- 1Circular City + Living Systems Lab, Department of Architecture, College of Built Environments, University of Washington, Seattle, WA, United States
- 2Department of Civil & Environmental Engineering, College of Engineering, University of Washington, Seattle, WA, United States
Growing in popularity, the circular city framework is at the leading-edge of a larger and older transitional dialogue which envisions regenerative, circular, and symbiotic systems as the future of urban sustainability. The need for more research supporting the implementation of such concepts has been often noted in literature. To help address this gap, this holistic review assesses a range of pertinent sustainability frameworks as a platform to identify actionable strategies which can be leveraged to support and implement circular city goals. This assessment is grounded in a holistic overview of related frameworks across interdisciplinary and scalar domains including circular city, the food-water-energy nexus, circular economy, bioeconomy, industrial symbiosis, regenerative design, and others. Building on these interrelationships, the applied strategies espoused within these publications are synthesized and assessed in the context of circular city implementation. From an initial 250 strategies identified in literature, thirty-four general implementation strategies across six thematic areas are distinguished and discussed, finding strong overlaps in implementation strategies between frameworks, and opportunities to further develop and harness these synergies to advance circular city toward sustainable urban futures.
1 Introduction
With increasing urbanization, cities have become particularly dependent on imported flows of food, water, energy, and materials, which are brought into the urban system and consumed whilst ensuing waste streams are sent back out of the city to treatment, landfill, and as emissions and environmental contamination (Dzene et al., 2016). This linear model has allowed urban regions to develop environmental footprints which outweigh their natural bio-capacities and has weakened the resilience of cities (Doughty and Hammond, 2004; Corcelli et al., 2019). The impacts of consuming ever-dwindling raw materials at a rate outpacing nature’s ability to replenish them and reach new equilibriums “is a matter of serious global concern,” Katsou et al. (2020) assert. The intensity of human activities within cities, where socioeconomic systems and natural systems interact constantly, has considerable implications for natural environments (Meng et al., 2019). Cities vitally impact global water, energy, material, and nutrient cycles such that revising current paradigms will be essential to global sustainable development and climate action (Kennedy and Hoornweg, 2012; Lenhart et al., 2015; UN, 2020). The circular city (CC) framework has become part of a larger transitional dialogue which envisions regenerative circularity and symbiotic resource flows across scales and contexts. Implementing circular principles in cities can involve actions such as scaling up integrated networks, retrofitting existing businesses, and creating new operational practices across scales. In doing so, challenges often exist, and there is a need for new tools, innovation, and approaches to future planning (Baganz et al., 2020). Even in the case of the more established framework of circular economy (CE), the development of scientific literature and discussion of concepts is ongoing, and the design and development of practical implementation strategies remains in an emerging state (Korhonen et al., 2018; Suárez-Eiroa et al., 2019).
Current deployment of CC strategies is often guided by a range of frameworks under various terms to describe sustainable future cities (Castán-Broto and Bulkeley, 2013; Petit-Boix and Leipold, 2018; Corcelli et al., 2019). Recognizing that the CC framework is a part of a lineage of circular, symbiotic, and regenerative theory, a critical approach to CC implementation must address both its ideological relationships to existing frameworks and investigate the range of implementation strategies which emerge from them and their role in enacting and operationalizing the CC. The varied array of circular frameworks and concepts across scales as a platform of insights and strategies can be leveraged to serve and help implement CC goals through recontextualization, and do not have to be seen merely as competing or confounding ideologies. This article therefore seeks to bolster the identification of implementation strategies for the CC by synthesizing the definitions and relationships of a selection of prominent circular, regenerative, and symbiotic frameworks and analyzing the applied strategies they espouse. The review first addresses a cross section of frameworks relevant to CC implementation from an interdisciplinary range of economic, industrial, urban, and built-environment sustainability concepts. Building on these interrelationships, and review of the applied strategies espoused within the reviewed publications, six thematic areas of thirty-four circular actions and supporting implementation strategies are identified across the spectrum of circular concepts and discussed. In doing so, opportunities to further develop and harness these synergies to advance CC are uncovered. Likewise, the under-expressed value of leveraging related sustainability frameworks toward of shared goals and actualization is brought into new light in pursuit of closing loops without reinventing wheels, as momentum to sustainable urban futures through the CC framework builds.
2 Methods
To address the research aims of this investigation within the large conceptual umbrella the frameworks occupy, two phases of review were conducted, the first of the conceptual characteristics of the frameworks, and the second of the implementation strategies they expressed (Figure 1). To gather pertinent conceptual descriptions of frameworks with relevance to circularity-oriented sustainability, articles on an array of frameworks were collected and reviewed for conceptual descriptions (Table 1). The frameworks were selected for qualities they contained (circular, regenerative, and/or symbiotic) relating to aspects of CC.
This review was conducted via keyword searches of the framework names independently and paired with a selection of phrases aimed at finding implementation-oriented publications which included “applied,” “implementation,” “built environment,” “design,” “neighborhood,” “district,” “city,” and “eco-district.” The search was conducted in the university’s libraries catalogue, which consists of a selection of databases to which the libraries subscribe. Search results were filtered for peer-reviewed journal articles on these topics. The initial review pool based on the starting searches included 280 papers included based on abstract review. These were then filtered down via abstract and introduction review to a pool of 167 articles. Key internal citations within these papers were also added to the review pool at this point. From this narrowed pool, all articles were reviewed by a second close reading of the abstract and skimming of the entire paper for whether the paper offered or cited a definition of the given concept and/or whether they discussed implementation strategies for that concept, generating a pool of 84 core articles. These were included for review of framework definitions and implementation strategies and closely reviewed and coded for content pertaining to: definition of the concept/framework(s) discussed, scope of concept(s) described; scales of application inherent or described; implementation strategies presented; and references to other circular frameworks and conceptual relationships.
In the second phase of assessment, implementation strategies discussed in the core 84 papers were then reviewed and organized into a list of 250 identified implementation strategies and associated circular concepts. Through an iterative review process of grouping and consolidating similar strategies, these were sorted by content type and consolidated by qualitative alignment and equivalencies into 34 general strategies within six thematic areas. These strategies were summarized in a review table which notes the relevant citations in our review for the approaches. CC literature within the review pool was also closely reviewed, and highlighted on its own, in addition to the overview, due to the centrality of CC in our review. Furthermore, the co-occurrences of implementation strategies in the reviewed literature were used to form a Sankey diagram of the relationship between the circular concepts reviewed and the six thematic groups and thirty-four strategies. Circular concepts for which we found less than three strategies were excluded from this analysis for additional clarity and emphasis on influential and more applied concepts. This reflects an expected literature bias, as the starting pools of literature on the different topics varied greatly, with discussion of CE, for instance, published at greater magnitudes than other frameworks reviewed. This meant that even if only a subset of publications on a given framework discussed implementation on that framework, this impacted the strategy counts more significantly than for less widely published frameworks. We likewise expect that different counts and expanded results for phase two could be found if the analysis is repeated to account for passing time, or if more databases and search terms were added to the initial literature collection phase. This factor was limited to scope the initial investigation within a large conceptual umbrella while addressing the core research questions, as to assess and address conceptual relationships of the frameworks first is a necessary component of analyzing their overlapping relevance and potential for the implementation of CC.
3 Closing the Loop-Tracing and Defining Overlapping Concepts
There is general consensus that efficiently and synergistically closing resource cycles is vital to achieving sustainable urban futures (Hodson et al., 2012; Ranhagen and Groth, 2012; Lenhart et al., 2015; Dzene et al., 2016; Kujundzic and Vuckovic, 2019). Industrial ecology perspectives assert that the potential for symbiotic exchanges which are economically and environmentally advantageous is large, and that implementing circular transition requires identifying and supporting these types of synergies (Chertow, 2007). Such ideas are not entirely new, for instance, the need of innovations to create value from waste created by cities and large towns, like food by-products, was expressed as early as in 1862 (Simmonds, 1862). The larger scale perspective of planetary limits was advanced by Boulding in 1966, who highlighted the exhaustibility of natural resources on Earth (Boulding, 2017). Ensuing implications for an economy which could be shaped by labor-based loops were envisioned in 1976 by Stahel and Reday-Mulvey and the concept of CE has gained increasing dimension and attention in the following decades (Stahel and Reday-Mulvey, 1976). Today, the imperative for cities to transition to close resource loops in order to lessen global emissions and waste is becoming a cornerstone of urban sustainability discussions (Liang and Zhang, 2011; Williams, 2019). Counteracting wasteful linear models in cities through the implementation of circularity is accordingly receiving traction and attention from researchers, planners, and decision makers (Petit-Boix and Leipold, 2018; Prendeville et al., 2018; Williams, 2019; Zeller et al., 2019; Katsou et al., 2020).
Scholarship and implementation is advancing on circular approaches to urban resource consumption and production to help balance global management of finite resources. It is increasingly recognized that in order for contemporary cities to become more sustainable, they must develop more circular metabolisms through resource recycling and reuse, localized resource loops, and clean energy scenarios. Despite this, the discussion of closed-loop practices and circularity at the urban scale has not been the dominant academic dialogue (Doughty and Hammond, 2004; Corcelli et al., 2019; Williams, 2019). Williams (2019) asserts that the “circularity debate” has thus far been dominated by discussions of CE and closed-loop industrial systems by industrial ecologists and economists, producing insufficient frameworks for the complexity of the city (Williams, 2019). The answer to this gap has been the CC framework, which seeks to provide an urban-responsive approach to resource management (Williams, 2019).
The CC concept envisions the city as a regenerative and restorative system (Baganz et al., 2020). Aiming to eliminate waste and keep resources functioning at a high utility over time, CC applies many of the principles and goals of CE to foster coupling of resource flows such that by-products of one process are the input for another process (Baganz et al., 2020; Katsou et al., 2020). Prendeville et al. (2018) define the CC as one which practices CE principles, partnering with city stakeholders including community members, businesses, and researchers to close resource loops and create “a future-proof city” (Prendeville et al., 2018). The Ellen MacArthur Foundation (EMF)’s description of CC is similar, describing a city embedding CE principles across its functions to establish an intentionally regenerative, abundant, and accessible urban system (EMF, 2017). Elements of the CC include the built environment, production systems, energy systems, urban transportation systems, and urban bioeconomy (EMF, 2017). Some also note the importance of digital technology to enable the CC (Baganz et al., 2020). The definition given by the European Investment Bank includes the conservation, sharing, and reuse of resources, increasing the use and usefulness of assets, and minimizing both consumption and waste of resources (European Investment Bank, 2018). Paiho et al. (2020) suggest a useful synthesized definition: “A circular city is based on closing, slowing and narrowing the resource loops as far as possible after the potential for conservation, efficiency improvements, resource sharing, servitization and virtualization has been exhausted, with remaining needs for fresh material and energy being covered as far as possible based on local production using renewable natural resources” (Paiho et al., 2020). Through its origins and place among a shared portfolio of theoretical and applied circular, regenerative, and symbiotic frameworks which seek to foster ways of living on Earth within planetary boundaries, CC shares characteristics and goals with other concepts (Table 2). Given these relationships, CC can benefit from a self-reflective process of building on the momentum of other synergetic ideas and bringing the strategies for real-world change and implementation that they have proposed into the fold of CC as a means of strengthening and hastening vital circular transitions in urban settings. In the following seven sections, a review of the framing concepts of sustainability and relevant economic, industrial, urban, and built-environment frameworks with alignments to CC will formulate the ideological platform for a subsequent assessment of implementation strategies across multiple frameworks.
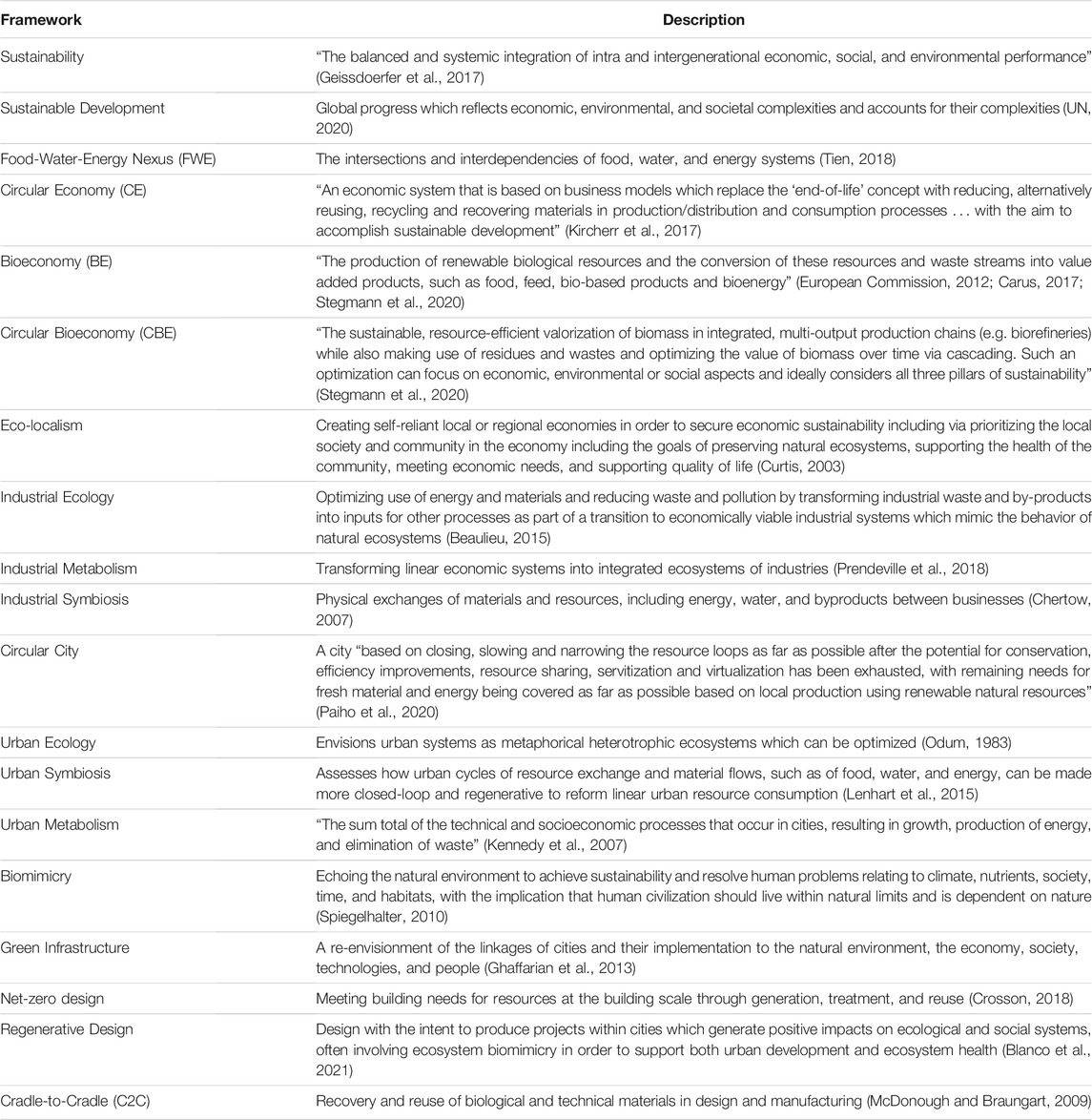
TABLE 2. Circular, regenerative, and symbiotic sustainability frameworks reviewed and prominent descriptions.
