- 1Department of Structures for Engineering and Architecture, School of Polytechnic and Basic Sciences, University of Naples “Federico II”, Naples, Italy
- 2Department of Architecture, Built Environment and Construction Engineering, Politechnic of Milan, Milan, Italy
The Italian territory is rich of constructions belonging to the architectural heritage which deserve to be protected against earthquakes. In seismic prone areas ecclesiastic complexes, including churches, bell towers, monasteries, basilicas, synagogues, cathedrals and so on, have shown to be very susceptible at damage, even with partial or total collapses, when undergoing earthquakes. Indeed, these constructions, which are usually designed to withstand gravity loads only, are characterized by slender walls, lack of horizontal floors, bad quality of the masonry apparatus, ineffective connections among walls and between roofs and walls and absence of tie-beams absorbing the thrusts of arches and vaults. All these issues are responsible of the damages suffered by these structures, as detected after the last Italian earthquakes, such as those occurred in L'Aquila (2009), Emilia-Romagna (2012), Central Italy (2016), and Ischia (2017). In the current paper the seismic vulnerability assessment of the bell tower of the SS. Rosario ecclesiastic complex in Finale Emilia (district of Modena, Italy) is presented and discussed. After the geometrical and structural surveys of the whole masonry structure have been performed, the global seismic analysis of the bell tower by the 3Muri analysis software has been done. In particular, the behavioral differences between the isolate condition of the tower and the case within the ecclesiastic complex have been highlighted, showing the aggregate beneficial effect. Finally, proper retrofitting interventions have been designed and applied to the masonry bell tower, considered both as isolate construction and aggregate one, and the different benefits deriving from these interventions in the two inspected cases have been emphasized.
Introduction
Italy is rich of masonry constructions belonging to the architectural heritage, but the majority of them require to be protected against earthquakes. In seismic areas, religious complexes, such as churches, bell towers, monasteries, etc., have shown to be very vulnerable, experiencing partial or total collapses (Doglioni et al., 1994; Krstevska et al., 2010; Tashkov et al., 2010; Lagomarsino, 2012; Brandonisio et al., 2013; Criber et al., 2015; D'Amato et al., 2018; Formisano et al., 2018b). Indeed, these constructions, which are usually designed to withstand gravity loads, are sometimes characterized by slender walls, flexible floors, bad quality of the masonry material, bad interlocking among perpendicular walls and between roof and walls and absence of tie-beams able to absorb the thrusts of arches and vaults. All these issues are responsible of critical damages suffered by such structures, as observed during the last Italian earthquakes, such as those occurred in L'Aquila (2009), Emilia-Romagna (2012), Central Italy (2016), and Ischia (2017). The evaluation of masonry seismic vulnerability is still an open issue, despite advanced research became popular in the last few years. Italy—as the country most reach in monumental churches and bell towers - has conceived ad-hoc Guidelines for the built heritage (DPCM, 2011), a code which provides dedicated methodologies mostly for churches, palaces and towers. Such methodologies are simplified by purpose, because they must be handled by common practitioners, which will produce quick assessments of the seismic vulnerability case by case. Whilst such Guidelines are probably one of the most advanced assessment method, they still exhibit important critical issues to be further improved or refined. Alternative approaches foresee large scale seismic vulnerability evaluation methods based on survey forms related to the main features of religious constructions and their damages suffered under last earthquakes (Criber et al., 2015; De Matteis et al., 2016; Formisano et al., 2017).
Limiting the discussion to churches, the ultimate load carrying capacity under horizontal loads, i.e., the ratio between horizontal failure acceleration and gravity acceleration, is estimated using the kinematic theorem of limit analysis on so called pre-assigned failure mechanisms, which are macro-blocks forming a kinematic chain. The material is assumed, on the safe side, as unable to withstand tensile stresses, so that internal power dissipation (which favors equilibrium) on cracks or yield lines is disregarded.
Since the actual failure mechanism activating is in principle unknown, an abacus of the most probable mechanisms as observed during past earthquakes is provided with exemplificative sketches on generic geometries. They are 28, all local, and the most common include façade and tympanum overturning, apse shear and rocking failure, triumphal arch four-hinges mechanisms, etc. (Casolo et al., 2000; Casolo and Uva, 2013; Casolo, 2017).
The repeated application of the kinematic theorem of limit analysis on the different mechanisms allowed to collect a database of normalized accelerations at collapse (ag/g) with their corresponding failure mechanism. The mechanism associated to the smallest collapse acceleration is that collapsing first with the highest probability. The classification of the most dangerous mechanisms is also important for an effective strengthening intervention; as a matter of fact, it is not sufficient to increase the load carrying capacity of the most critical mechanism, because it may happen that other local mechanisms, geometrically far from the previous one, exhibit load carrying capacities slightly larger. The resultant seismic improvement for the whole structure would be totally ineffective, because limited to the worst case without considering the other (almost) critical conditions.
Limit analysis on pre-assigned failure mechanisms appears therefore to the Authors rather appealing, because it can be applied immediately by users not familiar with structural analyses and earthquake engineering. However, there are also some limitations that one should point out, the most important being to limit the research of the active mechanism to only 28 different configurations. Designers are nowadays familiar with 3D geometric modelers integrating with Finite Element (FE) codes, a trend going exactly on the opposite direction. Limit analysis by Italian Guidelines requires at hand or semi-automatic calculations, or procedures that are still far to be fully automated, where the exact geometries and position of the loads in the church are unavoidably lost to simplify the approach.
The presence of complex clusters of arches, vaults and roofs cannot be handled manually if the problem is the passage between a 3D detailed model and limit analysis calculations. Then, the application of the gravity loads is done basically in an isostatic fashion, again disregarding the complex interaction in terms of thrust lines between vaults and columns at the intersection region. Typically, the collapse loads obtained with limit analysis result very conservative and very sensible to the assumptions done on the interlocking between perpendicular walls. However, to propose sophisticated nonlinear 3D FE approaches is not possible for common design studies (Betti and Vignoli, 2011; Milani and Venturini, 2011; Brando et al., 2015; Milani and Valente, 2015; Clementi et al., 2017; Giordano et al., 2019).
