- 1Department of Fisheries, Wildlife, and Conservation Science, Oregon State University, Corvallis, OR, United States
- 2Finch Research Network, Cincinnatus, NY, United States
Red Crossbills (Fringillidae: Loxia curvirostra) are finches specialized to pry open cones and extract seeds of coniferous trees. Within the species, variable bill morphology may provide more efficient foraging on some species of conifers than others. Subgroups also have distinctly different contact calls often given in flight (hereafter, call types). Variable morphology and discrete call types suggest the existence of distinct evolutionary lineages. Because coniferous trees produce seeds on irregular and unpredictable schedules, crossbills wander widely in search of food. The key conifer hypothesis suggests that each call type diverged by specializing on a single conifer species to maximize foraging efficiency, while other hypotheses have emphasized geographic isolation as a driver of lineage divergence. To quantify the degree to which call types occur with specific conifer species, we surveyed Red Crossbills in five ecoregions of western and central Oregon, USA, an area with high conifer diversity. Two of five call types (Types 5 and 10) were encountered in only one ecoregion and associated with their hypothesized key conifers. Three others (Types 2, 3, and 4) were found to wander widely and did not exclusively align geographically with their presumed key conifer species. Whereas types 2 and 3 were sometimes associated with their proposed key conifers, they wandered widely during our 2-year study and occurred with a wide variety of conifers. Type 4 was not significantly associated with its key conifer and also wandered widely during our study. Relationships of call types with key conifers were weaker in the winter and spring, when Types 3 and 4 were frequently encountered in hard-coned pines rather than their soft-coned key conifer species. In our study area, the key conifer hypothesis was strongly supported only for call type 10 as that call type was not encountered away from the coastal range of Sitka Spruce (Picea sitchensis). We found mixed support for the key conifer hypothesis based on our measure of geographic associations of crossbills with a diverse array of coniferous tree species. In most cases we observed, crossbills wandered widely and associated with multiple species of conifers.
1 Introduction
Diverse yet limited resources are a defining force in the evolution of biodiversity (Ford et al., 1973; Janz et al., 2001; Gavrilets et al., 2007; Lamichhaney et al., 2015; Anderson and Weir, 2022), but long time scales and shifting conditions can obscure potential links between ecology and speciation (Hendry, 2009; Sadedin et al., 2009). Adaptive divergence through resource specialization is well-studied in island systems (Gavrilets and Vose, 2007; Sadedin et al., 2009; Lamichhaney et al., 2015), and may also be important in the complex, interconnected landscapes that cover most of the globe (Hendry, 2009; Foote, 2012). Research focused on several wide-ranging vertebrates has revealed the potential for socially learned behaviors to mediate adaptive radiation, leading to rapid evolution of specialized forms, or ecotypes (Benkman, 1993; Sewall et al., 2004; Pilot et al., 2010; Foote et al., 2016). Red Crossbills (Frigillidae: Loxia curvirostra), finches specialized to forage on conifer seeds by prying open cones to extract seeds, form cultural groups with discrete flight call characteristics and show varying ecological and morphological distinctness (Benkman, 1993; Groth, 1993a; Groth, 1993b; Benkman et al., 2009). Flight call characteristics are thought to be entirely learned during the first autumn after hatching, and are not known to be dictated by morphology (Groth, 1993b). These ecotypes (hereafter “call types”) are broadly sympatric and engage in dynamic movements across overlapping ranges as they seek seed-producing conifers. Call types are thought to represent cohesive evolutionary lineages, as their flight calls are distinct and consistent, intermediate flight calls are rare, and types appear to largely mate assortatively (Groth, 1993a; Benkman et al., 2009). Call Types also differ in average bill size, which may facilitate their specialization on different conifer species (Benkman, 1993; Groth, 1993b). Despite the description of 12 North American call types (Young and Spahr, 2017; Young et al.), their distribution and ecology remains incompletely known, and multiple hypotheses for the causes of their adaptive radiation have been proposed (Benkman, 1993; Kelsey and Hahn, 2008; Martin et al., 2020). The existence of call types potentially specialized to forage on particular coniferous species provides interesting potential to illuminate the role of ecology in crossbill speciation.
The irruptive or nomadic behavior of Red Crossbills has evolved in response to spatially and temporally fluctuating availability of food resources as the mast cycles of conifers produce a shifting mosaic of available cone crops (Groth, 1993b; Benkman, 1999; Newton, 2006; Kelsey et al., 2008; Young, 2011; Cornelius et al., 2013; Teitelbaum and Mueller, 2019). Locating and exploiting cone crops requires movements across large geographic areas, especially if call types are specialized to particular conifer species or co-occurring conifer species with similar cone structures are geographically asynchronous in their mast cycles (Benkman, 1999; Kelsey et al., 2008; Cornelius et al., 2013). Crossbills breed in the late summer and facultatively in late winter, taking advantage of seasonal variation in food availability (Cornelius et al., 2013). Crossbill movements are known to vary in scale from local to continental (Groth, 1993b; Newton, 2006; Young, 2011). Each call type’s unique vocalizations have allowed researchers to track type-specific movements across vast areas of North America and Eurasia (Groth, 1993a; Groth, 1993b; Benkman, 2003; Martin et al., 2019), despite extreme similarity in morphological appearance. By recording flight calls, our understanding of spatial and temporal patterns of movement along with information on choice of coniferous tree species for foraging is quickly improving.
How and why has such morphological and vocal divergence occurred within Red Crossbills? Treatments of ecology and evolution in the Red Crossbill complex suggest two primary mechanisms that differ in the relative importance they ascribe to geographic isolation and resource specialization (Benkman, 1993; Kelsey and Hahn, 2008; Kelsey et al., 2008; Martin et al., 2020). In western North America, for example, studies of foraging performance and preference have suggested that each call type is adaptively specialized on a single conifer species, a postulate known as the “key conifer hypothesis” (Benkman, 1993; Benkman, 2003; Irwin, 2010). This hypothesis predicts that each call type forages most efficiently on a single tree species, causing close specialization on this species particularly during periods of resource scarcity (Benkman, 2003). Conifer seeds ripen in late summer and their availability diminishes through the following spring, so call types would be expected to forage mostly on their key conifers from late winter to early summer when seeds are scarce and the call type best adapted to each key conifer can efficiently extract its limited resources (Benkman, 1993; Cornelius and Hahn, 2012). The foraging efficiency data which underscore the key conifer hypothesis are compelling, but the geographic scope is limited and they omit some ecologically important conifer species (Benkman, 2003; Kelsey et al., 2008). Moreover, recent studies in western North America and Eurasia have proposed that call types are closely connected to geographic regions, or “core zones of occurrence”, to which they cyclically return, potentially allowing slow differentiation in parapatry (Kelsey and Hahn, 2008; Young et al., 2011; Martin et al., 2020). Call types are undoubtedly distinct in ecological preferences, even in sympatry (Benkman, 2003; Benkman, 2007; Young, 2011) but the small body of field data on habitat preferences has shown mixed results for conifer specialization (Kelsey and Hahn, 2008; Kelsey et al., 2008).
We evaluated associations of Red Crossbills with their hypothesized key conifer species in the Pacific Northwest of North America (Oregon, USA). No focused publications have treated call type distribution and ecology in the Pacific Northwest (but see (Groth, 1993b; Kelsey et al., 2008)), yet the region contains five proposed key conifers (henceforth PKCs), at least two other conifer species which crossbills are known to forage on, and five regularly occurring flight call types (Benkman, 2003; Meyers et al., 2015). By recording crossbills and quantifying the availability of cones at hundreds of sites across the Pacific Northwest over two years, we evaluated the prediction of the key conifer hypothesis that each call type should associate with its PKC, especially during the seasons of relative resource scarcity.
2 Methods
2.1 Field surveys
2.1.1 Study area
We characterized the seasonal distribution and conifer associations of Red Crossbill call types in western and central Oregon (Figure 1) from July 2020 through September 2022. We expected to find five call types (Figure 2) which are thought to be associated with five PKCs, including Type 2 (ponderosa pine, Pinus ponderosa), Type 3 (western hemlock, Tsuga heterophylla), Type 4 (Douglas-fir, Pseudotsuga menziesii), Type 5 (lodgepole pine, Pinus contorta), and Type 10 (Sitka spruce, Picea sitchensis) (Benkman, 1993). We delineated five study ecoregions based on the presence or absence of these five PKCs and five other conifers on which crossbills may forage (Engelmann spruce Picea engelmannii, mountain hemlock Tsuga mertensiana, western larch Larix occidentalis, shore pine Pinus contorta contorta, and western white pine Pinus monticola) (Figure 1, Appendix A). Ecoregions broadly matched those in the Flora of Oregon (Meyers et al., 2015), with modifications to separate the Coast Range and Windward Cascades due to different conifer compositions and to exclude principally unforested regions. The Outer Coast included all of the land area within 8 km of the Pacific Ocean. The Coast Range included all locations above 120 m in elevation that are east of the outer coast and west of the Willamette River. All locations above 120 m, below 1,200 m in elevation, east of 123 longitudinal degrees and west of the maximum elevation (crest) line of the Cascade Mountains were designated as Windward Cascades. The Cascade Crest included all locations west of the crest line of the Cascades that are above 1,200 m and all locations east of the crest line that are above 1,430 m. The Leeward Cascades included locations on the east slope of the Cascades that are above 1,000 m and below 1,430 m. Regions of the Coast Range south of 43.8 degrees latitude and regions of the Cascades south of 42.7 degrees latitude were excluded from the study.
