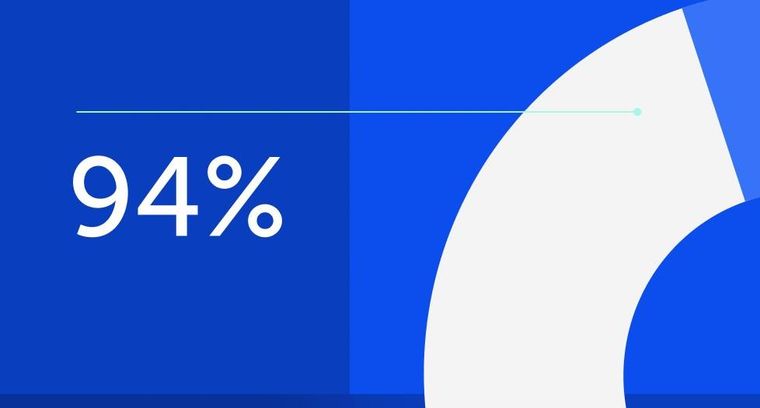
94% of researchers rate our articles as excellent or good
Learn more about the work of our research integrity team to safeguard the quality of each article we publish.
Find out more
ORIGINAL RESEARCH article
Front. Bioinform., 09 August 2024
Sec. Drug Discovery in Bioinformatics
Volume 4 - 2024 | https://doi.org/10.3389/fbinf.2024.1428539
This article is part of the Research TopicWomen in BioinformaticsView all 6 articles
Introduction: In malaria management, insecticides play a crucial role in targeting disease vectors. Benzothiazole derivatives have also been reported to possess insecticidal properties, among several other properties they exhibit. The female Anopheles mosquito is responsible for transmitting the malaria parasite when infected. Anopheles gambiae (Ag) and Anopheles funestus (Af) are two of the most notable Anopheles species known to spread malaria in Nigeria. Trehalase is an enzyme that breaks down trehalose. Recent research has proposed it as a viable target for inhibition since it aids in flight and stress adaptation.
Methods: This study aimed to investigate benzothiazole derivatives as potential inhibitors of trehalase of Anopheles funestus (AfTre) and Anopheles gambiae (AgTre) using toxicity profiling, molecular docking, and dynamic simulation for future insecticidal intervention. A total of 4,214 benzothiazole-based compounds were obtained from the PubChem database and subjected to screening against the 3D modelled structure of AfTre and AgTre. Compounds with some toxicity levels were optimised, and the obtained lead compounds were further investigated through molecular docking studies. Furthermore, the best hit was subjected to parameters such as RMSD, RMSF, SASA, Rg, and hydrogen bond to confirm its stability when in a complex with AfTre, and these parameters were compared to that of validamycin A (control ligand).
Results and discussion: The post-screening analysis showed binding affinities of −8.7 and −8.2 kcal/mol (compound 1), −8.2 and −7.4 kcal/mol (compound 2), compared to −6.3 and −5.1 kcal/mol (Validamycin A, a known inhibitor) against AfTre and AgTre, respectively. The molecular dynamics simulation showed that compound 1 (the best hit) had good stability when in complex with AfTre. These findings suggest that these best hits can serve as potential inhibitors for the development of novel insecticides in the control of malaria vectors.
Malaria eradication efforts have involved various strategies including malaria prophylaxis, mosquito nets, vaccines, and insecticides (Lees et al., 2019). Recently, Ghana, Malawi, and Kenya conducted malaria vaccine trials in children, showcasing scientific initiatives to combat the disease (WHO, 2022). Malaria transmission is primarily facilitated by infected female Anopheles mosquitoes (Olayinka, 2019). Among the well-known Anopheles species, the An. gambiae and An. funestus are major carriers of malaria-causing Plasmodium falciparum in humans (Okorie et al., 2011; Nolden et al., 2023). These species are commonly found in tropical sub-Saharan regions, such as Ethiopia, Ghana, Tanzania, Nigeria, Kenya, and South Africa. An. funestus exhibits endophilic behaviour, preferring to bite and rest indoors, and is anthropophilic, showing a significant preference for human blood over other vertebrates (Ghurye et al., 2019; Nambunga et al., 2020). An. funestus reaches its highest population during the dry season when An. gambiae is less prevalent; thus extending the period of malaria transmission (Serazin et al., 2009). Similarly, An. gambiae, a common and efficient vector, is found in large numbers with the ability to survive for extended periods, and a tendency to bite humans and transmit diseases (Akpan et al., 2018). An. gambiae normally reproduce in tiny transient pools and puddles created by rain, whereas An. funestus takes advantage of larger, permanent, or semi-permanent bodies of water that have emergent plants (Serazin et al., 2009). With better daily survival rates than other Anopheles species and resistance to common pyrethroid pesticides, An. funestus poses challenges for effective malaria control (Ghurye et al., 2019). As resistance to existing treatments has increased, the need for novel compounds with unique modes of action against mosquito vectors has become evident.
