- 1Department of Biology, Indiana University, Bloomington, IN, United States
- 2Department of Fisheries, Wildlife, and Conservation Sciences, Oregon State University, Corvallis, OR, United States
Many changes in species’ geographic distributions have been attributed to recent climate warming. One understudied phenomenon is the effect of climate change on differential migrants, species in which the sexes differ in distance migrated to and from the breeding range. We evaluated the impact of climate change on differential migration in the dark-eyed junco (Junco hyemalis) by assessing temporal and geographic changes in overall population abundance throughout the winter range over the past 60 years. We also compared the abundance of females in two wintering populations studied 15 years ago with historical abundances studied 45 years ago We observed a northward movement of the population as a whole and an increase in female abundance at higher latitudes that correlated with recent changes in winter climate. These findings suggest that climate change has reduced distance migrated in this species and increased the proportion of females wintering at higher latitudes, providing new insights into the impact of climate warming on migratory distance and winter distributions.
Introduction
Over the past 100 years, the earth’s temperature has increased on average 1.0° C and shown a particularly sharp increase since 1980 (NOAA, https://www.climate.gov/news-features/understanding-climate/climate-change-global-temperature), resulting in long-term, large-scale alterations in phenology, distribution, and population dynamics of animal and plant species (Burakowski et al., 2008; Tingley and Beissinger, 2009; Walther, 2010; Chen et al., 2011; Heath et al., 2012; Hurlbert and Liang, 2012). While numerous studies have documented climate-associated distributional changes (Rushing et al., 2020), none to our knowledge has considered the effects of climate change on differential migrants (i.e., species that segregate geographically on a seasonal basis based on sex differences in migratory distance traveled) (Ketterson and Nolan, 1983; Ruckstuhl, 2007). For differential migrants, which include many species of bats, whales, ungulates, and birds (Ketterson and Nolan, 1983; Cristol et al., 1999; Flemming and Eby, 2003; Ruckstuhl and Neuhaus, 2005), changes in distribution may be especially important. For example, changes in suitability in only a portion of the winter range might affect the operational sex ratio on the breeding range and have implications for management (Briedis and Bauer, 2018).
Long-standing hypotheses to explain the phenomenon of differential migration are closely linked to climate, and potentially, to climate change, including sex differences in the ability to withstand cold climates, intersexual competition for winter resources, and intrasexual competition for breeding resources (Ketterson and Nolan, 1983; Cristol et al., 1999; Catry et al., 2004; Ruckstuhl, 2007; Morbey and Ydenberg, 2008). If, for example, one sex is less able to withstand a cold climate, then a warming climate may allow for reduced sex-related differences in distance migrated, leading to reduced geographic variation in winter sex ratios.
Alternatively, if the distance migrated by the species is affected by climate but the sex difference in distance migrated is not associated with sex differences in the ability to withstand cold climates, then we might predict northward movement of the distribution in response to warming, but no change in the degree of sexual segregation.
Traditional reports of differential migration were often based on biased sex ratios observed in museum collections, among ring recoveries, or in populations sampled in the wild (for recent examples see (Catry et al., 2004; Snell et al., 2021). Because a strongly skewed sex ratio in a portion of the range with low abundance could be misleading, cases of differential migration must consider geographic variation in both sex ratio and abundance.
Modern day observations of differential migration are more likely to be based on individual tracking data or patterns of variation in stable isotopes (Gow and Wiebe, 2014; Fayet et al., 2016; Woodworth et al., 2016; Deakin et al., 2019; Carbeck et al., 2022). [For a case of no sex difference see (Pedersen et al., 2019), for cases of longer migrations by males see (Gow and Wiebe, 2014; Hedh and Hedenstrom, 2020)]. However, these newer techniques do not allow for comparisons with past population structure or past migratory behavior.
We investigated the impact of climate change on alterations in the migratory distribution of male and female dark-eyed juncos (J. hyemalis), a songbird that is a differential migrant. Historical field and museum data from eastern North America have shown that female juncos winter farther from the breeding range and at more southerly latitudes than males, and that this pattern of sexual segregation is highly correlated with winter climate (Ketterson and Nolan, 1976, 1979, 1983).
To assess whether the junco’s winter distribution has moved northward owing to climate warming we measured changes in abundances by latitude using Christmas Bird Counts. We anticipated an increase in density at northern sites that lie closer to the breeding range. To assess whether sex-related changes in junco migration have taken place, we compared the abundances of female juncos at two sites sampled in the mid-1970s (Ketterson and Nolan, 1983) with abundances from 2004–2009. As one of several possibilities, we anticipated that because the junco is a ground-feeding bird, declines in snow cover or colder temperatures at northern latitudes may have reduced the cost to females of over wintering at northern latitudes (Walther et al., 2002). Therefore, we predicted more equal numbers of males and females at northern sampling sites, than was true historically, and that any changes in sex ratios would be correlated with recent climate.
Methods
Study species
The dark-eyed junco is a songbird species whose non-breeding behaviors, migration schedules, and population structure throughout its winter range have been extensively studied (Ketterson and Nolan, 1976, 1982, 1983; Rogers et al., 1989; Nolan and Ketterson, 1990). The junco’s winter range in eastern North America extends from northern United States (US) and extreme southeast of Canada to US and portions of northern Mexico (Nolan et al. 2002).
Capture methods
Historical data (1977–1980) in this study were previously reported in Ketterson and Nolan (1983). Juncos were captured during two time periods: 1977–1980 (historical) and 2004–2009 (recent). Recent data were collected during the years 2004–2009, when a total of 748 dark-eyed juncos were captured using a combination of baited mist nets and potter traps between December 1st (the cessation of autumn migration) and March 1st (the onset of spring migration) at sites previously sampled by Ketterson and Nolan in the mid-1970s, including Kalamazoo, Michigan (42.29°N–85.58°W, n=190) and Bloomington, Indiana (39.16°N–86.49°W, n=558) (Ketterson and Nolan, 1976; Nolan and Ketterson, 1990).
To minimize the possibility of sex-biased capture, we employed methods prescribed by (Ralph et al. (2004) and Dunn and Ralph (Dunn and Ralph, 2004) that included constant mist netting efforts (daily capture from sunrise to noon), multiple capture sites >1km apart within locations, and multiple nets per site covering several habitat types. Historical data were collected from 1977–1980 using similar methods and rationale (Ketterson and Nolan, 1976, 1983). For historical data, juncos were sexed using a combination of morphological measurement and laparotomies to achieve >95% accuracy in determining sex (Ketterson and Nolan, 1976). For more recent data, we assessed sex through morphological methods as outlined in Ketterson and Nolan (Ketterson and Nolan, 1976) and molecular methods (described in detail below) to achieve 100% accuracy. Thus, for more recent data, upon capture we collected 10ul of blood. We also collected wing chord measurements (to nearest 0.5mm) and plumage characteristics to determine sex, which was corroborated or corrected using molecular sexing (Ketterson and Nolan, 1976). All capture methods and procedures used in this study were approved by the Bloomington Institutional Animal Care and Use Committee (Study# 06-242), U.S. Fish and Wildlife Bird Banding Laboratory (Permit #20261), and the Department of Natural Resources in Michigan (Permit # SC1353) and Indiana (Permit # 08-0006).
Molecular sexing
We followed the methods of (Griffiths et al. (2002) which has previously been validated for juncos (Grindstaff et al., 2001). DNA was extracted using standard phenol-chloroform protocols, and the CHD locus was amplified with fluorescently labeled primers (Operon) in 10 ul PCR reactions. The resulting product was then diluted (1:20) and mixed with a molecular size standard (GeneScan-500 LIZ, Applied Biosystems), and fragment size was analyzed with the ABI 3730 DNA Analyzer and the GeneMapper® 4.0 software. Females were identified genetically by the presence of 2 bands (346bp and 387bp) and males one band (346bp).
