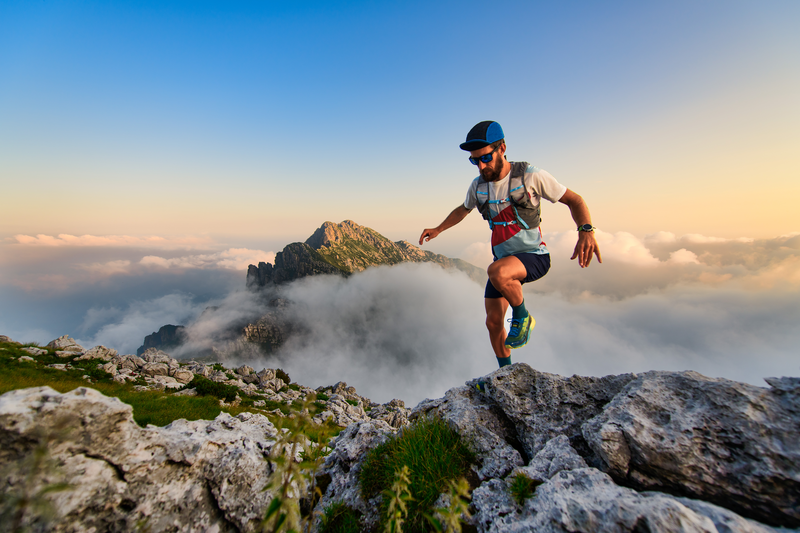
95% of researchers rate our articles as excellent or good
Learn more about the work of our research integrity team to safeguard the quality of each article we publish.
Find out more
ORIGINAL RESEARCH article
Front. Bioeng. Biotechnol.
Sec. Industrial Biotechnology
Volume 13 - 2025 | doi: 10.3389/fbioe.2025.1567923
The final, formatted version of the article will be published soon.
You have multiple emails registered with Frontiers:
Please enter your email address:
If you already have an account, please login
You don't have a Frontiers account ? You can register here
Protein aggregation, denaturation, and loss of potency often occur during Protein A chromatography due to the harsh acidic conditions required for antibody elution. This study presents a pH shift-based elution strategy that effectively mitigates these issues by introducing amino acid-based elution buffers to create a milder elution environment and increase the final elution pH. By optimizing the combination of pre-elution and elution buffers, the elution pool pH was increased up to 7.2, significantly enhancing protein stability. Among various elution buffers tested, amino acids with non-polar or polar uncharged side chains-such as leucine, glycine, and serine-exhibited the most effective pH transition, resulting in 0.5-2.9 units pH shifts. Additionally, the use of 50 mM Bis-Tris, pH 7.2 as a pre-elution buffer demonstrated the highest capacity for stabilizing pH shifts. The scalability of this approach was validated using a 10 cm diameter column, where yields remained comparable to small-scale experiments, and elution pool stability was able to be maintained for 72 hours at 26°C. These findings establish pH-shifting elution as a scalable, cost-effective method for improving the recovery and stability of low pH-unstable therapeutic antibodies in Protein A chromatography.
Keywords: pH Shift, low pH unstable protein, Protein A chromatography, amino acid-based elution, Downstream purification
Received: 28 Jan 2025; Accepted: 17 Mar 2025.
Copyright: © 2025 Senzhu and Yue. This is an open-access article distributed under the terms of the Creative Commons Attribution License (CC BY). The use, distribution or reproduction in other forums is permitted, provided the original author(s) or licensor are credited and that the original publication in this journal is cited, in accordance with accepted academic practice. No use, distribution or reproduction is permitted which does not comply with these terms.
* Correspondence:
Lin Senzhu, WuXi Biologics, Shanghai, China
Disclaimer: All claims expressed in this article are solely those of the authors and do not necessarily represent those of their affiliated organizations, or those of the publisher, the editors and the reviewers. Any product that may be evaluated in this article or claim that may be made by its manufacturer is not guaranteed or endorsed by the publisher.
Research integrity at Frontiers
Learn more about the work of our research integrity team to safeguard the quality of each article we publish.