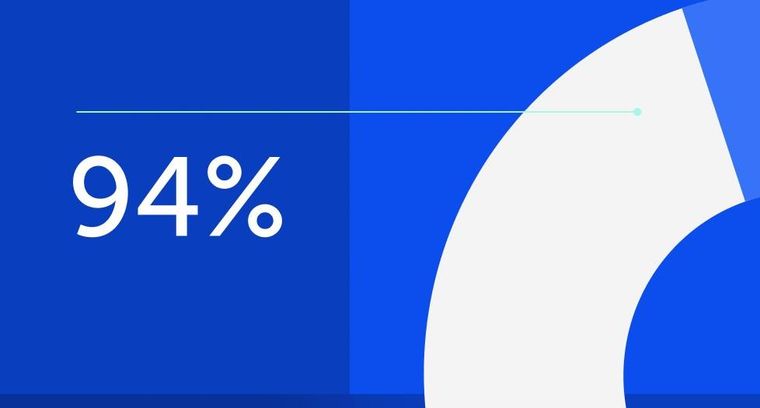
94% of researchers rate our articles as excellent or good
Learn more about the work of our research integrity team to safeguard the quality of each article we publish.
Find out more
REVIEW article
Front. Bioeng. Biotechnol., 27 March 2025
Sec. Tissue Engineering and Regenerative Medicine
Volume 13 - 2025 | https://doi.org/10.3389/fbioe.2025.1492811
Tendon injury is one of the most common musculoskeletal disorders that severely affect patients’ daily lives. Unfortunately, naturally healed tendons exhibit poor quality, as they have very limited regenerative ability. Recently, therapeutic strategies involving the administration of growth factors have been advocated to enhance tendon regenerative healing. Growth factors are peptide-signaling molecules that elicit biological functions such as cell proliferation, migration, and differentiation by acting through a complex organization of cell surface receptors and activating intracellular signaling pathways. Insulin-like growth factor-1(IGF-1) represents one such factor that has shown promising effects for enhancing tendon regenerative healing in vitro and animal models. However, it is disappointing that IGF-1 has not been shown to play a very significant role in promoting tendon healing in clinical trials, which could reflect our poor understanding of the molecular mechanisms by which IGF-1 is involved in promoting tendon regenerative healing. Therefore, in this review, we summarized the roles and mechanisms of IGF-1 for enhancing tendon regenerative healing. Nevertheless, much work is still needed to optimize its effectiveness.
Tendon injury is a common and disabling musculoskeletal condition, accounting for over 30% of all musculoskeletal consultations (Wang et al., 2019a). Unfortunately, the innate limited spontaneous regenerative capability of tendons poses a substantial treating challenge for clinicians as the healed tendons often heal through the formation of scar tissue with the inferior biomechanical and biological properties compared to the intact tendon tissue (Li S. et al., 2023). Current therapeutic strategies for tendon injury can be broadly divided into operative or conservative treatments (Adjei-Sowah et al., 2023). When conservative treatments fail, treatments frequently resort to surgical treatment (Liang et al., 2024). Unfortunately, both conservative and surgical approaches for managing tendon injuries present significant limitations (Silbernagel et al., 2020). Conservative treatment typically requires prolonged healing periods, ranging from 4 to 6 weeks for mild injuries, 6–12 weeks for moderate cases, and up to 3–6 months or longer for severe injuries (Kosiol et al., 2023). Similarly, surgical intervention entails an extended recovery timeline, with an average of 6–8 weeks for initial wound healing and several months for full functional restoration (Reda et al., 2020). In terms of re-rupture rates, conservative treatment is associated with a risk of 10%–30%, influenced by factors such as injury type, severity, and patient adherence to rehabilitation protocols (Seow et al., 2023). Although surgical treatment demonstrates a comparatively lower re-rupture rate (5%–15%), it still poses a substantial clinical challenge. Given these limitations, there is an urgent need to develop novel therapeutic strategies to improve outcomes in tendon injury management.
Recent studies have highlighted the potential of various growth factors in promoting tendon regenerative healing. Preclinical research has demonstrated that factors such as vascular endothelial growth factor (VEGF), fibroblast growth factor-2 (FGF-2), transforming growth factor-β1 (TGF-β1), and insulin-like growth factor-1(IGF-1) may contribute to tendon repair (Lai et al., 2022; Guo et al., 2020; Deng et al., 2024). However, their therapeutic applications are limited by significant drawbacks. For instance, VEGF has been shown to disrupt the extracellular matrix (ECM) of tendon tissues (Liu et al., 2021), while TGF-β1 is associated with the formation of adhesions and scar tissue, leading to fibrosis and restricted tendon mobility (Li H. et al., 2023). Furthermore, exogenous administration of FGF-2 in a canine model failed to improve the biomechanical properties of injured tendons (Thomopoulos et al., 2010).
Compared to other growth factors, compelling evidence suggests that IGF-1 plays a pivotal role in enhancing the regenerative potential of injured tendons. For instance, Kurtz et al. demonstrated that exogenous IGF-1 administration reduces functional deficits and accelerates recovery in a rat Achilles tendon injury model (Kurtz et al., 1999). In vitro studies further support its therapeutic potential, showing that IGF-1 modulates inflammatory responses, stimulates tenocyte proliferation and migration, and enhances collagen synthesis (Gulotta and Rodeo, 2009; El Essawy and Baar, 2023). However, despite its success in preclinical models, clinical trials have shown limited efficacy of IGF-1 in promoting sustained recovery in patients with tendinopathy (Olesen et al., 2021). These discrepancies may stem from an incomplete understanding of the mechanisms underlying IGF-1’s actions during tendon healing. In this review, we aim to summarize the roles and mechanisms of IGF-1 in tendon regenerative healing, providing insights into its potential clinical applications for patients with tendon injuries. Nevertheless, further research is essential to optimize its therapeutic effectiveness and translate preclinical findings into clinical success.