3.1 Origins in Sustainable Development
It is near impossible to discuss improving humanity’s ability to use resources regeneratively while mitigating harmful impacts without using the eminent phrase-sustainability. While its definition varies contextually, an increasingly prevalent definition is well represented by Ghisellini et al. (2016) who “define sustainability as the balanced integration of economic performance, social inclusiveness, and environmental resilience, to the benefit of current and future generations” (Ghisellini et al., 2016; Prieto-Sandoval et al., 2018). The word sustainability has origins in the French verb for “to hold up or support,” soutenir (Brown et al., 1987; Geissdoerfer et al., 2017). Our omnipresent, contemporary use of the term comes from its usage in forestry and silviculture, and the idea that the amount of wood being harvested should not outweigh the amount that can grow again (Geissdoerfer et al., 2017). This further evolved into ecological contexts as the recognition of nature’s ability to regenerate itself, and the necessity of respecting this. There are several hundred definitions of sustainability today (Geissdoerfer et al., 2017). These range from conducting human activities in a manner conserving the functioning of Earth’s ecosystems (ISO 15392, 2008), to the Brundtland Commission’s influential definition of sustainability to mean “development that meets the needs of the present without compromising the ability of future generations to meet their own needs” (Brundtland, 1987). The triple bottom line, or three pillars of sustainability, which are reflected in Ghisellini’s definition above, are a core aspect of recent interpretations of sustainability. The three pillars in this meaning are people, profit, and planet, or as a triple bottom line, sustainable economic, environmental, and social integration and performance (Elkington, 1997; Geissdoerfer et al., 2017; Rahbarianyazd, 2017). The three aspects in these interpretations “are systemically intertwined and continuously and cumulatively affect one another through mutual causality and positive feedbacks” (Mckelvey, 2002; Geissdoerfer et al., 2017). Interestingly, this description of interdependency among the three spheres, or pillars of sustainability, shares a common thread of the recognition of systems complexity and interconnectedness across scales which is echoed by CC and by many of the frameworks discussed in this review.
While sustainable development is rooted in an environmental perspective, it has been expanded to encompass an array of visions for global progress. This added complexity reflects the three pillars of sustainability and views of their interdependencies, a dynamic aptly addressed by Kates et al. who express that “the concrete challenges of sustainable development are at least as heterogeneous and complex as the diversity of human societies and natural ecosystems around the world” (Kates et al., 2005; Geissdoerfer et al., 2017). The seventeen 2030 United Nations Sustainable Development Goals (SDGs) are a leading example of the range and complexity of topics which form a global vision for a sustainable future (UN, 2020). Circularity as a sustainable development strategy is most often broached in the context of urban developments, where action to improve environmental sustainability and resource efficiency is urgently needed (Mendoza et al., 2017; Gallego-Schmid et al., 2020). Accordingly, CE has been highlighted as a means to achieve SDG 12 of responsible production and consumption, and SDG 11, which seeks to “make cities and human settlements inclusive, safe, resilient and sustainable” (Huovila et al., 2020). CE principles are consequently viewed as essential to realize sustainable development, and several of the SDG targets can contribute to the actualization of a CE (Mendoza et al., 2017; Gallego-Schmid et al., 2020; Ddiba et al., 2021). The alignments between CC and sustainable development goals and efforts provide a ripe platform for coordinated efforts and sharing of implementation strategies. They also provide a reminder of the vitality of supporting regenerative sustainability transitions in developing urban settings and the consideration of context-responsive implementation strategies for CC. Shared visions for more sustainable cities also gain valuable perspective and grounded approaches to implementation through the consideration of resource system nexuses.
3.2 Nexus Perspectives
Approaching sustainability and sustainable development across scales from global to local also necessitates nexus approaches, which recognize the interconnectedness and interdependence of multiple systems. For instance, the urban water-energy (WE) nexus is especially relevant for circular systems transitions in cities, as it describes the intersection of globally prevalent changes of urbanization, water scarcity, and energy transitions (De Stercke et al., 2020). Water and energy systems are interlinked, with bidirectional interactions, for instance, energy is required to treat and convey water to end users and water is needed in aspects of energy supply such as for cooling and hydropower (Gleick, 1994; De Stercke et al., 2020). Within cities, demand for energy and water services is concentrated, with urban settings accordingly forming a key reason for the way these systems are structured and the problems which have arisen within the nexus (De Stercke et al., 2020).
The conceptualization of this nexus can be further expanded to the food-water-energy nexus (FWE). A FWE nexus approach between the three sectors has been called for by academic and political communities in order to better understand and address synergies and impacts between food, water, and energy systems which are strongly interwoven beyond the acknowledgement shown through many current management approaches (Bazilian et al., 2011; Bizikova et al., 2013; Mukuve and Fenner, 2015; Newell, 2019). The FWE nexus is commanded largely by supply value chains and market mechanisms which are not yet capable of revealing societal and environmental risks and stands to benefit from regenerative approaches (Allan et al., 2015). As a result of the intense resource use of cities, FWE systems interactions in urban settings play a dominant role in driving global demand for all types of flows (Grimm et al., 2008; Cordell et al., 2009; McDonald et al., 2014; Kennedy et al., 2015; Ramaswami et al., 2017; Newell, 2019). Cities depend on FWE systems to function, though these resources are often produced outside of urban confines (Skar et al., 2019). Urban regions are thus particularly vulnerable in their FWE systems, especially in highly populated cities (Garcia and You, 2016; Tobosco-Chavero et al., 2018). There is a need for sustainable FWE systems solutions, including integration of food transport and waste systems as an important aspect of cities’ adaptation to climate change (Corcelli et al., 2019). Combining assessment of urban FWE nexus interactions with the implementation of more circular actions toward regenerative urban resource cycles may offer a means of advancing CC frameworks in application.
Carbon also plays an important part in urban nexuses, and the energy-water-carbon (EWC) nexus provides a valuable lens for CC transitions. Within urban economic supply chains, flows of energy, water, and carbon dioxide interact with complexity and interdependence (Meng et al., 2019). Energy and water security, as well as the need to reduce carbon dioxide emissions are core environmental issues affiliated with cities, and are receiving growing attention in research and practice (Meng et al., 2019). Emission reduction and resource efficiency are likewise a core aspect within CC perspectives. In cities, EWC systems are closely integrated with each other in product supply chains (Meng et al., 2019). For example, consumption of energy in cities contributes significantly to global CO2 emissions (Meng et al., 2019). Meng et al. argue the importance of accurately describing and revealing the mechanisms of urban metabolism in order to achieve sustainability in urban management (Meng et al., 2019). The urban EWC nexus, like many of the other frameworks, is limited in its usefulness for studies due to “unclear system boundaries of a nexus or city and imprecise urban inner structures” (Meng et al., 2019). Carbon emissions are also tied to land use patterns, which can impact one another in processes of urban transitions (Xia and Chen, 2020). Nonetheless, these two factors have been often handled as isolated from one another in the consideration of urban carbon emission mitigation (Xia and Chen, 2020). A spatial land-carbon (LC) nexus framework has been proposed for investigating the interconnections of land-use changes and carbon emissions (Xia and Chen, 2020). The carbon balance of urban systems is significantly influenced by ranging intensities of land use, scales, and impacts which shape carbon stock and emission inputs and outputs (Xia and Chen, 2020). Magnitudes and scales of resource flows, their interactions and interconnections, and contexts as assessed through nexus perspectives including those of WE, FWE, EWC, and LC, are all deeply relevant to CC implementation. Assessment and coordination of larger systemic nexus frameworks can help navigate the invariable systems complexity at the urban scale which shapes potential routes for successful circular transitions.
3.3 Circular Economy
The framing of resource flows through an economic lens is also a core perspective which can bolster CC advancement. Arguably the most closely tied to the development of CC, CE is a concept for a rebalanced production and consumption cycle which aims to decouple economic growth from the degradation of the environment and depletion of natural resources (Jackson, 2009; Beaulieu, 2015; Williams, 2019). Prendeville et al. describe that while it has gained recent recognition, the concept of CE developed gradually and was influenced by seminal thinkers in ecology, environmental economics, and systems thinking to describe how resource flows in economies can become closed-loop at different scales (Allenby and Graedel, 1993; Chertow, 2000; Prendeville et al., 2018). Early work in CE was discussed also as “closed-loop economy,” and was described by Boulding as the circular, closed-loops of the Earth and its systems and the resulting inference that the economy should exist in harmony within the boundaries of Earth’s natural systems and capacity (Boulding, 2017). Rachel Carson’s Silent Spring and Meadows et al.,‘s 1970s “Limits to Growth” have been suggested as having likely also played a role in shaping an early CE perspective (Carson, 1962; Meadows et al., 1972; Winans et al., 2017). CE was further developed by Stahel and Reday-Mulvey in the mid-70s, who described a looping economy with aspects of industrial scale waste prevention, regional labor economies, and resource efficiency (Stahel and Reday-Mulvey, 1976; Geissdoerfer et al., 2017). Conceptual frameworks were further developed by Pearce and Turner in 1990 who described the influence of natural resources on the economy and the open-endedness of contemporary linear economic systems (Pearce and Turner, 1990; Geissdoerfer et al., 2017; Winans et al., 2017; Williams, 2019). They are sometimes attributed with the introduction of CE, such as by (Andersen, 2007; Su et al., 2013; Ghisellini et al., 2016; Geissdoerfer et al., 2017). Stahel and Reday-Mulvey’s (1976) work influenced economic policies in Japan and Germany in the 1980s and 90s, which in turn fostered the integration of CE concepts in industry (Moriguchi, 2007; Winkler and Bilitewski, 2007; Williams, 2019).
The recent growth of popularity of CE has been attributed by some to the last decade of efforts toward international sustainable development (Moriguchi, 2007). Whereas industrial processes in a linear economy are oriented around one-directional material flow in which raw materials are used to make a product and waste, and the product when its use is over, disposed of, CE features waste valorization and resource recovery which keep materials in the supply chain in order to decouple the growth of the economy and pressures on the natural environment (Ghisellini et al., 2016; Elia et al., 2017). Three principle aims of the CE approach include reducing consumption of resources and production of waste, preserving natural resources and ecosystem services, and designing out negative environmental, social, and economic externalities (Williams, 2019). Likewise, the CE approach is closely tied to the 3Rs-reduce, reuse, and recycle (Ginelli et al., 2020a; Ginelli et al., 2020b). Some also consider a fourth “R” for recovery, to represent recovering materials and energy from waste (Kirchherr et al., 2017; Joensuu et al., 2020). The Ellen MacArthur Foundation (EMF) is a prominent force in the popularization of CE, describing a vision for an economy which is by design, regenerative and restorative, and that keeps materials, products, and components at a high value and usability over time (EMF, 2017; Hart et al., 2019). The EMF’s work has been influenced by a variety of other frameworks. Blue Economy, for instance, proposes an economic system wherein multiple monetary flows close a waste to value loop as an alternative to linear value creating systems that deplete natural resources (Pauli, 2010; Prendeville et al., 2018).
Peters et al. suggest that there are four key components to the definition of CE: The first is recirculating energy and resources, minimizing demand for resources, and valorising waste streams; the second is using a multilevel approach; the third is the importance of CE as a means to realize sustainable development; and the fourth is the importance of societal processes of innovation (Peters et al., 2007; Prieto-Sandoval et al., 2018). Karhu and Linkola expand upon this to describe CE in a three-aspect manner akin to a triple bottom line approach, which in addition to the creation of economic and environmental value, includes fostering social value such as preventing unhealthy and unethical labor conditions (Karhu and Linkola, 2019). Given that there is a certain ambiguity to the scale of economy in CE, interventions have been discussed to include three levels, micro (single companies, customers, products), meso (eco-industrial parks), and macro (cities, regions, and nations) (Ghisellini et al., 2016; Elia et al., 2017; Kirchherr et al., 2017; Prieto-Sandoval et al., 2018; Baganz et al., 2020). Geissdoerfer et al. describe the aim of these multiple levels of operations as accomplishing sustainable development by “creating environmental quality, economic prosperity and social equity, to the benefit of current and future generations” (Geissdoerfer et al., 2017; Prieto-Sandoval et al., 2018; Thiriet et al., 2020). CE is thus intended to produce a regenerative system where emissions, resource input and waste, and energy inefficiencies are minimized via the reduction, integration, and refinement of material and energy loops (Bocken, 2016; Prieto-Sandoval et al., 2018; Paiho et al., 2020).
