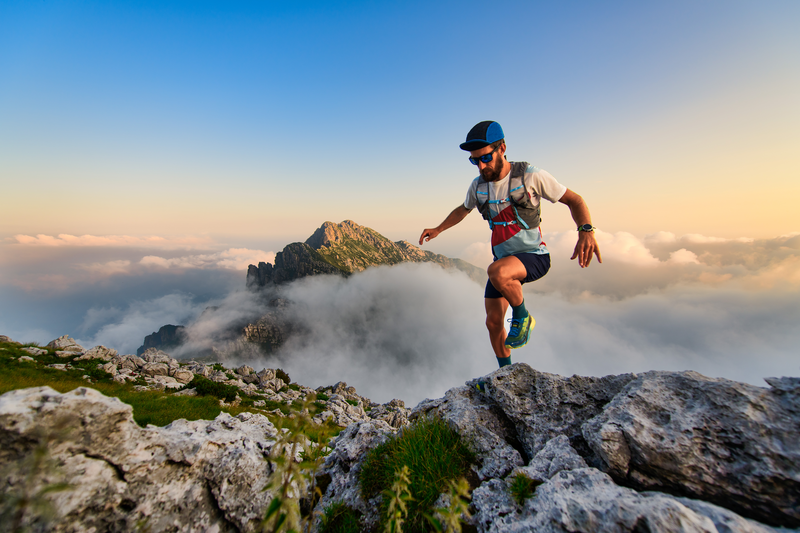
95% of researchers rate our articles as excellent or good
Learn more about the work of our research integrity team to safeguard the quality of each article we publish.
Find out more
ORIGINAL RESEARCH article
Front. Built Environ. , 04 November 2022
Sec. Indoor Environment
Volume 8 - 2022 | https://doi.org/10.3389/fbuil.2022.1014473
This article is part of the Research Topic Sustainable and Eco-friendly Building Materials View all 5 articles
The study has two objectives. First, it experimentally measures the indoor and outdoor temperatures of a building in Peshawar and conducts validation with CFD modeling. Second, it simulates the building with the addition of locally available, natural, and recycled insulator materials on the rooftop to keep the indoor environment within a comfortable temperature range, especially in the winter and summer seasons. To achieve these objectives, experimental temperature data for January and June were recorded and validated, followed by a simulation, using ANSYS-Fluent 16 CFD, of the residential building with the application of waste thermal insulators such as straw bale, sheep wool, and recycled glass materials on the rooftop to reduce the indoor temperature. Experimental temperature measurement showed that the lowest recorded indoor temperature was 15°C on 2 January 2022 and that the highest recorded indoor temperature was 41°C on 11 June. The predicted and validated temperature results were similar, with a slight difference of less than 15%. Recycled glass positively and significantly reduced the indoor temperature in summer by 10.2% and thermal amplitude by 48.3%, with a time lag increase of 100% and an increase in the period of comfort hours of 380%. In winter, the daily average temperature increased by 7.4%, thermal amplitude was reduced by 59.3%, and the time lag increased by 100% in comparison with the baseline case results. The study concludes that recycled glass distribution gives the best improvement compared to straw bale and sheep wool.
Over the past few decades, unplanned development in Peshawar city and Pakistan’s Khyber Pakhtunkhwa (KP) province has been a key cause of discomfort in its residential buildings. The country’s highly ranked climate effects are being explored in order to overcome issues of thermal discomfort in its buildings and to achieve optimal utilization of energy. Sustainable development and the utilization of fewer thermally conductive materials significantly reduce energy consumption and increase thermal comfort in buildings (Mirrahimi et al., 2016; Shafigh et al., 2018). Global warming is affecting the earth’s temperature (Ahmad et al., 2019) and, as a result, thermal discomfort in residential buildings has increased. The thermal comfort properties and heat conductivity of construction materials are important to study before their use in buildings. The demand for higher thermal comfort in buildings has increased (Yang et al., 2014). Globally, buildings consume about 40% of energy and produce an estimated 35% of carbon dioxide (CO2) emissions (Yang et al., 2014; Ahmad et al., 2021a). The heating and cooling of buildings consume a substantial amount of energy (Shafigh et al., 2018). Residential energy consumption significantly increased during the COVID-19 lockdown period, when people across the world were spending more time at home (Krarti and Aldubyan, 2021). Energy consumption in buildings located in harsh climatic zones poses a significant challenge, especially in countries going through energy crises.