To assume masonry behaving as a no-tension material is certainly done on the safe side, but some important features of the masonry material are neglected, such as orthotropy, limited compressive strength and shear-normal stress interaction (Milani et al., 2006).
For towers, it is intuitive that the most accurate approach to deal with the analysis under horizontal loads should require specific ad-hoc FE approaches, because the extreme level of complexity necessitates a certain accuracy. Again however, to use non-linear methods and full 3D Finite Element models is quite uncommon, requires powerful and expensive FE codes and skilled users. Alternative effective investigations are provided by structural health monitoring analyses (Ubertini et al., 2017, 2018). Using the same philosophy adopted for churches, the Italian code for the built heritage (DPCM, 2011) allows to utilize a simplified model which considers the tower as a cantilever beam, where only flexural failure is possible. The advantage is again the utilization of a method that can be handled without a FE code and with no particular structural expertise, whereas the disadvantage is here the impossibility to account for a combined shear and flexural failure of the towers, which in practice is common in case of low slenderness (Milani et al., 2018). In the case of towers, this is a major limitation, because the risk is to identify possible zones of weakness on the base of a wrong failure mode, with the subsequent implementation of an ineffective strengthening intervention.
In this scenario, where Italian Guidelines push the research forward on simplified methods and the most diffused international trend is to use very sophisticated FE codes (Milani et al., 2011; Casolo et al., 2013; Acito et al., 2014; Valente and Milani, 2016; Marra et al., 2017; Sarhosis et al., 2018), a research lying in between such two extreme positions is needed.
In particular, in the present paper, the capabilities and limitations of a simplified modeling technique based on the “Equivalent Frame” are discussed on a historical masonry church severely damaged by an earthquake. The substitution of piers and spandrels with an equivalent frame made by non-linear beams proved to be effective also for historical buildings, at least in presence of generally regular walls, giving thus the possibility to all practitioners working in the safety assessment of historical buildings, to perform easily pushover analyses. However, also in case of regular constructions, it could be questionable to use this approach, regularly applied to framed structures, for masonry church characterized by massive piers and spandrels not comparable to slender columns and beams composing RC and steel frames. Moreover, the poor performances exhibited by historical and monumental masonry constructions during the last earthquakes required the use of proper upgrading and retrofitting interventions, whose effectiveness has been investigated by many researchers (Faggiano et al., 2009; Grande et al., 2011; Grande and Milani, 2016, 2018; Formisano and Marzo, 2017; Mosoarca et al., 2017; Formisano et al., 2018a, 2019), also with reference to large scale applications on whole historical centers (Brando et al., 2017; Rapone et al., 2018; Chieffo et al., 2019).
The meaningful case under study is the SS. Rosario church located in Finale Emilia with particular reference to its bell tower. The aim is to provide conclusions and recommendations that could hold also in different cases, using as base a simple numerical model that can be used by anyone. After the geometrical and mechanical characterization of the masonry complex, first the global seismic analysis of the bell tower by the 3Muri software is done. In particular, the behavioral differences between the isolate condition of the tower and the case when the whole neighboring complex is modeled, show that the presence of the aggregate has a beneficial effect on the tower. Finally, proper retrofitting interventions are designed and applied to the masonry bell tower, considered both as isolate or not; the different benefits deriving from these interventions in the two inspected cases are also emphasized.
The Emilia-Romagna Earthquake
On 2012 May 20th and 29th two earthquakes with local magnitude (ML) of 5.9 and 5.8 on the Richter scale, respectively, struck the Emilia-Romagna region of Italy. The first mainshock had epicenter between Mirandola and Finale Emilia, in the district of Modena, and hypocentre at about 6.3 km. It was followed by several aftershocks, also with magnitude greater than 5. The second mainshock had epicenter at Medolla, very close (about 10 Km west) to the first mainshock location, and hypocentre at about 10.2 km. Also this earthquake was followed by numerous aftershocks (http://ambiente.regione.emilia-romagna.it/en/geologia/temi/sismica/earthquake-20-may-2012).
The performed analyses (Galli et al., 2012) classified the largest shocks (seven with magnitude greater or equal than 5 in the period May-June 2012) as very strong with levels VII-VIII on the MCS intensity scale. The same intensity was also recorded in this area in 1346, in 1570 and in 1796 (Locati et al., 2011). In particular, the 2012 earthquakes had many analogies with the 1570 seismic event occurred in Ferrara.
From the geological point of view, the territory struck by the seismic sequence belongs to the Po Plain and is morphologically uniform, whereas the subsoil is rather more complex, with a series of geological structures, running parallel to the Apennines, which are seismically active.
Peak Ground Acceleration (PGA) values recorded during the 2012 earthquakes were rather high in some areas, in some cases also over 20% the gravity acceleration. Contrary, the ground accelerations expected on the basis of the Italian seismic hazard map were equal to about 0.15 g. This significant acceleration increase can be attributed to the subsoil local characteristics, leading to the so-called site effects connected to the lithological and geomorphologic features. In particular, loose, and poorly consolidated terrains (i.e. recent alluvial sediment, lacustrine and marine deposits, etc.) can modify the propagation of seismic waves upwards, amplifying the shaking extent and duration.
This amplification effect in the subsoil upper portion was also one of the causes of the liquefaction, that is the most striking environmental phenomenon observed during the Emilia-Romagna seismic sequence. The liquefaction is connected to different physical phenomena, such as cyclic mobility, cyclic and flow liquefactions, observed in saturated sandy deposits during strong earthquakes and extending in some cases for tens of meters. The phenomenon occurs when some conditions (uncemented and loose sand at a depth of less than15-20 m, depth of water table less than 15 m, size of sand grains from 0.02 to 2 mm and fine sediment content less than 15%) meet given earthquake properties (magnitude above 5.5, PGA equal to 15% of g and duration of shaking at least 15-20 s).
In the Po plain area the occurred strong earthquakes released the energy required to activate the liquefaction phenomenon. While in the past earthquakes liquefaction occurred away from built areas, during the 2012 seismic events the phenomenon involved several urbanized zones (especially S. Carlo, a hamlet of S. Agostino, and Mirabello, both in the district of Ferrara), causing extensive damages, such as rigid translation settlements of constructions, in some cases with a slight rotational component, and shear failure of joints, occurred in structurally weak buildings (garages, sheds, etc.), which were demolished after the earthquake.