The application of CE to urban environments through urban mining approaches and the consideration of systems of anthropogenic urban resource management of food, water, energy, and waste is discussed often (Prendeville et al., 2018). Some have pointed out that there are challenges and limits to the environmental sustainability of CE, particularly in defining system boundaries of CE and managing flows between different actors (Korhonen et al., 2018; Baganz et al., 2020). A notable organizing principle of CE applications is based on material flow type, dividing CE into technical and biological cycles (Mendoza et al., 2017; Gallego-Schmidt et al., 2020). The technical cycle is made up of inorganic material flows, meaning that they are non-biodegradable such as metals, traditional plastics, and minerals (Joensuu et al., 2020). The CE biological cycle is made up of organic materials, which are biodegradable and contain biological nutrients which can re-enter into the biosphere (Kirchherr et al., 2017; Joensuu et al., 2020). The consideration of both technical and biological flows is essential to CC implementation, as inorganic and organic resources flow through cities at high magnitudes, and the way that they are managed reflects evolving concepts and applications of urbanisms, which must shift towards increasing circularity and urban scaled regenerative practices to actualize CC transitions.
3.4 Biological and Ecosystem Driven Circularity
Biological cycles and resource recovery from organic waste streams has also been the focus of considerable research within the frameworks of bioeconomy (BE), circular bioeconomy (CBE), and urban bioeconomy (Ddiba et al., 2021). BE is less immediately concerned with circularity, though due to the inherent regenerative cycles of organic flows, the connection is still significant. Historical BE reaches early into human history when the population lived primarily off of the land until the mid-1700s when the industrial revolution shaped changing paradigms (James, 2018). Population growth and industrial development make a return to regenerative BE a greater challenge than in the past, however, a transition is needed in the face of spiraling resource depletion and global climate change (James, 2018). Early formulations of BE are sometimes credited to Georgescu-Roegen’s (1975) bioeconomics work advocating for a biophysical way of looking at the economy (Georgescu-Roegen, 1975; D’amato et al., 2017). More recently, BE was defined by the EU as “the production of renewable biological resources and the conversion of these resources and waste streams into value added products, such as food, feed, bio-based products and bioenergy” (European Commission, 2012; Carus, 2017; Stegmann et al., 2020). Most conceptualizations of BE are based on the perspective that inputs for industrial activities, such as energy, material, and chemicals, should come from renewable biological resources, with necessary transformational processes supported by research and innovation (Bugge, 2016; Kleinschmit et al., 2014; Pfau et al., 2014; D’amato et al., 2017). Having bio-based alternatives to non-renewable sources is a key aspect of BE, and thus industries like agriculture and forestry are vital to help fill this niche (Roos and Stendahl, 2015; D’amato et al., 2017). In this vein, Carraresi et al. (2018) describe the cross-industry nature of BE, in which a range of sectors, production systems, and technologies help shape renewable resource streams and by-product reuse. Cities can also foster unique opportunities for BE, and urban bioeconomy has been described “as a form of environmental value creation,” a valuable perspective for inclusion in CC (Buck, 2017).
Bio-based industries and manufacturing already fit well in CE when they use organic residues and waste flows as biorefinery feedstocks, and given the circular cycles and metabolic pathways inherent in biology, however while the BE practices can be circular, they are not automatically so (James, 2018). Accordingly, the integration of CE principles in BE has been called for, including the assertion that this will be necessary for true resource efficiency from BE (D’amato et al., 2017). The intersection of BE and CE forms the concept of CBE (James, 2018). Stegmann et al. (2020) discuss a route for CBE optimization which is possible through cascading uses of biomass, meaning using resources sequentially for different purposes. They likewise define CBE as focusing on “the sustainable, resource-efficient valorization of biomass in integrated, multi-output production chains (e.g., biorefineries) while also making use of residues and wastes and optimizing the value of biomass over time” (Stegmann et al., 2020). Focus of optimization measures can be tailored to specific environmental, economic, or societal factors but in ideal scenarios should consider all of the three facets of sustainability (Stegmann et al., 2020).
Local scale circularity in the economy reoccurs in several sustainability frameworks, and has been described in particular focus within the context of eco-localism. Eco-localism is centered around the idea of creating self-reliant local or regional economies in order to secure economic sustainability (Curtis, 2003). This involves prioritizing the local society and community in the economy by preserving natural ecosystems, supporting the health of the community, meeting economic needs, and supporting quality of life (Curtis, 2003). Environmental geographies (e.g., bioregions) are often used to describe the natural limits and boundaries of eco-local economies (Sale, 1985; Curtis, 2003). The view of eco-localism is that ecological limits should shape the size of the economy, whose scale is vital to sustainability, and asserts that as ecosystems are heterogenous and symbiotic local capital unique, opportunities to replace natural capital with other types are limited (Curtis, 2003). Eco-local capital is made up of five forms of local scale capital-physical, financial, natural, social, and human, which interact symbiotically and reinforce one another (Curtis, 2003; Williams, 2019). For instance, local natural capital is reinforced by local social capital that guides preservation and restoration of ecosystems (Williams, 2005; Williams, 2019). Social capital also helps to make local resource sharing scenarios and loops more feasible and bolsters the outcomes of investing in human capital and physical infrastructure (Putnam, 1993; Williams, 2019). Physical capital, such as infrastructure and the built environment, can also help facilitate resource sharing and economic circularity in local economies; this is enhanced by physical proximity of those producing and utilizing resource flows such as utilities and industries (Williams, 2019). Several elements of eco-localism are particularly relevant for CC, especially as the idea of closing loops at the city scale anticipates some level of localization of resource flows and economic systems, within which the concept of local scale capital types becomes especially useful for development and assessment of implementation approaches for urban circularity.
3.5 Symbiosis and Circularity in Industrial Systems
One of the foundational precursors of CC, industrial ecology (IE) is a field of research and application focusing on creating and maintaining a closed-loop industrial ecosystem. As part of an envisioned transition to economically viable industrial systems which mimic the behavior of natural ecosystems, its aims include optimizing use of energy and materials and reducing waste and pollution by transforming industrial waste and by-products into inputs for other processes (Beaulieu, 2015). The perspective of IE frames industrial systems as part of a complex interrelated ecosystem-a view derived from the central concept that modeling human systems after natural systems is a path to greater sustainability (Spiegelhalter, 2010). A core aim in an urban context, therefore, is to holistically integrate technologies and processes which use energy and materials efficiently and with minimal greenhouse gas emissions (Spiegelhalter, 2010). A concept closely related to IE is industrial metabolism, which was described by Frosch and Gallopoulos (1989) as the concept of the transformation of a “linear economic system into an integrated industrial ecosystem” (Prendeville et al., 2018). Frosch and Gallapoulos’ influential article in Scientific American described a vision for industrial ecosystems wherein “the consumption of energy and materials is optimized and the effluents of one process…serve as the raw material for another process” (Frosch and Gallopoulos, 1989; Chertow, 2007). Industrial symbiosis (IS) is essential to this vision and requires close attention to material and energy flows through economies at a local and regional scale (Chertow, 2000).
IS is the concept of the physical exchanges of materials and resources, including energy, water, and byproducts between businesses (Chertow, 2007). This can involve the engagement of and interaction between industries that have been traditionally separate in order to arrive at an integrated, collective strategy to exchange resource flows and achieve a competitive advantage and resource efficiency (Chertow, 2000). Three main types of resource exchange are described by Chertow: by-product reuse, utility/infrastructure sharing, and joint provision of services (Chertow, 2007). By-product reuse is essentially exchange of industrial material flows between two or more businesses in which the excess material from one operation is used in the other in replacement of raw materials or commercial products (Chertow, 2007). Utility/infrastructure sharing is the shared organization and utilization of core resources like water, energy, and wastewater (Chertow, 2007). Similarly, joint provision of services is coordination such as shared arrangements of transportation, food supply, and other common needs across neighboring industries and businesses (Chertow, 2007). Types of physical manifestations of industrial symbiosis have been described as industrial ecosystems, eco-industrial networks, and eco-industrial parks. Eco-industrial park initiatives form around a close locational proximity between coordinating firms to exchange material and informational resources to reduce waste, curtail and optimize use of raw materials and energy, and foster multidimensional sustainable relationships between businesses and key actors (Winans et al., 2017). The same concept extends to industrial symbiosis networks and eco-industrial networks where it is instead applied to a larger geographic region, even up to the scale of a state/province or country (Winans et al., 2017). Circularity in industrial systems is a necessary and influential piece of the transition to circularity in economies and cities, and can be integrated into other scalar perspectives and systems, even helping to provide examples and methods for CC via the existing lineage of industrial ecology thinking and development. Strides forward in CC transitions are increasingly seen taking effect in industrial economic sectors, with governance and programs promoting IS serving to also directly further key aspects of CC itself. Connecting and translating successful IE driven approaches to the full urban scale can leverage symbiotic industrial frameworks and be of great benefit, however also require further development to address and respond to the vast integrated systems of cities which among other differences include vital socio-political forces and actors.
3.6 Conceptualizing Urban Circularity
As discussed within CC perspectives, cities contain exceptionally complex interactions of resources flows, urban dwellers, and urban space. While many resources used in cities originate from nature, the reality still remains that within current modes most are utilized and then disposed of in a linear fashion (Skar et al., 2019). Circular approaches to urban resource use explore options for regenerative management of these flows through reduction, reuse, and recycling (Skar et al., 2019). This fosters the new formulations of the design and interrelationships of urban, peri-urban, and rural spaces, leading to closed-loop approaches such as localized food systems that take into account broader systems perspectives such as that of an urban/peri-urban FWE nexus (Skar et al., 2019). Unsurprisingly, the CC concept shares modes of thought with several other urban scale metabolic conceptualizations of sustainability. Many of these metabolic, circular conceptions of the city take on aspects of biomimicry. Biomimicry (bios-life, mimesis-to imitate) seeks to echo the natural environment to achieve sustainability and resolve human problems relating to climate, nutrients, society, time, and habitats, with the implication that human civilization should live within natural limits and is dependent on nature (Spiegelhalter, 2010; Prendeville et al., 2018). These limits are perceived through the mediating systems of technology, culture, and socio-economics (Spiegelhalter, 2010). Drawing on knowledge transfer and emulation of living organisms and ecosystems, biomimicry aims to foster greater sustainability (Blanco et al., 2021). Though biomimicry tends to imply the interpretation of nature-based ideas into physical designs, it can also prove a useful concept for other human systems like economies and cities (Pomponi and Moncaster, 2017). Spiegelhalter (2010) express that “it is almost an imperative attempt to biomimic natural processes towards a circular metabolism where city outputs seem to become resources for new inputs”. This can facilitate a strong conceptual starting point for urban environments; however, it has been argued that when biological analogies are applied to entire cities and regions, they need to be paired with other approaches and can otherwise lose usefulness and utility as an analytical lens for city functions (Gandy, 2004). Urban symbiosis, for instance, refashions the concepts of IS into the dimensions of a city (Lenhart et al., 2015). Urban symbiosis assesses how urban cycles of resource exchange and material flows, such as of food, water, and energy, can be made more closed-loop and regenerative to reform linear urban resource consumption (Lenhart et al., 2015). Consideration of governing and decision makers’ roles in shaping these dynamics is considered an important element of the framework, aligning strongly with CC (Lenhart et al., 2015).
Notably, the interconnected flows of modern cities are somewhat frequently referred to as a metabolism of integrated resource flows through urban space that rely on external energy, material, and information inputs (Gandy, 2004). Urban Metabolism (UM) accordingly describes cities as ecosystems under development which need resources and have metabolic flows of materials, nutrients and food, water, and energy (Kennedy et al., 2007; Gagliano et al., 2015; Paiho et al., 2020). Kennedy et al. define UM as “the sum total of the technical and socioeconomic processes that occur in cities, resulting in growth, production of energy, and elimination of waste” (Kennedy et al., 2007). In addition to quantification studies, UM can include assessment of application of circular metabolism in cities and application of biomimetic urban design strategies (Spiegelhalter and Arch, 2010; Buck, 2017; Prendeville et al., 2018). UM was developed in the context of environmental deterioration of water and air in the 1960s US and introduced the quantification of city resource fluxes (Wolman, 1965). Precursors and closely aligned conceptual antecedents to Wolman’s UM arguably emerged much earlier, particularly within the 1800s. For example, scientific developments in this period fostered a public health movement which promoted the hygienist city (Gandy, 2004). With the advancement of biological and physical sciences, sanitation systems and infrastructure were developed to control and separate resource flows in the city with the aims of better protecting human health. Along with these developments, recognizable linear flows in modern cities became more established and thinking around urban conditions evolved, for example wastewater flows were viewed as a danger to be removed from urban cycles and sent out of cities, and their potential value as a nutrient source largely dismissed. UM can be a useful analogy and framework for analyzing the relationships between biophysical processes and societies, and it has been advocated that viewing it distinctly from its precursors is an important caveat to harnessing its usefulness (Gandy, 2004). Gandy describes that current day “urban metabolism can illuminate the circulatory processes that underpin the transformation of nature into essential commodities such as food, energy and potable water” and provide insights into “commodity chains, the particularities of local context and the fluidity of urban form” (Gandy, 2004). With its core qualities of conceptualizing resource flow dynamics and their assessment, UM fundamentally underpins CC perspectives and that of many related circular and sustainability frameworks including aspects of CE and urban symbiosis, further manifesting within the means of circular transitions that they espouse.