Approximately 90% of the papers mentioned in this review (total number of papers included in this review: 103) were found by a search in the Web of Science/Google Scholar databases using the keywords “tendon injury, IGF-1, tendon regenerative healing, tendon repair, tenocyte, mechanisms” (the search was performed during the period of May to September 2024 and only literature written in English was reviewed) (Figure 1). The remaining 10% were cited in the references found within this search, with the exception of some very recent papers. The reviewed literature was published from 1988–2024. Original research papers and review papers were included. We focused on IGF-1 administration to tendon injury, either by single injection or released in a sustained manner from a delivery device. Additionally, full transections of tendons, partial lacerations, and tendinopathy animal models, such as collagenase-induced tendinopathy, were included and the effects and mechanisms of IGF-1 on the healing process were discussed.
IGF-1, organically named somatomedin C, is a multifunctional peptide mitogen possessing a molecular weight of 7,649 daltons (Geffken et al., 2022). It consists of 70 highly conserved amino acids in a single chain with three intramolecular disulfide bridges, with high structural homology with insulin (Al-Samerria and Radovick, 2021). IGF-1 is synthesized in various tissues like the liver, skeletal muscle, bone, and tendon (Lygren et al., 2013). Significantly, the liver is the main source, accounting for 80% of IGF-1 synthesis and secretion. The remaining 20% is synthesized locally, often by connective tissue cells (Roberts et al., 2023).
Following either local secretion or systemic circulation to target tissues, IGF-1 primarily exerts its biological effects through binding to the IGF-1 receptor (IGF-1R), with minimal interaction occurring through the insulin receptor (IR) (Figure 2) (Werner, 2023). The IGF-1R, which is ubiquitously expressed across nearly all cell types in the organism, consists of two α and two β subunits linked by disulfide bonds (Bailes and Soloviev, 2021). The β subunits contain intracellular tyrosine kinase domains that are activated upon IGF-1 binding to the extracellular α subunits, initiating downstream signaling cascades (Muraguchi et al., 2019). Ligand binding to IGF-1R triggers tyrosine kinase domain activation, resulting in autophosphorylation of tyrosine residues (Rieger and O'Connor, 2020). This phosphorylation event subsequently activates various substrates, including insulin receptor substrate (IRS) and SRC homology 2 domain-containing protein (SHC), which in turn recruit additional signaling molecules to activate two major intracellular pathways: the phosphoinositide 3-kinase (PI3K)/serine-threonine kinase (AKT) pathway and the mitogen-activated protein kinase (MAPK)/extracellular signal-regulated kinase (ERK) pathway (Wang J. et al., 2023; Scott et al., 2005). The MAPK/ERK signaling cascade primarily mediates cellular proliferation, while the PI3K/AKT pathway predominantly regulates cell survival and apoptosis (Macvanin et al., 2023; Matsushita et al., 2022). Notably, research has demonstrated that ginsenoside Rg1-mediated activation of IGF-1R and MAPK signaling pathways significantly enhances tendon healing in a rat Achilles tendon injury model, underscoring the crucial role of these signaling pathways in tendon repair processes (Wu et al., 2022).
In addition to IGF-1R, insulin-like growth factor binding proteins (IGFBPs) are also required for IGF-1 bioavailability because they interact with IGF-1 with the same affinity as IGF-1R (Perks, 2023). IGFBPs are synthesized by various cell types, including hepatocytes, fibroblasts, and osteoblasts (Zerti et al., 2021). As primarily secreted proteins, IGFBPs are released into the extracellular space, where they interact with IGF-1 (Oxvig and Conover, 2023). These proteins can circulate in the bloodstream or reside in tissue interstitial fluid, enabling them to bind freely circulating IGF-1 or IGF-1 associated with cell-surface receptors (Wang et al., 2021). Interestingly, emerging evidence indicates that IGFBPs can also localize to the cell membrane, where they may interact with membrane receptors or other surface-associated proteins (Tian et al., 2024). This membrane-bound form of IGFBPs plays a regulatory role in IGF-1 signaling, either by modulating IGF-1 binding to its receptors or by initiating distinct signaling pathways, thereby fine-tuning cellular responses (Zhang et al., 2023). It has been reported that IGFBPs can bind approximately 98% of all circulating IGF-1, and their binding affinity for IGF-1 is nearly 10 times higher than that for IGF-1R (Zhong et al., 2023). Thus, the function and concentration of IGFBPs are critical to regulating the biological action of IGF-1. In a rat Achilles tendon injury model, Western blot and immunobiological staining results showed more IGF-1 in the injured tendon following local administration of IGFBP-4. Downstream of IGF-1, AKT was activated and phosphorylated, subsequently promoting tendon healing (Wang H. et al., 2023).
Following a tendon injury, the healing process unfolds through three overlapping phases: (1) the inflammatory phase, (2) the proliferative phase, and (3) the remodeling phase (Leong et al., 2020). The inflammatory phase initiates within minutes post-injury and persists for several days, marked by the dynamic recruitment of immune cells. These cells secrete a cascade of proinflammatory cytokines and growth factors, including VEGF, TGF-β, and IGF-1, which amplify the inflammatory response (Alim et al., 2020). Subsequently, the proliferative phase commences, lasting several weeks. During this stage, there is a significant increase in ECM production—primarily type III collagen—and cellularity, predominantly tenocytes (Leahy et al., 2023). Macrophages transition from a proinflammatory to a reparative phenotype, releasing growth factors and chemoattractants that promote tenocyte proliferation and recruitment (Li H. et al., 2023). The remodeling phase begins 1–2 months after injury and can extend beyond a year. This phase is characterized by a shift toward collagen type I synthesis and improved ECM alignment (Li et al., 2024). Concurrently, tenocyte density and synthetic activity gradually decline. Despite these repair mechanisms, the healing process often results in fibrotic tissue formation around the injury site, characterized by disorganized and excessive ECM deposition. This fibrotic tissue is mechanically inferior to native tendon and contributes to functional impairment (Korcari et al., 2022).
