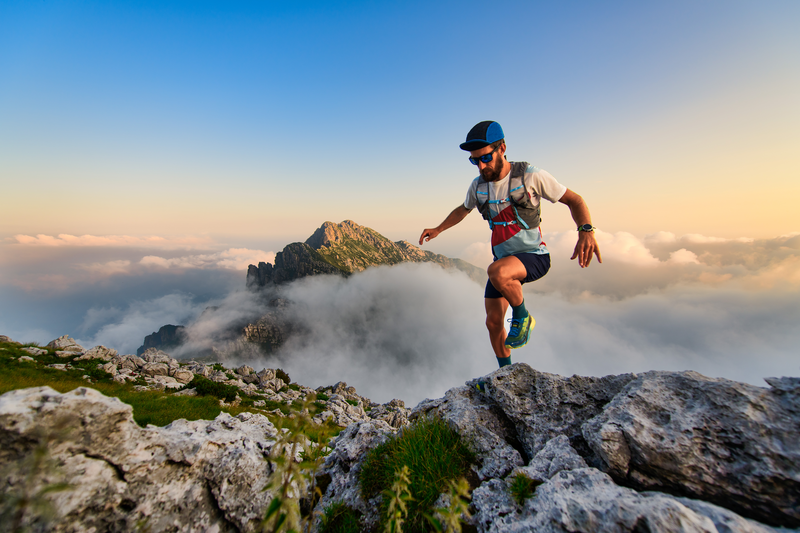
94% of researchers rate our articles as excellent or good
Learn more about the work of our research integrity team to safeguard the quality of each article we publish.
Find out more
MINI REVIEW article
Front. Biomater. Sci. , 10 January 2024
Sec. Delivery Systems and Controlled Release
Volume 2 - 2023 | https://doi.org/10.3389/fbiom.2023.1336842
This article is part of the Research Topic Women in Biomaterials Science 2023 View all 12 articles
Modulating the immune system using engineered materials is an emerging strategy to combat bacterial infections. Bacteria adopt immune evasion strategies to ensure their survival, ultimately leading to persistence and recurrence of infections. With a rise in antimicrobial resistance and a decrease in antibiotic efficacy, host-directed therapies using immunomodulatory biomaterials are a promising approach to infection management. Here, we review biomaterials developed to modulate the immune system, with an emphasis on innate immunity. We specifically highlight the recent implementation of functionalized surfaces for immunomodulation, including metal ion releasing coatings, stimuli-responsive polymeric coatings, and interleukin releasing surfaces. We also describe immunomodulatory nanoparticles, including lipid-based nanoparticles, biomimetic nanoparticles, and inorganic nanocarriers. Lastly, we explore immunomodulatory hydrogels used primarily for the treatment of wound infections. These approaches offer new strategies for treating bacterial infections and enhancing existing antimicrobial approaches, all while avoiding complications associated with antimicrobial resistance.
Bacterial infections are among the leading causes of death worldwide. In 2019, 1 in 8 deaths was caused by these infections, leading to 7.7 million deaths globally (Ikuta et al., 2022; Murray et al., 2022). The immune system, comprising of adaptive and innate immunity, serves as the primary host-defense mechanism against bacterial pathogens. The adaptive immune response eliminates pathogens by enabling the recognition of “non-self” antigens, mediated by antigen-specific T cells and B cells (Marshall et al., 2018). The innate response is a non-specific first line of defense that involves the rapid recruitment of phagocytic cells (e.g., macrophages, neutrophils, dendritic cells), basophils, eosinophils, and mast cells to the site of infection. Most innate immune effector cells possess pattern-recognition receptors (PRR) that recognize pathogen-associated molecular patterns (PAMPs) (Marshall et al., 2018; Paludan et al., 2020). Upon PAMP recognition, the various PRR families activate inflammatory signaling cascades, including the secretion of cytokines. Hematopoietin (Class I) cytokines, interferons (INFs), and tumor necrosis factor (TNF) cytokine families are key regulators of inflammation (Kany et al., 2019; Cho, 2022). Macrophages are one of the essential innate immune cells. The undifferentiated M0 macrophages have the ability to polarize into pro-inflammatory, M1, and anti-inflammatory, M2 macrophages (Yao et al., 2019). The ability of macrophages to phagocytose and kill bacteria reduces the risk of infection (Li et al., 2019).
In addition to evading antibiotic mediated clearance, bacteria can manipulate innate immunity to promote infections (Diacovich and Gorvel, 2010). For example, they can modulate apoptosis/autophagy by altering apoptotic signaling pathways, interfere with Toll-like receptors (TLRs) to dampen inflammation, and they can alter reactive oxygen species (ROS) and reactive nitrogen intermediates (RNI) by producing ROS and RNI scavengers that ultimately suppresses innate immune activation (Finlay and McFadden, 2006; Diacovich and Gorvel, 2010; Chiang et al., 2018). Bacterial biofilms can also activate or impair macrophage activity, leading to macrophage secretion of pro-inflammatory and anti-inflammatory cytokines, and alter genetic switches to affect the activity of immune cells (González et al., 2018; Le et al., 2018; Kaya et al., 2020; Guzmán-Soto et al., 2021).
As the efficacy of existing antibiotics is rapidly declining in the face of rising antimicrobial resistance, there is growing interest in host-directed approaches against infections (Kalelkar et al., 2021; Super et al., 2021). With numerous advances spanning decades, the versatility of biomaterials enables the development of unique approaches to regulate the immune system with the potential to combat bacterial infections (Figure 1). Immunomodulatory biomaterials have been explored for a variety of disease states, with cancer and viral infections having garnered the greatest attention (Backlund et al., 2023; Yousefpour et al., 2023); the role of these materials in bacterial infections has been less explored. These materials can have a tremendous impact in combating bacterial infections and improving outcomes following these infections. Here, we explore biomaterials approaches taken for immunomodulation to treat bacterial infections, with a primary focus on the innate immune response. Specifically, we describe recent strategies involving functionalized surfaces, nanoparticle therapies, and hydrogels.