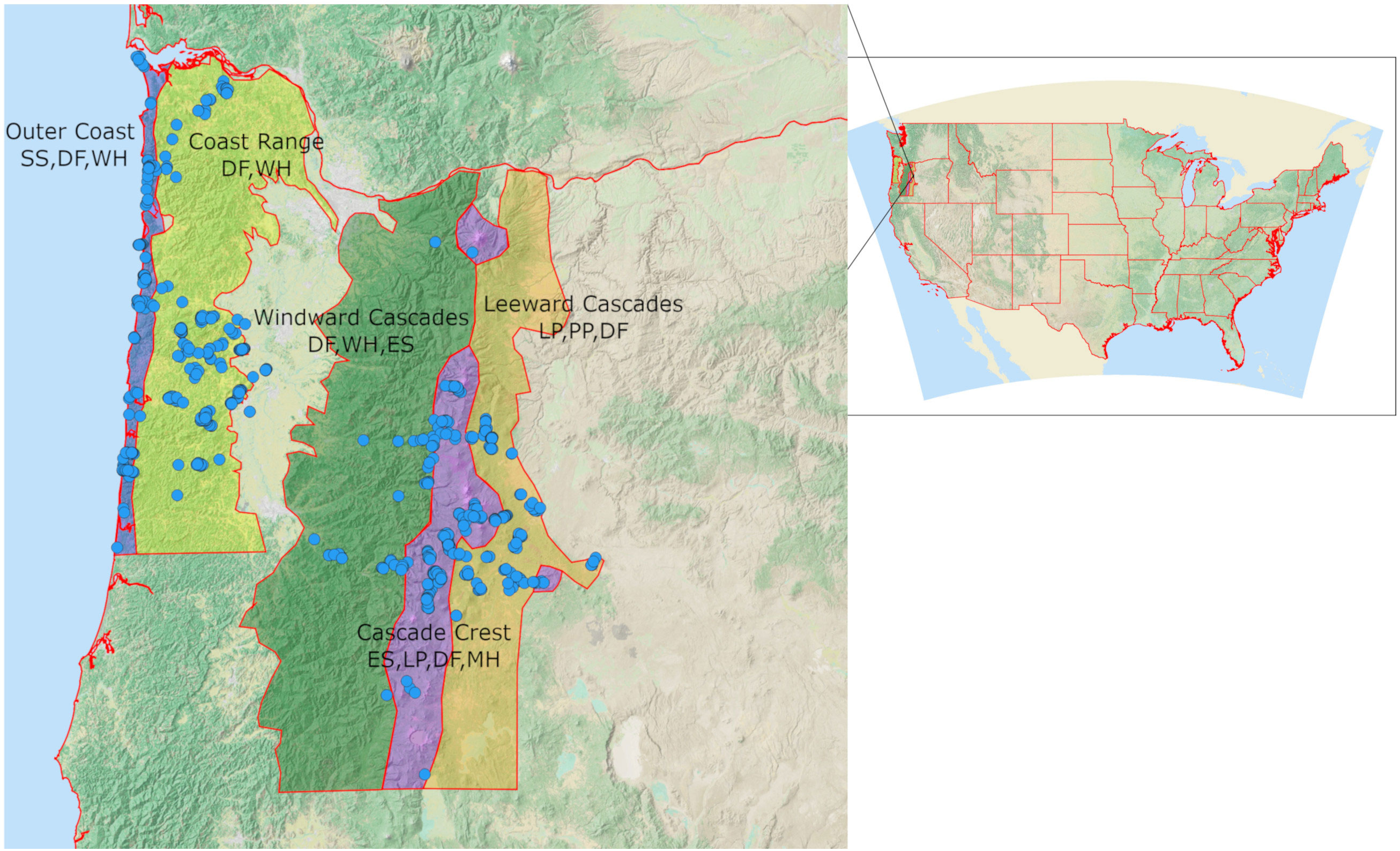
Figure 1 Location of the study area in the contiguous United States of America and Distribution of all 923 stationary Crossbill/conifer survey sites overlain over the 5 survey regions. Some sites were surveyed more than once, and others were in close proximity. The Central Cascades and Central Coast Range were surveyed more intensely than other parts of those regions, while the Outer Coast was surveyed fairly evenly. Several points in the low-elevation Willamette Valley are outside the targeted survey regions. PKCs and other known forage trees are denoted for each study region. SS, Sitka spruce; DF, Douglas-fir; WH, western hemlock; ES, Engelmann spruce; LP, lodgepole pine; MH, mountain hemlock; PP, ponderosa pine.
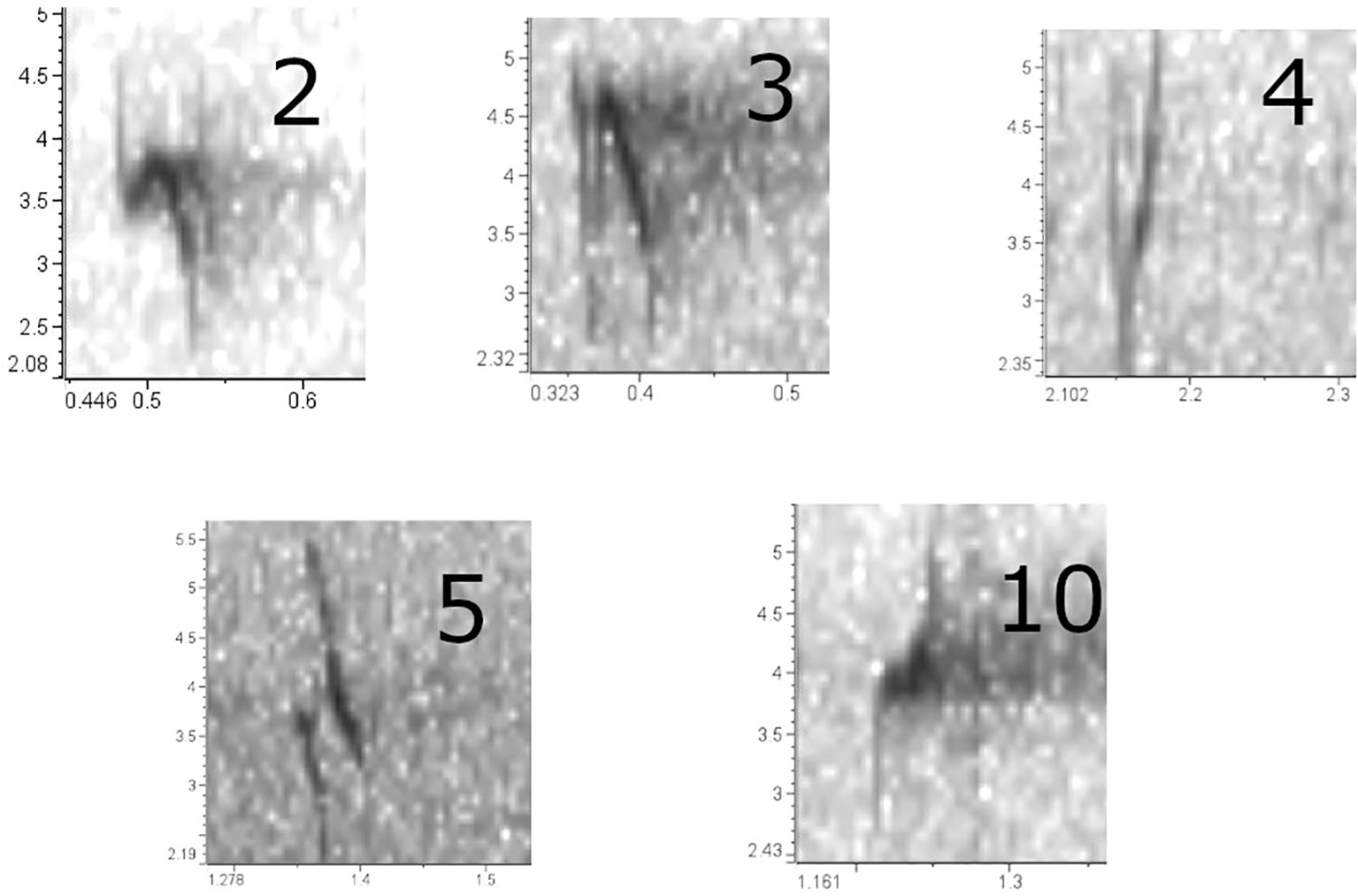
Figure 2 Flight call sonograms the five types typically encountered in Oregon, generated with Raven Lite 2.0.4. These prints are from recordings obtained between 2020 and 2023 in our study area. PKCs are as follows: ponderosa pine (Type 2), western hemlock (Type 3), Douglas-fir (Type 4), lodgepole pine (Type 5), and Sitka spruce (Type 10).