Measuring sex ratios and sex-specific abundance
We estimated sex ratios at two sites during two time periods as the proportion of females captured per total captures. We then estimated sex-specific changes in the abundance of junco populations using data collected during bird counts made by the National Audubon Society Christmas Bird Counts (CBC) that corresponded to the latitudinal band of our sampling sites (39°N and 42°N) and data collection timing (between December 1976–January 1980 and December 2004–January 2009; count years 77–80 and 105–109). We included counts taken east of 103°2’33.18”W where juncos are almost exclusively the migratory slate-colored junco. Comparisons between CBC data and studies that have used more traditional ecological methods for measuring density have indicated that CBC data provide reliable indices of population abundance (Bock and Lepthien, 1974; Bock and Root, 1981). We calculated the sex-specific abundance of juncos by multiplying the observed sex ratio (total number of females captured divided by total number of captured birds; Table 1) for each location by the mean number of juncos reported in the CBC data (number dark-eyed juncos seen per party per hour, i.e., number of birds in a count divided by the number of hours spent counting; Table 1).
Measuring overall abundance
To determine overall abundance, we adapted previously used methods (Ketterson and Nolan, 1976) to analyze CBC data from across the junco’s entire wintering range in east-central North America (i.e., east of longitude 103° 2’33.18”W, the western boundary of Texas (excluding the panhandle)) from December 1965 to January 2023 (count years 66–123). We combined all subspecies of junco within our dataset to avoid issues with observer error and name changes but note again that with few exceptions, the juncos in this area are the eastern migratory slate-colored junco. We grouped counts into 10 latitudinal bands. Most bands (28–51°N) were 3° latitude wide, corresponding to Ketterson and Nolan (1976); however, the bands at the range edges varied in width with the southernmost band including detections ≤27°N (2° latitude wide) and the northernmost band including detections ≥52°N (11° latitude wide). While previous work excluded count data at the edge of the junco winter range, we included those data here to ascertain whether junco winter populations have expanded northwards while continuing to use the southern edge of their winter range or if the entire range has shifted northwards (Ketterson and Nolan, 1976, 1983). For each latitudinal band and for each year, we calculated the median number of juncos per party hour to determine overall abundances across the range. We also assessed longitudinal variation in abundance. Because land mass in the eastern North America declines with latitude, we restricted our analysis of abundance by longitude to comparable land mass between 74°W and 103°W.
Sex-specific and overall abundance
To relate change in overall abundance to change in winter climate, we amassed data from the U.S. National Oceanic and Atmospheric Administration’s (NOAA) National Centers for Environmental Information (NCEI) using the Global Summary of the Month (GSOM, Version 1 portal). We assembled weather data from December 1965 to February 2023 for all stations east of longitude 103° 2’33.18”W (the western boundary of Texas, excluding the panhandle). As before, we binned station data for this range into latitudinal bands that matched the CBC abundance data described above. Data for four weather variables were summarized including: mean monthly temperature (TAVG, °F), minimum monthly temperature (TMIN, °F), total snowfall per month (SNOW, mm) and total monthly precipitation (snow, rain, hail, etc.; PRCP, tenths of mm). These weather variables were then compiled for an annual winter average (December–February) to assess whether migratory decisions might be based on responses to current climate. Early work indicated that the variable ‘days per month with temperatures below 0°F (−17°C; DT00)’ was an informative weather variable to consider because it was a measure of severe extreme (Ketterson and Nolan, 1976). However, we chose to exclude this variable because it was so highly correlated with TAVG that it was statistically redundant (Spearman correlation coefficient r = −0.94). Because of this high correlation, DT00 can be interpreted via TAVG in the results. Additionally, use of DT00 would have effectively reduced the geographic range of our dataset to latitudes ≤48°N as only US weather stations included this variable. Data from higher latitudes were important to include in these analyses as it allowed us to investigate changes in range limits.
Climate variable
Because the selected weather variables were highly correlated, a principal component analysis was performed to reduce the weather variables to a smaller number of composite orthogonal variables based on the highest resulting components [R v.4.3.2 (Team, 2023), packages: FactoMineR (Lê et al., 2008) and factoextra (Kassambara and Mundt, 2020)]. The weather variables snow and precipitation were ln-transformed and scaled to improve fit. In general, principal components analysis (Table 2) revealed high loadings of monthly average temperature, monthly minimum temperature, precipitation, and snowfall on the first component. The composite variable (PC1) was then used in place of the individual weather variables in our models. Because winter abundance and climate had a non-linear relationship that could not be corrected via transformation; we used a quadratic (climate2) term rather than the linear term in the models relating abundance to climate.
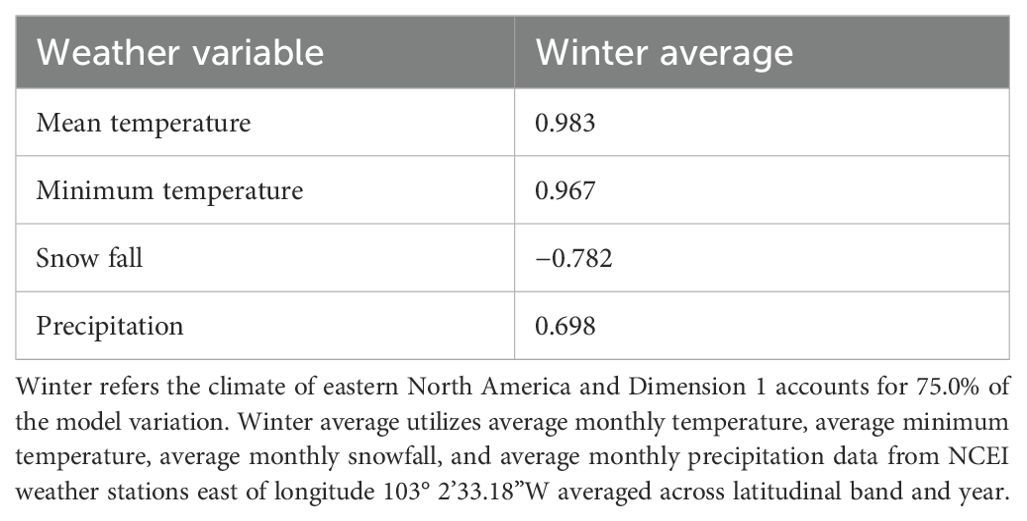
Table 2. Principal component loadings from dimension 1 derived from the analysis of 4 weather variables averaged over December–February for abundance relative to sex and/or overall abundance analyses.
Statistics
Changes to sex ratios of wintering juncos
To determine if the proportion of overwintering males and females has changed between historical and current populations, we used a linear mixed model to compare the sex ratios of captured birds between our sampling locations [(Michigan (42.29°N) and Indiana (39.1°N)] for 2 different time periods [historical (1977–1980) and current (2004–2009)]. To account for interannual variation within the model, year was included as a random effect (Satterthwaite df correction) (packages: lme4 (Bates et al., 2015), lmerTest (Kuznetsova et al., 2017)). Post-hoc analyses were used to determine if sex-ratios differed between location and current vs. historical time periods (package emmeans (Lenth, 2023)). The p-values for these multiple comparisons were corrected using a Dunnett adjustment.