FIGURE 1. Biomaterials approaches to modulate the innate immune system and combat bacterial infections. Various biomaterial strategies have been used to modulate host immunity ranging from functionalized surfaces to nanoparticles and hydrogels, which are discussed in this article. Immune cells that are often the target of this biomaterials-mediated immune modulation, include macrophages (M1 and M2 polarization), neutrophils, and dendritic cells. The inflammatory response during a bacterial infection can be investigated by examining the secretion of pro-inflammatory and anti-inflammatory factors, including cytokines. Some of the common factors studied are shown in this figure (interleukin (IL), interferon-gamma (INF-γ), tumor necrosis factor-alpha (TNF-α), neutrophil elastase myeloperoxidase (MPO)). Created with BioRender.com.
Surface functionalization strategies, including the application of biomaterials as surface coatings, can serve as a host immunomodulatory approach to mitigate bacterial infections, particularly implant-associated infections. These infections involve the attachment of bacteria on implant surfaces and eventual biofilm formation (Wang and Shukla, 2022; Deusenbery et al., 2023). Many strategies including metal ion releasing coatings, stimuli-responsive coatings, and interleukin releasing surfaces have shown great promise and are described here.
Copper (Cu) is among the most common elements integrated in metallic surface coatings for immunomodulation. It is involved in maintaining immune function by inducing macrophage polarization to an M1 phenotype (Huang et al., 2018; Wang et al., 2021; Huo et al., 2023). Cu also contributes to the generation of endogenous and exogenous ROS, enhancing bacterial killing (Li et al., 2016; Huang et al., 2019). In one example aimed at trapping and killing methicillin-resistant Staphylococcus aureus (MRSA) (a common source of implant-associated infections), Liu et al. developed a porous Cu-nanoparticle coated sulfonated polyetheretherketone (SPEEK) orthopedic implant (Liu et al., 2019). They found that the surface functionalized implant served as an immunomodulator by promoting M1 macrophage polarization. In vitro incubation of murine macrophage cell line, RAW264.7, on the orthopedic implants with Cu functionalization revealed higher levels of inflammatory cytokine secretion (e.g., TNF-α and IL-6) compared to polyetheretherketone (PEEK) and SPEEK without Cu functionalization. Similarly, in vitro co-culture of RAW264.7 macrophages and MRSA exhibited greater macrophage phagocytic ability of MRSA compared to PEEK and SPEEK without Cu functionalization. Huang et al. developed a hybrid metal/ceramic coating on a titanium (Ti) substrate with micro- and nanoscale topographical features that incorporated Cu2+ (Huang et al., 2018). This surface coating was found to exhibit immunomodulatory properties, including M1 macrophage polarization indicated by increased production of IL-6 and inhibition of anti-inflammatory cytokines, IL-4 and IL-10, compared to non-Cu containing surfaces. Incubation of RAW264.7 macrophages on the Cu2+ containing surfaces with S. aureus led to an increase in macrophage phagocytic activity and enhanced bacterial killing after being phagocytosed in comparison to non-Cu containing surfaces.
Immunomodulatory coatings with metal ions have been investigated for treatment of osteomyelitis. One example consisted of using PEEK implants coated with Cu2+-chelated polydopamine to modulate inflammation (Lyu et al., 2022). RAW264.7 cells incubated with the Cu-doped implants exhibited upregulation of pro-inflammatory genes (inducible nitric oxide synthase (iNOS) and TNF). Applying these materials in a rat implant MRSA osteomyelitis model showed a 50% decrease in bacterial burden along with mild inflammation for the Cu-containing implant compared to the PEEK-only implant. In another study aimed at treatment of osteomyelitis, Li et al. developed a cobalt (Co) doped titanium dioxide (TiO2) semiconductor-based Ti implant coating to interrupt extracellular and intracellular proton transfer, while also releasing Co2+ ions that enhance the innate immune response via M1 macrophage polarization (Li et al., 2019). The Co-containing implants significantly reduced bacterial adhesion to the implant compared with non-Co doped implants in a rat femoral MRSA osteomyelitis model.
Polymeric coatings, comprised of both synthetic and biologically-derived polymers, generally offer broad physicochemical tunability, can serve as a depot for various bioactive components, and can be rendered responsive to intrinsic and extrinsic stimuli. For example, photothermally responsive polymeric coatings have been developed using photoresponsive polymers and/or nanocarriers for immunomodulation. In an attempt to eliminate bacterial biofilms, Ding et al. coated a polydopamine-modified Ti substrate with a hyaluronic acid/gelatin hydrogel coating loaded with a composite consisting of zeolitic imidazolate framework-90, cerium oxide (CeO2) nanoparticles, and bismuth (Bi) nanoparticles (Ding et al., 2022). The hydrogel coating provided an anti-adhesive surface, while the Bi nanoparticles were included as the near infrared red (NIR)-responsive photothermal agent, and both the Bi and CeO2 nanoparticles from the composite served as ROS scavengers (Figures 1A, 2). In a mouse femoral S. aureus implant infection model, Ti implants coated with the composite hydrogel led to an ∼90% or greater reduction in S. aureus colonies in both the medullary cavity and on the Ti implant upon NIR irradiation compared to a Ti implant alone; use of the composite hydrogel coated implant also showed increased ROS scavenging (Figures 1B, C, 2). Similarly, ROS-scavenging coating formulations including nitric oxide releasing polymer coatings have been developed to modulate bacteria-induced inflammation (Pant et al., 2018; Li et al., 2020; Sapkota et al., 2023).