Several target locations, including the γ-aminobutyric acid (GABA) receptor, acetylcholinesterase (AChE), and mitochondrial electron transport, have been studied to control mosquito vectors (Adedeji et al., 2020). Trehalase, an enzyme involved in the conversion of trehalose to glucose, has been identified as a potential target for inhibition that limits the hydrolysis of trehalose into glucose (Shukla et al., 2015). Trehalose, a sugar molecule consisting of two glucose units linked together, plays a crucial role in the survival strategies of many organisms, including mosquitoes. Trehalose acts as a main energy reserve in mosquitoes, providing a stable form of energy that can swiftly be utilized upon demand (Marten et al., 2020; Zhong et al., 2023). It also aids in preventing dehydration by reinforcing cell structures and membranes, and it safeguards against freezing damage by stopping ice crystals from forming inside cells, enabling mosquitoes to endure colder climates. Inhibiting the enzyme that breaks down trehalose would disrupt its functions in metabolism, protection against environmental stresses, and energy conservation (Chen et al., 2010; Liu et al., 2013; Shukla et al., 2015). Trehalose is expressed in the fat body of An. gambiae female adults. Its production is triggered by environmental factors such as high temperature or low humidity. AgTreT1 can be a target for suppression and is necessary for mosquito vector development of the malaria pathogen and stress adaptability (Liu et al., 2013). Targeting trehalase instead of specific genes or pathways offers a broader, more sustainable approach to controlling mosquito populations because it targets a fundamental biological process common among many organisms, reducing the likelihood of rapid evolutionary resistance compared to targeting rapidly evolving genes (Shukla et al., 2015; Adedeji et al., 2020; Marten et al., 2020; Neyman et al., 2024).
Organic compounds have emerged as promising candidates for the development of sustainable treatments for various health problems. Thiazoles, a class of organic compounds, have several applications in the pharmaceutical industry, particularly as pesticides (Maienfisch and Edmunds, 2017; Singh et al., 2022). Examples of commercially available pesticides from thiazole templates include clothianidin, thiamethoxam, ethaboxam, thiabendazole, diclomezotiaz, oxathiapiprolin, fluensulfone, and thifluzamide (Figure 1). Benzothiazole, a benzo-fused thiazole, and its functionalised derivatives have been reported to exhibit antimicrobial (Lafzi et al., 2021), antiviral (Kumar and Dubey, 2022), anticancer (Abd El-Meguid et al., 2022), fungicidal, and herbicidal properties (Maienfisch and Edmunds, 2017). Designing effective therapeutic candidates can be time-consuming and expensive in the pharmaceutical industry (Jampilek, 2019). However, computational methods, such as Computer-Aided Drug Discovery (CADD), have significantly simplified the process by assessing the potential pharmacological actions of chemical compounds before manufacturing (Saravanan et al., 2018; Oduselu et al., 2023). Hence, this study aimed to investigate benzothiazole derivatives as potential inhibitors of AfTre and AgTre, using in silico ADMET properties, molecular docking and molecular dynamic simulation for future insecticidal interventions against malaria-transmitting mosquitoes.
Ab initio modelling was conducted to generate the 3D structure of the protein due to the unavailability of the experimentally determined crystallised structure on the Protein Data Bank (PDB). The protein sequences of the target Trehalase from two different mosquito strains, An. funestus and An. gambiae, were obtained from the UniProt Knowledgebase (UniProtKB), with corresponding accession numbers A0A182RST3 and Q7PZS4. Three different Ab initio web servers, Robetta (RoseTTAFold), Contact-guided Iterative Threading ASSEmbly Refinement (C-I TASSER), and I-TASSER web servers, were used to conduct a blast using these sequences. The predicted 3D models generated were assessed using the SWISS-MODEL structure assessment tool, which included parameters such as Qualitative Model Energy Analysis (QMEAN), Ramachandran plot, outliers, and the number of C-beta deviations. The SAVES web server was used to evaluate the model’s accuracy through ERRAT and VERIFY3D analyses. Additionally, active site prediction was carried out using PrankWeb and CASTp (Computed Atlas of Surface Topography of proteins) web servers (Adedeji et al., 2022). Structure alignment was carried out using Chimera software 1.14. Sequence alignment was performed on BLASTp (Basic Local Alignment Search Tool for Amino Acid Sequences) web server (Altschul et al., 1997), and Chimera software 1.14 using the Needleman-Wunsch algorithm to align the best model from RoseTTAFold of the two proteins. After the assessment, the best model was selected and prepared for further analysis.
The benzothiazole template was utilised for the compounds search on the PubChem database for ligand design (Kim et al., 2023) which is a comprehensive compound repository. The search was conducted using a similar structure option and the compounds were filtered using Lipinski’s rule of five (LO5). Furthermore, the Tanimoto threshold was set and a total of about 4,214 compounds were downloaded in Structure-Data Files (SDF) format. These compounds were then converted to Autodock formats (PDBQT) using the Open Babel in PyRx.
Here all the 4,214 compounds were screened against via AutoDock vina. The grid box for the virtual screening was established using the predicted binding pockets of AfTre to adjust the XYZ dimensions of 29.1004, 19.2672, 20.9740, and XYZ centre of 25.8515, 27.9404, and 28.8368. An exhaustiveness value of 8 was used. Additionally, virtual screening was performed against the AgTre using top hits, and optimised compounds (obtained from AfTre investigations). AgTre’s binding pocket had xyz coordinates of 28.6748, 29.3685, and 26.8692, with an xyz center of 0.4168, 7.6228, and 20.4605. The binding energies for the top 9 hits and the control ligand were obtained after the virtual screening. The interaction between An. funestus trehalase and the ligand was visualised using Discovery Studio.