Pakistan is among the countries with four seasons, and normally the weather is arid and hot in summer and cold in winter. Summer season in Pakistan starts in April and ends in August; however, June is extremely hot, and August is monsoon season. Winter season in Pakistan starts in November and ends in March; however, December and January have considerably dry and cold weather (Yang et al., 2014; Ahmad et al., 2021a). Indoor temperatures were recorded for buildings in the city of Peshawar, the densely populated city in KP province. KP is a topographically diverse province situated in the northwest region of Pakistan. Its weather cycle is extremely hot in summer, up to 50°C, and its winters are dry and cold at 3°C, with moderate rainfall annually (Shah et al., 2012; Wahid et al., 2018). The Global Climate Risk Index 2020 (Eckstein et al., 2019) ranked Pakistan as the fifth most vulnerable country. Pakistan’s contribution to greenhouse gas emission and global warming is negligible, yet it is among the top-listed climate change-affected countries because of its large-scale deforestation, rapid and unmanaged urbanization, and inactive and neglected environmental ministry (Mahar et al., 2019).
Climate change is directly linked with global warming, heatwaves, and temperature increases (Bartholy and Pongrácz, 2018; Eckstein et al., 2019; Semahi et al., 2019). In the past, thermal comfort has been achieved using less-conductive materials such as wood, grass, and leaves, which are no longer used in modern building systems. Rijal et al. (2007) evaluated the relationship between window adjustment and indoor thermal comfort. In modern buildings, most of a building’s materials are good heat conductors, which increases the thermal conductivity of the building; as a result, occupants suffer from discomfort in the winter and summer. Modern buildings are equipped with mechanical systems for controlling indoor temperature. No study has discussed thermal comfort in the buildings of Pakistan.
The study had two objectives: measurement of indoor temperature and simulation of a building upon the installation of locally available, natural, and recycled insulator materials on its rooftop. The theoretical significance of the study is its utilization of waste materials with lower thermal conductivities to improve thermal comfort in buildings, and it also has a practical contribution given that Pakistan is experiencing huge energy crises at the same time as it is facing climate impacts. Building thermal comfort, as achieved by the utilization of waste materials, will significantly reduce the overall contribution of greenhouse gas emissions from buildings.
Green building development attempts to create environmentally sustainable buildings which significantly improve indoor thermal comfort, but its initial high cost has caused it to be greatly discouraged in Pakistan, especially among people budgeting for low-cost buildings (Yang et al., 2014). Thus, more practical steps—such as installing recycled and economical materials with low thermal conductivity on the rooftops and walls of a structure—may improve indoor thermal comfort. The rooftops and walls of a building are exposed to direct sunlight, and major solar heat transfer occurs through its roofing and walls. Figure 1 shows the conduction process of heat transfer through the roof into the indoor building.
Horizontal and plain building rooftops absorb higher solar radiation intensity because of their direct exposure to sunlight and maximum solar irradiation as compared to the vertical walls of the building (Zingre et al., 2015; Ahmad et al., 2021b). Major thermal conduction in indoor buildings occurs through the rooftop, and its improvement would significantly reduce their energy consumption (Ahmad et al., 2021b). Therefore, thermal insulation on the rooftop is one of the most effective strategies for achieving energy conservation and thermal comfort during cold winters and warm summers in a composite climate. Thermal insulation reduces unwanted heat loss and gain and hence decreases the energy demands of heating and cooling systems (Ascione et al., 2013; Berardi and Naldi, 2017). Straw bales, used as a building material, significantly reduce the solar heat effect. Ahmad et al. (2021a) have highlighted the notable effects of straw bale utilization in buildings and have obtained encouraging results.
ASHRAE (ASHRAE, 2013; Ličina et al., 2018) has defined thermal comfort as the “condition of mind that expresses satisfaction with the surrounding environment temperature.” Humankind spends most of its time indoors in housing, commercial, and office buildings; therefore, construction materials play an important role in building structure design. Fundamentally, the heat balance model and the adaptive model are used to determine thermal comfort. The heat balance model assumes that thermal comfort is when the human body expends less effort for thermal comfort, which could be achieved through mechanical processing such as air conditions or heaters in the building. The adaptive model describes human nature as interacting with the environment and adapting to its condition without the installation of mechanical equipment (Binici et al., 2010; ASHRAE, 2013; Toe and Kubota, 2013; Haba et al., 2017; Cozzarini et al., 2020). In naturally ventilated buildings, the adaptive model perfectly provides flexibility where indoor temperature and outdoor temperature are assumed to be similar. Eq. 1 expresses the optimum comfort temperature in a ventilated building under the adaptive model (Yao et al., 2009; Heirung et al., 2017):
where
where
Thermal insulation materials are constantly subjected to climatic stress conditions; therefore, materials should meet the standard requirement before they are considered for use in a building. The thermal and mechanical properties of the materials must meet international standards such as ASTM, EN, ISO, and BS standards. Available thermal insulator materials are classified as organic or non-organic and as commercial or non-commercial materials (Abu-Jdayil et al., 2019):
Fibrous insulations (e.g., ceramic fiber, glass mineral wool, and rock mineral wool).
Cellular insulations (e.g., polyurethane, polystyrene, and polypropylene).
Granular insulations (e.g., calcium silicate, perlite expanded, and vermiculate).