FIGURE 2. Immunomodulatory biomaterials for the treatment of bacterial infections. (1) Near infrared (NIR)-responsive polymer-based coatings: (1A) Schematic of immunomodulatory and antibacterial coating for the treatment of implant-associated infections. (1B) Quantification of the antibacterial efficacy of uncoated titanium (Ti) implants, Ti implants coated with hyaluronic acid/gelatin hydrogels, and Ti implants coated with these hydrogels loaded with bismuth (Bi) nanoparticles, zeolitic imidazolate framework-90 (ZIF-90), and cerium oxide (CeO2) nanoparticles with and without NIR irradiation in a mouse femoral Staphylococcus aureus implant infection model. (1C) Anti-inflammatory effect of Ti-based implants assessed via fluorescence staining of intracellular reactive oxygen species (ROS) (red indicates generation of ROS) (adapted with permission from Ding et al. (2022). Copyright 2023 American Chemical Society). (2) Immunomodulatory lipid-based nanoparticles: (2A) Schematic illustrating the fusogenic nanparticle formulation and proposed macrophage uptake mechanism. (2B) S. aureus colony enumeration from healthy and infected lungs upon nanoparticle treatment (dashed line represents the average CFU from healthy mice) (adapted with permission from Kim et al. (2018). Copyright 2018; Springer Nature). (3) Inorganic nanocarriers to combat bacterial infections: (3A) Schematic of nano neuro-immune blockers (NNIBs). Top shows fabrication and implementation of the glioma cell membranes on gold-silver nanocages. Bottom depicts the proposed mechanism of action to neutralize Streptococcus pyogenes infections. (3B) Quantification of neutrophil recruitment to the site of infection after treatment with NNIBs or non-membrane coated nanocage core (GSNCs) and NIR irradiation (adapted with permission from Zhao et al. (2019). Copyright 2019 American Chemical Society). (4) Immunomodulatory hydrogel wound dressing: (4A) Schematic of the extracellular matrix mimetic hydrogels. (4B) Representative images of methicillin-resistant S. aureus (MRSA) biofilms upon treatment with the hydrogel (GM-P@HA-P) and a chitosan (CS) hydrogel control. Top shows the biofilms after laser scanning confocal microscopy (live bacteria = green; membrane damaged bacteria = red). Bottom shows scanning electron microscopy images of these biofilms. (4C) M2 polarization of RAW264.7 macrophages upon treatment with the immunomodulatory hydrogel. Laser scanning confocal microscopy images of the CD206 surface marker for M2 polarization in controls stimulated with or without IL-4 and macrophages treated with hydrogels. (From Liu et al. (2022). ⓒ The Authors, some rights reserved; exclusive licensee AAAS. Distributed under a CC BY-NC 4.0 license http://creativecommons.org/licenses/by-nc/4.0/”. Reprinted with permission from AAAS).
In another study by Ding et al., an antibiofouling polymeric coating of konjac gum and gelatin served as a carrier for self-assembled nanoparticles consisting of a hydrophobic tyrosine core and a hydrophilic tannic acid (TA) outer shell (Ding et al., 2023). The TA-derived nanocarrier exhibited NIR-responsive photothermal properties; upon NIR-irradiation moderate hyperthemia was observed along with bacterial cell membrane damage in vitro. Ti implants functionalized with this photothermally responsive coating were examined in a rat implant-associated S. aureus infection model, inflammatory cell infiltration of the tissue surrounding the implant showed a significant reduction in bacteria and neutrophils for irradiated coated implants compared to non-coated Ti implants. Further, immunofluorescent staining of macrophages in regions surrounding the implant 7 days post-infection confirmed that the coating had the ability to modulate inflammation by reversing M1 macrophages to an M2 phenotype, not observed for the non-coated Ti implants.
Given their prominent role in inflammation, several strategies for antibacterial implant surface functionalization have directly incorporated either pro-inflammatory (e.g., IL-23 and IL-12) or anti-inflammatory (e.g., IL-4) cytokines (He et al., 2021; Lian et al., 2022; Pizarek et al., 2023). In one such example, He et al. designed a dual drug release system that eliminates bacteria, while also mitigating the pro-inflammatory response that occurs during this process. The drug delivery system comprised of IL-4 loaded TiO2 nanotubes coated with a catechol-modified methacrylated gelatin hydrogel containing calcium peroxide (CaO2) nanoparticles, which was applied to a Ti implant surface (He et al., 2021). CaO2 nanoparticles undergo rapid degradation in acidic infection environments to yield hydrogen peroxide (H2O2); this ROS production, while eliminating bacterial pathogens, can also cause an undesirable increase in pro-inflammatory processes. IL-4 release from the coating helps mitigate inflammation by modulating the innate immune response. The coated Ti implants were examined in a murine subcutaneous S. aureus infection model. Samples were placed along the infection site, following which the soft-tissue was sutured. The CaO2 nanoparticles embedded in the hydrogel coating with the IL-4 loaded TiO2 nanotubes were found to reduce neutrophil infiltration during the bacterial infection compared to non-coated Ti implants. Additionally, enhanced expression of CD206 positive cells (a M2 macrophage marker) and decrease in iNOS and TNF-α levels upon treatment with the dual-drug delivery system coated implant, demonstrated M2 macrophage polarization compared to treatment with non-coated Ti implants.
In another interleukin delivery approach, Lian et al. fabricated a three-layer coating on a Ti implant. Here, a layer of sodium alginate containing IL-12-loaded liposomes was sandwiched between layers of the mineral, vaterite. The vaterite layers were loaded with the antibiotic, vancomycin (Lian et al., 2022). In vitro, the functionalized Ti surfaces reduced bacterial growth by 82%–87% and 89%–93% for methicllin-sensitive S. aureus (MSSA) and MRSA, respectively. To examine the sustained release of IL-12 and the ability of the three-layer coating to rebuild the immune system, a rat tibia implant MRSA infection model was implemented. The coated Ti was implanted in a MRSA infected tibia channel. The sustained release of IL-12 from the liposomes regulated the innate and adaptive immune responses. Specifically, IL-12 stimulated interferon-gamma (IFN-γ), which promotes differentiation of T cells to pro-inflammatory T helper type 1 cells (Vignali and Kuchroo, 2012). Overall, there was an ∼99% bacterial clearance in the bone tissue treated with the three-layer coating implant compared to the infection-only with no implant control, the vaterite-only implant, and the vaterite with sodium-alginate implant.
Nanoparticles have been widely explored for a range of drug delivery applications and are of great interest in immunomodulation due to the plethora of formulation approaches and tunability of composition (Ben-Akiva et al., 2018; Yousefpour et al., 2023). Several polymeric nanoparticle formulations have been developed for use in bacterial infections that focus on reprogramming macrophages from an M2 phenotype to M1 via specific cytokine delivery (Yu et al., 2023) or inducing macrophage ROS production (Dai et al., 2022), among other approaches. We focus here on lipid-based nanoparticles, along with membrane wrapped polymeric nanoparticles, which are among the most commonly utilized immunomodulatory nanoparticles explored in the context of bacterial infection, along with discussing some of the inorganic nanoparticle approaches recently reported.