Sex-specific abundance
We next used linear models to investigate how sex-specific abundance has changed over time, with latitude, and in response to climate. Sex-specific abundance data were normalized through natural log transformation. Because the predictive power of sex-specific abundance is limited in this dataset, and change in climate is potentially confounded by time, we ran two sets of analyses to decouple these effects. The first model compared sex-specific abundance to time and latitude. The second model compared sex-specific abundance to climate after controlling for latitude. Post hoc analyses were conducted as above. Due to the limited sample size, we could not include a random term in either model.
Overall abundance
Temporal and spatial variation in overall abundance were analyzed using linear models that included time (in decades), climate, latitudinal band, and their 2- and 3-way interaction terms. If none of the interaction terms were significant, we excluded them from our analyses. Overall abundances were ln-transformed for normality. Post-hoc comparisons (Dunnett adjusted) were used to determine how overall abundance varied across decade and latitude. Plots were generated using R packages ggplot2 (Wickham, 2016) and ggpmisc (Aphalo, 2023).
To address the possibility that abundance may also vary with longitude, we combined data on abundance west of 73oW and east of 103oW into three 10-degree bands and present the results as a supplementary visual that reports latitude by longitude by decade.
Results
Changes to sex ratios of wintering juncos
A linear mixed model analysis of sex ratio with respect to location and time period indicated that the proportion of females changed over time (historical vs. current; F1, 11 = 11.6, p = 0.006), but did not differ by latitude (F1, 11 = 1.7, p = 0.220). Post-hoc analyses revealed significant changes in sex ratio at both latitudes over time (p = 0.009; Figure 1), but that sex ratio did not differ between latitudes (p = 0.220). This result indicates that the proportion of females wintering at latitudinal bands 39°N (Indiana) and 42°N (Michigan) has increased over time [historical (1977–1980) vs. current (2004–2009)] to become less male-biased (Figure 1).
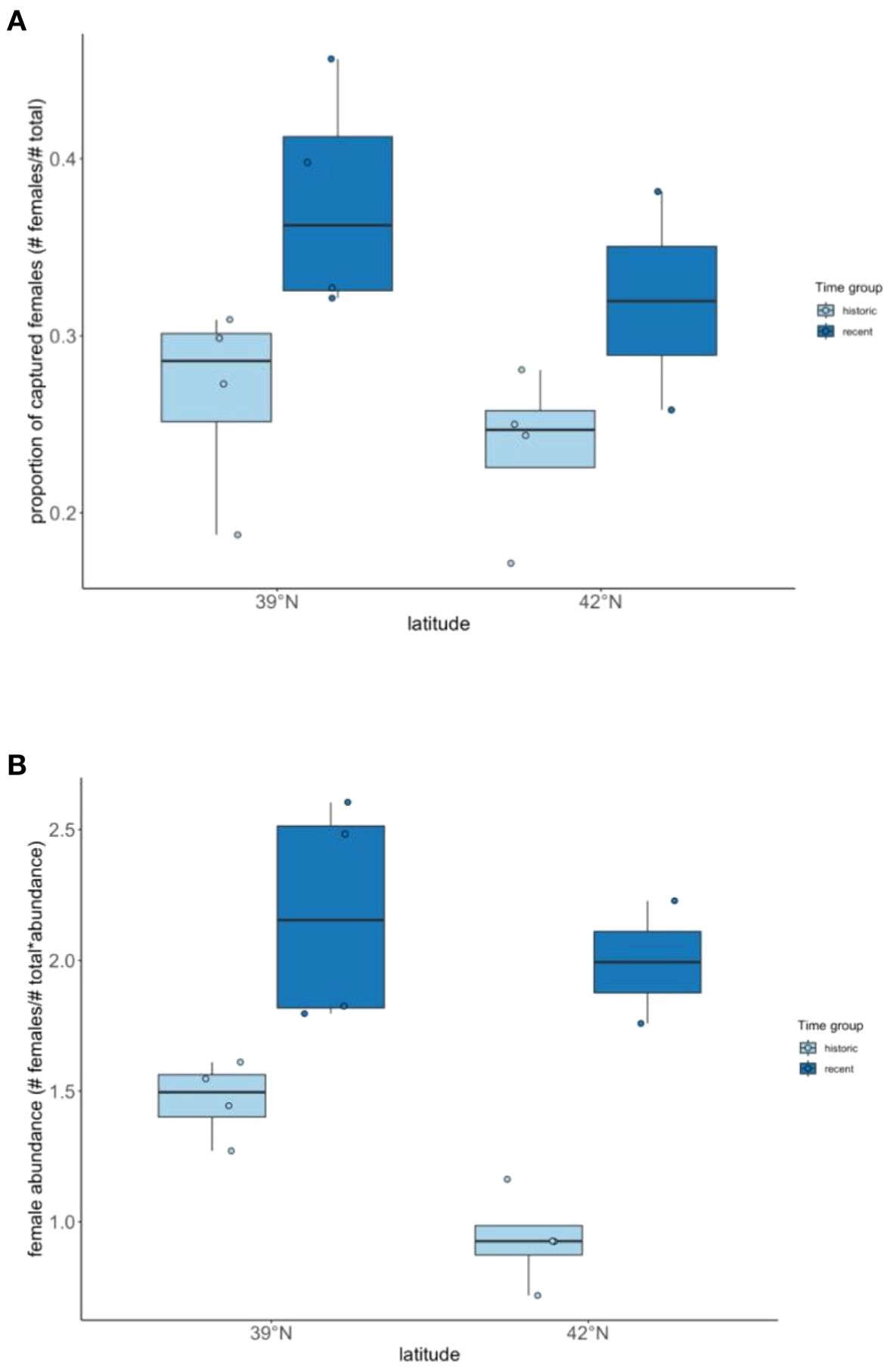
Figure 1. (A) Proportion of females captured by location (39° and 42° N latitude) and time (historical and recent)[medians and quartiles]. (B) Relative abundance of female juncos by capture site. Relative abundances were calculated by multiplying the proportion of females among birds captured in the field by abundance, as determined from Christmas Bird Counts for latitudes representative of each sampling location [Kalamazoo, MI (42.29°N 85.58°W, n= 190) and Bloomington, IN (39.16°N 86.49°W, n=558)]. Figure reveals winter populations becoming less male-biased in recent years as compared to historical data. Historical data from Ketterson and Nolan (1983).
Sex-specific abundance
The results of our analysis of sex-specific changes in abundance with respect to latitudinal band and time indicated there was a significant effect of latitude (F1, 11 = 8.6, p = 0.014) and time (historical vs. current; F1, 11 = 31.9, p < 0.001) on female abundance (Figure 2). Post-hoc analyses revealed significant differences in female abundance between latitudes (p = 0.014) and over time (p < 0.001; Figure 1). Female abundances were historically higher at 39°N than at 42°N degrees, but in recent years female abundance has increased at both latitudes.