The combined effect of high magnitude earthquakes with liquefaction phenomena gave rise to a long seismic sequence causing heavy damages and collapses (partial or total) especially to cultural heritage constructions, namely castles and towers, churches, bell towers and palaces. In the paper the case study of a masonry bell tower affected by the 2012 earthquake has been faced with the purpose of highlighting its seismic behavior as a part of an ecclesiastic complex.
The SS Rosario Ecclesiastic Complex
Knowledge Phase and Historical Information
The knowledge of historical masonry constructions is a fundamental prerequisite both for the purpose of a reliable seismic safety evaluation and the choice of effective upgrading or retrofitting interventions. The problems are those common to all the existing buildings even if, in the case of the cultural heritage to be protected, even more important is to know the original characteristics of the construction, the changes occurred during the time, the damage phenomena caused by anthropic transformations, the aging of materials and the occurred hazardous events. It is, therefore, necessary to refine analysis techniques and interpretation of historical artifacts through cognitive phases of different reliability degree also in relation to their impact. The knowledge can be achieved with different levels of deepening in terms of survey operations accuracy, historical researches and experimental investigations.
The study of the construction features is aimed at the definition of an interpretative model that allows for both a qualitative interpretation of the structural functioning and the structural analysis for a quantitative seismic evaluation. The degree reliability of this model will be closely related to the used deepening level and to the available data. From this point of view, different knowledge levels, namely limited, adequate and accurate according to increasing information levels, can be attained, they being linked to corresponding confidence factors [Ministerial Decree (NTC), 2008, 2018; Ministerial Circular (MC), 2009]. The knowledge path can be traced back to the following activities:
- Identification of the construction, its localization in relation to particular areas at risk and its relationship with the urban context. In particular, the analysis consists on the schematic survey of the artifact and the identification of any valuable elements (fixed decorative apparatus, movable artistic goods, etc.) that can affect the level of risk; geometric survey of the construction in the present state, including any cracks and deformation phenomena; relief of materials to completely identify the resistant structure of the artifact, keeping in mind their quality and preservation state.
- The evolution of the construction, understood as a sequence of transformation phases from the hypothetical original configuration to the present state;
- The identification of seismic-resistant elements, with particular attention to the erection techniques, to the constructive details and to the connections among elements;
- The recognition of materials, together with their state of decay and mechanical properties;
- Knowledge of the subsoil and of the foundation structures, also with reference to the changes and failures that took place during the time.
The knowledge phase activities above listed have been used to characterize the SS. Rosario ecclesiastic complex placed in Finale Emilia, that is the most eastern municipality of the province of Modena, in the corner between Andrea Costa street and Guglielmo Oberdan one (Figure 1A).
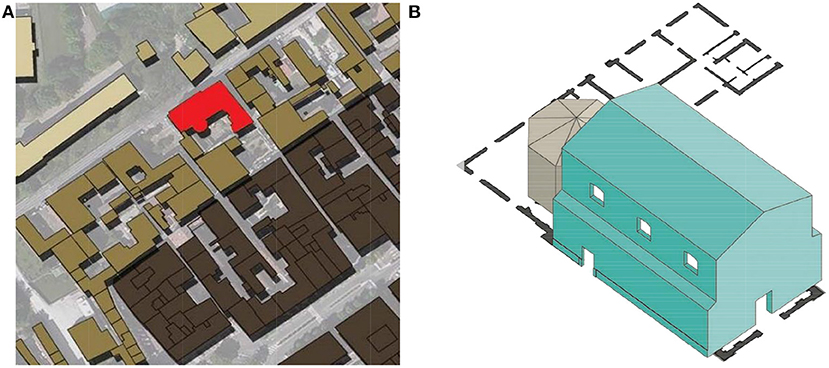
Figure 1. Bird-eye-view (highlighted in red) (A) and original configuration (B) of the SS. Rosario ecclesiastic complex in Finale Emilia.
The Church of the Rosary was built around 1580. The original structure, built on the ground of the old ditch of the northern walls, was originally smaller and simpler than the current one, which dated back to the second half of the seventeenth century, when behind the main altar there was probably also a sacristy compartment. A century later, the altar was set back and a compartment of octagonal shape, housing the sacristy, was created (Figure 1B). In 1646 the carved altars made of timber were manufactured and the ground was acquired to obtain a forecourt. Probably, in that time the church was enlarged. In the first decades of the nineteenth century, with the Napoleon's government, the church passed to the demesne and it was used as a barracks for the French soldiers under the orders of General Montrizard. Such a condition caused enormous damage to the building. With the fall of Napoleon and after the request of the bishop, the confreres of the Rosary resumed possession of their ancient seat, restored the Brotherhood and undertook to reopen the church, which was however deprived of many of its assets. In 1814 the sacristy modified its shape from octagonal to rectangular, also annexing the rectory and the stairs for access to the upper floor (Figure 2A). The bell tower was built only in 1856 and later on, in 1890, the façades were also transformed in the neoclassical style (Figure 2B). Starting from 1928, the church and the bell tower were restored. Damaged during the bombardments of the Second World War, the bell tower was again restored in 1955. In 1975 the building was closed to the faithful cult to eliminate the problems of humidity by means of both the total renovation of the roof and floor and the renovation of walls. Suspended and resumed several times during 90s, the restoration interventions ended in 1997.
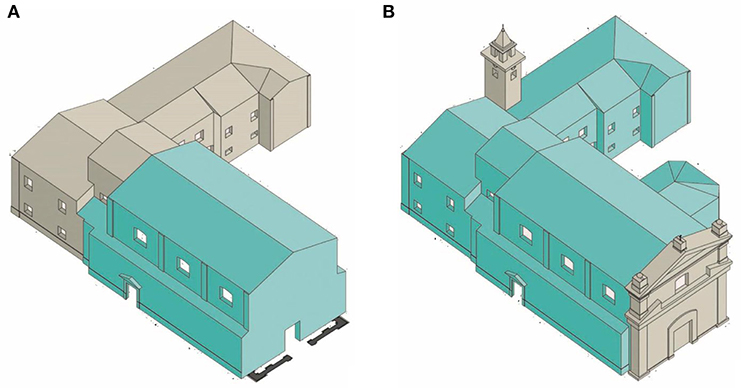
Figure 2. Transformed configuration dated back to the 1814 of the SS. Rosario church (A) and renovation intervention of the façade in 1890 (B).