A controlled delivery strategy for IGF-1, which accounts for the spatiotemporal complexity of the injury microenvironment and synergizes with endogenous IGF-1 production through feedback mechanisms, holds significant promise for enhancing IGF-1 therapy (Prabhath et al., 2018). Therefore, understanding the endogenous expression profile of IGF-1 during tendon healing is crucial for determining the optimal timing of exogenous IGF-1 administration. In the following sections, we review studies investigating IGF-1 expression during tendon healing in animal models, categorized based on the timing of IGF-1 expression peaks.
In the early-peaked studies, Hansson et al. observed a transient increase in IGF-1 expression at day 3 following vibration-induced Achilles tendon injury in rats (Hansson et al., 1988). Similarly, in a chicken flexor tendon repair model, IGF-1 expression was significantly upregulated at day 3 but returned to baseline levels by day 9 and remained stable through day 21 (Chen et al., 2008).
In late-peak studies, In a rat supraspinatus tendon injury model induced by overloading, IGF-1 immunostaining was minimal at 4 and 8 weeks but markedly increased at 12 and 16 weeks (Scott et al., 2007). Dahlgren et al. reported a 40% decrease in IGF-1 protein levels during the early stages of tendon healing compared to intact tendons, with levels peaking at 4 weeks and remaining elevated through 8 weeks post-injury (Dahlgren et al., 2005). In a rabbit patellar tendon model, Lyras et al. found that IGF-1 expression peaked at week three and gradually declined thereafter. Notably, IGF-1 expression was predominantly localized in inflammatory and endothelial cells during the first 2 weeks, shifting to tenocytes in the later stages of healing (Lyras et al., 2010). Another study using a rat flexor tendon transection-and-repair model demonstrated significantly elevated IGF-1 mRNA and protein levels at weeks four and eight compared to controls (Tang et al., 2019). Similarly, Berglund et al. observed a modest increase in IGF-1 mRNA levels by the end of the first week in a rabbit flexor tendon repair model, which surged nearly six-fold by week three and remained elevated at week 6 (Berglund et al., 2011).
In the single-time-point study, Tsubone et al. investigated IGF-1 expression in a canine flexor tendon repair model at 10 days post-operation using immunohistochemical staining, revealing a significant increase in IGF-1 levels (Tsubone et al., 2004). Kobayashi et al. reported elevated IGF-1 expression at day 5 in a rabbit supraspinatus tendon healing model (Kobayashi et al., 2006).
Although the timing of IGF-1 upregulation varies across studies, likely due to differences in species, experimental methods, and tendon locations, the consensus is that IGF-1 expression is significantly elevated during tendon healing. These findings deepen our understanding of the relationship between IGF-1 and tendon repair and provide a foundation for optimizing IGF-1 delivery strategies to enhance healing outcomes.
Numerous in vivo studies using animal models have demonstrated the therapeutic benefits of local IGF-1 treatment in tendon healing (Table 1).
Kurtz et al. investigated the effects of IGF-1 in a rat Achilles tendon transection model. Rats were divided into three groups: sham surgery, transection alone, and transection with recombinant human IGF-1 (rhIGF-1) injection (25 μg). The results revealed that IGF-1 enhanced DNA, collagen, and proteoglycan synthesis, accelerating functional recovery by day 15. However, no significant improvement in tendon biomechanical failure loads was observed compared to untreated controls (Kurtz et al., 1999).
Following the above study, Dahlgren et al. explored IGF-1’s effects in a collagenase-induced equine flexor tendon injury model. Equines were divided into a control group (0.9% NaCl injections) and an experimental group (0.2 μg rhIGF-1 injections every other day for 10 doses). Evaluations at 0, 2, 3-, 4-, 6-, and 8-week post-treatment demonstrated that the rhIGF-1-treated group exhibited increased cell proliferation, improved healing quality, enhanced collagen content, reduced tissue swelling, and superior mechanical properties compared to controls (Dahlgren et al., 2002).
Furthermore, in a rat model of Achilles tendon injury, Li et al. developed an innovative drug delivery system utilizing clay nanoparticles to enhance rhIGF-1 adsorption through their large surface area. These nanoparticles were subsequently assembled into microparticles and physically entrapped within a toughened hydrogel network. The hydrogel system was fabricated through a facile approach that combined clay nanoparticle incorporation, biodegradable hydrogel formation, and lyophilization treatment, achieving sustained and controlled release of rhIGF-1 at a concentration of 8 mg/mL. Notably, the IGF-1-treated group demonstrated significantly enhanced tendon biomechanical properties compared to the control group without growth factor treatment. Histological analysis further confirmed substantial improvement in tendon healing quality after 2 weeks of treatment (Li et al., 2018).
Despite its therapeutic potential, rhIGF-1 encounters several clinical limitations, primarily due to its structural homology with insulin, which may lead to hypoglycemia and growth hormone (GH) suppression (Jozefiak et al., 2021). To overcome these challenges, researchers have developed pegylated IGF-1, which preserves anabolic activity while mitigating adverse effects through reduced ligand-receptor interaction kinetics (Liang et al., 2022). Furthermore, rhIGF-1’s therapeutic application is constrained by its short in vivo half-life and susceptibility to proteolytic degradation. In comparison, chemically modified IGF-1 mimics demonstrate superior stability profiles. To evaluate these advantages, Prabhath et al. conducted a comprehensive study examining the effects of pegylated IGF-1 mimics in a rat supraspinatus tendon model. The researchers encapsulated both pegylated IGF-1 mimics and control formulations (unpegylated IGF-1 mimics and rhIGF-1) within polycaprolactone-based matrices, enabling localized and sustained delivery to the repaired tendons. The experimental results demonstrated that pegylated IGF-1 mimic treatment significantly reduced fibrotic scar formation, enhanced tendon structural regeneration, and improved biomechanical properties at 8 weeks post-treatment compared to control groups (Prabhath et al., 2022).