Urban Ecology likewise envisions urban systems as metaphorical heterotrophic ecosystems which can be optimized (Odum, 1983). Akin to complex living systems, cities metabolize resources and contain producers and consumers who interact to characterize resource flows and loops (Wolman, 1965; Kennedy et al., 2007; Williams, 2019). The urban ecosystem can be kept healthy through looping, or cycling, waste resources at various scales, which is further supplemented by the localization of resource cycles and the protection of natural ecosystem services (Orr, 1992; Williams, 2019). These actions align strongly with CC goals. McDonnell et al. describe such an urban ecosystem as containing distinct and integrated systems of the natural environment, the socio-economic environment, and the built environment (McDonnell et al., 2009).
3.7 Regenerative Built Environment Concepts
The built environment will play a key role in the implementation of CC concepts. Indeed, some argue that the built environment is the sector that generates the most pressure on the natural environment (Pomponi and Moncaster, 2017). Pomponi and Moncaster critique CE’s lack of consideration of the built environment, describing that “in framing building research from a… [circular] perspective there is a lack of focus on buildings, with most research designed either around cities and neighbourhoods or construction materials” (Pomponi and Moncaster, 2017). According to the literature, assert Joensuu et al. (2020), the built environment should be understood to refer not only to buildings, infrastructure, and their construction, but also include their operation, maintenance, and use, which forms varying environmental impacts over a full life cycle. Several concepts in the built environment seek to facilitate greater circularity and regeneration in construction and operation of infrastructure and buildings.
At the infrastructural scale, Green Infrastructure has been described as a reenvisionment of the linkages of cities and their implementation to the natural environment, the economy, society, technologies, and people (Ghaffarian et al., 2013). Likewise, net-zero design, which can focus on specific resources at building or district scales, often features decentralized infrastructural approaches rather than conventional centralized infrastructure. Net zero water (NZW) is the concept of water independence, which can be achieved at building scales or district scale. To be net zero for water means that all of a building or district’s water needs are met via localized capture, closed-loop systems, and reuse, including full management of storm water such that any discharged water is managed to benefit local ecology (Crosson, 2018). Net-zero energy (NZE) is similar, wherein site-based energy production and consumption sum to zero or even negatively such that production outweighs consumption. Some difference exists, however, as NZE can still include grid integration with centralized energy grids, while NZW exists independently from larger municipal systems (Crosson, 2018). Net-zero design relates strongly to the CC due to shared goals of closing resource loops, albeit at distinct, though potentially overlapping scales.
Buildings functioning with net-zero principles are sometimes referred to as “circular buildings.” Pomponi and Moncaster define the term as referring to “a building that is designed, planned, built, operated, maintained, and deconstructed in a manner consistent with CE principles” (Pomponi and Moncaster, 2017). The term living building has also been used to refer to highly sustainable, self-sufficient buildings, particularly in the context of the Living Building Challenge (ILFI, 2021). In this context, buildings and sites are also increasingly viewed as having the potential to be regenerative. Regenerative design aims to produce projects within cities which generate positive impacts on ecological and social systems, often involving ecosystem biomimicry in order to support both urban development and ecosystem health (Blanco et al., 2021). The regenerative design concept was proposed by John Tillman Lyle who described the possibility of building human systems and places “with a circular logic by reincorporating the essential elements of life, such as energy conversion, water treatment, and nutrient cycling in human designed urban spaces” (Lyle, 1994). Contemporary regenerative design practices espouse understanding urban systems through holistic and ecological lenses in order to achieve mutual benefit between ecological and social systems (Reed, 2007; Blanco et al., 2021).
Within the built environment, life cycle perspectives are seeing increasing uptake and usage. Consideration of the life cycle of materials from production and construction to disassembly further foster contemplation of circularity and opportunities for reuse. Braungart and McDonough’s cradle-to-cradle (C2C) concept is an example of this perspective and promotes recovery and reuse of biological and technical materials (McDonough and Braungart, 2009). C2C has overlapping concepts with CE, but particular emphasis on reuse and recycling and further centers design as a means of closing material cycle loops (McDonough and Braungart, 2009; Joensuu et al., 2020). As the built environment disciplines strive for greater sustainability in design and practice, key opportunities to synergize efforts taking place under multiple certification systems and frameworks (e.g., C2C, LEED, Living Building Challenge, etc.) and influential concepts such as regenerative and net-zero design may exist as a means of furthering CC design and implementation and merit further consideration.
4 Implementing Circularity
Implementing CC concepts will require a multifaceted portfolio of strategies, many of which can be adopted from related frameworks. A range of approaches that bridge from theory to actualization have been suggested in literature. The similarities and variations of many of the frameworks discussed in section three are reflected in the strategies for implementing them. Within our selected literature pool, 250 individual strategies were identified which could be grouped into 34 general strategies within six thematic areas: Resource and Material Management, Systems Development and Integration, Bio-based Solutions, Governance and Stakeholder Engagement, Data, Measurement, and Modeling, and Research and Education (Figure 2). These implementation strategies vary from direct actions to supporting and regulatory approaches. The first three thematic areas include direct actions to advance circularity in practice. Resource and Material Management describes actions that involve the creation, transformation, and movement of urban resource flows such as through recycling, reuse, and construction. Systems Development and Integration strategies consist of actions to integrate systems of various types and scales and to develop new modes of operation, adapting and transitioning linear systems into regenerative ones. Bio-based Solutions are strategies fundamentally tied to living systems such as organic resource flows and processes, nature-based aspects of CC, and strategies involving the food system. The other three thematic areas of strategies which emerged through the review are supporting strategies, which help to advance, strategize, and enact CC goals. While less direct, these thematic areas are absolutely critical to CC implementation. For instance, Governance and Stakeholder Engagement strategies leverage policy and collaboration to foster CC transitions. Likewise, Data, Measurement, and Modeling strategies are essential to closing loops, supporting effective design and decision making, as is Research and Education, the sixth thematic area of strategies.
A noteworthy variation across the 34 strategies is the degree to which they rely on organic alliances and developments versus top-down approaches to circularity. This varies even within specific strategies such as in implementation strategy five, Industrial Developments. Figure 2 summarizes the strategies, with definitions and examples derived from the literature review. A literature review of implementation strategies documented in CC publications can be found in Figure 3.
There is significant overlap between strategies advocated under different frameworks particularly between CE, which included 33 of the 34 strategies, and CC, for which we identified 29 (Figure 4). Three strategies in particular saw strong occurrence in CC publications, 7. Infrastructure developments and Infrasystems integration, 8. Circular business models, and 22. Developing and Leveraging datasets. The other frameworks which included the most implementation strategies in literature include the FWE nexus, BE, IE, IS, CBE, and net-zero design (Figure 4). To rule out a bias of interpretation, we only attributed strategies to concepts for which journal articles specifically mentioned the implementation strategies in question. Despite strong theoretical overlaps between all concepts, a heavier emphasis on application is observed in CC, CE, and FWE-Nexus publications, which included more strategies for implementation, pointing toward a tension and area of opportunity in the space between theoretical and applied circular sustainability.
4.1 Resource and Material Management
Managing resources and materials regeneratively is one of the most core functions of a CC. Circular actions of looping and adapting and supporting actions of localization and optimization are key to these implementation strategies (Williams, 2019; Figure 2). The first implementation strategy Reduction, Reuse, and Recycling of materials is by far one of the most central aspects of achieving circularity through CC, CE, and IE. Elia et al. note a growing interest in waste management, including reducing waste and increasing efficiency and sustainability of resources, which has progressed in US policy although CE and CC policy lags in the US compared to European efforts (Elia et al., 2017). Logistical Improvements comprise an essential aspect of a transition to greater circularity by shaping how flows and exchanges can occur in a more closed-loop manner. Strategy 2, localization, is also a core element across the frameworks, occurring in six of the eleven included in Figure 4. Of the frameworks reviewed, eco-localism perhaps most directly centers around the concept of localization, calling for decentralized sustainable technologies adapted to local environmental, cultural, community, and economic conditions (Curtis, 2003).
Green Construction, which forms an entire field of its own, is also a key strategy to implement urban circularity due to the fundamental role of the built environment in the city and in shaping how resources are used. There are opportunities to better integrate CC concepts into green construction. Munaro et al. (2020) note for instance, that attention to CE in the built environment, has been growing in both theory and practice. They describe the need for more research on component reuse, the development of the secondary materials market, and more research and development of operating circular value models in the built environment (Munaro et al., 2020). They assert that “this is a critical gap because the role of managing and applying circular innovation in the built environment is often neglected” and describe that the implementation of circularity is held back by insufficient knowledge about the definitions of CE and similar frameworks, and about how to implement these within business models (Adams et al., 2017; Munaro et al., 2020). Karhu and Linkola (2019) also have discussed that approaches to implementing circularity in the built environment form an active topic of discussion and that understanding is under development as applied experiences grow. Gallego-Schmid et al. conducted a review which found that publication of research on CE in construction had not yet found a “home” and was published across a variety of journals and in conference proceedings, indicating “a new, growing area, with researchers first testing their ideas in a conference setting before publishing them as journal articles” (Gallego-Schmid et al., 2020). Building level considerations of CC, or CE, can view buildings as “material banks,” though building level circularity can also reflect further principles which bridge across scales (Cheshire, 2016; Geldermans, 2016; Giorgi et al., 2020). Giorgi et al. describe three areas of principles relevant to the building scale “design process aimed at adaptability and reversibility; resource/waste management aimed at reuse and recycling; [and] business models aimed at extending life and value of products while also changing the concept of ownership” (Giorgi et al., 2020). They express that waste prevention needs to be the first goal to use resources efficiently and effectively, and that this can be achieved through a combination of strategies including extending building life, greater product durability, and repairing, reusing, and maintaining resources, such as in implementation strategy one- Reduction, Reuse, and Recycling of materials (Giorgi et al., 2020). Green construction and building scale circularity intersect with several thematic areas and implementation strategies which emerged through our review. These include Systems Development and Integration, implementation strategy six Building Integration, and strategy eight Circular Business models, thematic area three Bio-based solutions, and thematic area five Data, Measurement, and Modeling, wherein many key supporting strategies and methodologies, such as LCA, increasingly used in green construction contexts, occur.
4.2 Systems Development and Integration
The second thematic area which emerged is that of Systems Development and Integration, which is particularly relevant to the urban scale and context of CC. As sustainable urban developments proliferate globally, they will need to harness systems integration strategies, note Pandis-Iveroth et al. Systems integration approaches may include the generation of energy and transport fuels from domestic and organic waste streams, treatment of grey water for irrigation or other secondary uses, fertilization of agricultural areas with treated sludge or various forms of recovered nutrients, and many more strategies, some of which we will discuss further below (Pandis-Iveroth et al., 2013). Integrated solutions advance circular urban metabolisms over linear processes, manifesting endeavors to close material flow and energy cycles in cities such that they take on ecosystem qualities (Pandis-Iveroth et al., 2013). A number of common strategies were advocated for by literature for CC and CE -which both mentioned all strategies in this thematic area-as well as by Industrial Symbiosis, the FWE-Nexus, and Regenerative Design (Figure 4). BE literature called specifically for the implementation and development of strategy eight Circular Business Models, although the principles of BE align well with the other implementation strategies in this thematic area. The strategies identified within this thematic area include strategy five Industrial Developments, strategy six Building Integration, strategy seven Infrastructure developments and infrasystems integration, strategy eight Circular Business Models, and strategy nine Integrative and Eco-Design.
The development of systems integration through industrial developments has been discussed for some time in the contexts of IS, IE, and CE, and has been more recently discussed with regard to CC and regenerative design. While industrial symbiosis and developments of integrated industrial networks are a key component of realizing the CC, it will be important to look to the previous observations of barriers and failures of industrial symbiotic partnerships which have been noted in industrial ecology and symbiosis literature so as to find successful supporting strategies and avoid repeating shortcomings in past implementation attempts (Chertow, 2007). Drivers of circular industrial developments range from social, environmental, and regulatory in nature, and manifest differently within different geographies and cultures (Chertow, 2007). Whilst there are many quantifiable benefits, Chertow argues the importance of asking why more cases of successful industrial symbiosis are not yet seen (Chertow, 2007). Gibbs et al. observed that difficulties in planning successful industrial ecosystems have been long noted by researchers and policymakers, leading to them to conclude that “initiatives based upon the interchange of wastes and cascading of energy are few in number and difficult to organize” (Gibbs et al., 2005; Chertow, 2007). Though this has begun to change in more recent years, contemporary efforts can benefit from considering past research of historical implementation failures. Literature identification and discussion of barriers to industrial symbiosis has noted these include typical business development challenges and other issues rooted in the operational, behavioural, and financial difficulties of working across organizations (Lowe et al., 1996; Chertow, 2000; Chertow, 2007).
Building Integration, strategy six, in some instances directly overlaps with symbiotic industrial development, though can also pertain to individual closed-loop systems at the building scale. This implementation strategy refers to the practice of integrating resource flow management and circularity at the building scale, which can occur through various means including building integrated water management, building scale energy production, integrated sustainable technologies or food production, and redistributing surpluses between buildings (Figure 2). The role of further research, which will reoccur in the context of thematic area six, is especially relevant for the building level as there is currently a lack of research addressing the link between micro and macro levels of circularity which intersect at the building scale. Giorgi et al. describe “a link between…two levels [wherein] circular requirements (e.g., exchange and use of reused/recycled materials) at building level can activate circular practices on an urban level and with regards to materials’ composition” (Giorgi et al., 2020). Similar intersections exist between buildings and the FWE-Nexus. Tien (2018) describes the inextricable linkage of the two, especially in the case of water and energy systems, for which buildings often act as the end distribution points for municipal systems (Tien, 2018). Another form of building integration discussed by Tien is building integration of agriculture, which was mentioned in several articles of our literature review pool and is a means of FWE-Nexus implementation as well as of CE and CC. Building-integrated agriculture intersects with strategy eleven discussed below, Controlled Environment Agriculture. Agricultural systems and greenhouse structures can be integrated with buildings to produce benefits including synergetic use of heat and utilization of harvested rainwater (Delor, 2011; Proksch, 2016; Tien, 2018). Building resource flows and systems can also be integrated between buildings and in synergetic partnerships with productive and industrial processes, such as the building integration of aquaponics, and the building integration of aquaponics with a brewery (Proksch et al., 2019; Horn and Proksch, 2020a).