2.1.2 Crossbill surveys
Red Crossbills are nomadic and frequently absent from coniferous woodlands across our study area. Consequently, use of standard geographic sampling designs would be inefficient at locating crossbills over most of the area. We conducted targeted (i.e. ad hoc) surveys aimed at maximizing our ability to encounter crossbills. Selection of field sites was based on data available in the eBird citizen science database (eBird.org) (eBird, 2023), personal experience, and scouting trips distributed in all five ecoregions (Figure 1). We periodically surveyed sites where mature forests including each conifer species of interest were present. We spent at least eight hours surveying each of the focal ecoregions at least once every 3 to 4 months. High-elevation surveys were limited in the winter of 2020-2021 due to weather. We surveyed for crossbills by standing and observing birds at a stationary position for 3-120 minutes, with an average survey time of 25 minutes. Typical survey time was 3-5 minutes, but times were extended when crossbills were present to facilitate capturing recordings for identification of call types. Stationary points were selected by travelling on roads or trails and stopping at points with coniferous trees over 3 meters tall present. GPS coordinates were recorded for each survey site. We spaced survey locations at least 500 m distant from each other, and many distances were greater due to lack of suitable habitat. Locations and durations of all field surveys, as well as crossbill recordings, were deposited in eBird. We visited several sites more than once in different seasons or years to track temporal changes in occurrence of crossbills.
Except in the briefest and most distant encounters, we collected audio recordings of all crossbill encounters using smartphones. We stored recordings as .wav files using Voice Record Pro on iOS and the default “Voice Recorder” app on a Samsung Galaxy S10 Plus. Red Crossbill call types were identified using sonogram analysis of flight calls in Raven Pro 1.6. To our knowledge, no automatic recognition models exist for crossbill call types, so all flight calls on each recording were identified manually based on shape, peak frequency, and frequency range according to the most recent available literature on call type determination (Groth, 1993b; Irwin, 2010; Young and Spahr, 2017).
2.1.3 Conifer and cone surveys
Starting in July 2020, we collected data on conifer species at each crossbill survey site. At 835 survey locations, we scanned mature potentially cone-producing trees (>3 m tall) in a 360 degree radius to determine species composition and cone crop. We randomly identified approximately 25 conifers at each field site (if available) to standardize for differing levels of visibility. Conifer species were identified according to the Flora of Oregon (Benkman, 2007). We identified many coneless true firs (Abies sp.) and cultivated conifers to the genus level, and we treated hybrid complexes within Abies as single species. We included all tree-like conifer species except for western juniper (Juniperus occidentalis) and yews (Taxus sp.), which have berry-like seed cones on which crossbills are not known to forage. Conifer species were only recorded if they were visible and within 350 m of each survey site, so sites with a wide variety of visibility were surveyed in each region. We categorized each species as dominant when they occupied at least one quarter of the visible landscape. See Appendix B for a full list of conifer species identified.
We scored cone abundance for all conifer species using similar systems to previous cone assessment studies focused on crossbills (Hahn and Schultz, 2012; Bisi et al., 2016) with modifications to increase efficiency (Appendix B). We used binoculars to estimate average cone count for mature trees of each species from a stationary position within 50 m of each survey site. We scored cone crop on a categorical scale from 0 to 6 based on a combination of estimated average number of cones per tree for each species and the maximum number of cones seen on any individual tree. For species with less than five individuals present at a point, we estimated the number of cones on each tree and averaged the counts. For species with more than five individuals at a point, we randomly selected five trees and averaged their counts. To estimate the number of cones on each single tree evaluated, we counted ten cones individually and then extrapolated visually to estimate the total cone count in groups of ten. Cones were counted from a stationary position, so only the cones visible from one side of each tree were counted. We only counted cones that were less than 1 year old, with the exception of cones of hard-coned pine species. However, it was not always possible to confidently separate previous generations of cones between January and May.
2.2 Data analysis
We used Chi-square tests to associate occurrence of each Red Crossbill type with PKC species that were present, dominant or had a new cone crop. Connecting Red Crossbill occurrence directly to individual trees or even particular species is challenging because dense conifer foliage dominates the habitats where crossbills live, which obscured our abilities to watch them forage. Furthermore, crossbills move frequently, so locating specific trees in which they forage can be challenging in remote, heavily forested landscapes, so we focused on describing associations of crossbills with information on the conifers at the study sites. Crossbill numbers varied widely from many locations with zero birds to flocks ranging up to 200 birds, so we simplified crossbill numbers to presence-absence information in our analyses. We evaluated key conifer associations by choosing sites where each conifer was present and asking if the expected Red Crossbill call type was most often associated with those sites or if other crossbill types were more or equally likely to have been encountered. To test for statistical differences, we set the expected values as the proportion of locations where the expected call type occurred, then evaluated if the other call types were significantly different from those values. We used this approach for sites where each PKC was present and where it was considered dominant. We conducted comparisons for all data from the study period and for the period of food scarcity, which we considered to be the cold months at the end of the cone cycle (January-April) (Benkman, 1993; Cornelius and Hahn, 2012). Finally, we evaluated associations of crossbill call type occurrence with new cone crops and larger new cone crops (score 3-6) by comparing occurrence among sites with each of the six categories of cone abundance. We conducted all analyses with JMP (JMP 2023).
3 Results
We conducted 923 surveys between June 2020 and October 2022, 633 of which encountered Red Crossbills (Figure 1). Three call types (2, 3, and 4) occurred in all five survey regions, while two types (5 and 10) were restricted to one or two adjacent ecoregions. We encountered Type 2s on 118 surveys (Figure 3A), with the most observations in the Leeward Cascades (72) and Coast Range (26). We found Type 3s on 365 surveys spread widely throughout all 5 regions (Figure 3B). Type 4s were found on 58 surveys, with most encounters in the Coast Range (31) and Leeward Cascades (21) (Figure 3C). In contrast, type 10s were only found on the Outer Coast (78 surveys) and Type 5s were sparsely distributed only on the Cascade Crest (5 surveys) (Figure 3D).