Gene delivery offers a promising alternative by introducing exogenous genetic material into cells to modulate protein expression. This approach ensures natural protein synthesis by host cells, avoiding reduced bioactivity and immune responses associated with exogenous biomolecule delivery (Jin et al., 2022; Ling et al., 2023). It also enables long-term protein release, reducing the need for repeated injections (Boye et al., 2022). Dines et al. demonstrated the efficacy of IGF-1 gene delivery in a rat rotator cuff tendon repair model. Rat tendon fibroblasts were transduced with an IGF-1 gene via a retroviral vector and seeded onto a bioabsorbable PGA scaffold. After 6 weeks, the gene-modified group exhibited improved toughness, maximal load to failure, and organized collagen bundles compared to controls (Dines et al., 2007). Tang et al. utilized ultrasound-targeted microbubble destruction (UTMD), a novel gene delivery strategy with high transfection efficiency and low immunogenicity, to deliver IGF-1 cDNA into rat Achilles tendons (Zhang et al., 2021). UTMD-mediated IGF-1 transfection enhanced inflammatory cell infiltration, reduced scar tissue formation, and improved maximum load, stiffness, and ultimate stress at weeks 2 and 8 (Tang et al., 2015).
Collectively, these studies highlight the significant therapeutic potential of IGF-1 in tendon healing across various animal models. However, challenges such as metabolic side effects and short half-life necessitate innovative delivery strategies, including Pegylation and gene therapy, to optimize its clinical application.
Although the aforementioned studies demonstrate the therapeutic potential of IGF-1 in promoting tendon regenerative healing in vivo, the underlying mechanisms remain incompletely understood. To address this knowledge gap, in vitro cell and tissue culture studies have been conducted to elucidate the biological mechanisms by which IGF-1 enhances tendon repair. These investigations reveal that IGF-1 facilitates tendon regenerative healing through multiple pathways, including modulation of inflammatory responses, promotion of cell proliferation and migration, enhancement of collagen production, and induction of cell differentiation (Figure 3). These mechanisms will be discussed in detail in the following sections. However, it is important to note that the efficacy of IGF-1 is highly dependent on its dosage, which significantly influences its biological effects.
On the one hand, the inflammatory response, as the first stage of tendon healing, is necessary for injury tissue regenerative healing through killing pathogens and removing cell debris to prevent wound infection (Ribitsch et al., 2021). On the other hand, persistent inflammation inflicts scar tissue formation, resulting in poor remodeling and seriously hampers tendon regenerative healing (Wang et al., 2019b). During this phase, various inflamed cells invade the injured site and secreted several growth factors, mainly including IGF-1, VEGF, platelet-derived growth factor (PDGF), and TGF-β (Chartier et al., 2021). These growth factors diffuse into surrounding tissues and chemotactically attract neutrophils and monocytes into the damaged site. Subsequently, the monocytes differentiate into macrophages that regulate various healing processes (Huang et al., 2020). In fact, during the progression of the inflammatory process, macrophages have two different functional phenotypes, the M1 and M2 phenotypes (Wang et al., 2024). M1 macrophages are mainly activated by the innate immune system and are responsible for amplifying inflammatory reactions, while M2 macrophages are associated with the resolution of inflammation and tissue healing (Chen et al., 2021; Yang et al., 2022). Based on this, it has been proposed that enhancing the polarization of M1 to M2 macrophages could accelerate tendon regenerative healing. Potentially, the presence of IGF-1 in tendon regenerative healing has been reported to be associated with the activation of M2 macrophages (Prabhath et al., 2018). However, how IGF-1 affects M2 macrophages remains unknown. Further, in a study conducted by Kurtz et al., the Achilles tendons of 20 rats were transected, followed by an injection of the proinflammatory agent carrageenan. Subsequently, rhIGF-1 was applied to treat the injured site, and functional and biomechanical data were collected. It was found that the rats that received IGF-1 had a much less functional deficit induced by the carrageenan than rats that did not receive IGF-1, which suggests that IGF-1 may suppress the inflammatory responses during tendon healing (Kurtz et al., 1999).
Altogether, IGF-1 thus have the ability to both promote and dampen inflammatory response, suggesting a complicated regulatory influence on tendon healing. However, the mechanisms by which IGF-1 plays in the inflammatory response have been mostly elusive.
The limited number of resident tendon cells is one of the important reasons for the limited inherent regeneration ability of tendons (Chartier et al., 2021). Therefore, it is postulated that increasing the number of tenocytes within the injured site allows better tendon healing.
Several studies have confirmed that IGF-1 can enhance tenocyte proliferation and migration. For example, Costa et al. conducted a study to explore the effect of IGF-1 on three different cell populations of rabbit flexor tendons: synovial sheath, epitenon, and endotenon. Cell cultures were supplemented with IGF-1 (10, 50, and 100 ng/mL), and the results showed that cell proliferation was more significant in all cell types after IGF-1 stimulation compared to the control group (received no IGF-1). Moreover, the cells from the synovial sheath displayed a positive dose-response curve, whereas epitenon and endotenon were not dose-dependent and the highest values were reached at 10 and 100 ng/mL, respectively (Costa et al., 2006). These results suggest that the effect of IGF-1 on cell proliferation is cell-specific. Similar findings with IGF-1 (10, 50, and 100 ng/mL) treatment were noted using human tenocytes in vitro, leading to increased cell proliferation. However, this effect is not dose-dependent, and the highest values were reached with 50 ng/mL on day 3 after IGF-1 administration (Raghavan et al., 2012). A further study reported that tenocytes stimulated with IGF-1 (100 ng/mL) for 7 days exhibited a significantly increased cell proliferation compared to the tenocytes in cell groups cultured without IGF-1 (Durgam et al., 2012). Moreover, investigations on tenocytes of genetically modified mice further confirmed the effect of IGF-1 on cell proliferation. Given that many tenocytes expressing the gene scleraxis (SCX) play critical roles during tendon healing (Best et al., 2021), the researchers conditionally deleted the IGF-1R in SCX-expressing tenocytes by using a tamoxifen-inducible Cre-recombinase system. It was observed that mice that lack IGF-1R in their tenocytes displayed reduced cell proliferation compared to the control Scx-expressing IGF-1R-positive mice, suggesting that IGF-1 may have a role in enhancing cell proliferation. More importantly, it was also revealed that IGF-1 exerts its cell-proliferation-promoting effect through activating the PI3K the PI3K/protein kinase B and ERK pathways (Disser et al., 2019). Additionally, Caliari et al. also examined the effect of IGF-1 (10, 50, and 200 ng/mL) on equine tenocytes seeded on collagen-glycosaminoglycan scaffolds after 7 days. The results showed a dose-dependent effect of IGF-1 on tenocyte migration and proliferation compared to the non-supplemented group (Caliari and Harley, 2013). Separate extracts from epitenon and the internal compartment of avian flexor tendons can stimulate tenocyte DNA synthesis (mitotic activity); DNA synthesis was inhibited by an anti-IGF-1 antibody, suggesting that IGF-1 was the primary factor responsible for the extract’s mitogenic activity (Abrahamsson et al., 1991; Abrahamsson, 1997).