Geometrical and Structural Surveys
The church can be considered as composed, both from the purely geometrical point of view and the structural one, of two in-plane rectangles. In fact, thinking to the different “stratifications” followed during the time, the construction can be divided into two structural bodies:
- part A, understood as the original body of the complex, containing the central nave and the altar;
- part B, considered as the most recent structure, containing the sacristy, the dormitory and the hospitalization.
The structural part A (Figure 3) has plan measures of 24.00 × 11.20 m, excluding ornamental elements, and develops on a maximum total height (at the ridge) of 16.50 m. The coverage is represented by a pitched roof made of timber beams with pushing actions. On the long side of the church there are numerous shelters in the masonry walls in order to host the altars and the chapels, as well as two openings, one on the street side and the other toward the inner courtyard.
The structural part B (Figure 4), instead, has plan dimensions of 26.50 × 7.30 m and develops on two levels, one at the ground floor with height of 3.70 m and the other at the first floor with height equal to 4.60 m. The total height at the ridge of this structural part is 10.70 m. On the facades there are several openings with arched shape. Vaulted floors, which in some cases are masked by false ceilings, represent the horizontal structures.
From the structural point of view, since walls are covered by plaster, it is not simple to evaluate, on the basis of a visual investigation only, the typology of the load-bearing vertical structure.
Nevertheless, from the historical analysis of the artifact, it is possible to assume that the major structural criticisms are relative to the poor connections among masonry walls in the zone between body A and body B, which were erected in different historical periods.
The vertical structures able to withstand loading actions are made of masonry bricks held together by lime mortar joints according to the constructive techniques of that erection time. In order to acquire these information so to eliminate some sources of initial uncertainty, it is usually made recourse to non-destructive indirect investigation techniques (thermography, georadar, sonic tomography, etc.) or weakly destructive direct inspections (endoscopies, peeling of plasters, essays, etc.) to be performed in significant points of the structure. In the case under study, unfortunately, since it has not been possible to carry out any kind of investigation tests, only a limited knowledge level has been attained and assumptions on the safe side both in terms of masonry mechanical properties and confidence factor have been used. Therefore, the knowledge level LC1 according to the provisions of the current Italian technical code [Ministerial Decree (NTC), 2018] has been taken into account. This knowledge status has been attained since survey of the ecclesiastic complex (church, sacristy and bell tower) has been done and some limited visual observations on the quality of masonry and effectiveness of connections among walls have been carried out. As a consequence, the minimum levels of stresses and the average elastic modules for brick masonry deduced from the table reported in the Ministerial Circular 2 February 2009 [Ministerial Circular (MC), 2009], an explicative code of the NTC 2008 standard, have been considered.
No specific geological and geotechnical tests have been performed to know in detail the subsoil stratigraphy and the physical-mechanical characteristics of the soil. However, from a significant number of geotechnical investigations in areas close to the church one, it has been possible to identify from the seismic point of view, according to the NTC 2008, a soil of type D, that is “deposits of coarse-grained soils, sparsely thickly or poorly sizeable fine grained soils, with thicknesses exceeding 30 m, characterized by a gradual improvement of the mechanical properties with the depth”.
Seismic Vulnerability Assessment
Foreword
Seismic vulnerability analyses have been performed by using the 3Muri software, which uses the well-known Frame-by-Macro-Element (FME) approach to model masonry walls (S.T.A.DATA srl, 2018). In this software piers and spandrels are modeled as deformable macro-elements, while the nodes between vertical elements and horizontal ones are considered as rigid parts, considering that they exhibited very few damages under earthquakes. After the whole macro-elements structural model is setup, it is transformed into the classic Equivalent Frame Model (EFM), used to seismically analyse framed structures. On this EFM, dynamic linear and static non-linear analysis are carried out in the two main analysis directions aiming at evaluating the probable seismic damages.
In the case under study, the bell tower has been modeled as isolate structure and, subsequently, as a part of the ecclesiastic complex in order to evaluate the behavioral differences under seismic actions in the non-linear static field between the two modeling approaches. Based on the vulnerability assessment analysis results, proper seismic upgrading and retrofitting interventions have been considered and applied to both analysis models in order to increase the seismic performance of the inspected bell tower.
The Isolate Bell Tower
Initially, the masonry bell tower has been modeled as isolate structure. Geometrical and mechanical features of the structure have been taken according to the information achieved from the historical-critical analysis of the artifact. The geometrical layout and the FME model of this structure modeled with the 3Muri software are shown in Figure 5. Seismic actions have been taken according to the response spectra given by $$NTC 2008 at the Collapse, Life Safety, Operational and Damage Limit States for constructions of class II (use coefficient of 1.0) placed in Finale Emilia (district of Modena), founded on a soil type D with topographic class T1 (amplification coefficient equal to 1) and having nominal life of 50 years. The fundamental parameters used to characterize the elastic spectra at different limit states are depicted in Table 1.
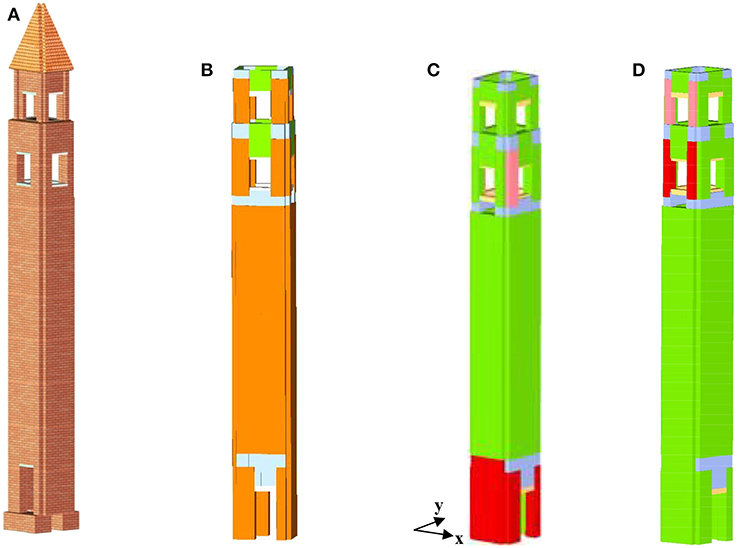
Figure 5. The isolate tower: architectural layout (A), FME model (B) and deformed shapes (pink: bending-compression plastic; red: bending-compression failure) from pushover analyses in the directions x (C) and y (D).