Strategy seven Infrastructure developments and infrasystems integration is an important means of resource and material management, particularly as a way of developing effective symbiotic urban systems for circular cities. Environmental benefits of infrasystem synergies include decreased energy and material usage and increased spatial concentration and use of secondary resources (Jonsson, 2000). Joensuu et al. (2020) note that urban symbiosis of infrasystems should apply cutting edge carbon neutral technological approaches to achieve greater benefit. Infrastructure developments and infrasystems integration and can also leverage frameworks such as C2C and the 3Rs, which align with implementation strategy one, Reduction, Reuse, and Recycling of materials, as well as industrial ecology approaches and integrated waste management (Kollikkathara et al., 2009; Joensuu et al., 2020). Joensuu et al. note that while an array of strategies to infrastructural integration can become a point of confusion to decision makers, approaches such as waste hierarchy can help to “prioritize the prevention of waste generation and to minimize processing which may provide costs savings opportunities” to material extraction and waste processing (Ribic et al., 2017; Joensuu et al., 2020). Decision making frameworks such as these can also be viewed as an implementation strategy themselves, a theme in literature which is synthesized via the supporting implementation strategy of Decision Making (strategy 19).
Considering infrasystems integration requires reflection on the relationships of urban and rural systems and resource exchanges, the dynamics of which need to be assessed and unraveled to facilitate efficient recovery of nutrients, energy, and materials in urban biotic cycles (Joensuu et al., 2020). Likewise, setting up effective working definitions and systems boundaries in applied contexts is a necessary step given the variability and overlap in conceptualizations of circularity. Continually asking what circular solutions make sense where and at what scales they are most effective and efficient should become a recurring practice in both research and professional fields. At what scale should loops be closed? Net-zero buildings and building level circularity is such a scenario where this type of critical reflection can help achieve more efficient circular cities. In urban cases, it is reasonable to apply a critical lens to assess in which cases closing loops at the building scale is more sustainable than integrated grid-level solutions. However, many of the interests, goals, and quantitative approaches being used within the built environment’s sustainability efforts are in clear alignment with circular goals, and if cutting edge approaches to circularity can be better integrated with conceptualizations of urban level system boundaries much stands to be gained. It is likely that a range of levels of integration with urban grids may form the most flexible and resilient scenario. More research is also needed on the connections of urban, peri-urban, and rural systems and what CC’s impact on their interconnections may be. Opportunities can be explored to leverage historical relationships and potentials for synergy while reforming linear paradigms.
4.3 Bio-Based Solutions
Within thematic area three, Bio-Based Solutions, four general implementation strategies were identified- Supporting sustainable local food production, Controlled Environment Agriculture, Nature Based Solutions, and Valorization of organic waste streams. All can contain overlapping features, though are also distinct and received specific mentions as implementation strategies in the reviewed literature. Skar et al. (2019) describe that localizing urban food systems and narrowing of cities’ foodsheds is vital to developing more healthy and sustainable cities. They express that this aligns “perfectly” with the CC concept, as organic waste flows can be processed to support other agricultural products, and that a more circular approach to food via systematic approaches is needed to combat contemporary linear models (Skar et al., 2019). Urban agriculture can be seen as a driving force behind new urban approaches to resource cycling, and can particularly materialize CC principles via integrated solutions such as meeting water requirements from sources originating within the city’s watershed rather than utilizing tap water (Fletcher et al., 2013; Tahir et al., 2018; Pratt et al., 2019; Skar et al., 2019). In addition to soil-based operations, a promising means of local food production in urban areas is found in Controlled Environment Agriculture (CEA), implementation strategy eleven. A field which has received increasing research interest and popularity in application, CEA consists of approaches to growing food wherein environmental conditions like light, water, temperature, and nutrients, are controlled, often through uses of technology like sensors, monitoring systems, and LEDs, to achieve highly efficient food production that is resilient to external environmental conditions. Several such growing typologies may sound familiar to many due to their growing popularity and media coverage and include aquaponics, hydroponics, vertical growing (often hydroponic), and building integrated agriculture such as integrated rooftop greenhouses (Ceron-Palma et al., 2012; Gehrke, 2014; Pons et al., 2015; Santos, 2016; Ercilla-Montserrat et al., 2017; Nadel et al., 2017; Stadler et al., 2017; Sanjuan-Delmas et al., 2018; Gentry, 2019; Baganz et al., 2020; Manriquez-Altamirano et al., 2020). Notable existing examples include ECF in Berlin, Germany, BIGH Ferme Abattoir in Brussels, Belgium, Gotham Greens, in New York, United States, and Vertical Harvest Farms in Jackson, Wyoming, United States. Urban agriculture is sometimes seen as overlapping with a concept regularly mentioned in association with CC- Nature Based Solutions (strategy 12).
An emerging concept, Nature-based solutions (NBS) promote circular resource use of nutrients, water, and energy via the integration of ecosystem driven approaches like consideration of biodiversity, ecosystem services, green infrastructure, and enhancing natural capital (Katsou et al., 2020). Our review found NBS recommended as an implementation strategy under the umbrellas of CC, CE, the FWE-Nexus, and Urban Metabolism. Katsou et al. (2020) assert that NBS can be seen as an intermediate link between CE and CC as its enhancement of natural capital and efficient resource utilization enable transitioning from linear urban flows to circular cities (Katsou et al., 2020). Implementation strategy thirteen, the Valorization of organic waste streams is an important means of transitioning from linear to circular biotic flows. This refers to giving organic waste streams a higher inherent value by redirecting them into new uses and forms of societal, economic, and environmental value. This is a growing area of research and fundamentally emerges from BE and CBE. Organic waste streams in the city can include food waste, wastewater/human wastes, agricultural wastes such as animal manure, and certain industrial process wastes. Through technologies such as anaerobic digestion, organic waste can be processed into useful nutrients, heat, and gasses (Horn and Proksch, 2020b). Various other methods exist to harvest these resources from organic waste streams, which are often closely tied to next uses in agricultural settings to produce food to the cities, and offer a means of actualizing food system circularity and organic flow regeneration in the CC.
4.4 Supporting Strategies
The final three thematic areas contain supporting strategies and actions, fundamental to successful CC transitions by guiding, bolstering, and driving changes. Thematic area four consists of approaches with qualities of shaping accountability and driving implementation through governance, regulation, and stakeholder engagement. This thematic area of Governance and Stakeholder Engagement was broadly advocated for and cited within literature across all frameworks and concepts reviewed in section two. A fundamental aspect of this is implementation strategy fourteen, Policy and Regulatory Changes, which are closely connected to strategies 19–21 Decision Making, Economic Incentives and Disincentives, and Frameworks and Codes. These are made more successful through implementation strategies 16–18, which ground such changes in societal realities and bring in key stakeholders and urban dwellers through Consideration of consumer behaviors, Inclusive Communication, and Collaboration and Cooperation. These social dimensions of implementation are vital not to overlook when shaping successful CC transitions, though in some similar cases this has been the case. Paiho et al. note, for instance, that prominent sustainable city examples such as the Hammarby Model focus on local stocks and flows while overlooking residential behavior and realities such as transportation, they therefore call for “broader and more holistic analyses” through which to assess “interdependencies and identify synergies between versatile urbanization challenges” (Paiho et al., 2020). Effective CC implementation will include consideration of societal impacts and human health in addition to environmental and economic elements, as all are inextricably interconnected in application.
Data, Measurement, and Modeling, the fourth thematic area of implementation strategies is an essential component of actualizing circularity and realizing regenerative potentials in urban contexts. Accordingly, it was unsurprising to find that strategies in this genre were highly discussed across the range of reviewed frameworks (Figure 4). Data, Measurement, and Modeling acts as a mode of characterizing circularity and supporting optimization. Developing and leveraging data consists of approaches like creating and utilizing datasets, information models, and database resources for circularity (Figure 2). This allows for establishing baselines, keeping track of strategies, documenting resources and integration possibilities, and much more. Closely aligned is strategy 29, Mapping, which plays a similar role, with additional analytical potentials, in a spatial dimension. Strategies 23 and 24, Impacts assessments, and Life Cycle Approaches are closely aligned, and support effective decision making and design as well as evaluation of existing, potential, or new systems and strategies. Many methodologies exist and are under development to facilitate this and are necessary to implement CC effectively.
In our literature review, it is notable that LCA was one of the most frequently mentioned strategies, and Life Cycle Approaches received the most literature mentions of all 34 strategies. Ceron-Palma et al. describe the value of analysis and quantification of in-flows and out-flows of cities including energy, materials and natural resources, water, and emissions (Ceron-Palma et al., 2012). This perspective was echoed within many of the reviewed publications which called for systematic quantitative analysis (strategy 28) as an approach to the implementation of circularity. Paiho et al. make a key point on this count with regard to the definition of system boundaries for analysis, noting that it “is an important issue as it defines the breadth and depth of the analysis required to assess the circularity of a city” but that the concept of CC itself can shape a direction for use of concrete boundaries, targets, and indicators for realization of circularity (Paiho et al., 2020). The development of such approaches to the implementation and measurement of CC performance, as well as all previously discussed implementation strategies, will be vitally supported through Research and Education, thematic area six, which plays a role in characterizing and optimizing circularity as well as shaping accountability. While perhaps less direct than other implementation strategies, these approaches account for the generation and dissemination of knowledge necessary to achieve successful circularity, including Assessment of emerging technologies (30), Methodology and Framework development (31), Education (32), Use of transdisciplinary methods, and Applied Research Approaches (34). All 34 strategies stand to benefit from further research and development and continued investigation of how they can be most effectively implemented in order to realize a more holistically sustainable and regenerative future via CC.
5 Conclusion and Future Research Directions
Envisioning and implementing regenerative, symbiotic, and circular cities of the future through the CC framework will ultimately require a complex assortment of context specific approaches and implementation strategies across all six thematic areas- Resource and Material Management, Systems Development and Integration, Bio-based Solutions, Governance and Stakeholder Engagement, Data, Measurement, and Modeling, and Research and Education. Given the many shared goals, conceptualizations, and strategies espoused under the array of circular sustainability frameworks reviewed above, there is reason to discern value in looking to foundational and parallel concepts as a resource for the strengthening and amplification of CC in applied urban settings. We found considerable overlap between the reviewed frameworks and strong alignments between aspects of all, pointing to the value of conducting this type of investigation to identify opportunities to leverage these relationships and to hasten the integration of CC frameworks across scales and sectors. Promise resides in further reflection and learning from successes and failures, as well as in pursuing partnership and collaboration where opportunities arise to align efforts in pursuit of common goals between synergetic schools of thought and disciplines pursuing circularity at differing scales. There is a need to expand insight and understanding of how different scales and sectors pursuing circular sustainability fit together and can partner to drive change and transformation. On this, and all aspects, from the technical, economic, societal, and to the logistical and political, and beyond, more research on implementation of CC is needed. Participation and perspectives from transdisciplinary researchers and stakeholders should comprise no small part in this effort. Even when technological solutions are impeccable, the realities of implementation can be fraught with strategical, social, and political difficulties, making the inclusion of supporting actions in addition to technical solutions all the more vital.
Achieving successful circular cities may be particularly contingent upon a successful transition toward reframing externalities to account for the true invaluable nature of ecosystems health and human wellbeing within economic and urban systems, something which has become all the more evident in light of the COVID-19 pandemic. Likewise, changing societal views and behaviors toward more sustainable practices, which are already in motion, are needed to strengthen a driving and supporting force for change. Accordingly, we will crucially need effective policy and governance formed in partnership with stakeholders. Cities just venturing into this realm should increasingly look to leading circular cities and regions which are already piloting such approaches. Gathering case studies of successful implementation strategies and circular business models to help with legitimation and proof of concept at scale could play an influential role in helping businesses and stakeholders garner support to instigate circular transitions. There may be potential in partnering with existing sustainability certification systems for products, services, and buildings to incorporate further CC concepts in professional approaches with existing notoriety and traction among practitioners. Likewise, assessing existing and emerging technical innovation systems and taking functional analysis and value engineering approaches to evaluate applied solutions for the CC remains an underexplored niche which may offer new insights. Finally, education -both traditional and continuing-should not be overlooked as an invaluable part of larger social change and of forming new leaders, citizens, and innovators. In support of these outcomes, this review offers a new lens to the discussion of CC transitions and applied actions by revisiting the relationships of multiple synergetic sustainability frameworks and the coordination of the implementation strategies they advance to bolster an integrated and transdisciplinary approach. Further work may expand on this lens to find further synergies or deepen exploration of particular opportunities. Likewise, future investigations of particular implementation strategies and technologies can benefit from strategic reflection on the approach’s situation within a coordinated conceptual range, for which this review can provide a starting tool. Through such approaches, the metaphor of not reinventing wheels can become a powerful channel for closing loops and strengthening pathways to achieve successful implementation of a new circular, regenerative, and symbiotic reality for urban sustainability.
Author Contributions
EH was responsible for literature review, figures, analysis, and writing of this publication. GP advised and supervised the research process, contributed to figure concepts and creation, and provided review and guidance.