Overall, these results demonstrated the pivotal role of IGF-1 in enhancing cell proliferation and migration. However, limited studies have been conducted to understand the complete mechanisms of IGF-1 involved in cell proliferation and migration. Therefore, further research is warranted to examine the underlying mechanisms by which IGF-1 promotes cell proliferation during tendon healing.
During tendon healing, there is a unique pattern of collagen production. In the proliferative phase, type III collagen increases significantly to provide a “quick fix” for the injured site (Dietrich-Zagonel et al., 2021). During the remodeling phase, the tendon tissue replaces type III collagen with type I collagen to bring structure and strength to the damaged site (Maeda et al., 2022). Thus, of particular relevance considering that type III and type I collagen are pivotal for the healing processes of damaged tendons, there are several documentations suggesting a critical role for IGF-1 in the regulation of collagen production.
Rat tenocytes cultured with IGF-1 (5, 10, and 20 ng/mL) for 3 days displayed different responses. The 10 ng/mL of IGF-1 remarkably increased collagen production, while the 10 and 20 ng/mL of IGF-1 treatment did not significantly increase collagen production compared to the untreated cells (Musson et al., 2017). In another study using rabbit Achilles tenocytes, the administration of IGF-1 led to the increased expression of type I collagen gene at 0.1 and 1 ng/mL, followed by a decrease at 10 ng/mL (Rieber et al., 2023). Moreover, experiments from tendon explants of equine also confirmed the positive effect of IGF-1 on ECM production in horse tenocytes, as the administration of 250 mg/mL IGF-1 resulted in an increased collagen production (Dahlgren et al., 2001). Furthermore, it has been reported that tenocytes with IGF-1 (10, 50, and 200 ng/mL) displayed the increased expression of tenocyte-associated structural protein genes for type I collagen (COL1A2), and type III collagen (COL3A1), but this effect is not dose-dependent and highest values were achieved at 50 ng/mL (Caliari and Harley, 2013).
Furthermore, several studies examined the effect of IGF-1 on human tendons. For example, after systemic administration of GH for 14 days, human data have reported an increase in circulating IGF-1 and expression of IGF-1 in tendon tissue, accompanied by increased expression of tendon collagen in individuals (Vestergaard et al., 2012). This study suggests that IGF-1 may play a role in promoting collagen production in humans, but neither provides any direct proof for a causative effect nor is it able to identify whether IGF-I or rather GH is the regulating factor for collagen synthesis in human tendons. Subsequently, a stimulating effect of IGF-1 on collagen tissue in human tendon tissue is supported by in vivo studies, which have demonstrated that local administration of IGF-1 (1 mg) directly induced an elevation in collagen synthesis in human tendon tissue (Hansen et al., 2013). Moreover, Nielsen et al. reported that patients respond to IGF-1 injection (10 mg/mL), leading to an increased collagen production of patellar tendon tissue (Nielsen et al., 2024). Consistently, another study also confirmed these previous experiments by showing a main effect of 250 ng/mL IGF-1 to increase collagen production in 3D and different FBS enriched media, where the constructs were divided into four treatment groups: (1)0.5% FBS, (2) 0.5% FBS+250 ng/mL IGF-1, (3) 10% FBS, (4) 10% FBS+250 ng/mL IGF-1. The results showed that in both IGF-1 treated-group, collagen was significantly increased at day 28 compared to non-IGF-1 supplementation groups (Herchenhan et al., 2015).
The above findings combined to demonstrate that IGF-1 is sufficient to increase collagen production in tenocytes. However, the underlying mechanisms of IGF-1-induced collagen production still remain elusive and therefore warrant further investigations.
Current regenerative research has focused on the studies of growth factor supplementation that can induce stem cells into tenogenic lineage for an alternative cell source to replenish functional tendon cells at the injured site (Zhang et al., 2018). Mesenchymal stem cells (MSCs) and tendon stem/progenitor cells (TSPCs) have received increasing attention toward tenogenic differentiation and tendon regeneration (Wang et al., 2022). IGF-1 has been advocated to induce tenogenic differentiation of stem cells. For example, rat TDSCs treated with IGF-1 (1, 10, and 100 ng/mL) for 28 days exhibited the increased expression of tendon-specific marker (SCX) and significantly decreased expression of chondrogenic and osteogenic marker (collagen type II) (Holladay et al., 2016). Moreover, treatment with 10 ng/mL of IGF-1 for 10 days was demonstrated to be sufficient to induce tenogenic differentiation of bone marrow MSCs in 3D in vitro culture with significant upregulation of tenocytic markers (SCX, collagen type III, and decorin) (Durgam et al., 2012). These studies showed that IGF-1 can induce tenogenic differentiation of TSPCs and bone marrow MSCs. However, to date, no in vivo data are available regarding the efficacy of exogenously delivery of IGF-1 in combination with stem cells to treat tendon injury. Thus, future research is needed to determine the effect of the combination of IGF-1 and stem cells that leads to a regenerated tissue mimicking the intact tendon.