24 pushover analyses, different for analysis direction (x and y), loading type (proportional either to masses or to the first vibration mode) and eccentricities (positive and negative) between centroid and stiffness center, have been performed (Table 2). The pushover analysis results showed that, since the seismic risk coefficients α are always less than 1, all seismic checks are not satisfied. The worst results are related to the analyses n. 12 and n. 24 in directions x and y, respectively, which are highlighted with bold text in Table 2. The final deformed shapes of the masonry bell tower at the end of these pushover analyses are plotted in Figures 5C,D, where it is apparent that failure mechanisms are concentrated at the ground level in direction x and at the penultimate level in direction y. The MDOF pushover curves related to the main analysis directions are plotted in Figure 6. In Table 3 the seismic verifications in terms of displacements, leading to the so-called Vulnerability Index (VI), are reported. In this table Dmax and Du are the seismic demand displacement and the capacity one, respectively, while q* is the ratio between the elastic response resistance and the yielding resistance of the SDOF system. Also, comparing the results in terms of displacements, it is achieved that the bell tower is not able to resist the standard seismic actions and that the worst result is obtained in the direction y.
The Aggregate Bell Tower
The seismic behavior of the bell tower has been also investigated when it is included in the aggregate of constructions given by the ecclesiastic complex, whose 3D geometrical and macro-element models are depicted in Figure 7.
In this case the pushover curves of the bell tower have been reconstructed starting from those of the ecclesiastic complex by monitoring step-by-step both the base shear of own masonry walls (considering the influence of loads transmitted by the adjacent parts of the church) and the displacements of the top level centroid. For the sake of comparison, the MDOF bi-linear pushover curve of the bell tower related to the analysis n. 24 in direction y, which corresponds the worst result to, has been plotted and compared to that of the isolate structure, as reported in Figure 8A.
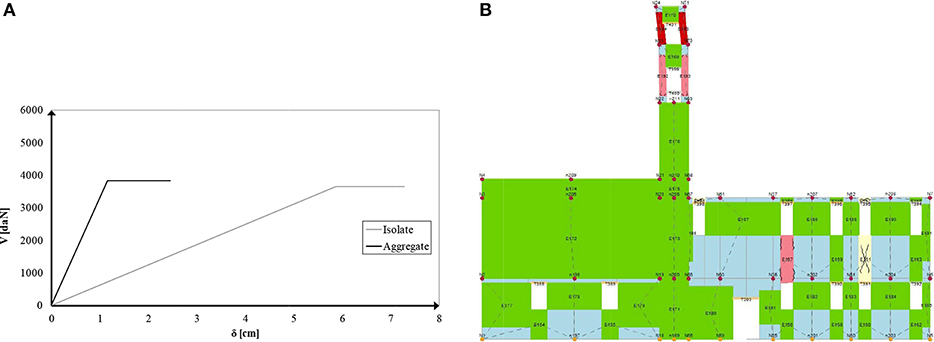
Figure 8. Comparison between bi-linear pushover curves in direction y (analysis n. 24) of the bell tower in the two structural configurations considered (A) and deformed shape of the church at the end of this pushing phase (B).
The final deformation of the structure corresponding to this analysis is shown in Figure 8B, where it is noticed that, differently from the isolate case, the bending-compression failure of the top level occurs.
From the comparison with the isolate condition it appears that, when the bell tower is inserted in the building aggregate, the base shear is basically unaltered, while the stiffness and ultimate displacement are reduced of about 80 and 65%, respectively.
Moreover, the comparison among the isolate case and the aggregate one has been performed in the ADRS format, providing the capacity curves of Figure 9. The vulnerability index, intended as the ratio between the demand displacement and the capacity one, assumes values of about 4.03 and 3.15 in the cases of the isolate tower and of the aggregate one, respectively. This means that the aggregate condition reduces the seismic vulnerability of more than 20%.
Anti-Seismic Interventions
The insufficient results deriving from seismic checks have required interventions on the masonry bell tower with the purpose to be economic and easy to be implemented. In the case under study, two different interventions based on the use of either glass fibers sheets, having acronym of G-FRP, or reinforced plaster have been foreseen. In both cases they have been applied at the two last levels together with the confinement of existing openings with portals made of steel profiles. All the above interventions have been applied both to the isolate tower and to the aggregate one. In particular, two layers of glass fibers sheets, having thickness of 0.035 mm, mesh of 25 × 25 mm, elastic modulus of 72 GPa and ultimate strain of 1.8%, have been used as jacketing system of the inadequate masonry walls. These sheets have been applied on both sides of the walls between two layers of mortars and they have been connected to each other by means of appropriate glass fibers connectors. On the other hand, reinforced plaster has been based on the use of two reinforced concrete jacketing walls armed with ϕ12 bars made of B450C steel arranged in meshes of 50 × 50 cm. The two reinforced walls have been connected to each other by means of ϕ12 steel bars placed each 50 cm in horizontal and vertical directions.
The application of the above mentioned reinforcing interventions has provided the capacity curves in the ADRS format of Figure 10, where the comparison with the seismic response of the isolate tower has been also reported.
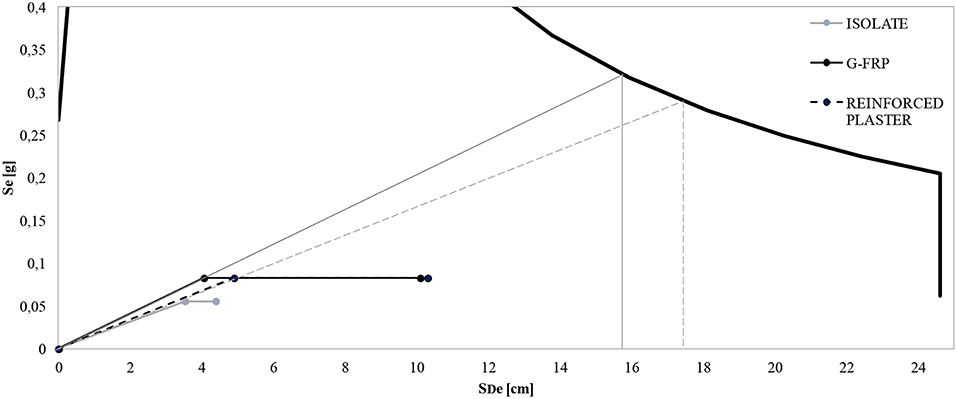
Figure 10. Comparison between capacity curves of the isolate bell tower in the ADRS format before and after retrofitting interventions.
