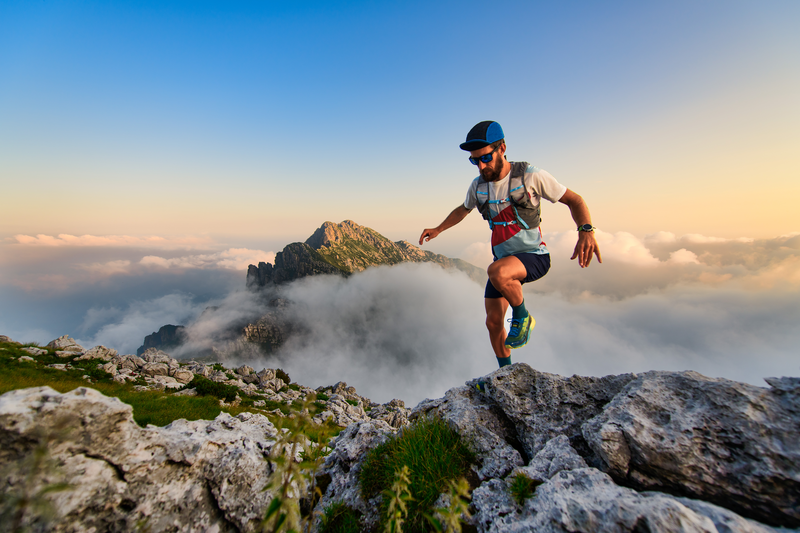
94% of researchers rate our articles as excellent or good
Learn more about the work of our research integrity team to safeguard the quality of each article we publish.
Find out more
HYPOTHESIS AND THEORY article
Front. Blockchain
Sec. Blockchain Technologies
Volume 8 - 2025 | doi: 10.3389/fbloc.2025.1544770
The final, formatted version of the article will be published soon.
You have multiple emails registered with Frontiers:
Please enter your email address:
If you already have an account, please login
You don't have a Frontiers account ? You can register here
Blockchain technology has caused a significant transformation in the global energy sector as it is increasingly applied in producing, distributing, trading, and managing energy. The incorporation of blockchain in the industry presents unprecedented opportunities for creating secure and decentralized systems of trading energy systems that are not only secure and resilient but also transparent.The paper explores a detailed analysis of various blockchain platforms and endeavors to collapse the existing gaps in the advanced research of blockchain systems supporting the development of energy trading applications. Precisely, this paper gives in-depth details of some of the popular blockchain platforms, and it primarily focuses on the platforms' security, scalability solutions, consensus methods, strategies for mitigating cyberattacks, privacy-preserving mechanisms, regulatory considerations, the integration of artificial intelligence for platform optimization and suitability in energy trading based on the existing information. It helps energy providers select the best blockchain platform for their energy trading projects. The detailed examination aims to further improve energy trading efficiency, reliability, and sustainability via the most suitable blockchain platform.
Keywords: Blockchain, Energy sector, blockchain platforms, Scalability, cyberattacks, Privacy-preserving mechanisms, Regulations, artificial intelligence
Received: 13 Dec 2024; Accepted: 24 Feb 2025.
Copyright: © 2025 Jayavarma, P K and Nair. This is an open-access article distributed under the terms of the Creative Commons Attribution License (CC BY). The use, distribution or reproduction in other forums is permitted, provided the original author(s) or licensor are credited and that the original publication in this journal is cited, in accordance with accepted academic practice. No use, distribution or reproduction is permitted which does not comply with these terms.
* Correspondence:
Preetha P K, Amrita Vishwa Vidyapeetham (Amritapuri Campus), Kollam, India
Disclaimer: All claims expressed in this article are solely those of the authors and do not necessarily represent those of their affiliated organizations, or those of the publisher, the editors and the reviewers. Any product that may be evaluated in this article or claim that may be made by its manufacturer is not guaranteed or endorsed by the publisher.
Research integrity at Frontiers
Learn more about the work of our research integrity team to safeguard the quality of each article we publish.