Several immunomodulatory lipid-based nanoparticles have been designed to either interact with and eliminate the effects of bacterial toxins or for gene therapy. Examples of the former include the development of high density lipoprotein-mimicking nanoparticles (Foit and Thaxton, 2016) and antimicrobial peptide, LL37, containing lipid nanoparticles (Garcia-Orue et al., 2016) that neutralize lipopolysaccharide (LPS) toxins upon exposure to Gram-negative bacteria.
The most extensive use of lipid nanoparticles targeting the immune response during bacterial infections has been for the delivery of small interfering RNA (siRNA). Kim et al. developed a liposome formulation that fuses with the macrophage cell membrane to deliver siRNA to the perinuclear region against the M1 macrophage interferon regulatory factor 5 (IRF5) gene (Figure 2:2A) (Kube et al., 2017; Kim et al., 2018). These fusogenic nanocarriers were found to effectively eliminate a murine lung infection established using a lethal dose of S. aureus (Figure 2:2B). Two possible mechanisms of action were hypothesized, one involving inhibition of pro-inflammatory cytokine release from M1 macrophages and one in which M2 macrophage polarization occurs leading to an anti-inflammatory, restorative response. The same nanoparticle platform was later applied as a broad-spectrum immunotherapy against both MRSA and Pseudomonas aeruginosa murine muscle and lung infection models, respectively. In a MRSA-induced abscess infection model, there was a large decrease in abscess size along with significant bacterial clearance upon intravenous injection of the targeted fusogenic nanoparticle compared to controls including treatment with a buffer, a non-fusogenic liposome, a fusogenic liposome with siRNA against luciferase, and vancomycin, a common antibiotic. In the P. aeruginosa lung infection model, similar reduction in bacterial infection and decrease in inflammation was observed upon intravenous injection of the treatment with fusogenic nanoparticles, compared to treatment with a buffer, a non-fusogenic liposome, a fusogenic liposome with siRNA against luciferase, and tobramycin, a common antibiotic used to treat P. aeruginosa infections. The fusogenic nanocarrier proved to be a highly versatile anti-inflammatory therapeutic (Kim et al., 2021).
Messenger RNA (mRNA) has also been encapsulated inside lipid-nanoparticles to promote the production of specific proteins of interest and enhance macrophage bactericidal activity (Hou et al., 2021). For example, mRNA that encodes for antimicrobial peptide, IB367, and lysosomal protein, cathepsin B (CatB), was encapsulated in vitamin C lipid nanoparticles. These nanoparticles were used to condition macrophages prior to adoptive transfer in immunosupressed mice for the treatment of multidrug-resistant bacteria-induced sepsis (Hou et al., 2020). The CatB served as a transporter for the IB367, into lysosomes that can then fuse with phagocytic macrophages and elicit bactericidal activity. Notably, Hou et al. demonstrated a significantly greater bacterial clearance and improved mouse survival rate for immunosupressed septic mice induced by multi-drug resistant S. aureus and Escherichia coli receiving the engineered macrophages compared with macrophages that were not treated with the mRNA nanoparticles.
Hybrid polymeric nanoparticles functionalized with cell membrane-derived coatings are being developed extensively for immunomodulation applications including treatment of bacterial infections due to their biomimetic properties. Polymeric nanoparticles have been wrapped with membranes derived from macrophages, neutrophils, natural killer cells, and red blood cells. For example, poly (lactic-co-glycolic acid) (PLGA) nanoparticle cores were wrapped with cell membranes derived from the J774 mouse macrophage cell line (Thamphiwatana et al., 2017). These macrophage-like nanoparticles were able to bind and neutralize LPS. Additionally, the membrane-wrapped nanoparticles sequestered pro-inflammatory cytokines, IL-6 and TNF-α, upon intraperitoneal injection in a murine uropathogenic E. coli sepsis model.
Membrane wrapped nanoparticles have also been used in bacterial infection vaccination strategies. Wang et al. demonstrated one of the earliest examples using red blood cell membranes to incorporate α-hemolysin (Hla), a major MRSA virulence factor. The toxin containing membrane was wrapped over a PLGA nanoparticle core (Wang et al., 2016). Subcutaneous injection of these nanoparticles in mice induced an anti-Hla antibody response, which led to protection of the mice upon introduction of a MRSA skin infection compared to those that received unloaded nanoparticles or a buffer control.
Recently, Li et al. developed a hyaluronic acid–polyethylenimine nanoparticle loaded with important microRNAs (miRNAs) identified as responsive for the immunoprotective effects of tumor-derived exosomes produced upon murine exposure to LPS (Li et al., 2022). These biomimetic nanoparticles enabled the therapeutic implementation of these miRNAs without necessitating the use of tumor-derived exosomes. The nanoparticles elicited sepsis protection and reduction in pro-inflammatory cytokine levels (IL-6, IL-8, and TNF-α) by ∼50% in a non-human primate LPS-induced sepsis model compared to the control group that had received a saline infusion.
Inorganic nanocarriers have also been implemented to enhance the innate immune response and combat bacterial infections. Gold nanocages in particular, have been explored due to their versatility, from surface functionalization to photothermal properties. Zhao et al. developed nano neuro-immune blockers (NNIBs) consisting of gold-silver nanocages coated with a glioma-derived cell-membrane. The cell membrane component contained virulence-factor inhibitors, developed to both reduce pain and enhance the innate immune response in necrotizing infections, while the nanocage itself served as a platform for photothermal therapy to regulate inflammation (Figure 2:3A) (Zhao et al., 2019). The nanocage formulation exhibits immunomodulatory properties by targeting calcitonin gene-related peptide (CGRP), which is associated with reduced immune cell recruitment. Subcutaneous injection of the NNIBs with NIR irradiation in a murine subcutaneous Streptococcus pyogenes infection model demonstrated CGRP inhibition and enhanced neutrophil recruitment by ∼80% compared to a saline control group (Figure 2:3B). In another study, enhanced bacterial recognition through TLRs was achieved by adding a macrophage coating on a gold nanocage (Wang et al., 2018). In a murine subcutaneous S. aureus infection model, the immune cell coating increased retention at the site of infection by ∼65% compared to a buffer control group due to the ability of the macrophage-nanoparticle coating to target and recognize PAMPs.