From the comparison it has been achieved that the vulnerability index passes from 4.03 (isolate tower) to about 1.55 and 1.70 in the cases of interventions with G-FRP and reinforced plaster, respectively. This means that in both cases the seismic upgrading of the bell tower is achieved. Nevertheless, from the seismic point of view, intervention with G-FRP is slightly preferable to that with reinforced plaster. In fact, in the former case a reduction of seismic vulnerability of about 62% is attained, whereas in the latter one the vulnerability decrease is about 58%. Contrary, a strong preference toward G-FRP is recognized in terms of Life Cycle Assessment, since glass fibers have environmental impact lower than that of plasters reinforced with steel bars.
Finally, the same interventions above described have been applied to the case of the aggregate bell tower and the comparison among capacity curves has been done, as depicted in Figure 11.
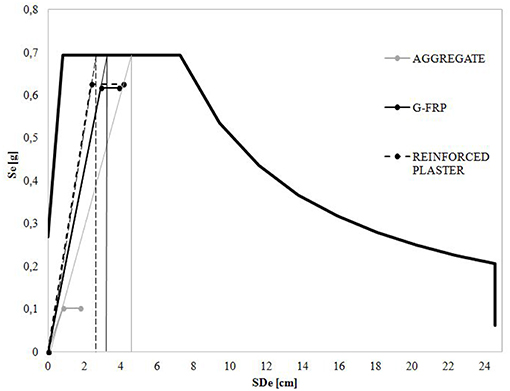
Figure 11. Comparison between capacity curves of the aggregate bell tower in the ADRS format before and after retrofitting interventions.
From the analysis results it is obtained that the vulnerability index is changed from 3.15 (aggregate condition) to 0.85 and 0.65 in case of interventions with G-FRP and reinforced plaster, respectively. This means that, since vulnerability indexes are lower than one, both considered interventions are able to retrofit seismically the bell tower. In addition, vulnerability indexes provided by interventions with G-FRP and reinforced plaster are about 27 and 21% of the index achieved on the aggregate tower, respectively. Finally, even if more impacting from the environmental point of view, reinforced plaster represents the best intervention, since it provides the lowest vulnerability index and it is less expensive than G-FRP.
Concluding Remarks
The current paper has estimated the seismic vulnerability of a masonry bell tower located in the municipality of Finale Emilia, in the district of Modena (Italy), hit by the 2012 Emilia-Romagna earthquake. In particular, the difference of behavior between the isolate case and the aggregate one, the latter when the bell tower is inserted in the constructions compound, has been evaluated. From analysis results it has been seen that, in both cases, the seismic checks are not satisfied. The comparison of results in terms of pushover curve has shown that, when the tower is in aggregate, the stiffness and ultimate displacement are reduced of about 80% and 65%, respectively, with respect to the case of the isolate structure. Besides, the aggregate condition reduces the tower seismic vulnerability of more than 20%. In the two analysis cases the same compression-bending damage mechanisms have been detected, but at different levels, i.e. the penultimate level for the isolate tower and the top level for the aggregate one.
Considering the bad seismic performance of the tower, two different interventions based on the use of either glass fibers (G-FRP) sheets or reinforced plaster have been foreseen. In both cases they have been applied at the two last levels together with the confinement of existing openings with portals made of steel profiles. These interventions have been considered both in the isolate case and in the aggregate one. From numerical analyses, it has been shown that both interventions are able to seismically upgrade the bell tower. When the isolate tower is taken into account, intervention with G-FRP, which gives a reduction of seismic vulnerability of about 62%, is slightly preferable to that with reinforced plaster, which corresponds a vulnerability decrease of about 58% to. Moreover, G-FRP is strongly preferred to reinforced plaster if Life Cycle Assessment issues are of concern, since the former technique has a lower impact on the environment.
Contrary, the same interventions applied to the aggregate tower have lead toward the seismic retrofitting of the structure, since the achieved vulnerability indexes are lower than one. In particular, vulnerability indexes provided by interventions with G-FRP and reinforced plaster are about 27 and 21%, respectively, of the index achieved on the basic aggregate tower. As conclusion, reinforced plaster intervention, while having a higher environmental impact, has represented the best retrofitting solution, since it has provided the lowest vulnerability index, allowing also to save money with respect to applications with G-FRP.
However, the results herein presented are related to the case study examined only and, therefore, in order to generalize the obtained outcomes, parametric analyses on other masonry bell towers with different geometrical and mechanical features should be performed as a further development of the current research.
Data Availability
All datasets generated for this study are included in the manuscript and/or the supplementary files.
Author Contributions
AF setup the methodology and elaborated the paper draft. GM revised the paper.
Conflict of Interest Statement
The authors declare that the research was conducted in the absence of any commercial or financial relationships that could be construed as a potential conflict of interest.