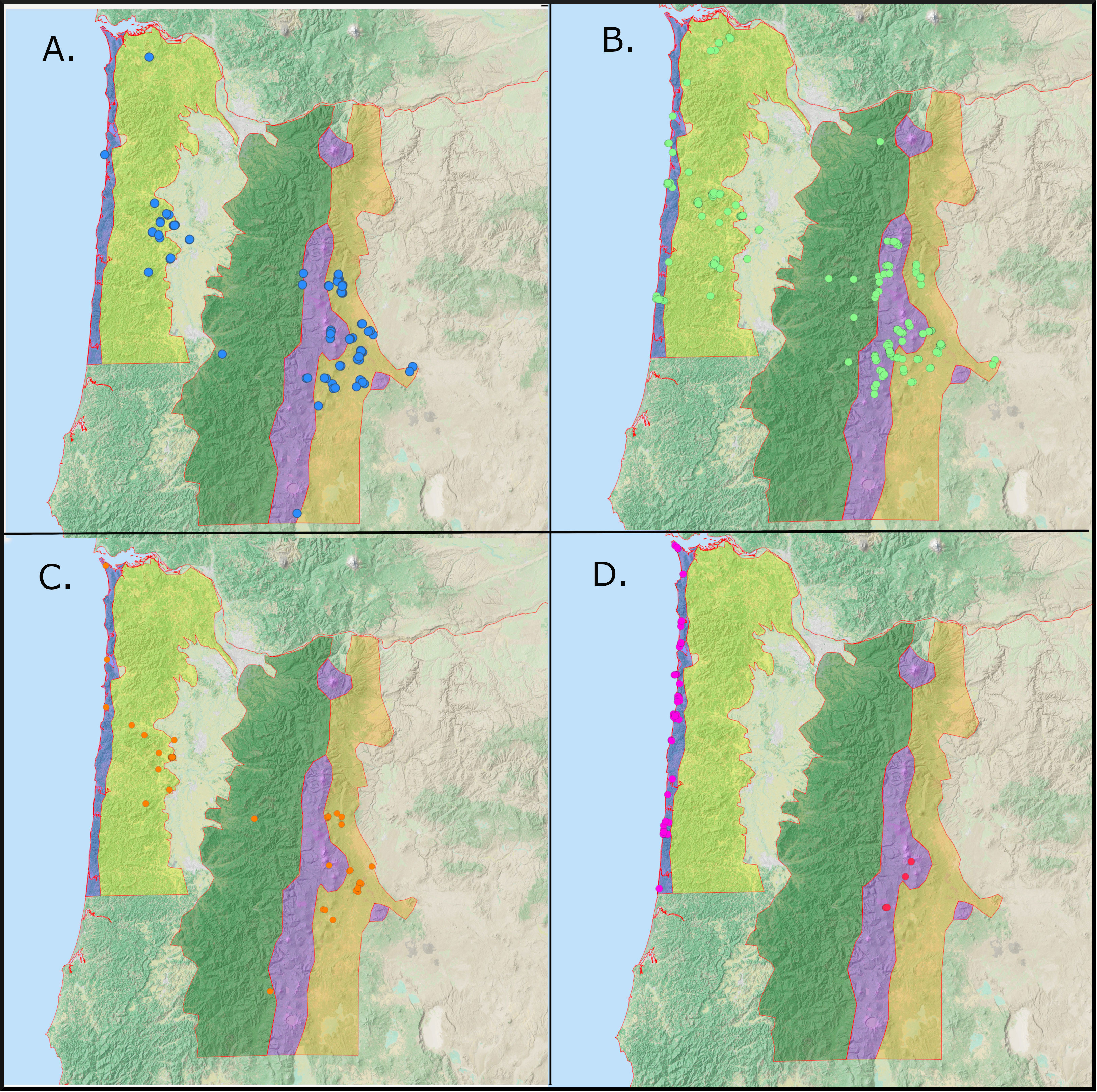
Figure 3 Distribution of Call Types 2 (A), 3 (B), 4 (C), 5 and 10 (D) (Key conifers: ponderosa pine (2), western hemlock (3), Douglas-fir (4), lodgepole pine (5), and Sitka spruce (10)). Each target icon is a point at which the type was encountered. Each location may involve more than one encounter of crossbills. In Panel D, red targets represent type 5 and magenta targets are type 10.
The four frequently detected call types (2, 3, 4, 10) varied considerably in their movements across ecoregions dominated by hard-coned pines (Leeward Cascades), regions dominated by soft-coned conifers (Coast Range and Windward Cascades) and areas where these conifer types significantly overlap (Outer Coast, Cascade Crest). Type 2s were found consistently in the range of their PKC, ponderosa pine, but also irrupted into the Coast Range in the autumn of 2020 and were detected twice near the Outer Coast in the summer of 2022 (Figure 3). Type 3s and Type 4s were found throughout the range of their PKCs (western hemlock and Douglas-fir, respectively), but their presence was not consistent in any habitat or region. Both types were present in the Coast Range in the summer, fall, and winter of 2020-2021. In the summer of 2021, Type 3s were encountered in smaller numbers throughout the range of their PKC, but were found more abundantly breeding elsewhere on the Cascade Crest. In the winter and spring of 2022, Type 3s and Type 4s were located on surveys in the low-elevation ponderosa pine forests of the Leeward Cascades. Type 3s were found in small numbers on the Outer Coast throughout the survey period. Type 4s were generally scarce, but were more concentrated during their 2020 irruption into the Coast Range. Type 10s were much less irruptive and were found consistently and exclusively in the range of Sitka spruce (their PKC) (Figure 3D).
3.1 Conifer associations
When a PKC was present or dominant at a sampling site, the percent of those sites with the presumed call type also present varied substantially (Tables 1, 2). For example, when lodgepole pine was dominant, type 3s were the most frequent type found. When ponderosa pine was dominant, type 2s were most often encountered (47.5%). Type 3s were the most likely type to be encountered at sites where Douglas-fir (44.4%), western hemlock (59.6%), and lodgepole pine (40.6%) were dominant. Types 2, 3, 5, and 10 were found more often at sites where their PKC was dominant compared to those where other key conifers were dominant, although type 3s were encountered at sites where lodgepole pine, ponderosa pine, and Douglas-fir were dominant with similar frequency. Type 4s were found slightly more often at sites where lodgepole pine and ponderosa pine were dominant than sites where Douglas-fir was dominant. When Sitka spruce was present, type 10 crossbills were detected at 51.4% of sites (Table 1) and when it was dominant, the most likely type to be detected was type 10 (63.6% of sites; Table 2). Associations between presence versus dominance of PKCs and call type occurrence were qualitatively very similar (Tables 1, 2).
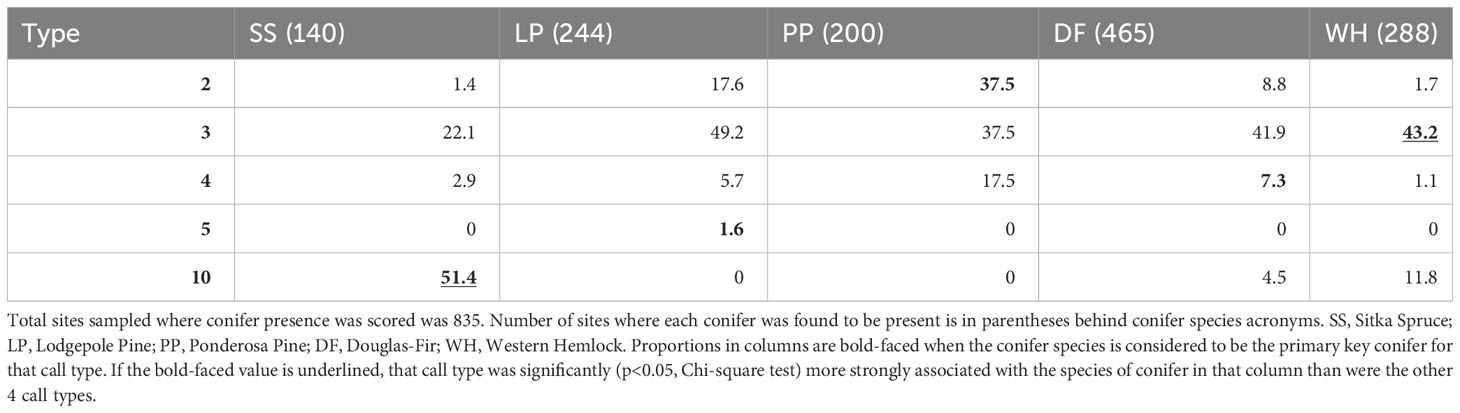
Table 1 Given that a primary key conifer species was present (columns), the percent of those sites where each crossbill call type was encountered is reported.
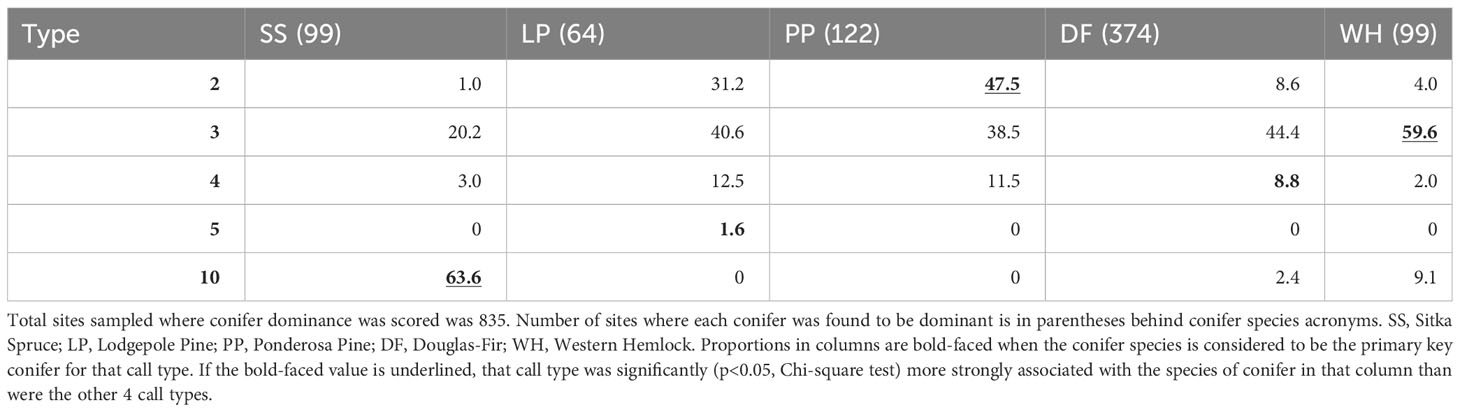
Table 2 Given that a primary key conifer species was dominant (columns), the percent of those sites where each crossbill call type was encountered is reported.