Although numerous studies have demonstrated the potential of IGF-1 in advancing tendon treatment strategies, significant challenges remain in developing an effective IGF-1-based therapy for tendon regenerative healing.
One major limitation is the short half-life of growth factors under physiological conditions. When directly injected, IGF-1 rapidly diffuses away from the injury site, making it difficult to maintain therapeutically effective concentrations (Caballero Aguilar et al., 2019). Consequently, high dosages or repeated injections are often required, which not only increase treatment costs but also raise the risk of adverse effects (Muller et al., 2015). These limitations underscore the need for advanced delivery systems that can localize and sustain IGF-1 at the injury site (Yang et al., 2021). While gene therapy and tissue-engineered scaffolds offer promising alternatives (as discussed earlier), further optimization of these platforms is essential for successful clinical translation.
Secondly, despite its promising results in vitro and in animal models, clinical trials have not consistently shown a significant role for IGF-1 in promoting tendon repair (Olesen et al., 2021). This discrepancy could be attributed to multiple factors. In clinical practice, patients are a heterogeneous group with diverse genetic backgrounds, lifestyles, and underlying health conditions. These individual differences may influence the body’s response to IGF-1. For example, genetic polymorphisms in the genes encoding IGF-1 receptors or components of its signaling pathway may lead to variations in the effectiveness of IGF-1 therapy (Cubbon et al., 2016). Some patients may have mutations or polymorphisms that result in reduced receptor affinity for IGF-1 or impaired downstream signaling, thus limiting the therapeutic effect (Weroha and Haluska, 2008). Additionally, the complex in vivo environment in humans is difficult to replicate in laboratory settings. Inflammation, immune responses, and the presence of other biological factors in the human body can interact with IGF-1 in ways that are not fully understood, potentially dampening its efficacy.
Another critical area requiring further investigation is the optimization of IGF-1 dosage, which is essential for its successful application in tendon injury treatment. As previously noted, studies have employed varying dosages, such as 25 μg, 0.2 μg, and 8 mg/mL, in animal models, yielding inconsistent effects on tendon regeneration. For instance, a high dosage of IGF-1 (100 ng/mL) was found to be detrimental in a mouse study, inducing ectopic ossification and impairing Achilles tendon healing. Conversely, lower dosages have demonstrated therapeutic potential in promoting tendon repair in animal models (Mao et al., 2024). However, translating these findings to human patients presents significant challenges due to the complexity of human physiology, including factors such as body size, metabolic rate, and individual variability. Additionally, the optimal dosage may vary depending on injury-specific factors, such as location, severity, and chronicity. Therefore, future research should focus on establishing standardized dosing protocols through well-designed preclinical and clinical studies, considering both biological and injury-related variables to maximize the therapeutic efficacy of IGF-1 in tendon repair.
Additionally, given the complex and multifaceted nature of tendon healing, it is likely that delivering combinations of growth factors will result in the most beneficial outcomes for tendon regenerative healing. For example, Xiang et al. used UTMD to transfect IGF-1 cDNA and TGF-β short hairpin RNA (shRNA) into a rat model of Achilles tendon repair. They found that the combination of these growth factors displayed a more significant maximum load and tensile stress and inhibited scar formation compared to the IGF-1 alone group, suggesting that combination of IGF-1 and TGF-β is more effective than IGF-1 alone in the treatment of tendon injury (Xiang et al., 2018). Thus, future research is needed to determine the optimum combination of IGF-1 and other growth factors, making full use of the synergistic effects of various growth factors to induce their therapeutic potential and, thus, better regulate tendon healing.
Moreover, the efficacy of IGF-1 therapy can be influenced by factors such as age, health conditions, and injury type. In aged tendons, tenocyte activity is impaired, and functionally compromised pro-inflammatory tenocytes accumulate (Korcari et al., 2023). These age-related alterations may disrupt IGF-1 signal transduction, hinder downstream signaling, and reduce tenocyte responsiveness, ultimately impairing tendon repair and regeneration. Similarly, health conditions such as obesity can alter the therapeutic outcome, as the pro-inflammatory microenvironment characterized by elevated cytokine levels in obese patients may interfere with IGF-1 signaling and impede tendon healing (Hjelholt et al., 2020). Furthermore, the type of tendon injury plays a critical role in determining the treatment response. While IGF-1 promotes cell proliferation and collagen synthesis in acute partial tears, its effectiveness in chronic tendinopathy is often limited due to persistent inflammation, tissue degeneration, fibrosis, and abnormal ECM composition, which collectively restrict IGF-1 diffusion and reduce cellular responsiveness. The response to IGF-1 treatment may vary depending on the type of tendon injury. In acute partial tears, IGF-1 has been shown to enhance cell proliferation and collagen synthesis (Miescher et al., 2023). However, in chronic tendinopathy, its efficacy may be compromised due to persistent inflammation, tissue degeneration, fibrosis, and abnormal ECM composition, which can impede IGF-1 diffusion and diminish the responsiveness of tendon cells (Tang et al., 2024). These adaptations emphasize that IGF-1 therapy cannot follow a universal protocol, but rather requires multidimensional optimization based on individual patient profiles and injury context. Future clinical trials should incorporate stratified randomization based on these key modifiers of treatment response.
Tendon injury is a major musculoskeletal disorder that causes pain, limited activity, significant disability, and lost productivity. Following a tendon injury, the tendon tissue fails to regenerate, often forming scar tissue. Currently, various therapeutic strategies for tendon injury are available, including conservative and surgical treatments. However, these treatments are not effective. Therefore, there is an urgent need to develop novel treatments for tendon injury. Over the last 2 decades, IGF-1 has been discovered providing a positive scientific basis for its use in treating tendon disorders. This review highlights the multifaceted role of IGF-1 in promoting tendon regenerative healing, including its ability to modulate inflammatory responses, stimulate cell proliferation and migration, and enhance ECM production. However, to fully realize its therapeutic potential, critical factors such as optimal dosage, timing of application, and delivery strategies must be further investigated and refined.