Funding
The study originates from the project CITYFOOD, which is part of the Belmont Forum and JPI Urban Europe initiated Food-Water-Energy-Nexus/Sustainability Urbanization Global Initiative (SUGI) Collaborative Research Action. It received funding from the U.S. National Science Foundation (Award 1832213).
Conflict of Interest
The authors declare that the research was conducted in the absence of any commercial or financial relationships that could be construed as a potential conflict of interest.
Publisher’s Note
All claims expressed in this article are solely those of the authors and do not necessarily represent those of their affiliated organizations, or those of the publisher, the editors and the reviewers. Any product that may be evaluated in this article, or claim that may be made by its manufacturer, is not guaranteed or endorsed by the publisher.
Acknowledgments
EH acknowledges, with much gratitude, JC, for proofreading, feedback, and invaluable support throughout the development of this paper.
References
Adams, K. T., Osmani, M., Thorpe, T., and Thornback, J. (2017). Circular Economy in Construction: Current Awareness, Challenges and Enablers. Proc. Inst. Civil Eng. - Waste Resource Manage. 170 (1), 15–24. doi:10.1680/jwarm.16.00011
Allan, T., Keulertz, M., and Woertz, E. (2015). The Water-Food-Energy Nexus: an Introduction to Nexus Concepts and Some Conceptual and Operational Problems. Int. J. Water Resour. Develop. 31 (3), 301–311. doi:10.1080/07900627.2015.1029118
Allenby, B. R., and Graedel, T. E. (1993). E05_0-13-046713-8_01.Pdf. Ind. Ecol. Available at: https://toc.library.ethz.ch/objects/pdf/e05_0-13-046713-8_01.pdf.
Andersen, M. S. (2007). An Introductory Note on the Environmental Economics of the Circular Economy. Sustainability Sci. 2 (1), 133–140. doi:10.1007/s11625-006-0013-6
Baganz, G., Proksch, G., Kloas, W., Lorleberg, W., Baganz, D., Staaks, G., et al. (2020). Site Resource Inventories - a Missing Link in the Circular City's Information Flow. Adv. Geosci. 54, 23–32. doi:10.5194/adgeo-54-23-2020
Bazilian, M., Rogner, H., Howells, M., Hermann, S., Arent, D., Gielen, D., et al. (2011). Considering the Energy, Water and Food Nexus: Towards an Integrated Modelling Approach. Energy Policy 39 (12), 7896–7906. doi:10.1016/j.enpol.2011.09.039
Beaulieu, L. (2015). Circular Economy: A Critical Literature Review of Concepts. Available at: https://www.academia.edu/20226696/Circular_Economy_a_Critical_Literature_Review_of_Concepts (Accessed October 6, 2020).
Bizikova, L., Roy, D., Swanson, D., Venema, H. D., and McCandless, M. (2013). The Water–Energy–Food Security Nexus: Towards A Practical Planning and Decision Support Framework for Landscape Investment and Risk Management. International Institute for Sustainable Development.
Blanco, E., Pedersen Zari, M. Z., Raskin, K., and Clergeau, P. (2021). Urban Ecosystem-Level Biomimicry and Regenerative Design: Linking Ecosystem Functioning and Urban Built Environments. Sustainability 13 (1), 404. doi:10.3390/su13010404
Bocken, N. M. P., de Pauw, I., Bakker, C., and van der Grinten, B. (2016). Product Design and Business Model Strategies for a Circular Economy. J. Ind. Prod. Eng. 33 (5), 308–320. doi:10.1080/21681015.2016.1172124
Boulding, K. E. (2017). The Economics of the Coming Spaceship Earth. Environ. Qual. Issues Growing Econ.
Brown, B. J., Hanson, M. E., Liverman, D. M., and Merideth, R. W. (1987). Global Sustainability: Toward Definition. Environ. Manage. 11 (6), 713–719. doi:10.1007/BF01867238
Buck, N. T. (2017). The Art of Imitating Life: The Potential Contribution of Biomimicry in Shaping the Future of Our Cities. Environ. Plann. B: Urban Analytics City Sci. 44 (1), 120–140. doi:10.1177/0265813515611417
Buck, N. T., and While, A. (2021). The Urban Bioeconomy: Extracting Value from the Ecological and Biophysical. J. Environ. Plann. Manage. 64 (2), 182–201. doi:10.1080/09640568.2020.1763931
Bugge, M., Hansen, T., and Klitkou, A. (2016). What Is the Bioeconomy? A Review of the Literature. Sustainability 8 (7), 691. doi:10.3390/su8070691
Build a Circular Economy (2021). Let’s Build a Circular Economy. Available at: https://ellenmacarthurfoundation.org/ (Accessed September 20, 2021).
Campioli, A., Mussinelli, E., Lavagna, M., and Tartaglia, A. (2020). “Design Strategies and LCA of Alternative Solutions for Resilient, Circular, and Zero-Carbon Urban Regeneration: A Case Study,” in Regeneration of the Built Environment from a Circular Economy Perspective. Research for Development. Editors S. D. Torre, S. Cattaneo, C. Lenzi, and A. Zanelli (Cham: Springer International Publishing), 205–215. doi:10.1007/978-3-030-33256-3_20
Caputo, P., Ferrari, S., and Zagarella, F. (2020). “Urban Renovation: An Opportunity for Economic Development, Environmental Improvement, and Social Redemption,” in Regeneration of the Built Environment from a Circular Economy Perspective. Research for Development. Editors S. D. Torre, S. Cattaneo, C. Lenzi, and A. Zanelli (Cham: Springer International Publishing), 125–135. doi:10.1007/978-3-030-33256-3_13
Carcassi, O. B., De Angelis, E., Iannaccone, G., Malighetti, L. E., Masera, G., and Pittau, F. (2020). “Bio-Based Materials for the Italian Construction Industry: Buildings as Carbon Sponges,” in Regeneration of the Built Environment from a Circular Economy Perspective. Research for Development. Editors S. D. Torre, S. Cattaneo, C. Lenzi, and A. Zanelli (Cham: Springer International Publishing), 237–247. doi:10.1007/978-3-030-33256-3_23
Carraresi, L., Berg, S., and Bröring, S. (2018). Emerging Value Chains within the Bioeconomy: Structural Changes in the Case of Phosphate Recovery. J. Clean. Prod. 183, 87–101. doi:10.1016/j.jclepro.2018.02.135
Carus, M. (2017). The Bioeconomy Is Much More Than a Circular Economy. Text/html. BLICKWINKEL. BLICKWINKEL. Available at: https://www.brain-biotech.com/blickwinkel/circular/the-bioeconomy-is-much-more-than-a-circular-economy/ (Accessed August 30, 2017).
Castán-Broto, V., and Bulkeley, H. (2013). A Survey of Urban Climate Change Experiments in 100 Cities. Glob. Environ. Change 23 (1), 92–102. doi:10.1016/j.gloenvcha.2012.07.005
Cerón-Palma, I., Sanyé-Mengual, E., Oliver-Solà, J., Montero, J.-I., and Rieradevall, J. (2012). Barriers and Opportunities Regarding the Implementation of Rooftop Eco.Greenhouses (RTEG) in Mediterranean Cities of Europe. J. Urban Technol. 19, 87–103. doi:10.1080/10630732.2012.717685
Chertow, M. R. (2007). "Uncovering" Industrial Symbiosis. J. Ind. Ecol. 11 (1), 11–30. doi:10.1162/jiec.2007.1110
Chertow, M. R. (2000). INDUSTRIAL SYMBIOSIS: Literature and Taxonomy. Annu. Rev. Energ. Environ. 25 (1), 313–337. doi:10.1146/annurev.energy.25.1.313
Cheshire, D. (2016). Building Revolutions: Applying the Circular Economy to the Built Environment. London: RIBA Publishing.
Chester, M. V. (2020). Industrial Ecology in Support of Climate Change Adaptation. J. Ind. Ecol. 24 (2), 271–275. doi:10.1111/jiec.13006
Corcelli, F., Fiorentino, G., Petit-Boix, A., Rieradevall, J., and Gabarrell, X. (2019). Transforming Rooftops into Productive Urban Spaces in the Mediterranean. An LCA Comparison of Agri-Urban Production and Photovoltaic Energy Generation. Resour. Conservation Recycling 144, 321–336. doi:10.1016/j.resconrec.2019.01.040
Cordell, D., Drangert, J.-O., and White, S. (2009). The Story of Phosphorus: Global Food Security and Food for Thought. Glob. Environ. Change 19 (2), 292–305. doi:10.1016/j.gloenvcha.2008.10.009
Crosson, C. (2018). Innovating the Urban Water System: Achieving a Net Zero Water Future beyond Current Regulation. Technol.|Architecture + De.n 2 (1), 68–81. doi:10.1080/24751448.2018.1420966
Curtis, F. (2003). Eco-Localism and Sustainability. Ecol. Econ. 46 (1), 83–102. doi:10.1016/S0921-8009(03)00102-2
D'Amato, D., Droste, N., Allen, B., Kettunen, M., Lähtinen, K., Korhonen, J., et al. (2017). Green, Circular, Bio Economy: A Comparative Analysis of Sustainability Avenues. J. Clean. Prod. 168, 716–734. doi:10.1016/j.jclepro.2017.09.053
Davidsson, Å., la Cour Jansen, J., Appelqvist, B., Gruvberger, C., and Hallmer, M. (2007). Anaerobic Digestion Potential of Urban Organic Waste: a Case Study in Malmö. Waste Manag. Res. 25 (2), 162–169. doi:10.1177/0734242X07075635
Ddiba, D., Andersson, K., Rosemarin, A., Schulte-Herbrüggen, H., and Dickin, S. (2021). The Circular Economy Potential of Urban Organic Waste Streams in Low- and Middle-Income Countries. Environ. Dev. Sustain. 2021, 1–29. doi:10.1007/s10668-021-01487-w
Delor, M. (2011). Current State of Building-Integrated Agriculture, its Energy Benefits and Comparison with Green Roofs - Summary. Sheffield, England: University of Sheffield.
De Stercke, S., Vaibhav, C., Buytaert, W., and Mijic, A. (2020). Water-Energy Nexus-Based Scenario Analysis for Sustainable Development of Mumbai. Environ. Modell. Softw. 134, 104854. doi:10.1016/j.envsoft.2020.104854
Dipeolu, A. A., Akpa, O. M., and Fadamiro, A. J. (2020). Mitigating Environmental Sustainability Challenges and Enhancing Health in Urban Communities: The Multi-Functionality of Green Infrastructure. Jcua 4 (1), 33–46. doi:10.25034/ijcua.2020.v4n1-4
dos Santos, M. J. P. L. (2016). Smart Cities and Urban Areas-Aquaponics as Innovative Urban Agriculture. Urban For. Urban Green. 20, 402–406. doi:10.1016/j.ufug.2016.10.004
Doughty, M. R. C., and Hammond, G. P. (2004). Sustainability and the Built Environment at and beyond the City Scale. Building Environ. 39 (10), 1223–1233. doi:10.1016/j.buildenv.2004.03.008
Drożdż-Szczybura, M. (2015). The Architectural Expression of Buildings Realising the Ideas of Urban Agriculture. Czasopismo Techniczne 2015, 29–52. doi:10.4467/2353737XCT.15.002.3747
Dzene, I., Barisa, A., Rosa, M., and Dobraja, K. (2016). A Conceptual Methodology for Waste-To-Biomethane Assessment in an Urban Environment. Energ. Proced. 95, 3–10. doi:10.1016/j.egypro.2016.09.002
Edwards, J., Othman, M., and Burn, S. (2015). A Review of Policy Drivers and Barriers for the Use of Anaerobic Digestion in Europe, the United States and Australia. Renew. Sustain. Energ. Rev. 52, 815–828. doi:10.1016/j.rser.2015.07.112
Elia, V., Gnoni, M. G., and Tornese, F. (2017). Measuring Circular Economy Strategies through Index Methods: A Critical Analysis. J. Clean. Prod. 142, 2741–2751. doi:10.1016/j.jclepro.2016.10.196
Elkington, J. (1999). Cannibals with Forks: The Triple Bottom Line of 21st Century Business. Choice Rev. Online 36 (07), 36–3997. doi:10.5860/CHOICE.36-3997
Ellen MacArthur Foundation (2017). Cities in the Circular Economy: An Initial Exploration. Available at: https://ellenmacarthurfoundation.org/cities-in-the-circular-economy-an-initial-exploration.
Ercilla-Montserrat, M., Izquierdo, R., Belmonte, J., Montero, J. I., Muñoz, P., De Linares, C., et al. (2017). Building-Integrated Agriculture: A First Assessment of Aerobiological Air Quality in Rooftop Greenhouses (I-RTGs). Sci. Total Environ. 598, 109–120. doi:10.1016/j.scitotenv.2017.04.099
Fiori, M., Poli, T., Mainini, A. G., Blanco Cadena, J. D., Speroni, A., and Bocchiola, D. (2020). “Assessing Water Demand of Green Roofs under Variants of Climate Change Scenarios,” in Regeneration of the Built Environment from a Circular Economy Perspective. Research for Development. Editors S. D. Torre, S. Cattaneo, C. Lenzi, and A. Zanelli (Cham: Springer International Publishing), 375–380. doi:10.1007/978-3-030-33256-3_35
Fletcher, T. D., Andrieu, H., and Hamel, P. (2013). Understanding, Management and Modelling of Urban Hydrology and its Consequences for Receiving Waters: A State of the Art. Adv. Water Resour. 51, 261–279. doi:10.1016/j.advwatres.2012.09.001
Folke, C., Jansson, Å., Larsson, J., and Costanza, R. (1997). Ecosystem Appropriation by Cities. Ambio 26 (3), 167–172.
