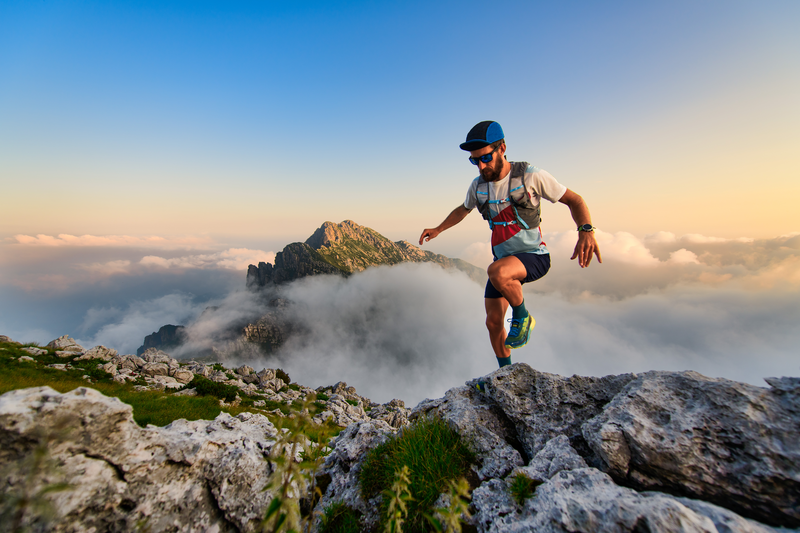
94% of researchers rate our articles as excellent or good
Learn more about the work of our research integrity team to safeguard the quality of each article we publish.
Find out more
REVIEW article
Front. Bioeng. Biotechnol.
Sec. Biomaterials
Volume 13 - 2025 | doi: 10.3389/fbioe.2025.1547343
This article is part of the Research Topic Advanced Functional Materials for Disease Diagnosis, Drug Delivery and Tissue Repair View all 15 articles
The final, formatted version of the article will be published soon.
You have multiple emails registered with Frontiers:
Please enter your email address:
If you already have an account, please login
You don't have a Frontiers account ? You can register here
Diabetes is a widespread metabolic disorder that presents considerable challenges in its management. Recent advancements in biomaterial research have shed light on innovative approaches for the treatment of diabetes. This review examines the role of biomaterials in diabetes diagnosis and treatment, as well as their application in managing diabetic wounds. By evaluating recent research developments alongside future obstacles, the review highlights the promising potential of biomaterials in diabetes care, underscoring their importance in enhancing patient outcomes and refining treatment methodologies.
Keywords: Biomaterials, diabetes, insulin delivery, Tissue Engineering, Biosensors
Received: 18 Dec 2024; Accepted: 30 Jan 2025.
Copyright: © 2025 Guan and Zhang. This is an open-access article distributed under the terms of the Creative Commons Attribution License (CC BY). The use, distribution or reproduction in other forums is permitted, provided the original author(s) or licensor are credited and that the original publication in this journal is cited, in accordance with accepted academic practice. No use, distribution or reproduction is permitted which does not comply with these terms.
* Correspondence:
Liang Zhang, Department of Human Anatomy, School of Basic Medicine, Shenyang Medical College, Shenyang, China
Disclaimer: All claims expressed in this article are solely those of the authors and do not necessarily represent those of their affiliated organizations, or those of the publisher, the editors and the reviewers. Any product that may be evaluated in this article or claim that may be made by its manufacturer is not guaranteed or endorsed by the publisher.
Research integrity at Frontiers
Learn more about the work of our research integrity team to safeguard the quality of each article we publish.