MW: Writing–original draft. JZ: Methodology, Writing–original draft. HL: Investigation, Software, Writing–review and editing. YL: Data curation, Writing–original draft. ZL: Methodology, Supervision, Writing–review and editing.
The author(s) declare that financial support was received for the research and/or publication of this article. Police physical Health monitoring and rehabilitation research center, Sichuan Police College (No. 202410A02); Sichuan Police College University-Level Education and Teaching Reform Project (No. 2024YB23); Luzhou Science and Technology Bureau Science and Technology Program (Project No. 2024KPP292); The Luzhou Social Sciences Application Research Quality Improvement Project (Project No. LZSK-GH087).
The authors declare that the research was conducted in the absence of any commercial or financial relationships that could be construed as a potential conflict of interest.
All claims expressed in this article are solely those of the authors and do not necessarily represent those of their affiliated organizations, or those of the publisher, the editors and the reviewers. Any product that may be evaluated in this article, or claim that may be made by its manufacturer, is not guaranteed or endorsed by the publisher.
Abrahamsson, S. O. (1997). Similar effects of recombinant human insulin-like growth factor-I and II on cellular activities in flexor tendons of young rabbits: experimental studies in vitro. J. Orthop. Res. 15 (2), 256–262. doi:10.1002/jor.1100150215
Abrahamsson, S. O., Lundborg, G., and Lohmander, L. S. (1991). Long-term explant culture of rabbit flexor tendon: effects of recombinant human insulin-like growth factor-I and serum on matrix metabolism. J. Orthop. Res. 9 (4), 503–515. doi:10.1002/jor.1100090406
Adjei-Sowah, E., Benoit, D. S. W., and Loiselle, A. E. (2023). Drug delivery approaches to improve tendon healing. Tissue Eng. Part B Rev. 29 (4), 369–386. doi:10.1089/ten.teb.2022.0188
Alim, M. A., Peterson, M., and Pejler, G. (2020). Do mast cells have a role in tendon healing and inflammation? Cells 9 (5), 1134. doi:10.3390/cells9051134
Al-Samerria, S., and Radovick, S. (2021). The role of insulin-like growth factor-1 (IGF-1) in the control of neuroendocrine regulation of growth. Cells 10 (10), 2664. doi:10.3390/cells10102664
Bailes, J., and Soloviev, M. (2021). Insulin-like growth factor-1 (IGF-1) and its monitoring in medical diagnostic and in sports. Biomolecules 11 (2), 217. doi:10.3390/biom11020217
Berglund, M. E., Hart, D. A., Reno, C., and Wiig, M. (2011). Growth factor and protease expression during different phases of healing after rabbit deep flexor tendon repair. J. Orthop. Res. 29 (6), 886–892. doi:10.1002/jor.21330
Best, K. T., Korcari, A., Mora, K. E., Nichols, A. E., Muscat, S. N., Knapp, E., et al. (2021). Scleraxis-lineage cell depletion improves tendon healing and disrupts adult tendon homeostasis. Elife 10, e62203. doi:10.7554/elife.62203
Boye, C., Christensen, K., Asadipour, K., DeClemente, S., Francis, M., and Bulysheva, A. (2022). Gene electrotransfer of FGF2 enhances collagen scaffold biocompatibility. Bioelectrochemistry 144, 107980. doi:10.1016/j.bioelechem.2021.107980
Caballero Aguilar, L. M., Silva, S. M., and Moulton, S. E. (2019). Growth factor delivery: defining the next generation platforms for tissue engineering. J. Control Release 306, 40–58. doi:10.1016/j.jconrel.2019.05.028
Caliari, S. R., and Harley, B. A. (2013). Composite growth factor supplementation strategies to enhance tenocyte bioactivity in aligned collagen-GAG scaffolds. Tissue Eng. Part A 19 (9-10), 1100–1112. doi:10.1089/ten.tea.2012.0497
Chartier, C., ElHawary, H., Baradaran, A., Vorstenbosch, J., Xu, L., and Efanov, J. I. (2021). Tendon: principles of healing and repair. Semin. Plast. Surg. 35 (3), 211–215. doi:10.1055/s-0041-1731632
Chen, C. H., Cao, Y., Wu, Y. F., Bais, A. J., Gao, J. S., and Tang, J. B. (2008). Tendon healing in vivo: gene expression and production of multiple growth factors in early tendon healing period. J. Hand Surg. Am. 33 (10), 1834–1842. doi:10.1016/j.jhsa.2008.07.003
Chen, W., Sun, Y., Gu, X., Cai, J., Liu, X., Zhang, X., et al. (2021). Conditioned medium of human bone marrow-derived stem cells promotes tendon-bone healing of the rotator cuff in a rat model. Biomaterials 271, 120714. doi:10.1016/j.biomaterials.2021.120714
Costa, M. A., Wu, C., Pham, B. V., Chong, A. K., Pham, H. M., and Chang, J. (2006). Tissue engineering of flexor tendons: optimization of tenocyte proliferation using growth factor supplementation. Tissue Eng. 12 (7), 1937–1943. doi:10.1089/ten.2006.12.1937
Cubbon, R. M., Kearney, M. T., and Wheatcroft, S. B. (2016). Endothelial IGF-1 receptor signalling in diabetes and insulin resistance. Trends Endocrinol. Metab. 27 (2), 96–104. doi:10.1016/j.tem.2015.11.009