Frosch, R. A., and Gallopoulos, N. E. (1989). Strategies for Manufacturing. Sci. Am. 261 (3), 144–152. doi:10.1038/scientificamerican0989-144
Gagliano, A., Nocera, F., D’Amico, A., and Spataru, C. (2015). Geographical Information System as Support Tool for Sustainable Energy Action Plan. Energ. Proced. 83, 310–319. doi:10.1016/j.egypro.2015.12.185
Gallego-Schmid, A., Chen, H.-M., Sharmina, M., and Mendoza, J. M. F. (2020). Links between Circular Economy and Climate Change Mitigation in the Built Environment. J. Clean. Prod. 260, 121115. doi:10.1016/j.jclepro.2020.121115
Gandy, M. (2004). Rethinking Urban Metabolism: Water, Space and the Modern City. City 8 (3), 363–379. doi:10.1080/1360481042000313509
Garcia, D. J., and You, F. (2016). The Water-Energy-Food Nexus and Process Systems Engineering: A New Focus. Comput. Chem. Eng. 91, 49–67. doi:10.1016/j.compchemeng.2016.03.003
Gehrke, I. (2014). “Building-Integrated Water Reuse in Combination with Urban Farming,” in SUSTAINABLE CITY 2014 Volume: 191, Siena, Italy, 1603–1607. doi:10.2495/SC141362
Geissdoerfer, M., Savaget, P., Bocken, N. M. P., Hultink, E. J., and Jan Hultink, Erik. (2017). The Circular Economy - A New Sustainability Paradigm? J. Clean. Prod. 143, 757–768. doi:10.1016/j.jclepro.2016.12.048
Geldermans, R. J. (2016). Design for Change and Circularity - Accommodating Circular Material & Product Flows in Construction. Energ. Proced. 96, 301–311. doi:10.1016/j.egypro.2016.09.153
Gentry, M. (2019). Local Heat, Local Food: Integrating Vertical Hydroponic Farming with District Heating in Sweden. Energy 174, 191–197. doi:10.1016/j.energy.2019.02.119
Georgescu-Roegen, N. (1975). Energy and Economic Myths. South. Econ. J. 41 (3), 347–381. doi:10.2307/1056148
GhaffarianHoseini, A., Dahlan, N. D., Berardi, U., Ghaffarian Hoseini, A., Makaremi, N., Ghaffarian Hoseini, M., et al. (2013). Sustainable Energy Performances of Green Buildings: A Review of Current Theories, Implementations and Challenges. Renew. Sustain. Energ. Rev. 25, 1–17. doi:10.1016/j.rser.2013.01.010
Ghisellini, P., Cialani, C., and Ulgiati, S. (2016). A Review on Circular Economy: The Expected Transition to a Balanced Interplay of Environmental and Economic Systems. J. Clean. Prod. 114 (15), 11–32. doi:10.1016/j.jclepro.2015.09.007
Gibbs, D., Deutz, P., and Proctor, A. (2005). Industrial Ecology and Eco‐industrial Development: A Potential Paradigm for Local and Regional Development? Reg. Stud. 39, 171–183. doi:10.1080/003434005200059959
Ginelli, E., Chesi, C., Pozzi, G., Lazzati, G., Pirillo, D., and Vignati, G. (2020a). “Extra-Ordinary Solutions for Useful Smart Living,” in Regeneration of the Built Environment from a Circular Economy Perspective. Research for Development. Editors S. D. Torre, S. Cattaneo, C. Lenzi, and A. Zanelli (Cham: Springer International Publishing), 347–356. doi:10.1007/978-3-030-33256-3_32
Ginelli, E., Pozzi, G., Lazzati, G., Pirillo, D., and Vignati, G. (2020b). “Regenerative Urban Space: A Box for Public Space Use,” in Regeneration of the Built Environment from a Circular Economy Perspective. Research for Development. Editors S. D. Torre, S. Cattaneo, C. Lenzi, and A. Zanelli (Cham: Springer International Publishing), 137–147. doi:10.1007/978-3-030-33256-3_14
Giorgi, S., Lavagna, M., and Campioli, A. (2020). “Circular Economy and Regeneration of Building Stock: Policy Improvements, Stakeholder Networking and Life Cycle Tools,” in Regeneration of the Built Environment from a Circular Economy Perspective. Research for Development. Editors S. D. Torre, S. Cattaneo, C. Lenzi, and A. Zanelli (Cham: Springer International Publishing), 291–301. doi:10.1007/978-3-030-33256-3_27
Glass, J., Greenfield, D., and Longhurst, P. (2017). Editorial: Circular Economy in the Built Environment. Proc. Inst. Civil Eng. - Waste Resource Manage. 170 (1), 1–2. doi:10.1680/jwarm.2017.170.1.1
Gleick, P. H. (1994). Water and Energy. Annu. Rev. Energ. Environ. 19 (1), 267–299. doi:10.1146/annurev.eg.19.110194.001411
Grimm, N. B., Faeth, S. H., Golubiewski, N. E., Redman, C. L., Wu, J., Bai, X., et al. (2008). Global Change and the Ecology of Cities. Science 319, 5864756–5864760. doi:10.1126/science.1150195
Hart, J., Adams, K., Giesekam, J., Tingley, D. D., and Pomponi, F. (2019). Barriers and Drivers in a Circular Economy: The Case of the Built Environment. Proced. CIRP 80, 619–624. doi:10.1016/j.procir.2018.12.015
Hodson, M., Marvin, S., Robinson, B., and Swilling, M. (2012). Reshaping Urban Infrastructure. J. Ind. Ecol. 16 (6), 789–800. doi:10.1111/j.1530-9290.2012.00559.x
Horn, E., and Proksch, G. (2020a). Building an Ecosystem: Integrating Rooftop Aquaponics with a Brewery to Advance the Circular Economy.
Horn, E., and Proksch, G. (2020b). Sourcing Energy from Waste in the Circular City: Integrated Anaerobic Digestion toward Long Term Decarbonization.
Huovila, P., Iyer-Raniga, U., and Maity, S. (2020). Circular Economy in the Built Environment: Supporting Emerging Concepts - IOPscience. Available at: https://iopscience-iop-org.offcampus.lib.washington.edu/article/10.1088/1755-1315/297/1/012003 (Accessed December 8, 2020).
Iacovidou, E., and Purnell, P. (2016). Mining the Physical Infrastructure: Opportunities, Barriers and Interventions in Promoting Structural Components Reuse. Sci. Total Environ. 557-558, 791–807. doi:10.1016/j.scitotenv.2016.03.098
International Living Future Institute (ILFI) (2020). Living Building Challenge | Living-Future.Org. Available at: https://living-future.org/lbc/ (Accessed February 19, 2020).
ISO 15392 (2008). 14:00-17:00. “ISO 15392:2008.” ISO. Available at: https://www.iso.org/cms/render/live/en/sites/isoorg/contents/data/standard/04/04/40432.html (Accessed September 20, 2021).
Jackson, T. (2009). Prosperity without Growth: Economics for a Finite Planet. London ; Sterling, VA: Earthscan.
James, D. (2018). The Novel as Encyclopedia. Realising the Circular Bioeconomy 2018, 74–90. doi:10.1017/9781316659694.006
Joensuu, T., Edelman, H., and Saari, A. (2020). Circular Economy Practices in the Built Environment. J. Clean. Prod. 276, 124215. doi:10.1016/j.jclepro.2020.124215
European Investment Bank Jonas, B. (2018). The 15 Circular Steps for Cities. Luxembourg: Publications Office of the European Union. Available at: https://data.europa.eu/doi/10.2867/39283.
Jonsson, D. (2000). Sustainable Infrasystem Synergies: A Conceptual Framework. J. Urban Technol. 7 (3), 81–104. doi:10.1080/713684136
Karhu, J., and Linkola, L. (2019). Circular Economy in the Built Environment in Finland - A Case Example of Collaboration. IOP Conf. Ser. Earth Environ. Sci. 297, 012024. doi:10.1088/1755-1315/297/1/012024
Kates, R., Parris, T., and Leiserowitz, A. (2005). What Is Sustainable Development? Goals, Indicators, Values and Practice. Available at: https://climatecommunication.yale.edu/publications/what-is-sustainable-development/.
Katsou, E., Nika, C.-E., Buehler, D., Marić, B., Megyesi, B., Mino, E., et al. (2020). Transformation Tools Enabling the Implementation of Nature-Based Solutions for Creating a Resourceful Circular City. Blue-Green Syst. 2 (1), 188–213. doi:10.2166/bgs.2020.929
Keeble, B. R. (1988). The Brundtland Report: 'Our Common Future'. Med. War 4 (1), 17–25. doi:10.1080/07488008808408783
Kennedy, C. A., Stewart, I., Facchini, A., Cersosimo, I., Mele, R., Chen, B., et al. (2015). Energy and Material Flows of Megacities. Proc. Natl. Acad. Sci. USA 112 (19), 5985–5990. doi:10.1073/pnas.1504315112
Kennedy, C., Cuddihy, J., and Engel-Yan, J. (2007). The Changing Metabolism of Cities. J. Ind. Ecol. 11 (2), 43–59. doi:10.1162/jie.2007.1107
Kennedy, C., and Hoornweg, D. (2012). Mainstreaming Urban Metabolism. J. Ind. Ecol. 16 (6), 780–782. doi:10.1111/j.1530-9290.2012.00548.x
Kirchherr, J., Reike, D., and Hekkert, M. (2017). Conceptualizing the Circular Economy: An Analysis of 114 Definitions. Resour. Conservation Recycling 127, 221–232. doi:10.1016/j.resconrec.2017.09.005
Kleinschmit, D., Lindstad, B. H., Thorsen, B. J., Toppinen, A., Roos, A., and Baardsen, S. (2014). Shades of Green: A Social Scientific View on Bioeconomy in the Forest Sector. Scand. J. For. Res. 29 (4), 402–410. doi:10.1080/02827581.2014.921722
Kollikkathara, N., Feng, H., and Stern, E. (2009). A Purview of Waste Management Evolution: Special Emphasis on USA. Waste Manage. 29 (2), 974–985. doi:10.1016/j.wasman.2008.06.032
Korhonen, J., Honkasalo, A., and Seppälä, J. (2018). Circular Economy: The Concept and its Limitations. Ecol. Econ. 143, 37–46. doi:10.1016/j.ecolecon.2017.06.041
Kujundzic, K., and Vuckovic, S. S. (2019). Cultural Landscape Devastation as a Consequence of Poor Sustainable Urban Development Practice Case Study: Kostanjica, Boka Bay, Montenegro. Jcua 3 (1), 121–131. doi:10.25034/ijcua.2018.4689
Langergraber, G., Pucher, B., Simperler, L., Kisser, J., Katsou, E., Buehler, D., et al. (2020). Implementing Nature-Based Solutions for Creating a Resourceful Circular City. Blue-Green Syst. 2 (1), 173–185. doi:10.2166/bgs.2020.933
Lee, U., Han, J., and Wang, M. (2017). Evaluation of Landfill Gas Emissions from Municipal Solid Waste Landfills for the Life-Cycle Analysis of Waste-To-Energy Pathways. J. Clean. Prod. 166, 335–342. doi:10.1016/j.jclepro.2017.08.016
Lenhart, J., van Vliet, B., and Mol, A. P. J. (2015). New Roles for Local Authorities in a Time of Climate Change: the Rotterdam Energy Approach and Planning as a Case of Urban Symbiosis. J. Clean. Prod. 107, 593–601. doi:10.1016/j.jclepro.2015.05.026
Lewandowski, M. (2016). Designing the Business Models for Circular Economy-Towards the Conceptual Framework. Sustainability 8 (1), 43. doi:10.3390/su8010043
Liang, S., and Zhang, T. (2011). Urban Metabolism in China Achieving Dematerialization and Decarbonization in Suzhou. J. Ind. Ecol. 15 (3), 420–434. doi:10.1111/j.1530-9290.2011.00343.x
Lowe, E. A., Moran, S. R., Holmes, D. B., Parks, E-I., Doyle, B., Lowe, E. A., et al. (1996). A Fieldbook for the Development of Eco-Industrial Parks.
Lyle, J. T. (1994). Regenerative Design for Sustainable Development. New York: John Wiley. Available at: https://archive.org/details/regenerativedesi0000lyle.
Manríquez-Altamirano, A., Sierra-Pérez, J., Muñoz, P., and Gabarrell, X. (2020). Analysis of Urban Agriculture Solid Waste in the Frame of Circular Economy: Case Study of Tomato Crop in Integrated Rooftop Greenhouse. Sci. Total Environ. 734, 139375. doi:10.1016/j.scitotenv.2020.139375
McDonald, R. I., Weber, K., Padowski, J., Flörke, M., Schneider, C., Green, P. A., et al. (2014). Water on an Urban Planet: Urbanization and the Reach of Urban Water Infrastructure. Glob. Environ. Change 27, 96–105. doi:10.1016/j.gloenvcha.2014.04.022
McDonnell, M., Amy, H., and Brueste, J. (2009). Ecology of Cities and Towns. Ecol. Cities Towns: A Comp. Approach. doi:10.1017/CBO9780511609763
McDonough, W., and Braungart, M. (2009). Cradle to Cradle: Remaking the Way We Make Things. New York City: Vintage.
Meadows, D., Meadows, D., Randers, J., and Behrens, W. (1972). The Limits to Growth. Available at: https://www.clubofrome.org/publication/the-limits-to-growth/.