Materials with thermal conductivity λ > 0.08 W/mK are considered poor insulators, and materials with thermal conductivity λ < 0.05 W/mK are considered good insulators (Kumar and Suman, 2013). Polyisocyanurate is the most widely used roof insulation material in North America, covering more than 50% of all commercial new or re-roofing applications. The thermal resistance of polyisocyanurate is higher than that of fiberglass or rock wool insulation (Lucero-Álvarez et al., 2016). The most common roofing system used in residential buildings in Pakistan is comprised of a concrete slab over a thermal sheet and a layer of mud or clay tiles. Polyurethane/polyisocyanurate foam, extruded and expanded polystyrene, mineral fiber, and fiberglass insulation materials are commonly used in Saudi Arabia (Abu-Jdayil et al., 2019).
Selecting an appropriate thermal insulation material depends mainly on the thermal properties of the materials and on the climate conditions of the installation area. As mentioned previously, the KP state of Pakistan has extremely cold and hot weather. This study considers two additional important parameters for the selection of thermal insulator materials: they should be made of natural or recycled material and must be available locally in Pakistan to reduce the cost. The study has chosen the five most commonly available natural and recycled thermal insulators.
Sheep wool is a natural, renewable, and sustainable material. It has generally been used in the textile industry, but advanced construction engineering has taken it to the next level by using it in construction to maintain thermal comfort in residential buildings (Abu-Jdayil et al., 2019). Sheep wool’s thermal conductivity is between 0.038 and 0.054 W/mK, and its specific heat is between 1.3 and 1.7 kJ/kgK, so it is considered a good thermal insulator material. The performance of sheep wool material has been found to be useful in the winter but poor in the summer (Zach et al., 2012). However, sheep wool can be effectively used in construction to maintain thermal comfort in buildings. Straw bale can be used to protect dwellings against weather factors and thermal effects and is recommended for use in walls and on building roofs (Ahmad et al., 2021a). On various occasions, natural and recycled thermal insulation material has been used worldwide to address the problem of thermal heat effects in dwellings. The most recommended materials are natural fibers such as straw bale, date palm, sheep wool, and rice husk, which have the lowest thermal conductivity, and recycled materials such as waste glass and polymer materials that effectively reduce the room temperature if used on the rooftop (Abu-Jdayil et al., 2019).
Thermal insulation materials do not stop heat transfer but only reduce the rate of heat transfer. Thicker layers of thermal insulation material allow a minimum rate of heat transfer but increase the cost of the thermal insulator (Kim and Moon, 2009). The optimal thickness of the insulation for allowing minimum heat transfer during the conduction process is 3–15 mm, as determined by various researchers, and varies depending on material types and their properties. Several studies have examined the effects of using natural and recycled thermal insulation materials, but many of these did not provide sufficient information other than a finding of thermal conductivity and recommendations showing that these materials are suitable for use in buildings to achieve thermal comfort. This study compares the use of three different natural and recycled materials using CFD simulation with real temperature data collected from Peshawar, Pakistan; predicted temperature is also compared. Table 1 lists commercial and non-commercial thermal insulation materials and their properties.
The study location selected was Peshawar city because of its weather conditions in summer and winter. Most of the year, the weather is dry and out of the comfort zone for occupants of contemporary-design houses, drastically increasing electricity consumption in the area.
The structural sketch of a residential building design commonly used in Peshawar is shown in Figure 2. Its total covered area is about 200 m2, with a floor height of about 3 m. The house has five rooms excluding the kitchen area. The building is comprised of reinforced concrete, cement blocks, and cement-mortar plaster and has a modern architectural style.
The indoor and outdoor temperatures of the building were measured during winter (January 2021) and summer (June 2021). Indoor and outdoor air temperatures were measured using a Hot Wire Anemometer Data Logger (HHF-SD1 Model ± 0.4% + 0.5°C). Surface temperatures of the building were measured using a HOBO series data logger (UX120 ± 0.21°C). The building’s indoor and outdoor structures were marked into 26 points for each room and were subdivided into called points. At each point, temperatures were recorded for the whole months of January and of June.
Table 1 shows the point division for each room. Inside and outside air temperatures in each room were measured about 1.5 m above the floor. The data measured at these points were used to evaluate the effectiveness of the current roofing system as well as to validate the 3D CFD model of the building. The other points (P6 to P26) were located on the outside surface of the building on its roof, vertical walls, windows, and doors. The measurements at these points were taken at the central area of each surface. The measurement data at these points were used to specify the boundary conditions of the developed 3D CFD model of the building. The measured locations and the usage of measured data are summarized in Table 2.
Roofs are highly susceptible to solar radiation and other environmental changes and thereby influence the internal temperature conditions of the occupants (Zach et al., 2012; Kumar et al., 2016; Mahar et al., 2019; Semahi et al., 2019). Selecting an appropriate thermal insulation material depends mainly on the thermal properties of the materials, including their thermal conductivities. This study considered two further important parameters for the selection of thermal insulator materials: they should be natural or recycled materials and must be available locally in Peshawar at the lowest cost possible. This study focused on the straw bale, recycled waste glass, and sheep wool thermal insulators commonly available in waste materials in Pakistan, as they can be reused based on their properties, which are shown in Table 3.