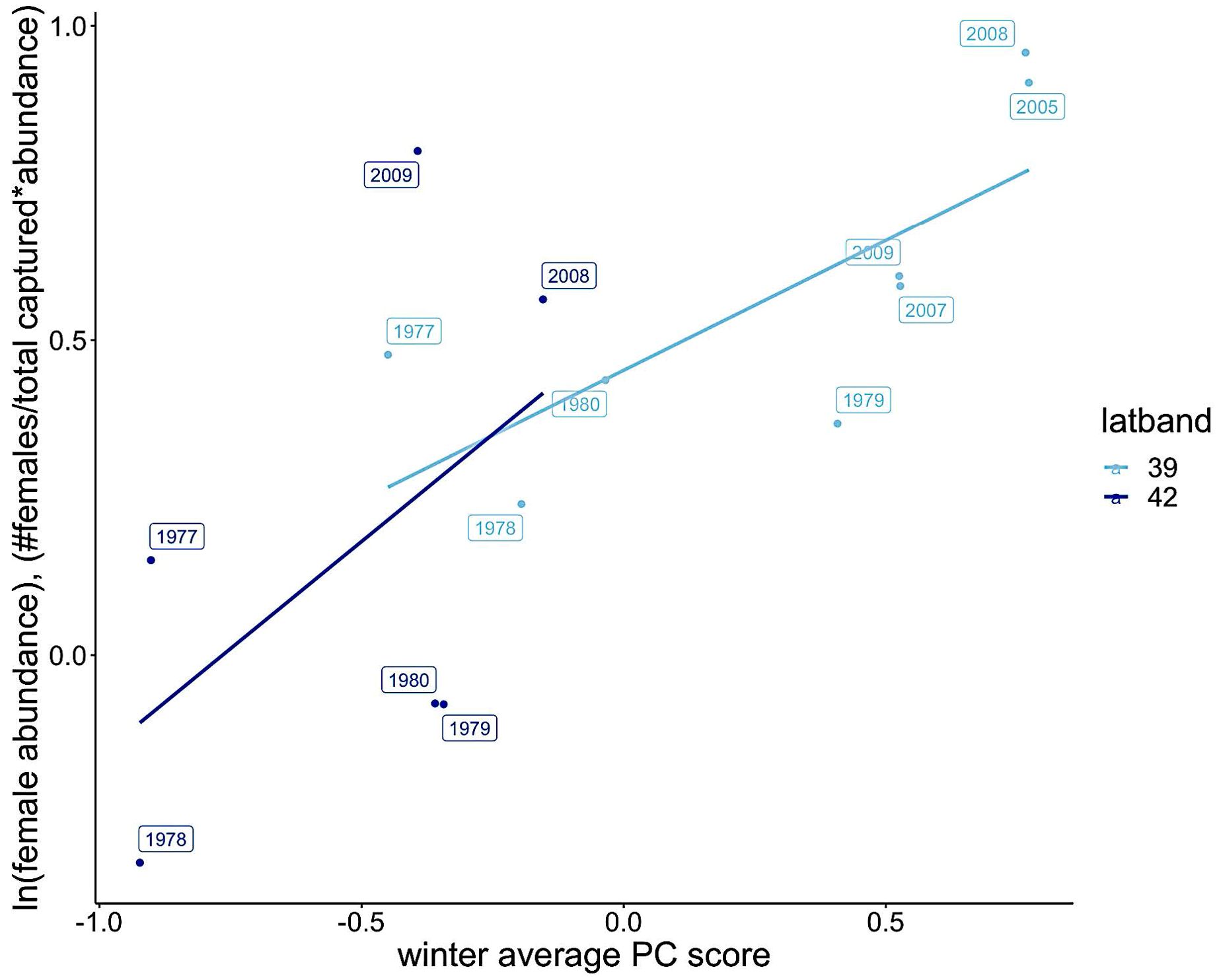
Figure 2. Relative abundance of female juncos in relation to average winter climate. Climate variables taken from the U.S. National Oceanic and Atmospheric Administration (NOAA) National Centers for Environmental Information (NCEI) and analyzed using principal component analysis for latitudinal bands 37–39°N and 40–42°N. Changes in relative abundance of females is associated with milder winters (higher average temperatures, higher mean minimum temperatures, lower snowfall, and lower precipitation).
Sex-specific changes in abundance and climate
We investigated how climate influenced the abundance of females. We found that climate was significantly related to female abundance (F1, 11 = 7.3, p = 0.020), after controlling for latitude (F1, 11 = 7.3 p = 0.020).
Overall abundance
Our analyses of overall winter junco abundance differed significantly between latitudinal bands (F9, 445 = 998.9, p < 0.001) while there was only a suggestive difference over time (decades F6, 445 = 1.9, p = 0.087). However, there was a significant interaction between latitudinal band and decade (F51, 445 = 8.2, p < 0.001), which suggests there is a time-dependent effect of latitude on winter abundance, that is abundance varies differently over time for each latitudinal band (Figure 3). Abundance west of 74°W and east of 103°W, is shown in Supplementary Figure 1 which plots abundance by decade against latitude.
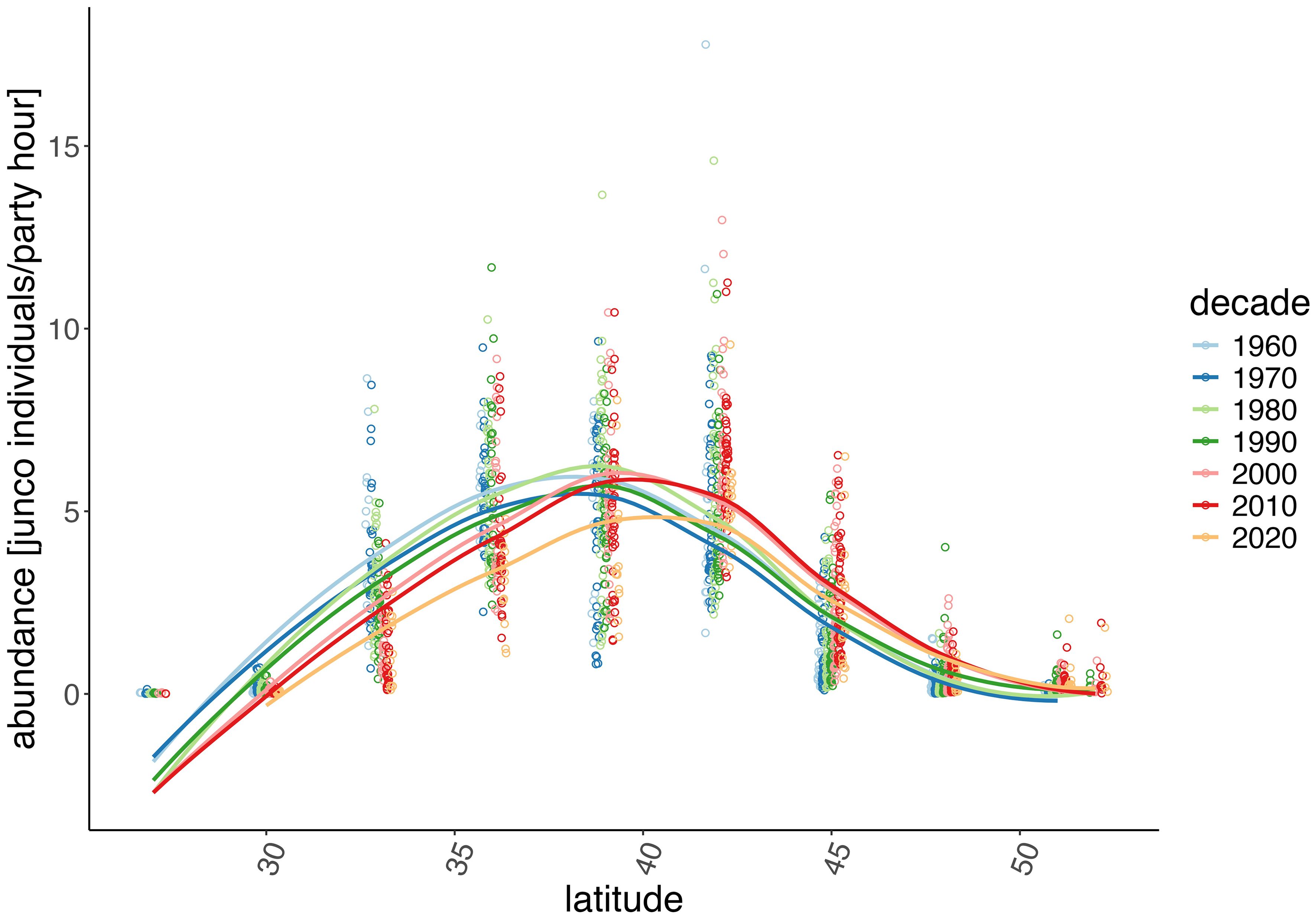
Figure 3. Comparing abundance by latitude and decade from historical 1966–2022 Christmas Bird Counts. The number of junco individuals reported per party hour for each count was binned across latitudinal bands of 3°. Data indicates a northward shift in junco winter range in recent decades.