References
Acito, M., Bocciarelli, M., Chesi, C., and Milani, G. (2014). Collapse of the clock tower in Finale Emilia after the May 2012 Emilia Romagna earthquake sequence: numerical insight. Eng. Struct. 72, 70–91. doi: 10.1016/j.engstruct.2014.04.026
Betti, M., and Vignoli, A. (2011). Numerical assessment of the static and seismic behaviour of the basilica of Santa Maria all'Impruneta (Italy). Constr. Build. Mater. 25, 4308–4324. doi: 10.1016/j.conbuildmat.2010.12.028
Brando, G., Criber, E., and De Matteis, G. (2015). The effects of L'Aquila earthquake on the St. Gemma church in Goriano Sicoli: part II - fem analysis. Bull. Earthquake Eng. 13, 3733–3748. doi: 10.1007/s10518-015-9793-3
Brando, G., De Matteis, G., and Spacone, E. (2017). Predictive model for the seismic vulnerability assessment of small historic centres: application to the inner Abruzzi Region in Italy. Eng. Struct. 153, 81–96. doi: 10.1016/j.engstruct.2017.10.013
Brandonisio, G., Lucibello, G., Mele, E., and De Luca, A. (2013). Damage and performance evaluation of masonry churches in the 2009 L'Aquila earthquake. Eng. Fail. Anal. 34, 693–714. doi: 10.1016/j.engfailanal.2013.01.021
Casolo, S. (2017). A numerical study on the cumulative out-of-plane damage to church masonry façades due to a sequence of strong ground motions. Earthquake Eng. Struct. Dyn. 46, 2717–2737. doi: 10.1002/eqe.2927
Casolo, S., Milani, G., Uva, G., and Alessandri, C. (2013). Comparative seismic vulnerability analysis on ten masonry towers in the coastal Po Valley in Italy. Eng. Struct. 49, 465–490. doi: 10.1016/j.engstruct.2012.11.033
Casolo, S., Neumair, S., Parisi, M. A., and Petrini, V. (2000). Analysis of seismic damage patterns in old masonry church facades. Earthquake Spectra 16, 757–773. doi: 10.1193/1.1586138
Casolo, S., and Uva, G. (2013). Nonlinear analysis of out-of-plane masonry façades: full dynamic versus pushover methods by rigid body and spring model. Earthquake Eng. Struct. Dyn. 42, 499–521. doi: 10.1002/eqe.2224
Chieffo, N., Formisano, A., and Miguel Ferreira, T. (2019). Damage scenario-based approach and retrofitting strategies for seismic risk mitigation: an application to the historical Centre of Sant'Antimo (Italy). Eur. J. Environ. Civ. Eng. doi: 10.1080/19648189.2019.1596164. [Epub ahead of print].
Clementi, F., Quagliarini, E., Monni, F., Giordano, E., and Lenci, S. (2017). Cultural heritage and earthquake: the case study of “Santa Maria della Carità” in Ascoli Piceno. Open Civ. Eng. J. 11, 1079–1105. doi: 10.2174/1874149501711011079
Criber, E., Brando, G., and De Matteis, G. (2015). The effects of L'Aquila earthquake on the St. Gemma church in Goriano Sicoli: part I - damage survey and kinematic analysis. Bull. Earthquake Eng. 13, 3713–3732. doi: 10.1007/s10518-015-9792-4
D'Amato, M., Laterza, M., and Diaz Fuentes, D. (2018). Simplified seismic analyses of ancient churches in Matera's landscape. Int. J. Archit. Herit. 1–20. doi: 10.1080/15583058.2018.1511000. [Epub ahead of print].
De Matteis, G., Criber, E., and Brando, G. (2016). Damage probability matrices for Three-Nave Masonry Churches in Abruzzi after the 2009 L'Aquila Earthquake. Int. J. Archit. Herit. 10, 120–145. doi: 10.1080/15583058.2015.1113340
DPCM 9/2/2011 (2011). Italian Guidelines for The Evaluation and The Reduction of The Seismic Risk for The Built Heritage With Reference to The Italian Norm of Constructions. Rome: Decree of the Ministries Council Presidency.
Faggiano, B., Marzo, A., Formisano, A., and Mazzolani, F. M. (2009). Innovative steel connections for the retrofit of timber floors in ancient buildings: a numerical investigation. Comput. Struct. 87, 1–13. doi: 10.1016/j.compstruc.2008.07.005
Formisano, A., Ciccone, G., and Mele, A. (2017). “Large scale seismic vulnerability and risk evaluation of a masonry churches sample in the historical centre of Naples,” in AIP Conference Proceedings (Thessaloniki), 1906. doi: 10.1063/1.5012360
Formisano, A., Di Lorenzo, G., Landolfo, R., and Mazzolani, F. M. (2018a). Seismic-volcanic vulnerability and retrofitting of a cultural heritage masonry palace in the Vesuvius area. Int. J. Masonry Res. Innov. 3, 244–268. doi: 10.1504/IJMRI.2018.093485
Formisano, A., and Marzo, A. (2017). Simplified and refined methods for seismic vulnerability assessment and retrofitting of an Italian cultural heritage masonry building. Comput. Struct. 180, 13–26. doi: 10.1016/j.compstruc.2016.07.005
Formisano, A., Vaiano, G., and Fabbrocino, F. (2019). Seismic and energetic interventions on a typical south Italy residential building: cost analysis and tax detraction. Front. Built Environ. 5:12. doi: 10.3389/fbuil.2019.00012
Formisano, A., Vaiano, G., Fabbrocino, F., and Milani, G. (2018b). Seismic vulnerability of Italian masonry churches: the case of the Nativity of Blessed Virgin Mary in Stellata of Bondeno. J. Build. Eng. 20, 179–200. doi: 10.1016/j.jobe.2018.07.017
Galli, P., Castenetto, S., and Peronace, E. (2012). The MCS macroseismic survey of the Emilia 2012 earthquakes. Ann. Geophys. 55, 663–672. doi: 10.4401/ag-6163
Giordano, E., Clementi, F., Nespeca, A., and Lenci, S. (2019). Damage assessment by numerical modelling of Sant'Agostino's sanctuary in Offida during the central Italy 2016-2017 seismic sequence. Front. Built Environ. 4:87. doi: 10.3389/fbuil.2018.00087
Grande, E., Imbimbo, M., and Sacco, E. (2011). Bond behaviour of CFRP laminates glued on clay bricks: experimental and numerical study. Compos. B 42, 330–340. doi: 10.1016/j.compositesb.2010.09.020
Grande, E., and Milani, G. (2016). Modeling of FRP-strengthened curved masonry specimens and proposal of a simple design formula. Compos. Struct. 158, 281–290. doi: 10.1016/j.compstruct.2016.09.017
Grande, E., and Milani, G. (2018). Interface modeling approach for the study of the bond behavior of FRCM strengthening systems. Compos. B 141, 221–233. doi: 10.1016/j.compositesb.2017.12.052
Krstevska, L., Tashkov, L., Naumovski, N., Florio, G., Formisano, A., Fornaro, A., et al. (2010). “In-situ experimental testing of four historical buildings damaged during the 2009 L'Aquila earthquake,” in COST ACTION C26: Urban Habitat Constructions Under Catastrophic Events - Proceedings of the Final Conference (Naples), 427–432.