The suitable locations and positioning of the insulation materials on the roof were configured based on the dynamic thermal behavior of the roof, at four locations on the rooftop (the inner, outer, and middle roof surface and the distribution across the roof structure). An ANSYS-Fluent 16 CFD model was developed, with simulation factored on daily average temperature, thermal amplitude, time lag, and period of comfort. The daily average temperature was measured, and its thermal amplitude—defined as the difference between the
FIGURE 3. Graphical representation of thermal amplitude, time lag, and average daily indoor temperature (Ascione et al., 2013).
The daily average temperatures for January and June 2021 were recorded in the building at 26 selected points as discussed in Methodology.
Daily average temperatures for June and January are shown in Supplementary Tables S4, S5 and graphically represented in Figure 4 and Figure 5, respectively. Figure 4 shows that the average daily minimum indoor temperature on the 2nd day of January was 7.3°C in room 1, followed by 7.5°C in room 3 and room 5.
Figure 5 shows an average daily maximum indoor temperature for 11th June 2021, with room 5 having the highest temperature of 33°C, followed by 32.8°C in room 4 and 32.7°C in room 3, whereas room 1 and room 2 had the lowest, at 32.5°C. The occurrence of lowest and highest temperatures throughout the year depends on the housing location’s area, direction of room facing, solar irradiation occurrence, and building materials, as well as the indoor occupancy of the room or house.
Figure 6 indicates that the recorded outdoor daily average temperatures were 15°C in January and 41°C in June, which are further validated by the average annual temperature in Peshawar (Peshawar Meteorological Department Data, 2021) as shown in Figure 7.
The recommended ASHRAE Standard 55 for thermal comfort indoors is 23–26°C in summer and 18.2–25.2°C in winter (Olesen and Brager, 2004). However, the study found that the indoor thermal comfortability of the house was not suitable and that indoor temperature occurrence was not in line with ASHARE recommendations. The data for both seasons show that indoor temperature occurrence is not in the comfort range, so the application of thermal insulation is essential for improving thermal comfort in the building.
The experimentally recorded hourly temperature data for January and June 2021, as shown in Supplementary Tables S3, S4, has been validated with predicted data using CFD modeling simulation. The outdoor temperature recorded was cross-validated with the Peshawar Meteorological Department’s annual temperature data for 2021, as shown in Figure 7. The average recorded maximum temperature in June was 41°C and the lowest was 15°C in January, which is in line with our measured outdoor field-temperature data.
A CFD (Ansys Mesh-R16 software) method was used to develop a 3D model to predict temperatures inside the building. A simplified 3D geometry of the building, as shown in Figure 2, was constructed using AutoCAD at the actual dimensions used at the Pakistan site. The building has an overall size of length = 27 m, width = 15 m, and height = 3.5 m. The building has 3 doors and 13 windows on the outside vertical wall. The door dimensions are 1.2 m in width and 2.0 m in height, while the dimensions of the windows are 1.2 m in width and 1.4 m in height. Ansys’s (Mesh-R16) unstructured cut-cell grids model was adopted to predict the temperature in the building. Predicted and experimental temperature data graphs are shown in Figure 8 and Figure 9 and show that the field data and CFD prediction model data are very close. Hence, the model was validated.
A parametric study was performed to identify the most suitable type of insulator material and thermal insulation layer location for application on the building’s roof for offering the greatest improvement in indoor thermal comfort during summer and winter. Straw bale, sheep wool, and recycled glass were used as insulation materials since they have lower conductivity and are locally available. The roof was configured using CFD simulation, and three cases were run for selected insulator materials distributed on the rooftop to examine their effects on indoor temperature. Factors affecting thermal comfort were compared, including the daily average temperature, thermal amplitude, time lag, and period of comfort.
Boundary conditions were defined based on actual field temperature data, as listed in Supplementary Table S3 for June and Supplementary Table S4 for January 2021. The highest temperature on 11th June and lowest temperature on 2nd January were selected, and the properties of the air, concrete, glass, and wood were obtained from the ASHRAE Standard 55 literature (Mirrahimi et al., 2016; Agoudjil et al., 2011; American Society of Heating, 2017; Kumar et al., 2016; Singh et al., 2016).
Table 4 indicates that the installation of thermal insulation materials on the roof had a significant positive effect in summer compared to the winter distribution on the rooftop. The results show that the application of insulation materials decreased the average room temperature in summer, with a reduction of up to 10.2% relative to the baseline case with thermal insulation materials. Recycled glass was the best-performing thermal insulation material. The thermal amplitude of thermal insulation materials shows a positive impact, and the lowest thermal amplitude was obtained when recycled glass thermal insulation material was distributed on the roof. The reduction in thermal amplitude with recycled glass insulation materials distributed on the roof reached 48.3% relative to the baseline case.