In addition to gold, other photoresponsive inorganic nanocarriers have been explored for immunodulation during bacterial infections. Zhang et al. developed a photocatalytic nanoparticle with an iron oxide (Fe3O4) core and TiO2 shell that was used to take advantage of the macrophage recruitment process which follows neutrophil apoptosis and release of inflammatory cytokines (Zhang et al., 2020). The photocatalytic activity of these nanoparticles was confirmed in vitro using near-ultraviolet (NUV) irradiation of the neutrophils loaded with these particles via phagocytosis. In this case, NUV irradiation led to ROS production, inducing neutrophil apoptosis. Macrophage migration in response to ROS production was also assessed in vitro using a transwell assay, showing RAW264.7 cells migration towards the photocatalytic nanoparticles following NUV irradiation. Further, nanoparticle accumulation at an infection site was investigated in a MRSA necrotizing fasciitis mouse model. The photocatalytic nanoparticles showed an 85% localized accumulation 24 h post-treatment (involving a 3 min NUV irradiation immediately after sample injection), thus preventing bacterial infection and potential dissemination into the blood.
Immunomodulatory hydrogels for use in bacterial infections have focused primarily on the treatment of bacterial wound infections. Hydrogels are highly hydrated biomaterials that can be designed to be biocompatible and exhibit a wide range of tunable properties, including the ability to respond to environmental stimuli (Correa et al., 2021; Alkekhia et al., 2022). Nucleic acid-loaded hydrogels that reprogram macrophages to an M2 phenotype have been developed (Gribova et al., 2022), along with hydrogels that target IL-1 cytokines to regulate the inflammatory response in diabetic wound biofilm infections (Zhou et al. (2023)). Here, we briefly expand on peptide functionalized immunomodulatory hydrogels.
In one design, a hydroxyethyl cellulose hydrogel was functionalized with thrombin-derived C-terminal peptide, TCP-25, to treat P. aeruginosa and S. aureus wound infections (Puthia et al., 2020). TCP-25 exhibits both antimicrobial and anti-inflammatory properties via association with PAMPs, leading to a reduction in pro-inflammatory processes. In a porcine S. aureus partial thickness wound infection model, the TCP-25 hydrogel treatment was applied daily as a dressing for 4 days. Pigs treated with this hydrogel were found to exhibit a significant decrease in bacterial load and reduction in the secretion of IL-6 and TNF-α after 48 h compared to treatment with a non-TCP-25 functionalized hydrogel. In another study, Liu et al. synthesized extracellular matrix mimetic hydrogels that promote M2 macrophage polarization for the treatment of MRSA chronic wound infections. The hybrid hydrogel consisted of an antibacterial peptide conjugated to glucomannan (GM-P) using an acid-labile linkage to control local inflammation mediated by GM interaction with mannose receptors on macrophages (Liu et al., 2022) (Figure 2:4A). Additionally, to support tissue regeneration, the hydrogel incorporated a collagen tripeptide conjugated to hyaluronic acid via a matrix-metalloproteinase sensitive linkage (HA-P). This hydrogel exhibited ∼98% and ∼97% bacterial killing in a bacterial biofilm in vitro assay with MRSA and E. coli, respectively (Figure 2:4B). Further, the immunomodulatory hydrogel led to macrophage M2 polarization, with an increase in IL-4 production, helping to clear the infection in an in vivo MRSA-infected chronic wound healing model (Figure 2:4C).
Engineering immunomodulatory biomaterials is a promising strategy for the treatment of bacterial infections. It is now widely recognized that we have entered a post-antibiotic era (Kwon and Powderly (2021)). Alternative strategies to antibiotic treatment are critical for effective treatment of bacterial infections and to prevent further emergence of multi-drug resistant bacteria or “superbugs”. Development of immunomodulatory biomaterials that target the host-immune response are the likely solution to this global healthcare crisis. Here, we discussed biomaterials-mediated immunomodulation approaches from surface functionalization to nanoparticle therapies and hydrogels. While the majority of biomaterials explored thus far for treatment of bacterial infections have focused on macrophage polarization to control inflammation, it is important to note that additional myeloid and lymphoid cells are also promising immune response targets. Further research in bacterial immune evasion and the host-pathogen response is imperative for the development of successful host-directed therapies to modulate the immune system during bacterial infections.
CGC: Conceptualization, Investigation, Writing–original draft, Writing–review and editing. AS: Funding acquisition, Resources, Writing–original draft, Writing–review and editing.
The author(s) declare financial support was received for the research, authorship, and/or publication of this article. AS and CGC acknowledge support from Brown University and the Office of Naval Research (award N00014-22-1-2336).
AS and CGC thank Brown University Ph.D. student, Veronica LaMastro for useful discussions related to article content. Figure 1 was created with BioRender.com.
The authors declare that the research was conducted in the absence of any commercial or financial relationships that could be construed as a potential conflict of interest.
All claims expressed in this article are solely those of the authors and do not necessarily represent those of their affiliated organizations, or those of the publisher, the editors and the reviewers. Any product that may be evaluated in this article, or claim that may be made by its manufacturer, is not guaranteed or endorsed by the publisher.