Mendoza, J. M. F., Sharmina, M., Gallego-Schmid, A., Heyes, G., and Azapagic, A. (2017). Integrating Backcasting and Eco-Design for the Circular Economy: The BECE Framework. J. Ind. Ecol. 21 (3), 526–544. doi:10.1111/jiec.12590
Meng, F., Liu, G., Liang, S., Su, M., and Yang, Z. (2019). Critical Review of the Energy-Water-Carbon Nexus in Cities. Energy 171, 1017–1032. doi:10.1016/j.energy.2019.01.048
Moglia, M. (2014). Urban Agriculture and Related Water Supply: Explorations and Discussion. Habitat Int. 42, 273–280. doi:10.1016/j.habitatint.2014.01.008
Moriguchi, Y. (2007). Material Flow Indicators to Measure Progress toward a Sound Material-Cycle Society. J. Mater. Cycles Waste Manag. 9 (2), 112–120. doi:10.1007/s10163-007-0182-0
Mukuve, F. M., and Fenner, R. A. (2015). Scale Variability of Water, Land, and Energy Resource Interactions and Their Influence on the Food System in Uganda. Sustainable Prod. Consumption 2, 79–95. doi:10.1016/j.spc.2015.07.009
Munaro, M. R., Tavares, S. F., and Bragança, L. (2020). Towards Circular and More Sustainable Buildings: A Systematic Literature Review on the Circular Economy in the Built Environment. J. Clean. Prod. 260, 121134. doi:10.1016/j.jclepro.2020.121134
Nadal, A., Llorach-Massana, P., Cuerva, E., López-Capel, E., Montero, J. I., Josa, A., et al. (2017). Building-Integrated Rooftop Greenhouses: An Energy and Environmental Assessment in the Mediterranean Context. Appl. Energ. 187, 338–351. doi:10.1016/j.apenergy.2016.11.051
Newell, J. P., Goldstein, B., and Foster, A. (2019). A 40-year Review of Food-Energy-Water Nexus Literature and its Application to the Urban Scale. Environ. Res. Lett. 14 (7), 073003. doi:10.1088/1748-9326/ab0767
Orr, D. W. (1992). The Problem of Education. New Directions Higher Educ. 77, 3–8. doi:10.1002/he.36919927703
Paiho, S., Mäki, E., Wessberg, N., Paavola, M., Tuominen, P., Antikainen, M., et al. (2020). Towards Circular Cities-Conceptualizing Core Aspects. Sustain. Cities Soc. 59, 102143. doi:10.1016/j.scs.2020.102143
Pandis Iveroth, S., Vernay, A.-L., Mulder, K. F., and Brandt., N. (2013). Implications of Systems Integration at the Urban Level: the Case of Hammarby Sjöstad, Stockholm. J. Clean. Prod. 48, 220–231. doi:10.1016/j.jclepro.2012.09.012
Pauli, G. A. (2010). The Blue Economy: 10 years, 100 Innovations, 100 Million Jobs. Brookline, Massachusetts: Paradigm Publications.
Pearce, D. W., and Kerry Turner, R. (1990). Economics of Natural Resources and the Environment. Baltimore, Maryland, USA: JHU Press.
Peters, G. P., Weber, C. L., Guan, D., and Hubacek, K. (2007). China's Growing CO2 EmissionsA Race between Increasing Consumption and Efficiency Gains. Environ. Sci. Technol. 41, 5939–5944. doi:10.1021/es070108f
Petit-Boix, A., and Leipold, S. (2018). Circular Economy in Cities: Reviewing How Environmental Research Aligns with Local Practices. J. Clean. Prod. 195, 1270–1281. doi:10.1016/j.jclepro.2018.05.281
Pfau, S., Hagens, J., Dankbaar, B., and Smits, A. (2014). Visions of Sustainability in Bioeconomy Research. Sustainability 6 (3), 1222–1249. doi:10.3390/su6031222
Pomponi, F., and Moncaster, A. (2017). Circular Economy for the Built Environment: A Research Framework. J. Clean. Prod. 143, 710–718. doi:10.1016/j.jclepro.2016.12.055
Pons, O., Nadal, A., Sanyé-Mengual, E., Llorach-Massana, P., Cuerva, E., Sanjuan-Delmàs, D., et al. (2015). Roofs of the Future: Rooftop Greenhouses to Improve Buildings Metabolism. Proced. Eng. 123, 441–448. doi:10.1016/j.proeng.2015.10.084
Pratt, T., Allen, T., Allen, L. N., Keller, A. A., Rosenberg, D. E., Keller, A. A., et al. (2019). Urban Agriculture and Small Farm Water Use: Case Studies and Trends from Cache Valley, Utah. Agric. Water Manage. 213, 24–35. doi:10.1016/j.agwat.2018.09.034
Prendeville, S., Cherim, E., and Bocken, N. (2018). Circular Cities: Mapping Six Cities in Transition. Environ. Innovation Societal Transitions 26, 171–194. doi:10.1016/j.eist.2017.03.002
Prieto-Sandoval, V., Jaca, C., and Ormazabal, M. (2018). Towards a Consensus on the Circular Economy. J. Clean. Prod. 179, 605–615. doi:10.1016/j.jclepro.2017.12.224
Proksch, G., Ianchenko, A., and Kotzen, B. (2019). Aquaponics in the Built Environment. Aquaponics Food Prod. Syst. 2019, 523–558. doi:10.1007/978-3-030-15943-6_21
Proksch, G. (2016). Creating Urban Agricultural Systems : An Integrated Approach to Design. 1st ed. New York: Routledge. Available at: https://search.proquest.com/agricenvironm/docview/1999775647/6BCE38C1DCA448F7PQ/1.
Putnam, R. (1993). The Prosperous Community: Social Capital and Public Life. The Am. Prospect 13, 35–42.
Rahbarianyazd, R. (2017). Sustainability in Historic Urban Environments: Effect of Gentrification in the Process of Sustainable Urban Revitalization. Jcua 1 (1), 1–9. doi:10.25034/1761.1(1)1-9
Ramaswami, A., Boyer, D., Nagpure, A. S., Fang, A., Bogra, S., Bakshi, B., et al. (2017). An Urban Systems Framework to Assess the Trans-boundary Food-Energy-Water Nexus: Implementation in Delhi, India. Environ. Res. Lett. 12 (2), 025008. doi:10.1088/1748-9326/aa5556
Ranhagen, U., and Groth, K. (2012). The Symbiocity Approach : A Conceptual Framework for Sustainable Urban Development. Stockholm, Sweden: SKL International. Available at: http://urn.kb.se/resolve?urn=urn:nbn:se:du-34271.
Reed, B. (2007). Shifting from 'sustainability' to Regeneration. Building Res. Inf. 35 (6), 674–680. doi:10.1080/09613210701475753
Ribić, B., Voća, N., and Ilakovac, B. (2017). Concept of Sustainable Waste Management in the City of Zagreb: Towards the Implementation of Circular Economy Approach. J. Air Waste Manage. Assoc. 67 (2), 241–259. doi:10.1080/10962247.2016.1229700
Roos, A., and Stendahl, M. (2015). “The Emerging Bio-Economy and the Forest Sector,” in Forests, Business and Sustainability (Oxfordshire, England, UK: Routledge).
Sale, K. (1985). Dwellers in the Land: The Bioregional Vision. Athens, Georgia: University of Georgia Press.
Sanjuan-Delmás, D., Llorach-Massana, P., Nadal, A., Ercilla-Montserrat, M., Muñoz, P., Montero, J. I., et al. (2018). Environmental Assessment of an Integrated Rooftop Greenhouse for Food Production in Cities. J. Clean. Prod. 177, 326–337. doi:10.1016/j.jclepro.2017.12.147
Simmonds, P. L. (1862). Waste Products and Undeveloped Substances: or, Hints for Enterprise in Neglected Fields. Hardwicke, NZ: R. Hardwicke.
Skar, S. L. G., Pineda-Martos, R., Timpe, A., Pölling, B., Bohn, K., Külvik, M., et al. (2019). Urban Agriculture as a Keystone Contribution towards Securing Sustainable and Healthy Development for Cities in the Future. Blue-Green Syst. 2, 1–27. doi:10.2166/bgs.2019.931
Spiegelhalter, T., and Arch, R. A. (2010). “Biomimicry and Circular Metabolism for the Cities of the Future,” in THE SUSTAINABLE CITY 2010 Volume: 129, La Coruna, Spain, 215–226. doi:10.2495/SC100191
Spiegelhalter, T. (2010). Designing Cities of the Future with the Principles of Biomimicry and Circular Metabolism. Des. Principles Practices: Int. Journal-Annual Rev. 4 (2), 267–280. doi:10.18848/1833-1874/CGP/v04i02/37857
Stadler, M. M., Baganz, D., Vermeulen, T., and Keesman, K. J. (2017). Circular Economy and Economic Viability of Aquaponic Systems: Comparing Urban, Rural and Peri-Urban Scenarios under Dutch Conditions. Acta Horticulturae 1176, 101. doi:10.17660/actahortic.2017.1176.14
Stahel, W., and Reday-Mulvey, G. (1976). Jobs for Tomorrow: The Potential for Substituting Manpower for Energy. January 1, 1981.
Stegmann, P., Londo, M., and Junginger, M. (2020). The Circular Bioeconomy: Its Elements and Role in European Bioeconomy Clusters. Resour. Conservation Recycling: X 6, 100029. doi:10.1016/j.rcrx.2019.100029
Su, B., Heshmati, A., Geng, Y., and Yu, X. (2013). A Review of the Circular Economy in China: Moving from Rhetoric to Implementation. J. Clean. Prod. 42, 215–227. doi:10.1016/j.jclepro.2012.11.020
Suárez-Eiroa, B., Fernández, E., Méndez-Martínez, G., and Soto-Oñate, D. (2019). Operational Principles of Circular Economy for Sustainable Development: Linking Theory and Practice. J. Clean. Prod. 214, 952–961. doi:10.1016/j.jclepro.2018.12.271
Tahir, S., Steichen, T., and Martin, S. (2018). Water and Circular Economy: A White Paper (Jeffries, N. ed.). Available at: https://nextgenwater.eu/wp-content/uploads/2018/10/Water_and_circular_economy-Co.Project_White_paper.pdf.
Thiriet, P., Bioteau, T., and Tremier, A. (2020). Optimization Method to Construct Micro-anaerobic Digesters Networks for Decentralized Biowaste Treatment in Urban and Peri-Urban Areas. J. Clean. Prod. 243, 118478. doi:10.1016/j.jclepro.2019.118478
Tien, I. (2018). Resilient by Design: The Case for Increasing Resilience of Buildings and Their Linked Food-Energy-Water Systems. Elem. Sci. Anth 6 (1), 142. doi:10.1525/elementa.142
Toboso‐Chavero, S., Nadal, A., Petit‐Boix, A., Pons, O., Villalba, G., Gabarrell, X., et al. (2018). Towards Productive Cities: Environmental Assessment of the Food‐Energy‐Water Nexus of the Urban Roof Mosaic. J. Ind. Ecol. 23, 767–780. doi:10.1111/jiec.12829
UN (2020). “Sustainable Development Goals.” unfoundation.Org. Available at: https://unfoundation.org/what-we-do/issues/sustainable-development-goals/ (Accessed September 20, 2021).
Weidner, T., and Yang, A. (2020). The Potential of Urban Agriculture in Combination with Organic Waste Valorization: Assessment of Resource Flows and Emissions for Two European Cities. J. Clean. Prod. 244, 118490. doi:10.1016/j.jclepro.2019.118490
Williams, J. (2005). Sun, Surf and Sustainable Housing-Cohousing, the Californian Experience. Int. Plann. Stud. 10 (2), 145–177. doi:10.1080/13563470500258824
Winans, K., Kendall, A., and Deng, H. (2017). The History and Current Applications of the Circular Economy Concept. Renew. Sustain. Energ. Rev. 68, 825–833. doi:10.1016/j.rser.2016.09.123
Winkler, J., and Bilitewski, B. (2007). Comparative Evaluation of Life Cycle Assessment Models for Solid Waste Management. Waste Manag. 27, 1021–1031. doi:10.1016/j.wasman.2007.02.023
Wolman, A. (1965). The Metabolism of Cities. Sci. Am. 213 (3), 178–190. doi:10.1038/scientificamerican0965-178
Xia, C., and Chen, B. (2020). Urban Land-Carbon Nexus Based on Ecological Network Analysis. Appl. Ener. 276, 115465. doi:10.1016/j.apenergy.2020.115465
Xue, J., Liu, G., Casazza, M., and Ulgiati, S. (2018). Development of an Urban FEW Nexus Online Analyzer to Support Urban Circular Economy Strategy Planning. Energy 164, 475–495. doi:10.1016/j.energy.2018.08.198
Zaman, A. U., and Lehmann, S. (2011). Challenges and Opportunities in Transforming a City into a "Zero Waste City". Challenges 2 (4), 73–93. doi:10.3390/challe2040073
Keywords: circular city, implementation strategies, literature review, circular economy, FWE-Nexus, regenerative design, systems integration
Citation: Horn E and Proksch G (2022) Symbiotic and Regenerative Sustainability Frameworks: Moving Towards Circular City Implementation. Front. Built Environ. 7:780478. doi: 10.3389/fbuil.2021.780478
Received: 21 September 2021; Accepted: 07 December 2021;
Published: 04 March 2022.
Edited by:
Nina Wessberg, VTT Technical Research Centre of Finland Ltd., FinlandReviewed by:
Hourakhsh Ahmad Nia, Alanya Hamdullah Emin Pasa University, TurkeyHui Liu, Central University of Finance and Economics, China
Copyright © 2022 Horn and Proksch. This is an open-access article distributed under the terms of the Creative Commons Attribution License (CC BY). The use, distribution or reproduction in other forums is permitted, provided the original author(s) and the copyright owner(s) are credited and that the original publication in this journal is cited, in accordance with accepted academic practice. No use, distribution or reproduction is permitted which does not comply with these terms.
*Correspondence: Erin Horn, ekh26@uw.edu