). Dynamic Response of Pedestrian Thermal Comfort under Outdoor Transient Conditions. Int. J. Biometeorol. 63, 979–989. doi:10.1007/s00484-019-01712-2
Lau, K. K.-L., Tan, Z., Morakinyo, T. E., and Ren, C. (2022). “Outdoor Thermal Comfort in Urban Environment,” in SpringerBriefs in Architectural Design and Technology (Singapore: Springer Singapore). doi:10.1007/978-981-16-5245-5
Leggero, R. (2018). “Citizens and Climate Microclimatic Patterns in Medieval Cities (Northern Italy),” in The Urban Microclimate as Artifact. Editors S. Roesler, and M. Kobi (Birkhäuser), 44–63. doi:10.1515/9783035615159-044
Lenzholzer, S., Klemm, W., and Vasilikou, C. (2018). Qualitative Methods to Explore Thermo-Spatial Perception in Outdoor Urban Spaces. Urban Clim. 23 (March), 231–249. doi:10.1016/j.uclim.2016.10.003
Marunmale, A. K., and Attar, A. C. (2014). “Designing, Developing and Testing of Cellular Lightweight Concrete Brick (CLC) Wall Built in Rat-Trap Bond,” in Current Trends in Technology and Science 3, 331–336. Available at: https://www.ctts.in/assets/upload/1596ctts-340117 vol-3 iss-4.pdf.
Mitterberger, D., Dörfler, K., Sandy, T., Salveridou, F., Hutter, M., Gramazio, F., et al. (2020). Augmented Bricklaying. Constr. Robot. 4 (3), 151–161. doi:10.1007/s41693-020-00035-8
Molter, P. L., and Chokhachian, A. (2021). “Experimental Study of Geometric Configuration and Evaporative Cooling Potential of Brick Elements,” in Eco-Efficient Materials for Reducing Cooling Needs in Buildings and Construction (Elsevier), 101–115. doi:10.1016/B978-0-12-820791-8.00006-7
Moughtin, C., and Mertens, M. (2003). Urban Design: Street and Square. Architectural Press. Available at: https://books.google.de/books?id=xMDRV10oURwC.
Negev, M., Khreis, H., Rogers, B. C., Shaheen, M., and Erell, E. (2020). City Design for Health and Resilience in Hot and Dry Climates. BMJ 46 (11), m3000. doi:10.1136/bmj.m3000
Ningrum, W. (2018). Urban Heat Island towards Urban Climate. IOP Conf. Ser. Earth Environ. Sci. 118 (1), 012048. doi:10.1088/1755-1315/118/1/012048
O’Malley, C., Piroozfarb, P. A. E., Farr, E. R. P., and Gates., J. (2014). An Investigation into Minimizing Urban Heat Island (UHI) Effects: A UK Perspective. Energy Procedia 62 (December), 72–80. doi:10.1016/j.egypro.2014.12.368
Peng, Y., Feng, T., and Timmermans, H. J. P. (2021). Heterogeneity in Outdoor Comfort Assessment in Urban Public Spaces. Sci. Total Environ. 790 (October), 147941. doi:10.1016/j.scitotenv.2021.147941
Rahman, M. A., Franceschi, E., Pattnaik, N., Moser-Reischl, A., Hartmann, C., Paeth, H., et al. (2022). Spatial and Temporal Changes of Outdoor Thermal Stress: Influence of Urban Land Cover Types. Sci. Rep. 12, 1–13. doi:10.1038/s41598-021-04669-8
Rizwan, A. M., Dennis, L. Y. C., and Liu, C. (2008). A Review on the Generation, Determination and Mitigation of Urban Heat Island. J. Environ. Sci. 20 (1), 120–128. doi:10.1016/S1001-0742(08)60019-4
Roesler, S., and Kobi, M. (2018). “Microclimates and the City,” in The Urban Microclimate as Artifact. Editors S. Roesler, and M. Kobi (Birkhäuser), 12–25. doi:10.1515/9783035615159-012
Santamouris, M. (2014). Cooling the Cities - A Review of Reflective and Green Roof Mitigation Technologies to Fight Heat Island and Improve Comfort in Urban Environments. Sol. Energy 103 (May), 682–703. doi:10.1016/j.solener.2012.07.003
Shen, Y., and He, C. (2021). Smart Construction under the Background of Population Aging. J. Phys. Conf. Ser. 1992 (4), 042016. doi:10.1088/1742-6596/1992/4/042016
Tarabieh, K., Abdelmohsen, S., Hassan, A., El-dabaa, R., and Elghazi, Y. (2018). “Parametric Investigation of Brick Extrusion Patterns Using Thermal Simulation Department of Architecture, American University in Cairo, New Cairo, Egypt Department of Architecture, Ain Shams University, Cairo, Egypt Department of Architectural Engin,” in BSO2018 Papers, 4th Building Simulation and Optimization Conference, Cambridge, UK, 11–12 September, 2018, 597–604. Available at: https://www.researchgate.net/publication/326274259_Parametric_Investigation_of_Brick_Extrusion_Patterns_Using_Thermal_Simulation
Tarabieh, K., Sen, S. A. M., Elghazi, Y., El-Dabaa, R., Hassan, A., and Amer, M. (2017). Parametric Investigation of Three Types of Brick Bonds for Thermal Performance in a Hot Arid Climate Zone. Proc. 33rd PLEA Int. Conf. Des. Thrive, PLEA 3 (July), 3699–3706.
Ullah, Z., Khan, A., and Thaheem, M. J. (2018). “Comparison of Performance of Rat Trap Brick Bond,” in First International Conference on High Performance Energy Efficient Buildings and Homes (HPEEBH 2018), Lhore, Pakistan, 255–262. Available at: https://www.researchgate.net/publication/326915020_Comparison_of_performance_of_rat_trap_brick_bond_with_the_conventional_brick_bond
Wernery, J., Ben-Ishai, A., Binder, B., and Brunner, S. (2017). Aerobrick - an Aerogel-Filled Insulating Brick. Energy Procedia 134 (October), 490–498. doi:10.1016/j.egypro.2017.09.607
World Health Organization (2017). “LIBRO 3. Towards More Physical Activity in Cities,” in Towards More Physical Activity in Cities. November 2018: 96.
Keywords: climate-resilient design, microclimate simulation, robotic assembly, human-robot collaboration, human thermal perception
Citation: Fleckenstein J, Molter PL, Chokhachian A and Dörfler K (2022) Climate-Resilient Robotic Facades: Architectural Strategies to Improve Thermal Comfort in Outdoor Urban Environments using Robotic Assembly. Front. Built Environ. 8:856871. doi: 10.3389/fbuil.2022.856871
Received: 17 January 2022; Accepted: 27 April 2022;
Published: 24 May 2022.
Edited by:
Salman Shooshtarian, RMIT University, AustraliaReviewed by:
Mohamed H. Elnabawi Mahgoub, United Arab Emirates University, United Arab EmiratesAtef Ahriz, University of Tébessa, Algeria
Copyright © 2022 Fleckenstein, Molter, Chokhachian and Dörfler. This is an open-access article distributed under the terms of the Creative Commons Attribution License (CC BY). The use, distribution or reproduction in other forums is permitted, provided the original author(s) and the copyright owner(s) are credited and that the original publication in this journal is cited, in accordance with accepted academic practice. No use, distribution or reproduction is permitted which does not comply with these terms.
*Correspondence: Julia Fleckenstein, anVsaWEuZmxlY2tlbnN0ZWluQHR1bS5kZQ==