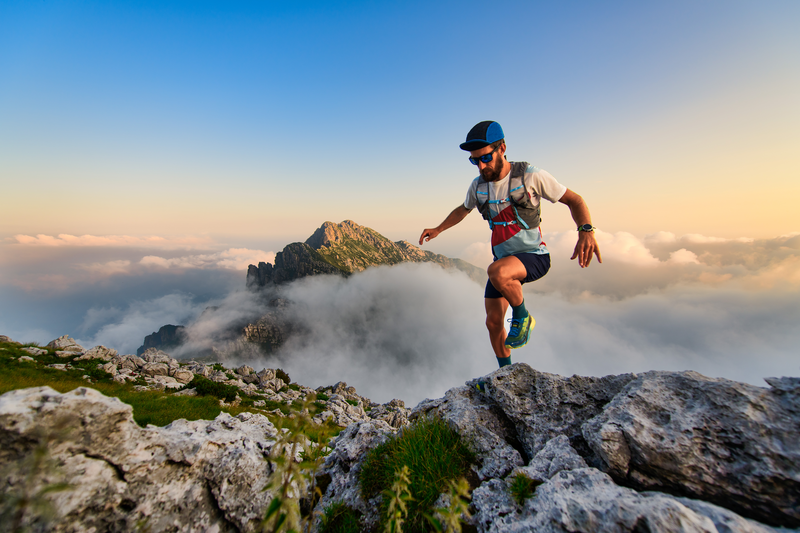
94% of researchers rate our articles as excellent or good
Learn more about the work of our research integrity team to safeguard the quality of each article we publish.
Find out more
REVIEW article
Front. Bioeng. Biotechnol.
Sec. Biomaterials
Volume 13 - 2025 | doi: 10.3389/fbioe.2025.1549439
The final, formatted version of the article will be published soon.
You have multiple emails registered with Frontiers:
Please enter your email address:
If you already have an account, please login
You don't have a Frontiers account ? You can register here
Titanium (Ti) implants are widely used in orthopedic and dental applications due to their excellent mechanical strength, corrosion resistance, and biocompatibility. However, their limited osteointegration and susceptibility to bacterial infections remain major clinical challenges. Recent advancements in surface modification techniques have significantly improved the osteogenic and antibacterial properties of Ti implants. This review summarizes key strategies, including ion doping, hydroxyapatite (HAp) coatings, nanostructured surfaces, and graphene-based modifications. Zinc (Zn)-doped coatings increase osteoblast proliferation by 25%, enhance cell adhesion by 40%, and inhibit Staphylococcus aureus by 24%. Magnesium (Mg)-doped Ti surfaces enhance osteoblast differentiation, with 38% increased alkaline phosphatase (ALP) activity and a 4.5-fold increase in cell proliferation. Copper (Cu)-doped coatings achieve 99.45% antibacterial efficacy against S. aureus and 98.65% against Escherichia coli (E. coli). Zn-substituted HAp promotes mineralized nodule formation by 4.5-fold and exhibits 16.25% bacterial inhibition against E. coli. Graphene-based coatings stimulate bone marrow stem cells (BMSCs) and provide light-responsive surface potentials for enhanced osteogenesis. Despite these advancements, challenges remain in optimizing ion release kinetics and long-term stability. Future research should focus on multi-functional coatings that integrate osteogenic, antibacterial, and immunomodulatory properties to enhance clinical performance and patient outcomes. Highlight: (1) Innovative Surface Modification Strategies: Integrating metal doping, HAp coatings, and graphene modifications to significantly enhance osseointegration, antibacterial properties, and biocompatibility.(2) Synergistic Effects of Multifunctional Coatings: Exploring interactions among metal ions, HAp, and graphene composite coatings to optimize osteogenesis, antibacterial efficacy, and mechanical properties.(3) Addressing Clinical Translation Challenges: Focusing on ion release control, long-term stability, and large-scale manufacturing to advance personalized implants and clinical applications.
Keywords: Titanium, Biomechanical, Surface modification, Osteointegration, Antibacterial, Macrophage polarization
Received: 02 Jan 2025; Accepted: 24 Feb 2025.
Copyright: © 2025 Zhang, Wu, Wang, Yan, Duan and Sun. This is an open-access article distributed under the terms of the Creative Commons Attribution License (CC BY). The use, distribution or reproduction in other forums is permitted, provided the original author(s) or licensor are credited and that the original publication in this journal is cited, in accordance with accepted academic practice. No use, distribution or reproduction is permitted which does not comply with these terms.
* Correspondence:
Xudong Duan, Department of Orthopedic Surgery, The First Affiliated Hospital of Shandong First Medical University & Shandong Provincial Qianfoshan Hospital, Jinan, Shandong Province, China
Huaqiang Sun, Department of Orthopedic Surgery, The First Affiliated Hospital of Shandong First Medical University & Shandong Provincial Qianfoshan Hospital, Jinan, Shandong Province, China
Disclaimer: All claims expressed in this article are solely those of the authors and do not necessarily represent those of their affiliated organizations, or those of the publisher, the editors and the reviewers. Any product that may be evaluated in this article or claim that may be made by its manufacturer is not guaranteed or endorsed by the publisher.
Research integrity at Frontiers
Learn more about the work of our research integrity team to safeguard the quality of each article we publish.