Overall abundance and climate
We compared median winter junco abundance to the main effects of climate2 (based on mean and minimum temperature, precipitation and snowfall as captured by PCA axis 1), latitudinal band, time, and their interactions. We found that latitudinal band accounted for most of the variation within the model (F9, 379 = 1188.0, p < 0.001), but also found significant effects of climate2 (F1, 379 = 18.0, p < 0.001), the 2-way interactions between latitudinal band and decade (F51, 379 = 8.9, p < 0.001) and decade and climate2 (F51, 379 = 3.2, p = 0.005), and the 3-way interaction term, latitudinal band*decade*climate2 (F50, 379 = 1.5, p = 0.019). The remaining main effects and interactions were not significantly related to winter abundance (decade: F6, 379 = 2.1, p=0.053; and latitudinal band*climate2: F9, 379 = 0.7, p = 0.758). The significant 3-way interaction term indicated the presence of a time-dependent effect of latitude and climate.
Wintering juncos were more abundant at middle latitudes with moderate winter climates, and those favorable moderate climate conditions have shifted north over time. To decouple the effects of latitudinal band and time, we ran separate linear models for each latitudinal band to determine how junco winter abundance changed over time (i.e., decade) and with climate. We kept climate in the model to determine if climate change influenced within-band abundance.
Across all latitudinal band models, we found that abundance was not significantly related to within-latitude climate (≤27°N: F1, 15 = 0.0, p = 0.929; 28–30°N: F1, 50 = 0.2, p = 0.661; 31–33°N: F1, 50 = 2.4, p = 0.132; 34–36°N: F1, 50 = 2.3, p = 0.134; 37–39°N: F1, 50 = 1.4, p = 0.235; 40–42°N: F1, 50 = 0.5, p = 0.465; 43–45°N: F1, 50 = 0.0, p = 0.846; 46–48°N: F1, 50 = 0.5, p = 0.505; 49–51°N:F1, 48 = 3.6, p = 0.064; >52°N: F1, 22 = 0.4, p = 0.552). At 49–51°N, there was a suggestive negative relationship between abundance and climate, i.e., abundance was lower when winter climate was colder with moderate precipitation/snow. We found that at the lowest latitudinal band (≤27°N) there were no significant changes in junco abundance (F5, 15 = 1.0, p = 0.461); however, abundances at these latitudes tend to be very low with some years having no CBC detections.
From 28–36°N, abundance generally decreased over time (28–30°N: F6, 50 = 8.8, p < 0.001; 31–33°N: F6, 50 = 31.7, p < 0.001; 34–36°N: F6, 50 = 9.6, p < 0.001). At the lower latitudes (28–30°N), abundances tend to be relatively low (<0.5 juncos/party hr), but these have declined further since the 1990s (results from post-hoc analyses: Supplementary Table 1; Figures 3, 4). At lower, middle latitudes (31–36°N) abundances have historically been high (1960s mean ± SEM: 31–33°N = 4.3 ± 0.8; 34–36°N = 5.7 ± 0.3); yet starting in the 1990s (28–33°N) or 2010s (34–36°N), abundances have declined with recent years reaching their lowest levels (2020s: 31–33°N = 0.9 ± 0.1; 34–36°N = 2.7 ± 0.3). At 37–39°N, we found a similar decrease in abundance (F6, 50 = 4.0, p = 0.003); however, this trend was driven by low abundances in the 2020s (compared to the 1970s–1980s).
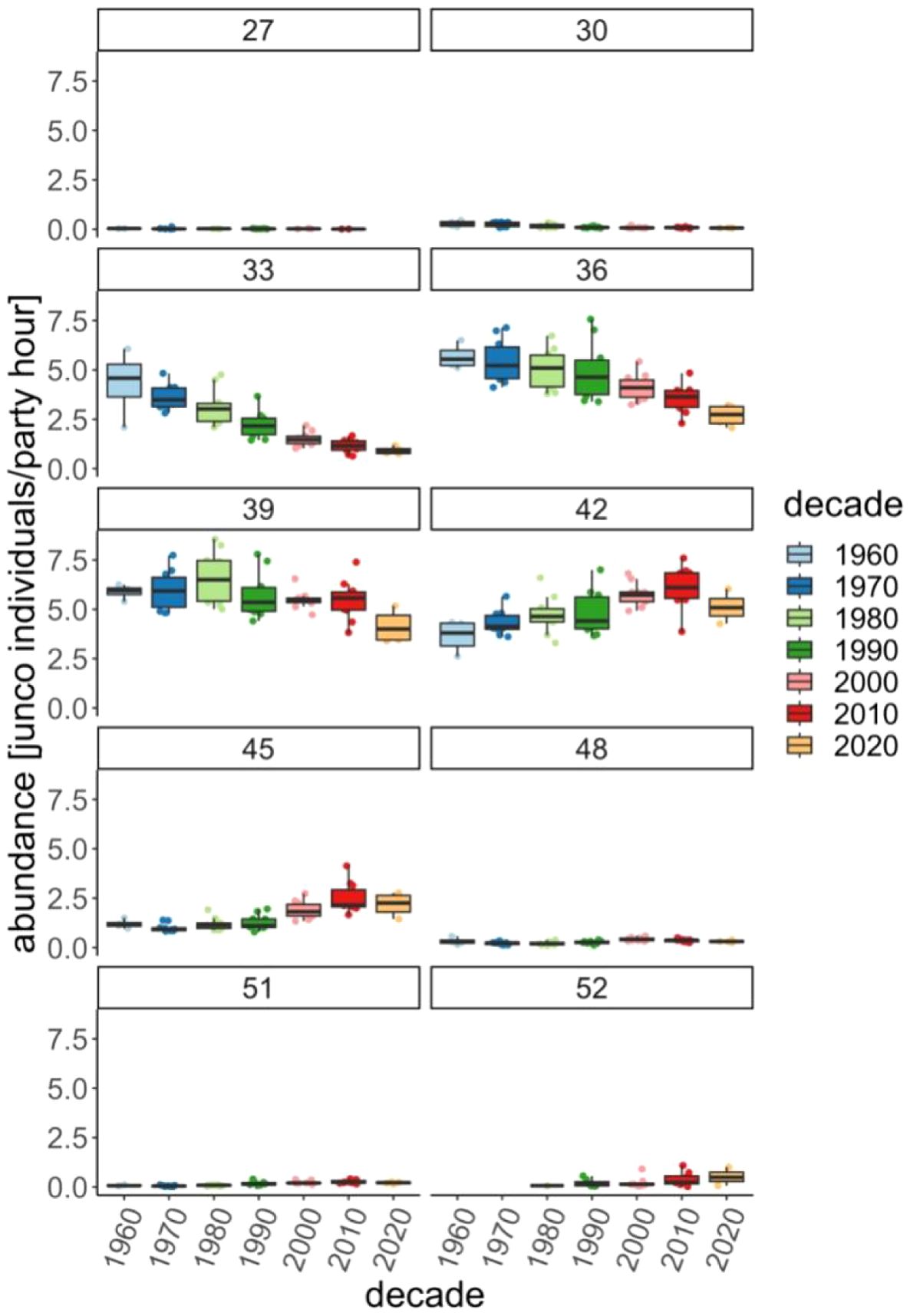
Figure 4. Median winter junco abundance (individuals/party hour) by decade for each latitudinal and [27 (<27°N), 30 (28–30°N), 33 (31–33°N), 36 (34–36°N), 39 (37–39°N), 42 (40–42°N), 45 (43–45°N), 48 (46–48°N), 51 (49–51°N), >52°N]. Data indicate decreased abundance at lower latitudes and increased abundance at middle latitudes. There has also been a range expansion north of >52°N starting in the 1980s.