The quantitative estimate of insecticide-likeness (QEI) was computed using QEPest.jar software designed by Avram et al. (2014). The Lipinski’s RO5 was used as a selection criterion. In silico ADMET (Absorption, Distribution, Metabolism, Excretion and Toxicity) properties of the active molecules were assessed using the SWISSADME and ADMETlab web server (Xiong et al., 2021; Azzam, 2023), Bee-Tox database (Crisan et al., 2022).
The scaffolds of a few top hits were improved upon using ADMETopt web server (Yang et al., 2018), to obtain new compounds with improved binding affinity. The optimisation search was performed by choosing non-toxic parameters on the server while the smiles of resulting compounds were downloaded and converted to PDB format. Further docking studies were conducted using the new compounds against AfTre and AgTre.
Molecular Dynamic simulation studies were conducted on the best poses of compound 1 and the control ligand following docking studies. The aim was to assess ligand binding stability on the AfTre protein (Gupta et al., 2020; Kushwaha et al., 2021). The molecular dynamic simulation run was performed at 100 ns using GROMACS (GROningen MAchine for Chemical Simulations) Version 2021.4 (Abraham et al., 2015). Ligand topologies and parameter files were generated using the SwissParam server (Zoete et al., 2011). The complexes were simulated using Charmm27 force field and TIP3P model inside a triclinic box with a 1 Å buffer distance. Energy minimisation was utilised to fix the backbone atoms in the protein. There were two equilibration steps involved: 100 ps NVT followed by 100 ps NPT (Kushwaha et al., 2021). The temperature of the system was raised to 300 K, while the coordinates for each system were stored every 10 ps (Parrinello and Rahman, 1981). Root Mean Square Fluctuation (RMSF), Root Mean Square Deviation (RMSD), hydrogen bonds, Solvent-Accessible Surface Areas (SASA), and Radius of Gyration (Rg) of the complexes were computed using gmx tools (Kushwaha et al., 2021). Principal Component Analysis (PCA) was performed on the European Galaxy server (Bray et al., 2020). Finally, the charts were made using the Qtgrace software (Gupta et al., 2020).
Among the various predicted 3D structures of AfTre and AgTre, the RoseTTAFold model had a confidence score (0.85 and 0.84) which is closer to 1, and a QMEAN score (0.25 and 0.18), which is closer to 0, indicating a high-quality structure. Similarly, the predicted structures of AfTre and AgTre from RoseTTAFold were found to have 0.17% and 0% of its residue in Ramachandran outliers, 97.36%, and 98.77% Ramachandran favoured with 0% for both Rotamer outliers which are better when compared to other models (Table 1). Based on these results, the predicted 3D structure of AfTre and AgTre from RoseTTAFold was used in further studies (Adedeji et al., 2022). Structural and sequence alignment was conducted using the predicted 3D structures of AfTre and AgTre from RoseTTAFold (Figure 2). The RMSD of 0.622 Å indicates that the backbone C-alpha atoms of the two structures were more aligned considering 607/570 residues. The sequence alignment conducted on AfTre and AgTre showed they had a percentage identity (%I) of 92.06% and a query coverage of 99%. A study by Crawford et al. (2010) further spotlighted the probable similarity between the amino acid residues of both species even though they exhibit several ecological differences (Crawford et al., 2010). The active site residues of trehalase enzymes play a critical role in substrate binding and catalysis. The active site prediction using CASTp indicated potential binding pockets for both AfTre and AgTre, with 101 and 102 pockets, respectively. The best pocket for AfTre had an area (SA) of 387.80 Å2 and a volume (SA) of 611.25 Å3, whereas, for AgTre, the best pocket had an area of 313.76 Å2 and volume of 359.47 Å3. Additionally, PrankWeb predicted 11 pockets for both AfTre and AgTre, with the highest-ranked pockets having probabilities of 0.984 and 0.983, respectively. The PrankWeb had more amino acid residues than that of CASTp for AfTre and AgTre. A total of 23 proposed active site residues of trehalase were conserved in both AfTre and AgTre, indicating a tendency for compounds to interact similarly with both organisms (Table 2). The similarity in the conserved amino acid residue of the active site further uncovers the predictions from the sequence alignment of both proteins. For the docking studies, the best predicted binding pockets from PrankWeb were used to set the grid box for the docking studies of AfTre and AgTre as shown in Table 2.
Table 1. Structural assessment of predicted 3D structures of Trehalase of An. funestus (AfTre) and An. gambiae (AgTre).
Figure 2. (A) Structural alignment of the predicted 3D structure of AfTre (Red) and AgTre (Purple) from RoseTTAFold. RMSD of 0.622 Å considering 607/570 residues; (B) Sequence alignment of the predicted 3D structure of AfTre and AgTre from RoseTTAFold gave % Identity of 92.06%. AfTre: trehalase of An. funestus and AgTre: trehalase of An. gambiae.
The virtual screening involved the evaluation of 4,216 compounds, along with the control ligand Validamycin A (443,629), against AfTre. Among the compounds screened, the top nine hits exhibited binding affinities ranging from −7.9 to −7.7 kcal/mol (Table 3). Notably, all the compounds displayed higher binding affinities than the reference compound for AfTre. The most favourable binding affinity −7.9 kcal/mol, was observed for the molecule with PubChem ID: 135570533. Post-docking investigations between the compound and the AfTre amino acid residue revealed typical hydrogen bonds, unfavourable donor-donor, pi-anion, pi-sulfur, and pi-pi T-shaped interactions. Typical hydrogen bonds with Glu160, Tyr207, and Asp307 were observed, reinforcing the connection formed between the compound and the protein’s active site. Conversely, unfavourable donor-donor interactions may indicate some level of instability of the compound within the active site. Additionally, pi-cation, pi-alkyl, attractive charge, unfavourable bump, and halogen (fluorine) interactions were observed in some of the top hits, significantly influencing the binding affinities and docking scores (Table 3).