Alkekhia, D., Larose, C., and Shukla, A. (2022). β-Lactamase-Responsive hydrogel drug delivery platform for bacteria-triggered cargo release. ACS Appl. Mater. Interfaces 14, 27538–27550. doi:10.1021/ACSAMI.2C02614
Backlund, C., Jalili-Firoozinezhad, S., Kim, B., and Irvine, D. J. (2023). Biomaterials-mediated engineering of the immune system. Annu. Rev. Immunol. 41, 153–179. doi:10.1146/ANNUREV-IMMUNOL-101721-040259
Ben-Akiva, E., Est Witte, S., Meyer, R. A., Rhodes, K. R., and Green, J. J. (2018). Polymeric micro- and nanoparticles for immune modulation. Biomaterials Sci. 7, 14–30. doi:10.1039/C8BM01285G
Chiang, C. Y., Uzoma, I., Moore, R. T., Gilbert, M., Duplantier, A. J., and Panchal, R. G. (2018). Mitigating the impact of antibacterial drug resistance through host-directed therapies: current progress, outlook, and challenges. mBio 9, e01932-17. doi:10.1128/MBIO.01932-17
Cho, J. Y. (2022). Recent advancements in microbial diversity: macrophages and their role in inflammation. J. Clin. Exp. Hepatol. 1–461. doi:10.1016/C2019-0-04189-1
Correa, S., Grosskopf, A. K., Lopez Hernandez, H., Chan, D., Yu, A. C., Stapleton, L. M., et al. (2021). Translational applications of hydrogels. Chem. Rev. 121, 11385–11457. doi:10.1021/ACS.CHEMREV.0C01177
Dai, X., Liu, X., Yang, L., Yuan, S., Xu, Q., Li, Y., et al. (2022). pH-Responsive non-antibiotic polymer prodrugs eradicate intracellular infection by killing bacteria and regulating immune response. Colloids surfaces. B, Biointerfaces 220, 112889. doi:10.1016/J.COLSURFB.2022.112889
Deusenbery, C., Gomez Casas, C., and Shukla, A. (2023). pH-responsive swelling micelles for the treatment of methicillin-resistant Staphylococcus aureus biofilms. ACS Appl. Polym. Mater. 5, 7400–7410. doi:10.1021/ACSAPM.3C01307
Diacovich, L., and Gorvel, J. P. (2010). Bacterial manipulation of innate immunity to promote infection. Nat. Rev. Microbiol. 8, 117–128. doi:10.1038/nrmicro2295
Ding, Y., Liu, G., Liu, S., Li, X., Xu, K., Liu, P., et al. (2023). A multifunction hydrogel-coating engineered implant for rescuing biofilm infection and boosting osseointegration by macrophage-related immunomodulation. Adv. Healthc. Mater. 12, 2300722. doi:10.1002/ADHM.202300722
Ding, Y., Ma, R., Liu, G., Li, X., Xu, K., Liu, P., et al. (2022). Fabrication of a new hyaluronic acid/gelatin nanocomposite hydrogel coating on titanium-based implants for treating biofilm infection and excessive inflammatory response. ACS Appl. Mater. Interfaces 15, 13783–13801. doi:10.1021/ACSAMI.2C23320
Finlay, B. B., and McFadden, G. (2006). Anti-Immunology: evasion of the host immune system by bacterial and viral pathogens. Cell 124, 767–782. doi:10.1016/J.CELL.2006.01.034
Foit, L., and Thaxton, C. S. (2016). Synthetic high-density lipoprotein-like nanoparticles potently inhibit cell signaling and production of inflammatory mediators induced by lipopolysaccharide binding Toll-like receptor 4. Biomaterials 100, 67–75. doi:10.1016/J.BIOMATERIALS.2016.05.021
Garcia-Orue, I., Gainza, G., Girbau, C., Alonso, R., Aguirre, J. J., Pedraz, J. L., et al. (2016). LL37 loaded nanostructured lipid carriers (NLC): a new strategy for the topical treatment of chronic wounds. Eur. J. Pharm. Biopharm. 108, 310–316. doi:10.1016/J.EJPB.2016.04.006
González, J. F., Hahn, M. M., and Gunn, J. S. (2018). Chronic biofilm-based infections: skewing of the immune response. Pathogens Dis. 76, 23. doi:10.1093/FEMSPD/FTY023
Gribova, V., Petit, L., Kocgozlu, L., Seguin, C., Fournel, S., Kichler, A., et al. (2022). Polyarginine as a simultaneous antimicrobial, immunomodulatory, and miRNA delivery agent within polyanionic hydrogel. Macromol. Biosci. 22, 2200043. doi:10.1002/MABI.202200043
Guzmán-Soto, I., McTiernan, C., Gonzalez-Gomez, M., Ross, A., Gupta, K., Suuronen, E. J., et al. (2021). Mimicking biofilm formation and development: recent progress in in vitro and in vivo biofilm models. iScience 24, 102443. doi:10.1016/J.ISCI.2021.102443
He, Y., Li, K., Yang, X., Leng, J., Xu, K., Yuan, Z., et al. (2021). Calcium peroxide nanoparticles-embedded coatings on anti-inflammatory TiO2 nanotubes for bacteria elimination and inflammatory environment amelioration. Small 17, 2102907. doi:10.1002/SMLL.202102907
Hou, X., Zaks, T., Langer, R., and Dong, Y. (2021). Lipid nanoparticles for mRNA delivery. Nat. Rev. Mater. 6, 1078–1094. doi:10.1038/s41578-021-00358-0
Hou, X., Zhang, X., Zhao, W., Zeng, C., Deng, B., McComb, D. W., et al. (2020). Vitamin lipid nanoparticles enable adoptive macrophage transfer for the treatment of multidrug-resistant bacterial sepsis. Nat. Nanotechnol. 15, 41–46. doi:10.1038/s41565-019-0600-1
Huang, Q., Li, X., Elkhooly, T. A., Liu, X., Zhang, R., Wu, H., et al. (2018). The Cu-containing TiO2 coatings with modulatory effects on macrophage polarization and bactericidal capacity prepared by micro-arc oxidation on titanium substrates. Colloids Surfaces B Biointerfaces 170, 242–250. doi:10.1016/J.COLSURFB.2018.06.020
Huang, Q., Ouyang, Z., Tan, Y., Wu, H., and Liu, Y. (2019). Activating macrophages for enhanced osteogenic and bactericidal performance by Cu ion release from micro/nano-topographical coating on a titanium substrate. Acta Biomater. 100, 415–426. doi:10.1016/J.ACTBIO.2019.09.030