While junco abundance below 39°N has experienced steady decline since the 1970s, abundances at higher latitudes (40–51°N) have generally increased (40–42°N: F6, 50 = 6.7, p < 0.001; 43–45°N: F6, 50 = 16.5, p < 0.001, 46–48°N: F6, 50 = 6.8, p < 0.001, 49–51°N: F6, 48 = 19.8, p < 0.001). The timing of abundance inflection points varied by latitude with most (40–48°N) increases occurring after the 2000s; however, at 49–51°N, abundance began increasing in the 1990s. We did not find a significant difference in junco abundance at the highest latitudes of their winter range (≥52°N; F4, 22 = 0.8, p = 0.548) because post hoc analyses only assessed decades when juncos were detected. There were no junco detections at ≥52°N until 1988. Since then, juncos have been detected on 36 CBCs in 7 different count circles. It should be noted that while abundances have increased at >40°N, at the higher latitudes (>46°N) abundances have historically been low (<0.6 juncos/party hr) and remain so compared to lower latitudes.
Discussion
We asked whether recent climate warming has led to a change in the winter range of the dark-eyed junco. We predicted that the winter range might have moved northward, and that prediction was confirmed by temporal change in abundance measured by Christmas Bird Counts. We also predicted that if climate historically accounted for longer migrations by females, then populations at higher latitudes might become less male-biased than previously if females have shortened their migrations and become relatively more abundant at higher latitudes. Based on capture data from the field at two sites over two time periods, these predictions were also confirmed. Whether females still migrate farther than males will need to be resolved by future capture data from a range of latitudes and longitudes as well as data derived from tagging and stable isotopes.
Among species that are differential migrants, females typically migrate farther than males (Cristol et al., 1999), giving rise to varying degrees of geographic variation in sex ratios during the non-breeding season. Based on hypotheses to explain the evolution of differential migration in relation to recent warming events, we predicted that milder climate might reduce male bias at northern latitudes in the junco. Our data revealed significant changes in population sex ratio at two locations (Michigan and Indiana) over a period of ~30 years, leading to more females relative to males wintering at northern locations than previously. The decline in males in MI from 1977–1980 to 2005–2009 was 8% and in IN it was 11%.
As predicted, the sex-specific changes in abundance in the winter range (sex ratio) were highly correlated with milder present-day winter climate. This pattern is further supported by increases in the overall abundance of juncos wintering at more northern latitudes closer to the breeding range and declines in overall winter abundance at southern latitudes or sites most distant from the breeding range. To our knowledge this is the first study documenting both sex-specific changes in migration as well as change in overall abundance in a differential migrant at the leading and trailing edges of its winter range. Deleted a sentence here seen by editor as conversational Large-scale sex-specific changes in the migratory behavior of juncos wintering in the northern half of the species’ winter range, along with changes in the overall abundance of juncos throughout their winter range as reported here, suggest that migratory behavior in this species is dynamic and that climatic effects on physiology and winter habitat may be mediating migratory decisions.
Results
The results presented here are congruent with previous field studies and several climate models that predict northward shifts in winter distribution by a multitude of species due to recent climate warming (Böhning-Gaese and Lemoine, 2004). By using similar methods to determine sex and to compare sex ratio and abundance at two time points separated by 30 years, our results are clear, but of course causes other than climate may account for some of the reported changes in relative abundance of the sexes and the species’ overall abundance in the eastern United States. For example, the juncos distribution-wide sex ratio may have changed over time, although there is no evidence for this. Changes in land use might also be a contributing factor. Future work will have to inform about these possibilities. The role of climate is further supported by the variation among years in the degree to which relative abundance of females varied, variation that was highly correlated with yearly variation in winter climate (Figure 2). In years with milder climate, females remained in larger numbers at intermediate and northern latitudes. When climate is similar to historical conditions (i.e., more days with lower temperatures and higher snow fall), winter sex ratios match historical data. The variation in relative abundance of the sexes between years suggests flexibility in junco migratory behavior and the possibility that this species may be able to adapt rapidly to changing climate. It remains possible that the relaxation of sexual segregation reported here could be attributable to the North Atlantic Oscillation (NAO). Given the relatively short time periods representing past and current climate, it’s conceivable that the change in sex ratio observed was caused by interannual variability associated with NAO or other indices such as Pacific North American index (PNAI), or the El Niño-Southern Oscillation index (SOI) as has been seen for female reproductive decisions (Sedinger et al., 2006; Tuttle et al., 2017). However, we think this unlikely because geographic variation in winter sex ratio had been stable until recently. Winter sex ratios based on populations sampled in nature and on museum collections comprised of birds collected in the 19th century were similar in the 1970s (Ketterson and Nolan, 1976). It is since the climate began to change markedly in the early 1980s that sex ratios have changed. Climate-associated changes in winter sex ratios, like that observed in eastern populations of the dark-eyed junco, raise important questions regarding the consequences of climate change for demography, fitness, and survival in differential migrants, as well as for the conservation of migration as an endangered phenomenon (Atwell et al., 2011). More equal sex ratios in wintering populations could lead to increases in both inter- and intra-sexual competition for winter resources, potentially reducing winter condition and survival of the non-dominant sex (in the case of the junco, females). Reductions in winter condition could influence both migration and breeding phenology, as seen in moose (Alces alces) (Singh et al., 2012) such that females must take more time to reach a suitable condition for spring migration and potentially breeding. Meanwhile, reductions in female winter survival could impact breeding sex ratios, reducing overall population growth as well as increasing competition amongst males, potentially influencing male breeding condition and survival. Associated changes in distribution and migration distances could also create new sympatric zones leading to increases in hybridization amongst subspecies (Winker, 2010) like that seen in blue whales (Balaenoptera musculus) (Attard et al., 2012), or lead to advances in breeding phenology as documented in many other avian species (Heath et al., 2012; Hurlbert and Liang, 2012) and as recently demonstrated by Kimmitt et al. for a different sub-species of junco (Kimmitt et al., 2022).
Much still remains to be discovered regarding the broad impact of climate change on differential migrants and what, if any, impact these changes in migratory behavior and the degree of sexual segregation observed will have on other aspects of the biology of these species.
Future directions
The research presented here preceded present-day technological advances that now allow tracking of individual males and females to determine distance traveled and resulting population-level variation in winter sex ratios. The research also preceded the use of stable isotopes in feathers to determine latitude of origin of birds comprising wintering populations or stable isotopes in claws to determine latitude of origin in birds comprising breeding populations. Both methods now allow for determinations of connectivity between breeding and wintering populations, and we encourage the use of both methods to learn more about current patterns of differential migration.