Time lag showed significant improvement, ranging from 7 h to 10 h, which is a 40–100% increment. The performance of the different insulation materials while distributed on the rooftop was almost identical and showed a positive impact. Of the studied cases, the best improvement rate for time lag—of up to 10 h, equivalent to a 100% increment relative to the baseline case—was obtained when recycled glass was distributed on the roof. The distribution of insulation materials on the rooftop had a positive effect, reducing the indoor temperature in summer and increasing the period of thermal comfort. Recycled glass showed the best performance and significantly improved indoor thermal comfort in summer, compared to straw bale and sheep wool materials. Recycled glass insulation material distributed on the rooftop has a higher potential to reduce both average temperature and thermal amplitude and to increase the lag time and period of comfort inside the room in summer, as compared to the other insulation materials.
Likewise, findings for daily average temperature, thermal amplitude, time lag, period of comfort, and their respective percentage changes for the winter season are listed in Table 4. Given the objective of attaining a comfortable environment for building occupants in summer and winter, the temperature must be increased in summer and decreased in winter to reach the comfort range.
The simulation result showed that distributing recycled glass on the roof significantly increased the temperature by 7.4%. Installing thermal insulation materials on the roof had a significantly positive effect on the all-thermal insulation materials. The comparison of the insulation materials findings shown in Table 4 indicates that recycled glass was the best thermal insulation material to be installed on the roof. Temperature, thermal amplitude, and time lag significantly improved with recycled glass as compared to straw bale and sheep wool. There was no achievement of thermal comfort in winter with recycled glass; however, the effect of thermal insulation materials was evident, as it helped to shift the time of occurrence of peak heat load and helped to reduce temperature fluctuations.
The impact of distributing recycled glass on roof temperature variation for 24 hours in summer and winter cases is plotted relative to the baseline in Figures 10, 11 and indicates that using thermal insulator materials can achieve thermal comfortability in a room.
FIGURE 10. Temperature variation over 24 h with recycled glass insulation material distributed on the roof in summer.
FIGURE 11. Comparison of temperature variation between recycled glass distribution and baseline case for roof and room 1 in winter.
The study has two objectives, first to experimentally measure indoor and outdoor temperatures in the Peshawar building and to validate it using ANSYS-Fluent 16 CFD modeling and, second, to simulate the building with locally available, natural, and recycled insulator materials used on the rooftop to keep the indoor environment within a comfortable temperature range, especially in the winter and summer seasons. The heating and cooling of buildings consume a substantial amount of energy (Shafigh et al., 2018; Mahar et al., 2019). Rooftops and walls of a structure are exposed all day to sunlight, and a major component of heat transfer occurs through the roofing and walls of a building. The horizontal and plain rooftops of buildings absorb higher solar radiation intensity compared to the vertical walls of the building (Zingre et al., 2015; Ahmad et al., 2021b). Major thermal conduction in indoor buildings occurs through the rooftop, and improvement here would significantly reduce the energy consumption of the building (Ahmad et al., 2021b). Therefore, rooftop thermal insulation is one of the most effective strategies for achieving energy conservation and thermal comfort during cold winters and warm summers in a composite climate. Thermal insulation reduces unwanted heat loss or gain and hence decreases the energy demands of heating and cooling systems (Ascione et al., 2013; Berardi and Naldi, 2017). Ahmad et al. (2021a) have highlighted the notable effects of straw bale utilization in the buildings and achieved encouraging results.
Daily average indoor temperatures were 9°C in January and 28°C in June; these measurements show that buildings are thermally discomforted as their temperature readings do not meet the ASHRAE Standard 55 recommendations of 25–27°C for summer and 17.2–23.2°C for winter (Olesen and Brager, 2004; Singh et al., 2016). The validation of field temperature data with computational analysis shows significant validity with less than a 5% difference. These findings suggest that the existing roofing system for the buildings is incapable of providing adequate thermal comfort to occupants and that the application of thermal insulation is essential to improve thermal comfort in the building. The conventional building simulation was analyzed by CFD, and thermal insulators were applied one by one on four different locations on the rooftop, and results were obtained. First, the thermal effect of the straw bale was examined, and findings indicated that installing this insulation material on the rooftop had a slight effect on indoor temperature: it slightly reduced the temperature during summer and slightly increased it during winter. However, the temperature was still out of the range specified by the ASHRAE Standard 55 and the literature. Ahmad et al. (Ahmad et al., 2021a) have highlighted the notable effects of straw bale utilization on buildings, finding indoor temperatures to be slightly reduced, but did not achieve the ASHRAE-recommended standard.
In the second attempt, sheep wool thermal insulation was examined. Findings show a slight reduction in the indoor temperature in summer and minor improvement in the winter; however, the ASHRAE Standard 55 threshed value was not achieved.