Table 3. Virtual screening done using benzothiazole-based compounds against Trehalase from An. funestus mosquito strain (UniProtKB: A0A182RST3).
The quantitative insecticidal-likeness (QEI) of the top nine compounds was assessed using six molecular descriptors along with the Tice rule and their respective scoring factors: molecular weight (MW of 150–500), lipophilicity (logP of 0–5), no. of hydrogen bond acceptors (HBA of 1–8), no. of hydrogen bond donors (HBD ≤2), no. of rotatable bond (RB ≤ 9), and no. of aromatic rings (arR) (Oduselu et al., 2019; Crisan et al., 2022). Among the compounds, PubChem IDs 60341156, 11055226, and 139744151 demonstrated the highest desirable QEI scores of 0.8226, 0.8177, and 0.6047 respectively (Table 4). All the compounds had MW within the acceptable range of 195.0–359 g/mol. The partition coefficient of a compound (log Po/w) is a measure of its lipophilicity. Table 5 provides the pharmacokinetic characteristics and potential toxicity risks of all the top hits, as determined by SWISSADME, ADMETlab, and BeeTox web servers. Concerns have been raised concerning how insecticides affect insect pollinators like bees. Further investigations were carried out to predict the toxicity level of the top nine hit compounds on bees using the BeeTox tool (BeeTox, 2019). The estimates indicated that some of the chemicals would not harm bees. The software determined that PubChem ID 60341156, which had the highest estimated QEI value and passed the Tice insecticidal regulations, was not hazardous to bees.
In Table 5, most compounds displayed a low likelihood of being non-biodegradable, with a probability of 0.55–0.56. The molecules had a lower synthetic accessibility score than Validamycin A with 6.34 and they may be simpler to synthesise than Validamycin A. Furthermore, only four compounds, namely, 153,773,424, 11,055,226, 60,341,156, and 67,693,703 had no structural brenk alert among the top hits (Adedeji et al., 2022). Similarly, ADMETlab was used in screening the compounds and most of the compounds were projected to be non-carcinogenic, non-sensitive to the skin, and non-irritant to the eye. According to ADMETlab predictions, among the top hits, compounds 59005679 and 135570533 had probabilities of 0.172 and 0.486 for DILI.
The scaffolds 135,570,533, 11,055,226, 135,451,258, 59,030,896, 59,005,679, 60,341,156, and 67,693,703 were further optimised using scaffold hopping available on the ADMETopt web server and screened to select non-toxic compounds. This yielded 240 compounds which were downloaded and further docked against AfTre and AgTre. Amongst the optimised compounds, Compound 1 (Z)-N,N'-(1-(2,6-dihydrobenzo[d]isothiazol-3-yl)prop-1-ene-1,2-diyl)bis(hydroxylamine)) had the highest binding energy of −8.7 kcal/mol which is predicted to be safe followed by compound 2 (Z)-N,N'-(1-(2,3-dihydrobenzo[d]isothiazol-5-yl)prop-1-ene-1,2-diyl)bis(hydroxylamine)) with a binding energy of −8.5 kcal/mol (Tables 5, 6).
The inhibitory activity of the control ligand, top hits, and optimised compounds were further investigated by docking against AgTre and compared to AfTre results as shown in Table 6. Among all other compounds, validamycin A had the lowest binding energy against AgTre (−5.1 kcal/mol), which was comparable to AfTre (6.3 kcal/mol). Compound 1 was predicted to have better binding energies for both AgTre (−8.7 kcal/mol) and AfTre (−8.2 kcal/mol). The 2D interactions of compounds 13,557,033, 1, and 2 with AfTre are shown in Figure 3. Compounds 1 and 2 had more hydrogen bonding interactions with the active site residue, resulting in better binding affinities than compound 13,557,033 which had a similar appearance but fewer hydrogen bonding interactions.
Figure 3. 2D and 3D interaction between AfTre and the best-hits (A) 2D interaction between compound (135,570,533) and AfTre; (B) 2D interaction between compound 1 and AfTre; (C) 2D interaction between compound 2 and AfTre; (D) 3D interaction between compound (135,570,533) and AfTre; (E) 3D interaction between compound 1 and AfTre; (F) 3D interaction between compound 2 and AfTre.
In an earlier discussion, the interaction between compounds 13557033, 1, and 2 with AfTre was investigated to examine the impact of the substituents and their location around the benzothiazole ring. After optimisation, the toxicity level of compound 1355703 was reduced owing to slight changes in the structural positioning. Some of the modifications include interchanging the nitrogen from position 3 to position 2 in the thiazole ring of compounds 1 and 2, hence converting them into isothiazoles, which are safer alternatives (Figure 4). Similarly, the bis-hydroxylamine substituent at position 3 to the sulphur in the thiazole ring was more advantageous for the interaction with AfTre. Optimisation increased the hydrogen bonding interaction in compounds 1 and 2 compared to that in compound 13557033. In designing several benzothiazepine compounds, Mostofi et al. (2018) conducted structural activity investigations. Bulky substituents at the para position of the thiazepine ring have been shown to improve the activity of the synthesised compounds compared to lighter substituents (Mostofi et al., 2018). Comprehensive SAR analyses will also aid in the optimisation of possible inhibitors that are environmentally safe.