To summarize current ways to document sex differences in migratory behavior across species, including distance traveled, we encourage the following. Beginning with traditional measures, we encourage greater use of banding recoveries and museum collections to detect sex differences in distance migrated past and present. Geographic variation in sex ratio can also be gleaned from e-bird for species that clearly differ in appearance by sex. E-bird data can also reveal latitudinal shifts in species abundance regardless of sex.
Of the multiple hypotheses for the causes of differential migration, such as body size, competition between the sexes during winter, and the advantage to males of early arrival on the breeding range, climate has been most closely associated with body size in association with fasting endurance. Explanations based on multiple considerations, i.e., sex-specific costs and benefits of early arrival, winter competition for resources, fasting endurance, and distance-related costs of migration all point towards a mediating effect of climate. Milder climate can reduce competition, require less fasting, and foster earlier springs and spring departure dates. The benefits of early arrival combined with a warming climate may favor males making shorter migrations, causing the population as a whole to move north. To test this, we recommend comparing current e-bird distributions and current Christmas Bird Count distributions with earlier Christmas Bird Count distribution. Finally, we encourage mechanistic species distribution models of sex-specific physiological phenotypes to better predict future distributional changes (Drymon et al., 2020).
Data availability statement
The raw data supporting the conclusions of this article will be made available by the authors, without undue reservation.
Ethics statement
The animal study was approved by Indiana University Bloomington Institutional Animal Care and Use Committee. The study was conducted in accordance with the local legislation and institutional requirements.
Author contributions
DO: Writing – original draft. SA: Writing – review & editing. EK: Writing – original draft, Writing – review & editing.
Funding
The author(s) declare financial support was received for the research, authorship, and/or publication of this article. This research was funded in part by the American Ornithologist’s Union (now American Ornithological Society) the Indiana Academy of Sciences, and the Office for the Vice-Provost for Research, Indiana University.
Acknowledgments
We thank Rich and Brenda Keith from the Pitsfield Banding Station for helping to capture birds in Michigan; and E. Snajdr, R. Kiley, Val Nolan Jr., and the rest of the United Junco Workers for assistance in Indiana. We also thank the many citizens who participated in the Audubon Society’s Annual Christmas Bird Count. We thank Tim Meehan for assistance with the CBC data. Special thanks to Dr. S. Robeson for helpful discussions and suggestions on the manuscript and E. Hernandez and the Indiana University’s Statistical Consulting Center for statistical assistance.
Conflict of interest
The authors declare that the research was conducted in the absence of any commercial or financial relationships that could be construed as a potential conflict of interest.
Publisher’s note
All claims expressed in this article are solely those of the authors and do not necessarily represent those of their affiliated organizations, or those of the publisher, the editors and the reviewers. Any product that may be evaluated in this article, or claim that may be made by its manufacturer, is not guaranteed or endorsed by the publisher.
Supplementary material
The Supplementary Material for this article can be found online at: https://www.frontiersin.org/articles/10.3389/fbirs.2024.1417867/full#supplementary-material
References
Attard C. R. M., Beheregaray L. B., Jenner K. C. S., Gill P. C., Jenner M. N., Morrice M. G., et al. (2012). Hybridization of Southern Hemisphere blue whale subspecies and a sympatric area off Antarctica: impacts of whaling or climate change? Mol. Ecol. 23, 5715–5727. doi: 10.1111/mec.12025
Atwell J. W., O'Neal D. M., Ketterson E. D. (2011). Migration as a moving target: Intra-species variation and responses to environment change, as illustrated in a sometimes migratory songbird. Environ. Law 2, 298–316.
Bates D., Mächler M., Bolker B., Walker S. (2015). Fitting linear mixed-effects models usinglme4. J. Stat. Software 1, 1–48. doi: 10.18637/jss.v067.i01
Bock C. E., Lepthien L. W. (1974). Winter pattern of bird species diversity and abundance in the United States and southern Canada. Am. Birds. 28, 556–562.
Bock C. E., Root T. L. (1981). The Christmas Bird Count and avian ecology. Stud. inAvian Biol., 17–23.
Böhning-Gaese K., Lemoine N. (2004). Importance of climate change for the ranges, communities and conservation of birds. Birds And Climate Change. 35, 211. doi: 10.1046/j.1523-1739.2003.01389.x
Briedis M., Bauer S. (2018). Migratory connectivity in the context of differential migration. Biol. Lett. 12, 20180679. doi: 10.1098/rsbl.2018.0679
Burakowski E. A., Wake C. P., Braswell B., Brown D. P. (2008). Trends in wintertime climate in the northeastern United States: 1965–2005. J. Geophysical Research: Atmospheres D20, D20114. doi: 10.1029/2008JD009870
Carbeck K., Wang T., Reid J. M., Arcese P. (2022). Adaptation to climate change through seasonal migration revealed by climatic versus demographic niche models. Glob Chang Biol. 14, 4260–4275. doi: 10.1111/gcb.v28.14
Catry P., Campos A., Almada V., Cresswell W. (2004). Winter segregation of migrant European robins Erithacus rubecula in relation to sex, age and size. J. Avian Biol. 3, 204–209. doi: 10.1111/j.0908-8857.2004.03266.x
Chen I. C., Hill J. K., Ohlemuller R., Roy D. B., Thomas C. D. (2011). Rapid range shifts of species associated with high levels of climate warming. Science 6045, 1024–1026. doi: 10.1126/science.1206432
Cristol D. A., Baker M., Carbone C. (1999). Differential migration revisted. Curr. Ornithology. 15, 33–88.
Deakin Z., Hamer K. C., Sherley R. B., Bearhop S., Bodey T. W., Clark B. L., et al. (2019). Sex differences in migration and demography of a wide-ranging seabird, the northern gannet. Mar. Ecol. Prog. Ser. 622, 191–201. doi: 10.3354/meps12986
Drymon J. M., Dedman S., Froeschke J. T., Seubert E. A., Jefferson A. E., Kroetz A. M., et al. (2020). Defining sex-specific habitat suitability for a northern Gulf of Mexico shark assemblage. Front. Mar. Sci. 7. doi: 10.3389/fmars.2020.00035
Dunn E. H., Ralph C. J. (2004). Use of mist nets as a tool for bird population monitoring. Stud. Avian Biol. 29, 1–6.
Fayet A. L., Freeman R., Shoji A., Boyle D., Kirk H. L., Dean B. J., et al. (2016). Drivers and fitness consequences of dispersive migration in a pelagic seabird. Behav. Ecol. 4, 1061–1072. doi: 10.1093/beheco/arw013
Flemming T. H., Eby P. (2003). “Ecology of bat migration,” in Bat Ecology. Eds. Kunz T. H., Fenton M. B. (University of Chicago Press, Chicago), 156–208.
Gow E. A., Wiebe K. L. (2014). Males migrate farther than females in a differential migrant: an examination of the fasting endurance hypothesis. R. Soc. Open Sci. 4, 140346. doi: 10.1098/rsos.140346
Griffiths R., Double M. C., Orr K., Dawson R. J. G. (2002). A DNA test to sex most birds. Mol. Ecol. 8, 1071–1075. doi: 10.1046/j.1365-294x.1998.00389.x
Grindstaff J., Buerkle A., Casto J., Nolan V., Ketterson E. (2001). Offspring sex ratio is unrelated to male attractiveness in dark-eyed juncos ( Junco hyemalis ). Behav. Ecol. Sociobiology 4, 312–316. doi: 10.1007/s002650100367
Heath J. A., Steenhof K., Foster M. A. (2012). Shorter migration distances associated with higher winter temperatures suggest a mechanism for advancing nesting phenology of American kestrels Falco sparverius. J. Avian Biol. 4, 376–384. doi: 10.1111/j.1600-048X.2012.05595.x
Hedh L., Hedenstrom A. (2020). The migration pattern of a monogamous shorebird challenges existing hypotheses explaining the evolution of differential migration. J. Theor. Biol. 487, 110111. doi: 10.1016/j.jtbi.2019.110111
Hurlbert A. H., Liang Z. (2012). Spatiotemporal variation in avian migration phenology: citizen science reveals effects of climate change. PloS One 2, e31662. doi: 10.1371/journal.pone.0031662
Kassambara A., Mundt F. (2020). _factoextra: Extract and Visualize the Results of Multivariate Data Analyses_. R package version 1.0.7.