Recycled glass was examined by installation at four different locations on the rooftop and produced a 7.4% indoor temperature increase in January and a 10.2% indoor temperature decrease in summer. Thus, recycled glass installation provides the best indoor thermal comfort compared to straw bale and sheep wool. Thermal comfort is important in reducing excessive demand on the electrical grid during peak hours. By reducing peak heat load and flux, smaller-capacity air conditioning equipment would be required, and these achievements would increase the energy efficiency of the overall system, ultimately moving toward an environment- and climate-friendly environment.
This study’s findings are:
1. Experimental measured and predicted temperature data show similar results for January and June in Peshawar, Pakistan. Study findings showed that 2nd January has the lowest (7.6°C) and 11th June has the highest (36.4°C) indoor building air temperature.
2. Indoor air temperature variations in winter and summer did not meet the ASHRAE Standard 55, which has recommended values of 23.9–30.9°C for summer and 18.2–25.2°C for winter. The existing roof system is not capable of providing a comfortable environment to occupants.
3. The study concluded that thermal insulation materials (straw bale, sheep wool, and recycled glass) positively and significantly improve temperatures in winter and summer.
4. The study found that recycled glass distribution gave the best improvement compared to straw bale and sheep wool, which are thermal insulation materials. Improvement was recorded for both seasons: in summer, the reduction in daily average temperature was 10.2%, the reduction in thermal amplitude was 48.3%, the increment in time lag was 100%, and the increment in the period of comfort hours was 380%.
5. In winter, the daily average air temperature increased by 7.4%, the thermal amplitude decreased by 59.3%, and the time lag increased by 100% in comparison with the baseline case results.
6. Overall, the results of this research confirm that the thermal insulation of a building’s roof has a significant impact on indoor air temperature. The theoretical implication of the study is that the utilization of waste material with lower thermal conductivity improves thermal comfort in buildings and has a practical contribution since Pakistan is enduring huge energy crises at the same time as the country is facing climate impacts. Achieving a building’s thermal comfort by utilizing waste material will significantly reduce its overall contribution to greenhouse gas emissions. This study is geographically limited to the Peshawar city. The general building design is another limitation, as it differs based on location. Importantly, only indoor and outdoor air temperature were recorded while humidity was ignored; the study was also limited to rooftop thermal conduction, and vertical walls, windows, and doors could be considered in the future. Importantly, the study concentrated on locally available waste or recycled materials, but non-commercial materials could also be used in the future. In the future, it is recommended to conduct a study on the effects of changing the thickness of the thermal insulation material, and of using thermal insulation for the vertical walls of the building, on indoor temperatures; moreover, other factors such as humidity could also be measured.
The datasets presented in this study can be found in online repositories. The names of the repository/repositories and accession number(s) can be found in the article/Supplementary Material.
Conceptualization, JT and MuA, methodology, MuA and AM, Formal analysis, RA-D’i and MuA, investigation, AM, MuA, KR, and JT, writing-MuA and MaA, Funding Arrangement: MaA and KR.
The authors declare that the research was conducted in the absence of any commercial or financial relationships that could be construed as a potential conflict of interest.
All claims expressed in this article are solely those of the authors and do not necessarily represent those of their affiliated organizations, or those of the publisher, the editors, and the reviewers. Any product that may be evaluated in this article, or claim that may be made by its manufacturer, is not guaranteed or endorsed by the publisher.
The Supplementary Material for this article can be found online at: https://www.frontiersin.org/articles/10.3389/fbuil.2022.1014473/full#supplementary-material
Abu-Jdayil, B., Mourad, A. H., Hittini, W., Hassan, M., and Hameedi, S. (2019). Traditional, state-of-the-art and renewable thermal building insulation materials: An overview. Constr. Build. Mater. 214, 709–735. doi:10.1016/j.conbuildmat.2019.04.102
Agoudjil, B., Benchabane, A., Boudenne, A., Ibos, L., and Fois, M. (2011). Renewable materials to reduce building heat loss: Characterization of date palm wood. Energy Build. 43 (2-3), 491–497. doi:10.1016/j.enbuild.2010.10.014
Ahmad, M., Al-Dala, R. N., Beddu, S., and Itam, Z. B. (2021). Thermo-physical properties of graphite powder and polyethylene modified asphalt concrete. Eng. Sci. doi:10.30919/es8d569
Ahmad, M., Al-Dala'ien, R. N., Ali, J., Beddu, S., and Manan, A. (2021). Straw bale installation on the roof top green projects effects in the indoor building temperature: Case study peshawar, Pakistan. J. Green Eng. 11, 122–140.