The Molecular dynamics simulation was successfully carried out with AfTre in a complex with validamycin A (control ligand) and compound 1. The GROMACS analytic tools were used to gather and analyse the data.
Root Mean Square Deviation (RMSD) measures the difference between the initial structural conformation of the protein’s backbone and its final position. Root Mean Square Fluctuation (RMSF) examines the portions of a structure that deviate from its average structure. The variations obtained during the simulation can be used to estimate the protein’s stability relative to its conformation. A protein structure is considered more stable when it has a lower deviation from its original conformation, which can be determined by analysing the RMSD plot. The simulation runtime was 100 ns and it was observed from Figure 5A that the compound 1-protein complex experienced an increase within the first 20 ns before the initial rise. Then it maintained a steady pattern between 0.8 and 1.4 nm till the runtime was completed, suggesting that compound 1 was stable within the complex. When compared to the control ligand-protein complex which increased for the first 10 ns then continued between 0.6 and 1.7 nm for the next 90 ns showing a bit of instability within the complex (Gupta et al., 2020). Regions with high RMSF values indicate significant deviation from the average location, indicating considerable structural mobility while areas with rigidity have low RMSF values. Figure 5B displays that compound 1-protein complex had a lesser fluctuation at 9,100 atom residue with 2 nm as compared to the control ligand with a fluctuation level at 2.7 nm showing that compound 1 showed a level of stability (Adedeji et al., 2022).
Figure 5. MD simulation trajectory plot of AfTre with compound and control ligand. (A) Protein-ligand complex RMSDs during 100 ns MD simulation; (B) The RMSFs values protein backbone during 100 ns MD simulation.
The formation of hydrogen bonds is crucial for stabilising protein and protein-ligand complexes (Oduselu et al., 2023). Figure 6 depicts the number of h-bonds observed between the active site of AfTre with compounds 1 and validamycin A (control ligand). The total h-bond for Compound 1 was 9 averaging at 6 during the last ns corresponding to the conventional hydrogen interaction. Also, validamycin A had 12 h-bonds during the simulation and an average of 5 at 100 ns (Figure 4). The possible conventional hydrogen interaction of the active side amino residue with compound 1 was found between N-H, NH-OH, and NH-O.
Figure 6. Hydrogen bond formation between AfTre complexed with Compound 1 (black) and Control ligand (red).
The solvent-accessible surface area determines the effects of the solvent on protein-ligand complex stability and was estimated for compound 1 and Validamycin A (control ligand) (Figure 7). This analysis provides useful information about the surface area of the protein that is exposed to solvent molecules in a given environment (Akash et al., 2023). The SASA for compound 1 and Validamycin A showed little difference with some instability after 20 ns and 43 ns the trend became stable with an average SASA value of 425 and 415 nm2 respectively.
Figure 7. SASA analysis for AfTre (black) complexed with Compound 1 (red) and Control ligand (green).
The radius of gyration (Rg) helps in the calculation of the protein-ligand complex compactness. A higher Rg signifies a reduced compactness of the protein and a lower Rg signifies a compacted protein. Figure 8 shows the Rg plot; on the introduction of compound 1 within the protein the Rg value increased to 2.8 nm whereas that of the control ligand had a slight shift to 2.7 nm (Thapa et al., 2023). The trend observed in the compound 1-protein complex was similar to that of the protein compared to that of the control ligand. The findings suggest that the presence of the compounds caused some structural flipping in the AfTre protein.
Principal component analysis (PCA) transforms correlated protein atom movements to linearly independent principal components. According to the eigenvalue rank plots in Figure 9, the first three principal components for compounds 1 and validamycin A, respectively, accounted for 83.5% and 69.4% of the overall variance. This shows that rather than the much less prevalent motions, the molecular dynamics simulation approach captured the significant or dominant motions. For Compounds 1 and validamycin A, PC1 was responsible for 55.2% and 30% of the variance, respectively. When PC1 was considered, validamycin A had the lowest variation while Compound 1 had the most variance. Additionally, the observed blue, white, and red colour fluctuation in the PCA plots demonstrated the protein’s changing conformations (Ademuwagun et al., 2023).
Figure 9. PCA graphs for each data point accompanied by an eigenvalue rank plot (A) Compound 1; (B) Control ligand.
A possible insecticidal target is trehalase which can be used in developing inhibitors for Anopheles mosquito. The prediction of probable interaction modes and binding affinities of benzothiazole derivatives with model AfTre in this study serves as a template for designing potential insecticides. An. gambiae was used as a comparison model to reinforce the usage of the suggested compounds as potential trehalase inhibitors in mosquitoes. Compounds 1 (Z)-N,N'-(1-(2,6-dihydrobenzo[d]isothiazol-3-yl)prop-1-ene-1,2-diyl)bis(hydroxylamine)), compound 2 (Z)-N, N'-(1-(2,3-dihydrobenzo[d]isothiazol-5-yl)prop-1-ene-1,2-diyl)bis(hydroxylamine)), and compound 153773424 had binding affinities of −8.7, −8.5 and −7.9 kcal/mol while the control ligand had a binding affinity of −6.3 kcal/mol. This study looked at SAR studies, an important tool in designing and optimising active molecules. Additionally, the proposed compounds 153773424, 1, and 2 showed no potential bee toxicity and favourable in silico ADMET properties, proving their suitability for further synthesis and development into effective commercial insecticides.