Huo, S., Lyu, Z., Su, X., Wang, F., Liu, J., Liu, S., et al. (2023). Formation of a novel Cu-containing bioactive glass nano-topography coating with strong bactericidal capability and bone regeneration. Compos. Part B Eng. 253, 110521. doi:10.1016/J.COMPOSITESB.2023.110521
Ikuta, K. S., Swetschinski, L. R., Robles Aguilar, G., Sharara, F., Mestrovic, T., Gray, A. P., et al. (2022). Global mortality associated with 33 bacterial pathogens in 2019: a systematic analysis for the Global Burden of Disease Study 2019. Lancet 400, 2221–2248. doi:10.1016/S0140-6736(22)02185-7
Kalelkar, P. P., Riddick, M., and García, A. J. (2021). Biomaterial-based antimicrobial therapies for the treatment of bacterial infections. Nat. Rev. Mater. 7, 39–54. doi:10.1038/s41578-021-00362-4
Kany, S., Vollrath, J. T., and Relja, B. (2019). Cytokines in inflammatory disease. Int. J. Mol. Sci. 20, 6008. doi:10.3390/IJMS20236008
Kaya, E., Grassi, L., Benedetti, A., Maisetta, G., Pileggi, C., Di Luca, M., et al. (2020). In vitro interaction of Pseudomonas aeruginosa biofilms with human peripheral blood mononuclear cells. Front. Cell. Infect. Microbiol. 10, 187. doi:10.3389/fcimb.2020.00187
Kim, B., Pang, H. B., Kang, J., Park, J. H., Ruoslahti, E., and Sailor, M. J. (2018). Immunogene therapy with fusogenic nanoparticles modulates macrophage response to Staphylococcus aureus. Nat. Commun. 9, 1969–2013. doi:10.1038/s41467-018-04390-7
Kim, B., Yang, Q., Chan, L. W., Bhatia, S. N., Ruoslahti, E., and Sailor, M. J. (2021). Fusogenic porous silicon nanoparticles as a broad-spectrum immunotherapy against bacterial infections. Nanoscale Horizons 6, 330–340. doi:10.1039/D0NH00624F
Kube, S., Hersch, N., Naumovska, E., Gensch, T., Hendriks, J., Franzen, A., et al. (2017). Fusogenic liposomes as nanocarriers for the delivery of intracellular proteins. Langmuir 33, 1051–1059. doi:10.1021/acs.langmuir.6b04304
Kwon, J. H., and Powderly, W. G. (2021). The post-antibiotic era is here. Science 373, 471. doi:10.1126/SCIENCE.ABL5997
Le, K. Y., Park, M. D., and Otto, M. (2018). Immune evasion mechanisms of Staphylococcus epidermidis biofilm infection. Front. Microbiol. 9, 359. doi:10.3389/fmicb.2018.00359
Li, J., Liu, W., Kilian, D., Zhang, X., Gelinsky, M., and Chu, P. K. (2019). Bioinspired interface design modulates pathogen and immunocyte responses in biomaterial-centered infection combination therapy. Mater. Horizons 6, 1271–1282. doi:10.1039/C8MH01606B
Li, M., Ma, Z., Zhu, Y., Xia, H., Yao, M., Chu, X., et al. (2016). Toward a molecular understanding of the antibacterial mechanism of copper-bearing titanium alloys against Staphylococcus aureus. Adv. Healthc. Mater. 5, 557–566. doi:10.1002/ADHM.201500712
Li, Y., Liu, X., Li, B., Zheng, Y., Han, Y., Chen, D. F., et al. (2020). Near-infrared light triggered phototherapy and immunotherapy for elimination of methicillin-resistant Staphylococcus aureus biofilm infection on bone implant. ACS Nano 14, 8157–8170. doi:10.1021/ACSNANO.0C01486
Li, Y., Zhang, H., Chen, C., Qiao, K., Li, Z., Han, J., et al. (2022). Biomimetic immunosuppressive exosomes that inhibit cytokine storms contribute to the alleviation of sepsis. Adv. Mater. 34, 2108476. doi:10.1002/ADMA.202108476
Lian, Q., Zheng, S., Shi, Z., Li, K., Chen, R., Wang, P., et al. (2022). Using a degradable three-layer sandwich-type coating to prevent titanium implant infection with the combined efficient bactericidal ability and fast immune remodeling property. Acta Biomater. 154, 650–666. doi:10.1016/J.ACTBIO.2022.10.033
Liu, W., Gao, R., Yang, C., Feng, Z., Ou-Yang, W., Pan, X., et al. (2022). ECM-mimetic immunomodulatory hydrogel for methicillin-resistant Staphylococcus aureus-infected chronic skin wound healing. Sci. Adv. 8, 7006. doi:10.1126/SCIADV.ABN7006
Liu, W., Li, J., Cheng, M., Wang, Q., Qian, Y., Yeung, K. W., et al. (2019). A surface-engineered polyetheretherketone biomaterial implant with direct and immunoregulatory antibacterial activity against methicillin-resistant Staphylococcus aureus. Biomaterials 208, 8–20. doi:10.1016/J.BIOMATERIALS.2019.04.008
Lyu, Z., Zhao, Y., Huo, S., Wang, F., Meng, X., Yuan, Z., et al. (2022). Mussel-inspired dopamine-CuII coated polyetheretherketone surface with direct and immunomodulatory effect to facilitate osteogenesis, angiogenesis, and antibacterial ability. Mater. Des. 222, 111069. doi:10.1016/J.MATDES.2022.111069
Marshall, J. S., Warrington, R., Watson, W., and Kim, H. L. (2018). An introduction to immunology and immunopathology. Allergy, Asthma Clin. Immunol. 14, 49–10. doi:10.1186/s13223-018-0278-1
Murray, C. J., Ikuta, K. S., Sharara, F., Swetschinski, L., Robles Aguilar, G., Gray, A., et al. (2022). Global burden of bacterial antimicrobial resistance in 2019: a systematic analysis. Lancet 399, 629–655. doi:10.1016/S0140-6736(21)02724-0
Paludan, S. R., Pradeu, T., Masters, S. L., and Mogensen, T. H. (2020). Constitutive immune mechanisms: mediators of host defence and immune regulation. Nat. Rev. Immunol. 21, 137–150. doi:10.1038/s41577-020-0391-5