Ahmad, M., Beddu, S., and Alanimi, F. B. (2019). State of the art compendium of macro and micro energies. Adv. Sci. Technol. Res. J. 13 (1), 88–109. doi:10.12913/22998624/103425
Ahmad, M., Al-Dala, R. N. S., Beddu, S., and Itam, Z. B. (2021). Thermo-physical properties of graphite powder and polyethylene modified asphalt concrete. Eng. Sci. 17, 121–132. doi:10.30919/es8d569
American Society of Heating (2017). Refrigerating and air-conditioning engineers. Thermal environmental conditions for human occupancy: ANSI/ASHRAE standard 55-2017. (Supersedes ANSI/ASHRAE Standard 55-2013) Includes ANSI/ASHRAE Addenda Listed in Appendix N. Ashrae.
Ascione, F., Bianco, N., de’Rossi, F., Turni, G., and Vanoli, G. P. (2013). Green roofs in European climates. Are effective solutions for the energy savings in air-conditioning? Appl. Energy 104, 845–859. doi:10.1016/j.apenergy.2012.11.068
Ashrae, A. N. (2013). Standard 55-2013: Thermal environmental conditions for human occupancy. American Society of Heating, Refrigerating, and Air-Conditioning Engineers, Inc. Atlanta.
Bartholy, J., and Pongrácz, R. (2018). A brief review of health-related issues occurring in urban areas related to global warming of 1.5 C. Curr. Opin. Environ. Sustain. 30, 123–132. doi:10.1016/j.cosust.2018.05.014
Berardi, U., and Naldi, M. (2017). The impact of the temperature dependent thermal conductivity of insulating materials on the effective building envelope performance. Energy Build. 144, 262–275. doi:10.1016/j.enbuild.2017.03.052
Binici, H., Gemci, R., Aksogan, O., and Kaplan, H. (2010). Insulation properties of bricks made with cotton and textile ash wastes. Int. J. Mater. Res. 101 (7), 894–899. doi:10.3139/146.110348
Cozzarini, L., Marsich, L., Ferluga, A., and Schmid, C. (2020). Life cycle analysis of a novel thermal insulator obtained from recycled glass waste. Dev. Built Environ. 3, 100014. doi:10.1016/j.dibe.2020.100014
Eckstein, D., Künzel, V., Schäfer, L., and Winges, M. (2019). Global climate risk index 2020. Bonn: Germanwatch. https://www.germanwatch.org/sites/germanwatch.org/files/20-2 01e%20Global%20Climate%20Risk%20Index%202020_13.pdf.
Haba, B., Agoudjil, B., Boudenne, A., and Benzarti, K. (2017). Hygric properties and thermal conductivity of a new insulation material for building based on date palm concrete. Constr. Build. Mater. 154, 963–971. doi:10.1016/j.conbuildmat.2017.08.025
He, L. (2021). Improve thermal conductivity of polymer composites via conductive network. ES Mat. Manuf. 13, 1–2. doi:10.30919/esmm5f460
Heirung, T. A., Ydstie, B. E., and Foss, B. (2017). Dual adaptive model predictive control. Automatica 80, 340–348. doi:10.1016/j.automatica.2017.01.030
Kim, J. J., and Moon, J. W. (2009). Impact of insulation on building energy consumption. InEleventh Int. IBPSA Conf. Build. Simul.
Krarti, M., and Aldubyan, M. (2021). Review analysis of COVID-19 impact on electricity demand for residential buildings. Renew. Sustain. Energy Rev. 143, 110888. doi:10.1016/j.rser.2021.110888
Kumar, A., and Suman, B. M. (2013). Experimental evaluation of insulation materials for walls and roofs and their impact on indoor thermal comfort under composite climate. Build. Environ. 59, 635–643. doi:10.1016/j.buildenv.2012.09.023
Kumar, S. M., Ooka, R., and Bansal, N. (2016). The effect of cool roof on Built environment in composite and hot and dry climates of India. Tokyo: 空気調和・衛生工学会大会 学術講演論文集, 73–76. doi:10.18948/shasetaikai.2016.5.0_73
Ličina, V. F., Cheung, T., Zhang, H., De Dear, R., Parkinson, T., Arens, E., et al. (2018). Development of the ASHRAE global thermal comfort database II. Build. Environ. 142, 502–512. doi:10.1016/j.buildenv.2018.06.022
Lucero-Álvarez, J., Rodríguez-Muñoz, N. A., and Martín-Domínguez, I. R. (2016). The effects of roof and wall insulation on the energy costs of low income housing in Mexico. Sustainability 8 (7), 590. doi:10.3390/su8070590
Mahar, W. A., Verbeeck, G., Singh, M. K., and Attia, S. (2019). An investigation of thermal comfort of houses in dry and semi-arid climates of quetta, Pakistan. Sustainability 11 (19), 5203. doi:10.3390/su11195203
Mirrahimi, S., Mohamed, M. F., Haw, L. C., Ibrahim, N. L., Yusoff, W. F., and Aflaki, A. (2016). The effect of building envelope on the thermal comfort and energy saving for high-rise buildings in hot–humid climate. Renew. Sustain. Energy Rev. 53, 1508–1519. doi:10.1016/j.rser.2015.09.055
Olesen, B. W., and Brager, G. S. 2004. A better way to predict comfort: The new ASHRAE standard 55-2004. UC Berkeley: Center for the Built Environment. https://escholarship.org/uc/item/2m34683k.