The datasets presented in this study can be found in online repositories. The names of the repository/repositories and accession number(s) can be found in the article/supplementary material.
TO: Formal Analysis, Methodology, Software, Writing–original draft. GO: Software, Writing–review and editing. OE: Writing–review and editing. OA: Supervision, Writing–review and editing. EA: Funding acquisition, Writing–review and editing.
The author(s) declare that financial support was received for the research, authorship, and/or publication of this article. The National Institutes of Health funded this research through the Fogarty National Institutes of Health Common Fund (Grant No: U2RTW010679).
The authors are grateful to Covenant University for the provision of infrastructure.
The authors declare that the research was conducted in the absence of any commercial or financial relationships that could be construed as a potential conflict of interest.
All claims expressed in this article are solely those of the authors and do not necessarily represent those of their affiliated organizations, or those of the publisher, the editors and the reviewers. Any product that may be evaluated in this article, or claim that may be made by its manufacturer, is not guaranteed or endorsed by the publisher.
Abd El-Meguid, E. A., Mohi El-Deen, E. M., Moustafa, G. O., Awad, H. M., and Nossier, E. S. (2022). Synthesis, anticancer evaluation and molecular docking of new benzothiazole scaffolds targeting FGFR-1. Bioorg. Chem. 119, 105504. doi:10.1016/j.bioorg.2021.105504
Abraham, M. J., Murtola, T., Schulz, R., Páll, S., Smith, J. C., Hess, B., et al. (2015). Gromacs: high performance molecular simulations through multi-level parallelism from laptops to supercomputers. SoftwareX 1–2, 19–25. doi:10.1016/j.softx.2015.06.001
Adedeji, E. O., Oduselu, G. O., Ogunlana, O. O., Fatumo, S., Koenig, R., and Adebiyi, E. (2022). Anopheles gambiae trehalase inhibitors for malaria vector control: a molecular docking and molecular dynamics study. Insects 13, 1070. doi:10.3390/insects13111070
Adedeji, E. O., Ogunlana, O. O., Fatumo, S., Beder, T., Ajamma, Y., Koenig, R., et al. (2020). Anopheles metabolic proteins in malaria transmission, prevention and control: a review. Parasit. Vectors 13, 465–530. doi:10.1186/s13071-020-04342-5
Ademuwagun, I. A., Oduselu, G. O., Rotimi, S. O., and Adebiyi, E. (2023). Pharmacophore-aided virtual screening and molecular dynamics simulation identifies TrkB agonists for treatment of CDKL5-deficiency disorders. Bioinform. Biol. Insights 17, 117793222311582. doi:10.1177/11779322231158254
Akash, S., Bayıl, I., Hossain, M. S., Islam, M. R., Hosen, M. E., Mekonnen, A. B., et al. (2023). Novel computational and drug design strategies for inhibition of human papillomavirus-associated cervical cancer and DNA polymerase theta receptor by Apigenin derivatives. Sci. Rep. 13, 16565. doi:10.1038/s41598-023-43175-x
Akpan, G. E., Adepoju, K. A., Oladosu, O. R., and Adelabu, S. A. (2018). Dominant malaria vector species in Nigeria: modelling potential distribution of Anopheles gambiae sensu lato and its siblings with MaxEnt. PLoS One 13, e0204233. doi:10.1371/journal.pone.0204233
Altschul, S. F., Madden, T. L., Schäffer, A. A., Zhang, J., Zhang, Z., Miller, W., et al. (1997). Gapped BLAST and PSI-BLAST: a new generation of protein database search programs. Nucleic Acids Res. 25, 3389–3402. doi:10.1093/nar/25.17.3389
Avram, S., Funar-Timofei, S., Borota, A., Chennamaneni, S. R., Manchala, A. K., and Muresan, S. (2014). Quantitative estimation of pesticide-likeness for agrochemical discovery. J. Cheminform. 6, 42–11. doi:10.1186/s13321-014-0042-6
Azzam, K. A. L. (2023). SwissADME and pkCSM webservers predictors: an integrated online platform for accurate and comprehensive predictions for in silico ADME/T properties of artemisinin and its derivatives. Complex Use Min. Resour. 325, 14–21. doi:10.31643/2023/6445.13
BeeTox (2019). Chemyang. Available at: http://chemyang.ccnu.edu.cn/ccb/server/beetox/index.php/prediction/index (Accessed July 21, 2023).