When we restricted analyses to the presumed season of food scarcity (January-April), call types generally showed weaker associations with their PKCs (Tables 3, 4). PKC associations were not significant for Type 2 during this period and were less significant for Type 10s. Type 3s were still the only type likely to be detected where there were western hemlocks, but Type 3s and Type 4s were more likely to be found where there were ponderosa pines and lodgepole pines than where their PKCs were present. Type 5s were not detected during this season.
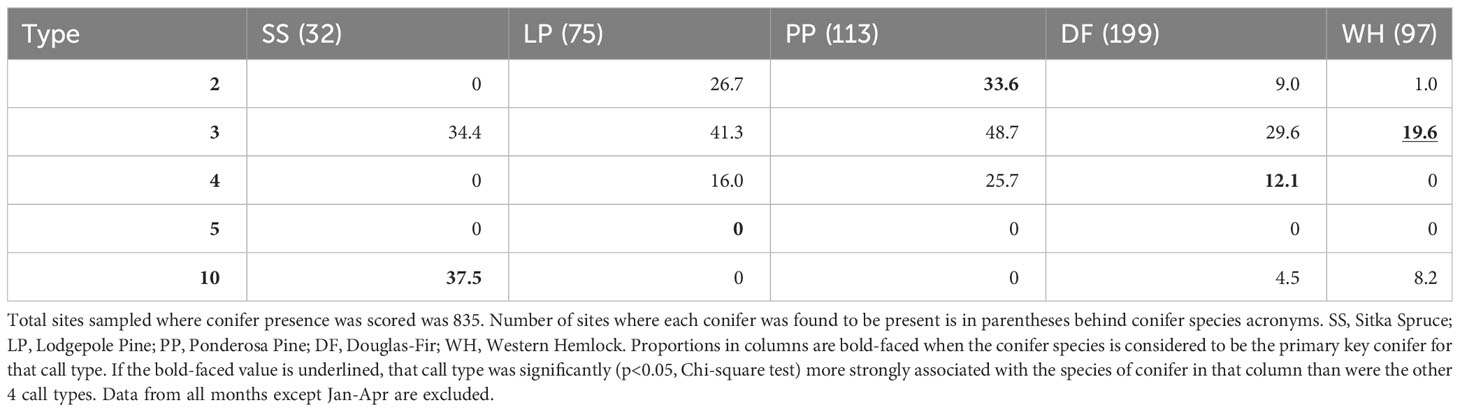
Table 3 Given that a primary key conifer species was present (columns), the percent of those sites where each crossbill call type was encountered during the predicted season of food scarcity from January to April is reported.
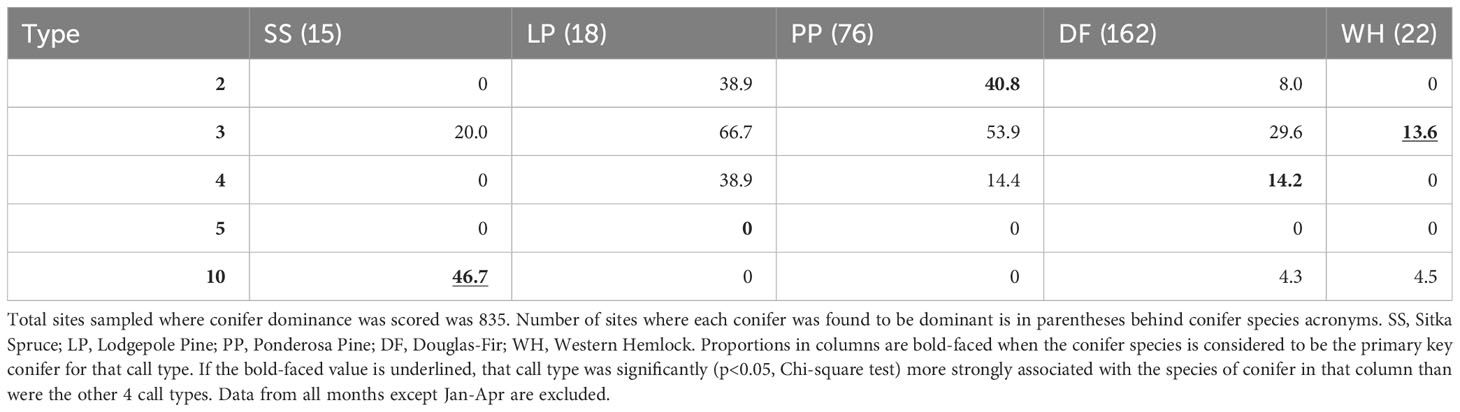
Table 4 Given that a primary key conifer species was dominant (columns), the percent of those sites where each crossbill call type was encountered during the predicted season of food scarcity from January to April is reported.
At locations defined by the presence of each call type instead of the presence or dominance of each conifer species, similar associations were shown (Figures 4, 5). Sitka spruce was present and dominant at over 80 percent of sites where Type 10s were found. Lodgepole pine was the only PKC present or dominant at the few sites where Type 5s were found. Douglas-fir and Ponderosa pine were present and dominant at over half of sites where Type 4s and Type 2s were encountered, respectively. At sites where we found Type 3s, however, Douglas-fir was more likely to be present or dominant than PKC western hemlock. For sites where Types 2, 4, 5, and 10 were encountered, each type’s corresponding PKC was significantly more likely to be the dominant tree species than any other PKC. Douglas-fir was most likely to be dominant by a significant margin at sites where Type 3s were encountered. Types 5 and 10 seemed to show an overwhelming preference for sites where their PKCs were dominant (>70% of sites), while no single conifer was dominant at over 70 percent of sites for Types 2, 3, or 4.
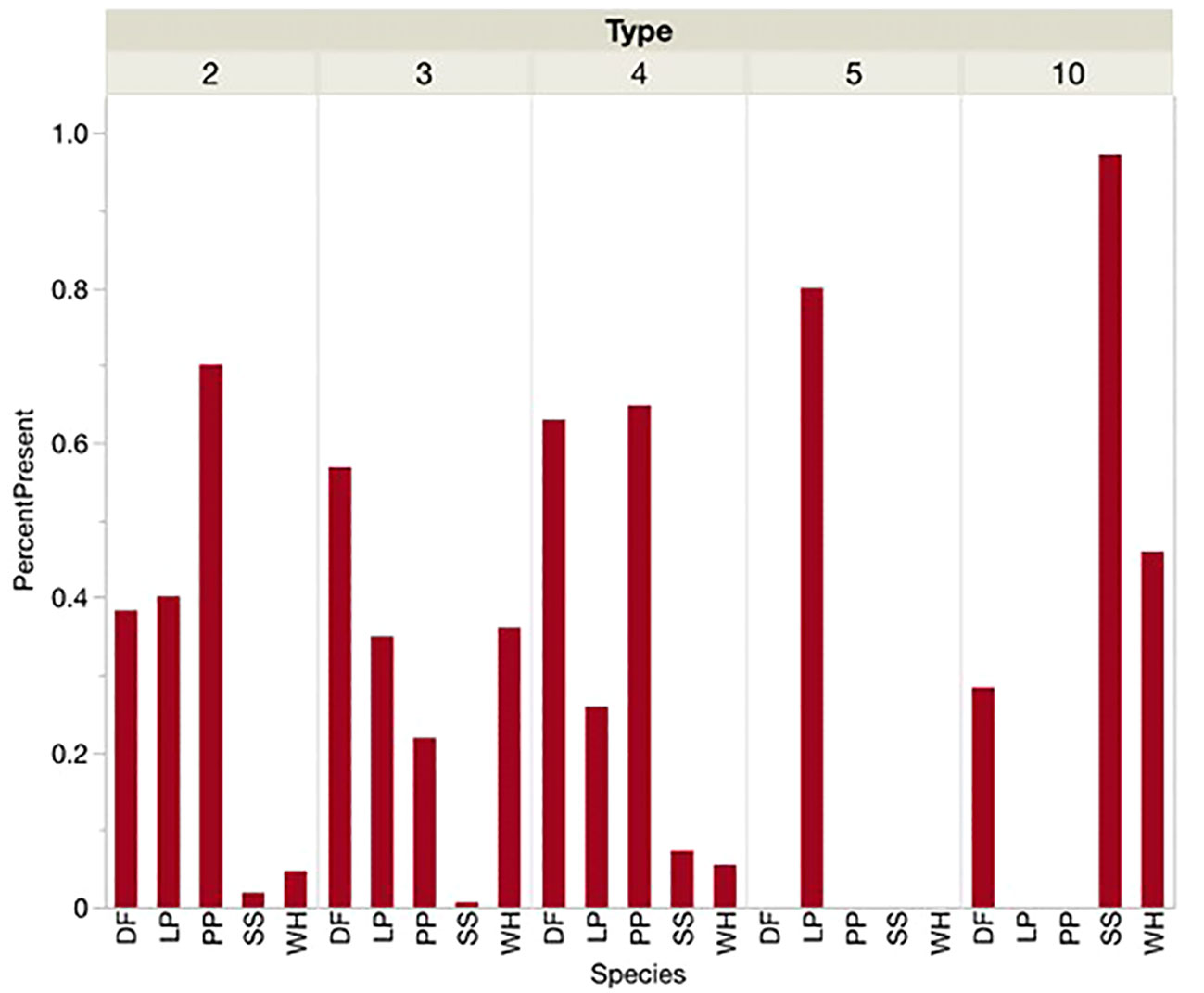
Figure 4 When each Red Crossbill call type was present, the percentage of sites where each conifer species was also present was noted. Conifer species acronyms were DF (Douglas-fir), LP (Lodgepole Pine), PP (Ponderosa Pine), SS (Sitka Spruce) and WH (Western Hemlock).