Rijal, H. B., Tuohy, P., Humphreys, M. A., Nicol, J. F., Samuel, A., and Clarke, J. (2007). Using results from field surveys to predict the effect of open windows on thermal comfort and energy use in buildings. Energy Build. 39 (7), 823–836. doi:10.1016/j.enbuild.2007.02.003
Schiavoni, S., Bianchi, F., and Asdrubali, F. (2016). Insulation materials for the building sector: A review and comparative analysis. Renew. Sustain. Energy Rev. 62, 988–1011. doi:10.1016/j.rser.2016.05.045
Semahi, S., Zemmouri, N., Singh, M. K., and Attia, S. (2019). Comparative bioclimatic approach for comfort and passive heating and cooling strategies in Algeria. Build. Environ. 161, 106271. doi:10.1016/j.buildenv.2019.106271
Shafigh, P., Asadi, I., and Mahyuddin, N. B. (2018). Concrete as a thermal mass material for building applications-A review. J. Build. Eng. 19, 14–25. doi:10.1016/j.jobe.2018.04.021
Shah, S. A., Nisa, S., Khan, A., and Rahman, Z. U. (2012). Trends and variability in climate parameters of Peshawar district. Sci. Technol. Dev. 31 (4), 341–347.
Singh, M. K., Ooka, R., Rijal, H. B., and Mahapatra, S. (2016). “Building simulation-based study to improve thermal performance of a traditional residential house,” in Proceedings of the 5th international conference on human-environment system (ICHES), 1–10. November 2, 2016).Nagoya
Toe, D. H., and Kubota, T. (2013). Development of an adaptive thermal comfort equation for naturally ventilated buildings in hot–humid climates using ASHRAE RP-884 database. Front. Archit. Res. 2 (3), 278–291. doi:10.1016/j.foar.2013.06.003
Wahid, U., Takaaki, N., Muhammad, N., Rahman, Z., and Muhammad, A. (2018). Understanding climate change vulnerability, adaptation and risk perceptions at household level in Khyber Pakhtunkhwa, Pakistan. Int. J. Clim. Chang. Strateg. Manag. 10 (3), 359–378. doi:10.1108/IJCCSM-02-2017-0038
Yang, L., Yan, H., and Lam, J. C. (2014). Thermal comfort and building energy consumption implications–a review. Appl. energy 115, 164–173. doi:10.1016/j.apenergy.2013.10.062
Yao, R., Li, B., and Liu, J. (2009). A theoretical adaptive model of thermal comfort–Adaptive Predicted Mean Vote (aPMV). Build. Environ. 44 (10), 2089–2096. doi:10.1016/j.buildenv.2009.02.014
Yarbrough, D. W., Wilkes, K. E., Olivier, P. A., Graves, R. S., and Vohra, A. (2005). Apparent thermal conductivity data and related information for rice hulls and crushed pecan shells. Therm. Conduct. 27, 222–230.
Zach, J., Korjenic, A., Petránek, V., Hroudová, J., and Bednar, T. (2012). Performance evaluation and research of alternative thermal insulations based on sheep wool. Energy Build. 49, 246–253. doi:10.1016/j.enbuild.2012.02.014
Keywords: thermal insulation, comfortable environment, recycled glass, straw bale, sheep wool, indoor
Citation: Ahmad M, Ali M, Turi JA, Manan A, Al-Dala’ien RNS and Rashid k (2022) Potential use of recycled materials on rooftops to improve thermal comfort in sustainable building construction projects. Front. Built Environ. 8:1014473. doi: 10.3389/fbuil.2022.1014473
Received: 08 August 2022; Accepted: 07 October 2022;
Published: 04 November 2022.
Edited by:
Hom Bahadur Rijal, Tokyo City University, JapanReviewed by:
Manoj Kumar Singh, University of Ljubljana, SloveniaCopyright © 2022 Ahmad, Ali, Turi, Manan, Al-Dala’ien and Rashid. This is an open-access article distributed under the terms of the Creative Commons Attribution License (CC BY). The use, distribution or reproduction in other forums is permitted, provided the original author(s) and the copyright owner(s) are credited and that the original publication in this journal is cited, in accordance with accepted academic practice. No use, distribution or reproduction is permitted which does not comply with these terms.
*Correspondence: Mushtaq Ahmad, bWFfNTA5OUB5YWhvby5jb20=
Disclaimer: All claims expressed in this article are solely those of the authors and do not necessarily represent those of their affiliated organizations, or those of the publisher, the editors and the reviewers. Any product that may be evaluated in this article or claim that may be made by its manufacturer is not guaranteed or endorsed by the publisher.
Research integrity at Frontiers
Learn more about the work of our research integrity team to safeguard the quality of each article we publish.