Ketterson E. D., Nolan V. (1976). Geographic variation and its climatic correlates in the sex ratio of eastern-wintering dark-eyed juncos (Junco hyemalis hyemalis). Ecology. 57, 679–693. doi: 10.2307/1936182
Ketterson E. D., Nolan V. (1979). Seasonal, annual, and geographic-variation in sex-ratio of wintering populations of dark-eyed juncos (Junco-Hyemalis). Auk 3, 532–536.
Ketterson E. D., Nolan V. (1982). The role of migration and winter mortality in the life-history of a temperate-zone migrant, the dark-eyed junco, as determined from demographic-analyses of winter populations. Auk 2, 243–259.
Ketterson E. D., Nolan V. (1983). The evolution of differential bird migration. Curr. Ornithol. 1, 357–402.
Kimmitt A. A., Becker D. J., Diller S. N., Gerlach N. M., Rosvall K. A., Ketterson E. D. (2022). Plasticity in female timing may explain earlier breeding in a North American songbird. J. Anim. Ecol. 10, 1988–1998. doi: 10.1111/1365-2656.13772
Kuznetsova A., Brockhoff P. B., Christensen R. H. B. (2017). lmerTest package: tests in linear mixed effects models. J. Stat. Software 13, 1–26. doi: 10.18637/jss.v082.i13
Lê S., Josse J., Husson F. (2008). FactoMineR: anRPackage for multivariate analysis. J. Stat. Software 1, 1–18.
Lenth R. (2023). _emmeans: Estimated Marginal Means, aka Least-Squares Means_. R package version 1.8.9.
Morbey Y. E., Ydenberg R. C. (2008). Protandrous arrival timing to breeding areas: a review. Ecol. Lett. 6, 663–673. doi: 10.1046/j.1461-0248.2001.00265.x
Nolan V., Ketterson E. D. (1990). Timing of autumn migration and its relation to winter distriubtion in dark-eyed juncos. Ecology 4, 1267–1278. doi: 10.2307/1938264
Nolan V. Jr., Ketterson E. D., Cristol D. A., Rogers C. M., Clotfelter E. D., Titus R. C., et al. (2002). Dark-eyed Junco (Junco hyemalis). Eds. Poole A., Gills F. (Philidelphia, PA: The Birds of North America, Inc).
Pedersen L., Jakobsen N. M., Strandberg R., Thorup K., Tøttrup A. P. (2019). Sex-specific difference in migration schedule as a precursor of protandry in a long-distance migratory bird. Sci. Nat. 7–8, 45. doi: 10.1007/s00114-019-1637-6
Ralph C. J., Hollinger K., Miller S. L. (2004). Monitoing productivity with multiple mist-net stations. Stud. Avian Biol. 29, 12–20.
Rogers C. M., Theimer T. L., Nolan V., Ketterson E. D. (1989). Does dominance determine how far dark-eyed juncos, Junco hyemalis, migrate into their winter range? Anim. Behav. 37, 498–506. doi: 10.1016/0003-3472(89)90096-1
Ruckstuhl K. E. (2007). Sexual segregation in vertebrates: proximate and ultimate causes. Integr. Comp. Biol. 2, 245–257. doi: 10.1093/icb/icm030
Ruckstuhl K. E., Neuhaus P. (Eds.) (2005). Sexual segregation in vertebrates: Ecology of the two sexes (Cambridge: Cambridge University Press).
Rushing C. S., Royle J. A., Ziolkowski D. J. Jr., Pardieck K. L. (2020). Migratory behavior and winter geography drive differential range shifts of eastern birds in response to recent climate change. Proc. Natl. Acad. Sci. U.S.A. 23, 12897–12903. doi: 10.1073/pnas.2000299117
Sedinger J. S., Ward D. H., Schamber J. L., Butler W. I., Eldridge W. D., Conant B., et al. (2006). Effects of El Nino on distribution and reproductive performance of Black Brant. Ecology 1, 151–159. doi: 10.1890/04-1013
Singh N. J., Borger L., Dettki H., Bunnefeld N., Ericsson G. (2012). From migration to nomadism: movement variability in a northern ungulate across its latitudinal range. Ecol. Appl. 7, 2007–2020. doi: 10.1890/12-0245.1
Snell K. R. S., Frederiksen M., Bregnballe T. (2021). Differential spatial migration programmes are both sex and age specific for migratory great cormorants. J. Ornithology 4, 1075–1085. doi: 10.1007/s10336-021-01906-9
Team R. C. (2023). R: A Language and Environment for Statistical Computing_ (R Vienna, Austria: Foundation for Statistical Computing).
Tingley M. W., Beissinger S. R. (2009). Detecting range shifts from historical species occurrences: new perspectives on old data. Trends Ecol. Evol. 11, 625–633. doi: 10.1016/j.tree.2009.05.009
Tuttle E. M., Grunst A. S., Grunst M. L., Korody M. L., Betuel A. M., Barcelo-Serra M., et al. (2017). Climatically driven changes in population composition and offspring sex-morph ratio in a polymorphic species. Ecosphere 4, e01762. doi: 10.1002/ecs2.2017.8.issue-4
Walther G. R. (2010). Community and ecosystem responses to recent climate change. Philos. Trans. R Soc. Lond B Biol. Sci. 1549, 2019–2024. doi: 10.1098/rstb.2010.0021
Walther G. R., Post E., Convey P., Menzel A., Parmesan C., Beebee T. J., et al. (2002). Ecological responses to recent climate change. Nature 6879, 389–395. doi: 10.1038/416389a
Wickham H. (2016). ggplot2: Elegant Graphics for Data Analysis (New York: Springer-Verlag). doi: 10.1007/978-3-319-24277-4_9
Winker K. (2010). Model of speciation in migratory animals. Ornithol. Monogr. 69, 1–30. doi: 10.1525/om.2010.69.1.1
Keywords: migration, differential migration, sex, dark-eyed junco, climate
Citation: O’Neal DM, Austin SH and Ketterson ED (2024) Impacts of a warming climate on the non-breeding distribution of a classic differential migrant. Front. Bird Sci. 3:1417867. doi: 10.3389/fbirs.2024.1417867
Received: 15 April 2024; Accepted: 14 October 2024;
Published: 20 November 2024.
Edited by:
Noah G. Perlut, University of New England, United StatesReviewed by:
Brian Olsen, University of Maine, United StatesRusty Gonser, Indiana State University, United States
Copyright © 2024 O’Neal, Austin and Ketterson. This is an open-access article distributed under the terms of the Creative Commons Attribution License (CC BY). The use, distribution or reproduction in other forums is permitted, provided the original author(s) and the copyright owner(s) are credited and that the original publication in this journal is cited, in accordance with accepted academic practice. No use, distribution or reproduction is permitted which does not comply with these terms.
*Correspondence: Ellen D. Ketterson, ketterso@iu.edu