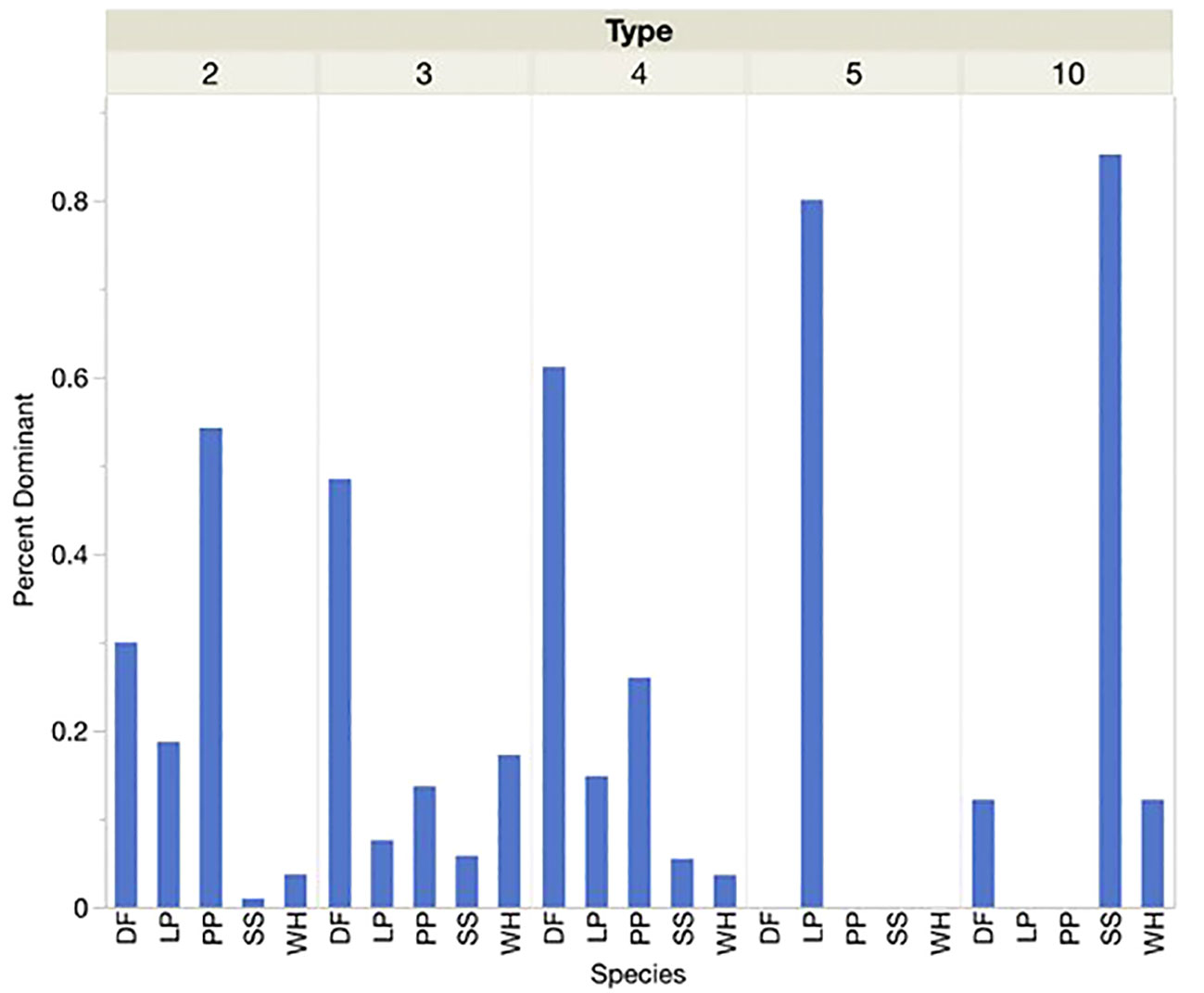
Figure 5 When each Red Crossbill call type was present the percentage of sites where each conifer species was dominant (25% or more of conifers present), was noted. Conifer species acronyms were DF (Douglas-fir), LP (Lodgepole Pine), PP (Ponderosa Pine), SS (Sitka Spruce) and WH (Western Hemlock).
Overall, counts of Red Crossbills were highly variable and that variation produced only a weak association between estimated cone crop sizes and crossbill counts. Patterns of occurrence of call types (presence/absence) when PKCs had new cone crops (categories 1-6 combined) were qualitatively similar to the patterns associated with presence and dominance of the PKCs (Table 5). Analysis of sites with higher cone crops (3-6) did not significantly change this result.
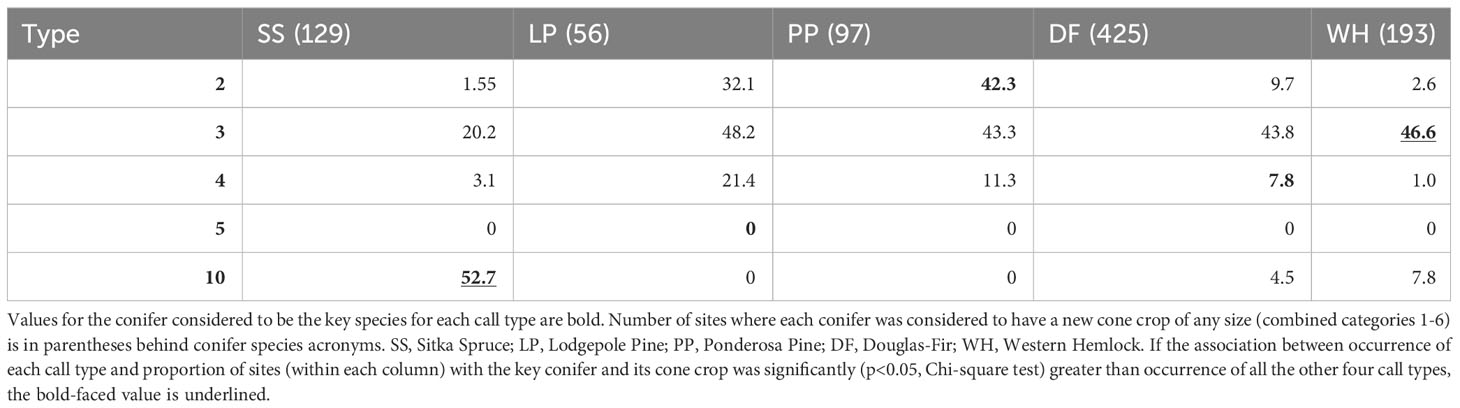
Table 5 Given that a primary key conifer species had new cones (columns), the percent of those sites where each crossbill call type was encountered is reported.
4 Discussion
In our study area, each of four Red Crossbill call types that we frequently encountered showed unique distributional dynamics and conifer associations. Our findings reveal a variety of levels of correspondence between geographic regions, primary key conifer species and crossbill call types. Some types, e.g., types 5 and 10, appeared to be strongly affiliated with a particular ecoregion and conifer species, while the other three types (2, 3 and 5) wandered more widely and occurred frequently in areas lacking their presumed PKC. Our observations that call types 2, 3 and 4 are widespread and associated with both their PKCs and additional tree species suggests potential multi-conifer associations, not reliance on single PKCs. In contrast, type 10 was apparently resident in a narrow band in the Outer Coast ecoregion and strongly associated with Sitka spruce. The patterns we observed suggest that the hypothesis of primary key conifer associations leading to evolutionary specialization of crossbill morphology may be reasonable for some call types but not all of them. Instead, three of the call types we studied appear to associate instead with several ecologically associated conifer species, which suggests regional specialization on sites with multiple food sources is more important than dependence on single conifer species.