Bray, S. A., Lucas, X., Kumar, A., and Grüning, B. A. (2020). The ChemicalToolbox: reproducible, user-friendly cheminformatics analysis on the Galaxy platform. J. Cheminform. 12, 40–47. doi:10.1186/s13321-020-00442-7
Chen, J., Tang, B., Chen, H., Yao, Q., Huang, X., Chen, J., et al. (2010). Different functions of the insect soluble and membrane-bound trehalase genes in chitin biosynthesis revealed by rna interference. PLoS One 5, 101333–e10213. doi:10.1371/journal.pone.0010133
Crawford, J. E., Guelbeogo, W. M., Sanou, A., Traoré, A., Vernick, K. D., Sagnon, N., et al. (2010). De novo transcriptome sequencing in anopheles funestus using illumina RNA-seq technology. PLoS One 5, e14202. doi:10.1371/journal.pone.0014202
Crisan, L., Funar-Timofei, S., and Borota, A. (2022). Homology modeling and molecular docking approaches for the proposal of novel insecticides against the african malaria mosquito (Anopheles gambiae). Molecules 27, 3846. doi:10.3390/molecules27123846
Ghurye, J., Koren, S., Small, S. T., Redmond, S., Howell, P., Phillippy, A. M., et al. (2019). A chromosome-scale assembly of the major African malaria vector Anopheles funestus. Gigascience 8, giz063–8. doi:10.1093/gigascience/giz063
Gupta, S., Kumar, A., Prakash, P., Sunita, K., Shuaib, M., Senapati, S., et al. (2020). Identification of potential natural inhibitors of SARS-CoV2 main protease by molecular docking and simulation studies. J. Biomol. Struct. Dyn. 0, 1–12. doi:10.1080/07391102.2020.1776157
Jampilek, J. (2019). Heterocycles in medicinal chemistry. Molecules 24, 3839–3842. doi:10.3390/molecules24213839
Kim, S., Chen, J., Cheng, T., Gindulyte, A., He, J., He, S., et al. (2023). PubChem 2023 update. Nucleic Acids Res. 51, D1373–D1380. doi:10.1093/nar/gkac956
Kumar, S., and Dubey, B. (2022). A review on emerging benzothiazoles: biological aspects. J. Drug Deliv. Ther. 12, 270–274. doi:10.22270/jddt.v12i4-s.5549
Kushwaha, P. P., Singh, A. K., Bansal, T., Yadav, A., Prajapati, K. S., Shuaib, M., et al. (2021). Identification of natural inhibitors against SARS-CoV-2 drugable targets using molecular docking, molecular dynamics simulation, and MM-PBSA approach. Front. Cell. Infect. Microbiol. 11, 730288–730317. doi:10.3389/fcimb.2021.730288
Lafzi, F., Kilic, D., Yildiz, M., and Saracoglu, N. (2021). Design, synthesis, antimicrobial evaluation, and molecular docking of novel chiral urea/thiourea derivatives bearing indole, benzimidazole, and benzothiazole scaffolds. J. Mol. Struct. 1241, 130566. doi:10.1016/j.molstruc.2021.130566
Lees, R., Praulins, G., Davies, R., Brown, F., Parsons, G., White, A., et al. (2019). A testing cascade to identify repurposed insecticides for next-generation vector control tools: screening a panel of chemistries with novel modes of action against a malaria vector. Gates Open Res. 3, 1464–1525. doi:10.12688/gatesopenres.12957.2
Liu, K., Dong, Y., Huang, Y., Rasgon, J. L., and Agre, P. (2013). Impact of trehalose transporter knockdown on Anopheles gambiae stress adaptation and susceptibility to Plasmodium falciparum infection. Proc. Natl. Acad. Sci. U. S. A. 110, 17504–17509. doi:10.1073/pnas.1316709110
Maienfisch, P., and Edmunds, A. J. F. (2017). Thiazole and isothiazole ring–containing compounds in crop protection. Advances in heterocyclic chemistry, 35–88. doi:10.1016/bs.aihch.2016.04.010
Marten, A. D., Stothard, A. I., Kalera, K., Swarts, B. M., and Conway, M. J. (2020). Validamycin A delays development and prevents flight in Aedes aegypti (Diptera: Culicidae). J. Med. Entomol. 57, 1096–1103. doi:10.1093/jme/tjaa004
Mostofi, M., Mohammadi Ziarani, G., and Lashgari, N. (2018). Design, synthesis and biological evaluation of benzofuran appended benzothiazepine derivatives as inhibitors of butyrylcholinesterase and antimicrobial agents. Bioorg. Med. Chem. 26, 3076–3095. doi:10.1016/j.bmc.2018.02.049
Nambunga, I. H., Ngowo, H. S., Mapua, S. A., Hape, E. E., Msugupakulya, B. J., Msaky, D. S., et al. (2020). Aquatic habitats of the malaria vector Anopheles funestus in rural south - eastern Tanzania. Malar. J. 19, 219–311. doi:10.1186/s12936-020-03295-5
Neyman, V., Quicray, M., Francis, F., and Michaux, C. (2024). Toxicological, biochemical, and in silico investigations of three trehalase inhibitors for new ways to control aphids. Arch. Insect Biochem. Physiol. 115, e22112–e22114. doi:10.1002/arch.22112
Nolden, M., Velten, R., Paine, M. J. I., and Nauen, R. (2023). Resilience of transfluthrin to oxidative attack by duplicated CYP6P9 variants known to confer pyrethroid resistance in the major malaria mosquito Anopheles funestus. Pestic. Biochem. Physiol. 191, 105356. doi:10.1016/j.pestbp.2023.105356
Oduselu, G. O., Afolabi, R., Ademuwagun, I., Vaughan, A., and Adebiyi, E. (2023). Structure-based pharmacophore modeling, virtual screening, and molecular dynamics simulation studies for identification of Plasmodium falciparum 5-aminolevulinate synthase inhibitors. Front. Med. 9, 1022429–1022515. doi:10.3389/fmed.2022.1022429