Lagomarsino, S. (2012). Damage assessment of churches after L'Aquila earthquake (2009). Bull. Earthquake Eng. 10, 73–92. doi: 10.1007/s10518-011-9307-x
Locati, M., Camassi, R., and Stucchi, M. (eds). (2011). DBMI11, Italian Macroseismic Database, 2011 Version. Milano and Bologna. Available online at: http://emidius.mi.ingv.it/DBMI11
Marra, A. M., Salvatori, L., Spinelli, P., and Bartoli, G. (2017). Incremental dynamic and nonlinear static analyses for seismic assessment of Medieval Masonry Towers. ASCE J. Perf. Constr. Facil. 31, 1–10. doi: 10.1061/(ASCE)CF.1943-5509.0001022
Milani, G., Casolo, S., Naliato, A., and Tralli, A. (2011). Seismic assessment of a medieval masonry tower in northern Italy by limit, nonlinear static, and full dynamic analyses. Int. J. Archit. Herit. 6, 37–41. doi: 10.1080/15583058.2011.588987
Milani, G., Lourenço, P. B., and Tralli, A. (2006). Homogenised limit analysis of masonry walls, Part I: failure surfaces. Comput. Struct. 84, 166–180. doi: 10.1016/j.compstruc.2005.09.005
Milani, G., Shehu, R., and Valente, M. (2018). A kinematic limit analysis approach for seismic retrofitting of masonry towers through steel tie-rods. Eng. Struct. 160, 212–228. doi: 10.1016/j.engstruct.2018.01.033
Milani, G., and Valente, M. (2015). Comparative pushover and limit analyses on seven masonry churches damaged by the 2012 Emilia-Romagna (Italy) seismic events: possibilities of non-linear finite elements compared with pre-assigned failure mechanisms. Eng. Fail. Anal. 47, 129–161. doi: 10.1016/j.engfailanal.2014.09.016
Milani, G., and Venturini, G. (2011). Automatic fragility curve evaluation of masonry churches accounting for partial collapses by means of 3D FE homogenized limit analysis. Comput. Struct. 89, 1628–1648. doi: 10.1016/j.compstruc.2011.04.014
Ministerial Circular (MC) (2009). Instructions for the Application of the ≪New Technical Standards for Buildings≫ Referred to in the Ministerial Decree of 14 January 2008 (in Italian). Official Gazette n.47 of 26/02/2009, Ordinary Supplement n.27, Rome.
Ministerial Decree (NTC) (2008). New Technical Codes for Constructions (in Italian). Official Gazette n. 29 of 04/02/08, Ordinary Supplement n. 30, Rome.
Ministerial Decree (NTC) (2018). Updating of Technical Codes for Constructions (in Italian). Rome: Official Gazette n. 42 of 20/02/18, Ordinary Supplement n. 8.
Mosoarca, M., Apostol, I., Keller, A., and Formisano, A. (2017). Consolidation methods of Romanian historical building with composite materials. Key Eng. Mater. 747, 406–413. doi: 10.4028/www.scientific.net/KEM.747.406
Rapone, D., Brando, G., Spacone, E., and De Matteis, G. (2018). Seismic vulnerability assessment of historic centers: description of a predictive method and application to the case study of Scanno (Abruzzi, Italy). Int. J. Archit. Herit. 12, 1171–1195. doi: 10.1080/15583058.2018.1503373
Sarhosis, V., Milani, G., Formisano, A., and Fabbrocino, F. (2018). Evaluation of different approaches for the estimation of the seismic vulnerability of masonry towers. Bull. Earthquake Eng. 16, 1511–1545. doi: 10.1007/s10518-017-0258-8
Tashkov, L., Krstevska, L., Naumovski, N., De Matteis, G., and Brando, G. (2010). “Ambient vibration tests on three religious buildings in Goriano Sicoli damaged during the 2009 L'Aquila earthquake,” in COST ACTION C26: Urban Habitat Constructions under Catastrophic Events - Proceedings of the Final Conference, 427-432; 433–438.
Ubertini, F., Cavalagli, N., Kita, A., and Comanducci, G. (2018). Assessment of a monumental masonry bell-tower after 2016 Central Italy seismic sequence by long-term SHM. Bull. Earthquake Eng. 16, 775–801. doi: 10.1007/s10518-017-0222-7
Ubertini, F., Comanducci, G., Cavalagli, N., Pisello, A. L., Materazzi, A. L., and Cotana, F. (2017). Environmental effects on natural frequencies of the San Pietro bell tower in Perugia, Italy, and their removal for structural performance assessment. Mech. Syst. Signal Process. 82, 307–322. doi: 10.1016/j.ymssp.2016.05.025
Keywords: masonry church, masonry bell tower, Emilia-Romagna earthquake, aggregate condition, collapse mechanisms, non-linear static analyses, upgrading and retrofitting interventions
Citation: Formisano A and Milani G (2019) Seismic Vulnerability Analysis and Retrofitting of the SS. Rosario Church Bell Tower in Finale Emilia (Modena, Italy). Front. Built Environ. 5:70. doi: 10.3389/fbuil.2019.00070
Received: 20 March 2019; Accepted: 09 May 2019;
Published: 28 May 2019.
Edited by:
Massimo Latour, University of Salerno, ItalyReviewed by:
Ali Koçak, Yildiz Technical University, TurkeyGiuseppe Brando, Università degli Studi G. d'Annunzio Chieti e Pescara, Italy
Ernesto Grande, Università degli Studi Guglielmo Marconi, Italy
Copyright © 2019 Formisano and Milani. This is an open-access article distributed under the terms of the Creative Commons Attribution License (CC BY). The use, distribution or reproduction in other forums is permitted, provided the original author(s) and the copyright owner(s) are credited and that the original publication in this journal is cited, in accordance with accepted academic practice. No use, distribution or reproduction is permitted which does not comply with these terms.
*Correspondence: Antonio Formisano, YW50b2Zvcm1AdW5pbmEuaXQ=