Crossbill types with smaller bill morphologies, such as type 3 (the smallest) and type 4 have been thought to specialize on soft-coned conifers due to low foraging efficiency on hard-coned pines (Benkman, 1993; Benkman, 2003). However, our observations indicate that these call types may have broader foraging preferences than we expected. During the two years of our study, all PKCs and two other potentially important conifers (Engelmann spruce and mountain hemlock) in the region showed marked periods of cone abundance and scarcity, which appeared to drive some of the movements of call types across our study area. However, hard-coned lodgepole and ponderosa pines and coastal Sitka spruces showed less pronounced variation in cone abundance. The consistency of cones on these tree species may help explain the apparent residency of type 10s in association with Sitka spruce and the consistent occurrence of type 2s in regions dominated by hard-cones pines. In contrast, Types 3 and 4 were associated with a variety of soft- and hard-coned conifers and were not consistently present in any ecoregion, apparently following abundant cone crops on a variety of conifers. Type 4s were generally found either in areas with large cone crops on their PKC, Douglas-fir, or in association with lodgepole and ponderosa pines, while type 3s showed the most diverse preferences, associating with significant cone crops on western hemlock (PKC), mountain hemlock, Douglas-fir, Sitka spruce, and Engelmann spruce. In early 2022, types 3 and 4 were notably observed in high numbers in forests of lodgepole and ponderosa pines. During this period, we found Type 3s foraging on ponderosa pine seeds, an observation which was independently confirmed in the same season (Cornelius, 2023). These observations contrast with the expectation that types 3 and 4 are specialists which would struggle to forage on hard-coned pines. However, dramatic shifts in conifer species selection are consistent with the concept of Red Crossbills as rich patch exploiters which rely on biogeographic networks of resources to maximize the reliability of foraging opportunities (Cornelius et al., 2013).
Past treatments of call type ecology have often focused on the importance of resource scarcity in the evolution of specialization (Benkman, 1993; Benkman, 2003; Kelsey et al., 2008), but we did not find greater specialization in expected seasons of low seed abundance. Assuming that late winter and early spring represents a period of resource scarcity due to dwindling conifer seeds and low temperatures, two irruptions we observed showed especially clear departures from this prediction. In the winter and spring of 2021, Type 2s irrupted into the Coast Range, where PKC ponderosa pine is rare on the landscape and seldom produces significant cones. Later, in the winter and spring of 2022, Type 3s and 4s irrupted extensively into the low-elevation East Cascades, where their PKCs and all soft-coned conifers are rare. Moreover, types 2, 3, and 4 are known to irrupt into eastern North America in fall, winter, and spring, where their PKCs are absent (Young, 2011), suggesting that greater specialization is unlikely during this period. These patterns raise questions about whether the winter and early spring, when breeding often occurs, is a true season of scarcity. The hypothesis that crossbills are rich patch opportunists suggests that they generally have access to abundant resources throughout the annual cycle and can limit breeding behavior under resource scarcity and high physiological demands, exemplified by an autumn pause in breeding even when resources are abundant (Cornelius et al., 2013). Red Crossbill fat deposits peak in May and June preceding regular migratory movements (Cornelius and Hahn, 2012), highlighting late spring and early summer as a potentially highly demanding period, but this may coincide with higher insect consumption (Kelsey et al., 2008). Generally, erratic and opportunistic movements of Type 3s and Type 4s throughout the annual cycle in our study did not show more consistent associations with key conifers in any of the periods between late winter and early summer.
Our data suggest that foraging preferences affect call type distributions, with some types more strongly affected by single versus multi-species conifer associations. The tight affinity between Type 10s and Sitka spruce despite the presence of other abundant conifers on the Oregon Coast shows strong evidence that the key conifer hypothesis can explain the distribution of resident or near-resident Crossbill populations. Similar patterns may be present in Cassia Crossbills (Loxia sinescurius) in Idaho and Type 8 Red Crossbills in Newfoundland, both associated with semi-serotinous tree species that bear reliable seed crops (Benkman, 1989; Benkman et al., 2009). Similarly, Type 4 seems to show a geographic affinity for the east slope of the Oregon Cascades, even though it had no overriding association with a single conifer. Type 3s continually shifted both their geographic and conifer associations, with high concentrations found from the Sitka spruce forests of the Outer Coast to the ponderosa pine forests of the East Cascades. This apparent generalist strategy highlights the potential importance of diverse networks of conifer ecosystems in the adaptive radiation of call types.
Ultimately, a multitude of selective forces act on Red Crossbills, and it is possible (or perhaps probable) that geography and resource specialization have worked in tandem to shape their evolution (Benkman, 2003; Kelsey et al., 2008; Cornelius and Hahn, 2012). Our results add to a growing body of evidence that Red Crossbill call types are each associated with a unique network of ecological resources which provides food security over broad geographic scales (Benkman, 1999; Cornelius et al., 2013; Martin et al., 2019). Call types in our data were distinguishable based on the set of conifers with which they were associated but varied in the number of tree species they favored. These discrete and consistent foraging preferences suggest ecological drivers for call type divergence and are consistent with previous findings that crossbills can specialize both on single, reliable conifer species or on regions of conifer diversity Benkman (1987). While our study is limited by its scope in space and time, the conifer associations observed may have geographic implications in a broader context. Type 3 was the only type to associate with western hemlock, a small-coned conifer restricted to moist, coastally influenced forests in the Pacific Northwest (Meyers et al., 2015). In contrast, Types 2 and 4 were only associated with conifers found throughout the interior west and into the Rocky Mountains. These patterns hint at potential core geographic areas proposed by Kelsey (Kelsey et al., 2008). Further study across broader geographic regions are needed to determine if our conclusions apply more widely across the ranges of the key conifer species. Crossbill call types rely on diverse resource networks and have the potential to evolve rapidly. Thus, they may be interesting indicator species that reveal biotic responses to structural and functional changes in the environment. Rangewide, multi-year studies of foraging, assortative mating, and genetics are needed to further elucidate the evolutionary origins of Red Crossbill call types.
Data availability statement
The raw data supporting the conclusions of this article will be made available by the authors, without undue reservation.
Ethics statement
Ethical approval was not required for the study involving animals in accordance with the local legislation and institutional requirements because This was a purely observational study.
Author contributions
CC: Conceptualization, Formal Analysis, Investigation, Methodology, Visualization, Writing – original draft, Writing – review & editing. WR: Conceptualization, Formal Analysis, Methodology, Visualization, Writing – review & editing. MY: Conceptualization, Methodology, Writing – review & editing.
Funding
The author(s) declare financial support was received for the research, authorship, and/or publication of this article. Funding was provided by the Robinson Ornithology Fellowship to CC and the Mace Endowed Professorship to WR.
Acknowledgments
Logistical support from the Finch Research Network and the Cornell Lab of Ornithology was necessary for the completion of this project. Tim Spahr assisted in identifying challenging recordings. Courtney Kelly Jett contributed a multitude of audio recordings (including the type 4 and 5 recordings for Figure 2) and assisted with field surveys. Jamie Cornelius and Aaron Liston provided guidance on the ecology of crossbills and conifers, respectively. Evan Centanni contributed knowledge of GIS software to assist in the mapping process. We thank all contributors who entered crossbill recordings into the eBird citizen science database, helping us locate call types efficiently.
Conflict of interest
The authors declare that the research was conducted in the absence of any commercial or financial relationships that could be construed as a potential conflict of interest.
The author(s) declared that they were an editorial board member of Frontiers, at the time of submission. This had no impact on the peer review process and the final decision.
Publisher’s note
All claims expressed in this article are solely those of the authors and do not necessarily represent those of their affiliated organizations, or those of the publisher, the editors and the reviewers. Any product that may be evaluated in this article, or claim that may be made by its manufacturer, is not guaranteed or endorsed by the publisher.