Oduselu, G. O., Ajani, O. O., Ajamma, Y. U., Brors, B., and Adebiyi, E. (2019). Homology modelling and molecular docking studies of selected substituted benzo[d]imidazole-1-yl methyl benzimidamide scaffolds on Plasmodium falciparum adenylosuccinate lyase receptor. Bioinform. Biol. Insights 13, 1–10. doi:10.1177/1177932219865533
Okorie, P. N., McKenzie, F. E., Ademowo, O. G., Bockarie, M., and Kelly-Hope, L. (2011). Nigeria Anopheles vector database: an overview of 100 years’ research. PLoS One 6, e28347–7. doi:10.1371/journal.pone.0028347
Olayinka, O. O. (2019). Nipping the malaria vectors in the bud: focus on Nigeria. Malaria, 1–11. doi:10.5772/intechopen.83554
Parrinello, M., and Rahman, A. (1981). Polymorphic transitions in single crystals: a new molecular dynamics method. J. Appl. Phys. 52, 7182–7190. doi:10.1063/1.328693
Saravanan, M., Ramachandran, B., and Barabadi, H. (2018). The prevalence and drug resistance pattern of extended spectrum β–lactamases (ESBLs) producing Enterobacteriaceae in Africa. Microb. Pathog. 114, 180–192. doi:10.1016/j.micpath.2017.11.061
Serazin, A. C., Dana, A. N., Hillenmeyer, M. E., Lobo, N. F., Coulibaly, M. B., Willard, M. B., et al. (2009). Comparative analysis of the global transcriptome of Anopheles funestus from Mali, West Africa. PLoS One 4, e7976. doi:10.1371/journal.pone.0007976
Shukla, E., Thorat, L. J., Nath, B. B., and Gaikwad, S. M. (2015). Insect trehalase: physiological significance and potential applications. Glycobiology 25, 357–367. doi:10.1093/glycob/cwu125
Singh, A., Malhotra, D., Singh, K., Chadha, R., and Bedi, P. M. S. (2022). Thiazole derivatives in medicinal chemistry: recent advancements in synthetic strategies, structure activity relationship and pharmacological outcomes. J. Mol. Struct. 1266, 133479. doi:10.1016/j.molstruc.2022.133479
Thapa, S., Suresha Biradar, M., Nargund, S. L., Ahmad Ravindra, I., Patel C Patel, H. R., and Lamsal, A. (2023). Synthesis, molecular docking, molecular dynamic simulation studies, and anti-tubercular activity evaluation of substituted benzimidazole derivatives. Adv. Pharmacol. Pharm. Sci. 2024, 1–14. doi:10.21203/rs.3.rs-3151345/v1
WHO (2022). Framework for the allocation of limited malaria vaccine supply. Available at: https://cdn.who.int/media/docs/default-source/immunization/mvip/framework-for-allocation-of-limited-malaria-vaccine-supply.pdf?sfvrsn=35b12e4_2&download=true.
Xiong, G., Wu, Z., Yi, J., Fu, L., Yang, Z., Hsieh, C., et al. (2021). ADMETlab 2.0: an integrated online platform for accurate and comprehensive predictions of ADMET properties. Nucleic Acids Res. 49, W5–W14. doi:10.1093/nar/gkab255
Yang, H., Sun, L., Wang, Z., Li, W., Liu, G., and Tang, Y. (2018). ADMETopt: a web server for ADMET optimization in drug design via scaffold hopping. J. Chem. Inf. Model. 58, 2051–2056. doi:10.1021/acs.jcim.8b00532
Zhong, F., Yu, L., Jiang, X., Chen, Y., Wang, S., Chao, L., et al. (2023). Potential inhibitory effects of compounds ZK-PI-5 and ZK-PI-9 on trehalose and chitin metabolism in Spodoptera frugiperda (J. E. Smith). Front. Physiol. 14, 1178996–1179012. doi:10.3389/fphys.2023.1178996
Keywords: ADMET properties, benzothiazole, insecticidal activity, malaria, vector control
Citation: Ogunnupebi TA, Oduselu GO, Elebiju OF, Ajani OO and Adebiyi E (2024) In silico studies of benzothiazole derivatives as potential inhibitors of Anopheles funestus and Anopheles gambiae trehalase. Front. Bioinform. 4:1428539. doi: 10.3389/fbinf.2024.1428539
Received: 06 May 2024; Accepted: 17 July 2024;
Published: 09 August 2024.
Edited by:
Tallulah Andrews, Western University, CanadaReviewed by:
Uchechukwu Chris Okoro, University of Nigeria, NigeriaCopyright © 2024 Ogunnupebi, Oduselu, Elebiju, Ajani and Adebiyi. This is an open-access article distributed under the terms of the Creative Commons Attribution License (CC BY). The use, distribution or reproduction in other forums is permitted, provided the original author(s) and the copyright owner(s) are credited and that the original publication in this journal is cited, in accordance with accepted academic practice. No use, distribution or reproduction is permitted which does not comply with these terms.
*Correspondence: Olayinka O. Ajani, b2xhLmFqYW5pQGNvdmVuYW50dW5pdmVyc2l0eS5lZHUubmc=; Ezekiel Adebiyi, ZS5hZGViaXlpQGRrZnotaGVpZGVsYmVyZy5kZQ==, ZXpla2llbC5hZGViaXlpQGNvdmVuYW50dW5pdmVyc2l0eS5lZHUubmc=
Disclaimer: All claims expressed in this article are solely those of the authors and do not necessarily represent those of their affiliated organizations, or those of the publisher, the editors and the reviewers. Any product that may be evaluated in this article or claim that may be made by its manufacturer is not guaranteed or endorsed by the publisher.
Research integrity at Frontiers
Learn more about the work of our research integrity team to safeguard the quality of each article we publish.