Pant, J., Goudie, M. J., Chaji, S. M., Johnson, B. W., and Handa, H. (2018). Nitric oxide releasing vascular catheters for eradicating bacterial infection. J. Biomed. Mater. Res. Part B Appl. Biomaterials 106, 2849–2857. doi:10.1002/JBM.B.34065
Pizarek, J. A., Fischer, N. G., and Aparicio, C. (2023). Immunomodulatory IL-23 receptor antagonist peptide nanocoatings for implant soft tissue healing. Dent. Mater. 39, 204–216. doi:10.1016/J.DENTAL.2023.01.001
Puthia, M., Butrym, M., Petrlova, J., Strömdahl, A. C., Andersson, M., Kjellström, S., et al. (2020). A dual-action peptide-containing hydrogel targets wound infection and inflammation. Sci. Transl. Med. 12, 6601. doi:10.1126/SCITRANSLMED.AAX6601
Sapkota, A., Mondal, A., Chug, M. K., and Brisbois, E. J. (2023). Biomimetic catheter surface with dual action NO-releasing and generating properties for enhanced antimicrobial efficacy. J. Biomed. Mater. Res. Part A 111, 1627–1641. doi:10.1002/JBM.A.37560
Super, M., Doherty, E. J., Cartwright, M. J., Seiler, B. T., Langellotto, F., Dimitrakakis, N., et al. (2021). Biomaterial vaccines capturing pathogen-associated molecular patterns protect against bacterial infections and septic shock. Nat. Biomed. Eng. 6, 8–18. doi:10.1038/s41551-021-00756-3
Thamphiwatana, S., Angsantikul, P., Escajadillo, T., Zhang, Q., Olson, J., Luk, B. T., et al. (2017). Macrophage-like nanoparticles concurrently absorbing endotoxins and proinflammatory cytokines for sepsis management. Proc. Natl. Acad. Sci. U. S. A. 114, 11488–11493. doi:10.1073/PNAS.1714267114
Vignali, D. A., and Kuchroo, V. K. (2012). IL-12 family cytokines: immunological playmakers. Nat. Immunol. 13, 722–728. doi:10.1038/ni.2366
Wang, C., Wang, Y., Zhang, L., Miron, R. J., Liang, J., Shi, M., et al. (2018). Pretreated macrophage-membrane-coated gold nanocages for precise drug delivery for treatment of bacterial infections. Adv. Mater. 30, 1804023. doi:10.1002/ADMA.201804023
Wang, F., Fang, R. H., Luk, B. T., Hu, C.-M. J., Thamphiwatana, S., Dehaini, D., et al. (2016). Nanoparticle-based antivirulence vaccine for the management of methicillin-resistant Staphylococcus aureus skin infection. Adv. Funct. Mater. 26, 1628–1635. doi:10.1002/ADFM.201505231
Wang, P., Yuan, Y., Xu, K., Zhong, H., Yang, Y., Jin, S., et al. (2021). Biological applications of copper-containing materials. Bioact. Mater. 6, 916–927. doi:10.1016/J.BIOACTMAT.2020.09.017
Wang, Y., and Shukla, A. (2022). Bacteria-responsive biopolymer-coated nanoparticles for biofilm penetration and eradication. Biomaterials Sci. 10, 2831–2843. doi:10.1039/D2BM00361A
Yao, Y., Xu, X. H., and Jin, L. (2019). Macrophage polarization in physiological and pathological pregnancy. Front. Immunol. 10, 792. doi:10.3389/FIMMU.2019.00792
Yousefpour, P., Ni, K., and Irvine, D. J. (2023). Targeted modulation of immune cells and tissues using engineered biomaterials. Nat. Rev. Bioeng. 1, 107–124. doi:10.1038/s44222-022-00016-2
Yu, Y. J., Yan, J. H., Chen, Q. W., Qiao, J. Y., Peng, S. Y., Cheng, H., et al. (2023). Polymeric nano-system for macrophage reprogramming and intracellular MRSA eradication. J. Control. Release 353, 591–610. doi:10.1016/J.JCONREL.2022.12.014
Zhang, P., Zhao, Q., Shi, M., Yin, C., Zhao, Z., Shen, K., et al. (2020). Fe3O4@TiO2-Laden neutrophils activate innate immunity via photosensitive reactive oxygen species release. Nano Lett. 20, 261–271. doi:10.1021/ACS.NANOLETT.9B03777
Zhao, Q., Wang, J., Yin, C., Zhang, P., Zhang, J., Shi, M., et al. (2019). Near-infrared light-sensitive nano neuro-immune blocker capsule relieves pain and enhances the innate immune response for necrotizing infection. Nano Lett. 19, 5904–5914. doi:10.1021/ACS.NANOLETT.9B01459
Keywords: biomaterials, immunotherapy, immunoengineering, nanoparticles, functionalized surfaces, hydrogels, bacteria, infection
Citation: Gomez Casas C and Shukla A (2024) Engineering immunomodulatory biomaterials to combat bacterial infections. Front. Front. Biomater. Sci. 2:1336842. doi: 10.3389/fbiom.2023.1336842
Received: 11 November 2023; Accepted: 13 December 2023;
Published: 10 January 2024.
Edited by:
Jenny Robinson, University of Washington, United StatesReviewed by:
Rupak Madu Rajachar, Marine Ecology and Telemetry Research (MarEcoTel), United StatesCopyright © 2024 Gomez Casas and Shukla. This is an open-access article distributed under the terms of the Creative Commons Attribution License (CC BY). The use, distribution or reproduction in other forums is permitted, provided the original author(s) and the copyright owner(s) are credited and that the original publication in this journal is cited, in accordance with accepted academic practice. No use, distribution or reproduction is permitted which does not comply with these terms.
*Correspondence: Anita Shukla, YW5pdGFfc2h1a2xhQGJyb3duLmVkdQ==
Disclaimer: All claims expressed in this article are solely those of the authors and do not necessarily represent those of their affiliated organizations, or those of the publisher, the editors and the reviewers. Any product that may be evaluated in this article or claim that may be made by its manufacturer is not guaranteed or endorsed by the publisher.
Research integrity at Frontiers
Learn more about the work of our research integrity team to safeguard the quality of each article we publish.