Supplementary material
The Supplementary Material for this article can be found online at: https://www.frontiersin.org/articles/10.3389/fbirs.2024.1321583/full#supplementary-material
References
Anderson S. A. S., Weir J. T. (2022). The role of divergent ecological adaptation during allopatric speciation in vertebrates. Science 378, 1214–1218. doi: 10.1126/science.abo7719
Benkman C. W. (1987). Food profitability and the foraging ecology of crossbills. Ecol. Monogr. 57, 251–267. doi: 10.2307/2937083
Benkman C. W. (1989). On the evolution and ecology of island populations of crossbills. Evolution 43, 1324–1330. doi: 10.2307/2409369
Benkman C. W. (1993). Adaptation to single resources and the evolution of crossbill (Loxia) diversity. Ecol. Monogr. 63, 305–325. doi: 10.2307/2937103
Benkman C. W. (1999). The selection mosaic and diversifying coevolution between crossbills and lodgepole pine. Am. Nat. 153, S75–S91. doi: 10.1086/303213
Benkman C. W. (2003). Divergent selection drives the adaptive radiation of crossbills. Evolution 57, 1176–1181. doi: 10.1111/j.0014-3820.2003.tb00326.x
Benkman C. W. (2007). Red crossbill types in Colorado: their ecology, evolution, and distribution. Colo. Birds 41, 153–163.
Benkman C. W., Smith J. W., Keenan P. C., Parchman T. L., Santisteban L. (2009). A new species of the red crossbill (Fringillidae: loxia) from idaho. Condor 111, 169–176. doi: 10.1525/cond.2009.080042
Bisi F., Hardenberg J. V., Berolino S., Wauters L. A., Imperio S., Preatoni D. G., et al. (2016). Current and future conifer seed production in the Alps: testing weather factors as cues behind masting. Eur. J. For. Res. 135, 743–754. doi: 10.1007/s10342-016-0969-4
Cornelius J. M., Hahn T. P. (2012). Seasonal pre-migratory fattening and increased activity in a nomadic and irruptive migrant, the Red Crossbill Loxia curvirostra. Ibis 154, 693–702. doi: 10.1111/j.1474-919X.2012.01266.x
Cornelius J. M., Watts H. E., Dingle H., Hahn T. P. (2013). Obligate versus rich patch opportunism: Evolution and endocrine mechanisms. Gen. Comp. Endocrinol. 190, 76–80. doi: 10.1016/j.ygcen.2013.04.003
eBird (2023). eBird: An Online Database of Bird Distribution and Abundance. (Ithaca, NY: Cornell Lab of Ornithology).
Foote A. D. (2012). Investigating ecological speciation in non-model organisms: a case study of killer whale ecotypes. Evol. Ecol. Res. 14, 447–465.
Foote A. D., Vijay N., Ávila-Arcos M. C., Baird R. W., Durban J. W., Fumagalli M., et al. (2016). Genome-culture coevolution promotes rapid divergence of killer whale ecotypes. Nat. Commun. 7, 11693. doi: 10.1038/ncomms11693
Ford H. A., Parkin D. T., Ewing A. W. (1973). Divergence and evolution in Darwin’s finches. Biol. J. Linn. Soc 5, 289–295. doi: 10.1111/j.1095-8312.1973.tb00707.x
Gavrilets S., Vose A. (2007). Case studies and mathematical models of ecological speciation. 2. Palms on an oceanic island. Mol. Ecol. 16, 2910–2921. doi: 10.1111/j.1365-294X.2007.03304.x
Gavrilets S., Vose A., Barluenga M., Salzburger W., Meyer A. (2007). Case studies and mathematical models of ecological speciation. 1. Cichlids in a crater lake. Mol. Ecol. 16, 2893–2909. doi: 10.1111/j.1365-294X.2007.03305.x
Groth J. G. (1993a). Call matching and positive assortative mating in red crossbills. Auk 110, 398–401.
Groth J. G. (1993b). Evolutionary Differentiation in Morphology, Vocalizations, and Allozymes Among Nomadic Sibling Species in the North American Red Crossbill (Loxia curvirostra) Complex Vol. 127 (Oakland, CA: Univ of California Press).
Hahn T., Schultz E. (2012). How conifer diversity and availability influence the abundance and biology of the red crossbill. UW-Natl. Park Serv. Res. Stn. Annu. Rep. 35, 3–13.
Hendry A. P. (2009). Ecological speciation! Or the lack thereof? This Perspective is based on the author’s J.C. Stevenson Memorial Lecture delivered at the Canadian Conference for Fisheries Research in Halifax, Nova Scotia, January 2008. Can. J. Fish. Aquat. Sci. 66, 1383–1398. doi: 10.1139/F09-074
Janz N., Nyblom K., Nylin S. (2001). Evolutionary dynamics of host-plant specialization: A case study of the tribe nymphalini. Evolution 55, 783–796. doi: 10.1554/0014-3820(2001)055[0783:EDOHPS]2.0.CO;2
Kelsey T. R., Cornelius J. M., Hahn T. P. (2008). “Biogeography, foraging ecology, and population dynamics of red crossbills in North America,” in Chapter 1: Geographical Patterns of Abundance and Morphology in a Nomadic Seed Specialist, the Red Crossbill (Davis, CA: University of California, Davis).
Kelsey T. R., Hahn T. P. (2008). “Biogeography, foraging ecology, and population dynamics of red crossbills in North America,” in Chapter 2: Local habitat and diet selection in a nomadic conifer specialist, the Red Crossbill (Loxia curvirostra) (Davis, CA: University of California, Davis).
Lamichhaney S., Berglund J., Almén M. S., Maqbool K., Grabherr M., Martinez-Barrio A., et al. (2015). Evolution of Darwin’s finches and their beaks revealed by genome sequencing. Nature 518, 371–375. doi: 10.1038/nature14181
Martin R., Rochefort J., Mundry R., Segelbacher ,. G. (2019). Delimitation of call types of Red Crossbill (Loxia curvirostra) in the Western Palearctic. Écoscience 26, 177–194. doi: 10.1080/11956860.2018.1564483
Martin R., Rochefort J., Mundry R., Segelbacher G. (2020). On the relative importance of ecology and geographic isolation as drivers for differentiation of call types of red crossbill Loxia curvirostra in the Palearctic. J. Avian Biol. 51 267–284. doi: 10.1111/jav.02358
Meyers S. C., Jaster T., Mitchell K. E., Hardison L. K. (2015). “Flora of oregon,” in Volume 1: Pteridophytes, Gymnosperms, and Monocots (Fort Worth, TX: Botanical Research Institute of Texas Press).
Newton I. (2006). Movement patterns of Common Crossbills Loxia curvirostra in Europe. Ibis 148, 782–788. doi: 10.1111/j.1474-919X.2006.00585.x
Pilot M., Dahlheim M. E., Hoelzel A. R. (2010). Social cohesion among kin, gene flow without dispersal and the evolution of population genetic structure in the killer whale (Orcinus orca). J. Evol. Biol. 23, 20–31. doi: 10.1111/j.1420-9101.2009.01887.x
Sadedin S., Hollander J., Panova M., Johannesson K., Gavrilets S. (2009). Case studies and mathematical models of ecological speciation. 3: Ecotype formation in a Swedish snail. Mol. Ecol. 18, 4006–4023. doi: 10.1111/j.1365-294X.2009.04320.x
Sewall K., Kelsey R., Hahn T. P. (2004). Discrete variants of evening grosbeak flight calls. Condor 106, 161–165. doi: 10.1093/condor/106.1.161
Teitelbaum C. S., Mueller T. (2019). Beyond migration: causes and consequences of nomadic animal movements. Trends Ecol. Evol. 34, 569–581. doi: 10.1016/j.tree.2019.02.005
Young M. A., Blankenship K., Westphal M., Holzman S. (2011). Status and distribution of Type 1 Red Crossbill (Loxia curvirostra): an Appalachian Call Type? North Am. Birds 65, 554–561.
Young M. A., Spahr T. (2017) Crossbills of North America: Species and Red Crossbill Call Types. Available at: https://ebird.org/ebird/news/crossbills-of-north-america-species-and-red-crossbill-call-types/.
Keywords: Red Crossbill, key conifer, resource specialization, call type, conifer, Oregon, ecotype
Citation: Centanni C, Robinson WD and Young MA (2024) Is resource specialization the key?: some, but not all Red Crossbill call types associate with their key conifers in a diverse North American landscape. Front. Bird Sci. 3:1321583. doi: 10.3389/fbirs.2024.1321583
Received: 14 October 2023; Accepted: 19 January 2024;
Published: 05 February 2024.
Edited by:
Piotr Tryjanowski, Poznan University of Life Sciences, PolandReviewed by:
Michal Budka, Adam Mickiewicz University in Poznań, PolandGianpasquale Chiatante, University of Tuscia, Italy
Copyright © 2024 Centanni, Robinson and Young. This is an open-access article distributed under the terms of the Creative Commons Attribution License (CC BY). The use, distribution or reproduction in other forums is permitted, provided the original author(s) and the copyright owner(s) are credited and that the original publication in this journal is cited, in accordance with accepted academic practice. No use, distribution or reproduction is permitted which does not comply with these terms.
*Correspondence: Caleb Centanni, Y2FsZWJAY2VudGFubmkuY29t