Dahlgren, L. A., Mohammed, H. O., and Nixon, A. J. (2005). Temporal expression of growth factors and matrix molecules in healing tendon lesions. J. Orthop. Res. 23 (1), 84–92. doi:10.1016/j.orthres.2004.05.007
Dahlgren, L. A., Nixon, A. J., and Brower-Toland, B. D. (2001). Effects of beta-aminopropionitrile on equine tendon metabolism in vitro and on effects of insulin-like growth factor-I on matrix production by equine tenocytes. Am. J. Vet. Res. 62 (10), 1557–1562. doi:10.2460/ajvr.2001.62.1557
Dahlgren, L. A., van der Meulen, M. C., Bertram, J. E., Starrak, G. S., and Nixon, A. J. (2002). Insulin-like growth factor-I improves cellular and molecular aspects of healing in a collagenase-induced model of flexor tendinitis. J. Orthop. Res. 20 (5), 910–919. doi:10.1016/s0736-0266(02)00009-8
Deng, X., Li, Q., Yuan, H., Hu, H., and Fan, S. (2024). Galangin promotes tendon repair mediated by tendon-derived stem cells through activating the TGF-β1/smad3 signaling pathway. Chem. Pharm. Bull. (Tokyo) 72 (7), 669–675. doi:10.1248/cpb.c24-00117
Dietrich-Zagonel, F., Hammerman, M., Bernhardsson, M., and Eliasson, P. (2021). Effect of storage and preconditioning of healing rat Achilles tendon on structural and mechanical properties. Sci. Rep. 11 (1), 958. doi:10.1038/s41598-020-80299-w
Dines, J. S., Grande, D. A., and Dines, D. M. (2007). Tissue engineering and rotator cuff tendon healing. J. Shoulder Elb. Surg. 16 (5 Suppl. l), S204–S207. doi:10.1016/j.jse.2007.03.004
Disser, N. P., Sugg, K. B., Talarek, J. R., Sarver, D. C., Rourke, B. J., and Mendias, C. L. (2019). Insulin-like growth factor 1 signaling in tenocytes is required for adult tendon growth. FASEB J. 33 (11), 12680–12695. doi:10.1096/fj.201901503r
Durgam, S. S., Stewart, A. A., Pondenis, H. C., Gutierrez-Nibeyro, S. M., Evans, R. B., and Stewart, M. C. (2012). Comparison of equine tendon- and bone marrow-derived cells cultured on tendon matrix with or without insulin-like growth factor-I supplementation. Am. J. Vet. Res. 73 (1), 153–161. doi:10.2460/ajvr.73.1.153
El Essawy, E. S., and Baar, K. (2023). Rapamycin insensitive regulation of engineered ligament structure and function by IGF-1. J. Appl. Physiol. 135 (4), 833–839. doi:10.1152/japplphysiol.00593.2022
Geffken, S. J., Moon, S., Smith, C. O., Tang, S., Lee, H. H., Lewis, K., et al. (2022). Insulin and IGF-1 elicit robust transcriptional regulation to modulate autophagy in astrocytes. Mol. Metab. 66, 101647. doi:10.1016/j.molmet.2022.101647
Gulotta, L. V., and Rodeo, S. A. (2009). Growth factors for rotator cuff repair. Clin. Sports Med. 28 (1), 13–23. doi:10.1016/j.csm.2008.09.002
Guo, D., Li, H., Liu, Y., Yu, X., Zhang, X., Chu, W., et al. (2020). Fibroblast growth factor-2 promotes the function of tendon-derived stem cells in Achilles tendon restoration in an Achilles tendon injury rat model. Biochem. Biophys. Res. Commun. 521 (1), 91–97. doi:10.1016/j.bbrc.2019.10.082
Hansen, M., Boesen, A., Holm, L., Flyvbjerg, A., Langberg, H., and Kjaer, M. (2013). Local administration of insulin-like growth factor-I (IGF-I) stimulates tendon collagen synthesis in humans. Scand. J. Med. Sci. Sports 23 (5), 614–619. doi:10.1111/j.1600-0838.2011.01431.x
Hansson, H. A., Dahlin, L. B., Lundborg, G., Lowenadler, B., Paleus, S., and Skottner, A. (1988). Transiently increased insulin-like growth factor I immunoreactivity in tendons after vibration trauma. An immunohistochemical study on rats. Scand. J. Plast. Reconstr. Surg. Hand Surg. 22 (1), 1–6. doi:10.3109/02844318809097928
Herchenhan, A., Bayer, M. L., Eliasson, P., Magnusson, S. P., and Kjaer, M. (2015). Insulin-like growth factor I enhances collagen synthesis in engineered human tendon tissue. Growth Horm. IGF Res. 25 (1), 13–19. doi:10.1016/j.ghir.2014.09.001
Hjelholt, A., Hogild, M., Bak, A. M., Arlien-Søborg, M. C., Bæk, A., Jessen, N., et al. (2020). Growth hormone and obesity. Endocrinol. Metab. Clin. North Am. 49 (2), 239–250. doi:10.1016/j.ecl.2020.02.009
Holladay, C., Abbah, S. A., O'Dowd, C., Pandit, A., and Zeugolis, D. I. (2016). Preferential tendon stem cell response to growth factor supplementation. J. Tissue Eng. Regen. Med. 10 (9), 783–798. doi:10.1002/term.1852
Huang, Y., He, B., Wang, L., Yuan, B., Shu, H., Zhang, F., et al. (2020). Bone marrow mesenchymal stem cell-derived exosomes promote rotator cuff tendon-bone healing by promoting angiogenesis and regulating M1 macrophages in rats. Stem Cell Res. Ther. 11 (1), 496. doi:10.1186/s13287-020-02005-x
Jin, J., Yang, Q. Q., and Zhou, Y. L. (2022). Non-viral delivery of gene therapy to the tendon. Polym. (Basel) 14 (16), 3338. doi:10.3390/polym14163338
Jozefiak, A., Larska, M., Pomorska-Mol, M., and Ruszkowski, J. J. (2021). The IGF-1 signaling pathway in viral infections. Viruses 13 (8), 1488. doi:10.3390/v13081488
Kobayashi, M., Itoi, E., Minagawa, H., Miyakoshi, N., Takahashi, S., Tuoheti, Y., et al. (2006). Expression of growth factors in the early phase of supraspinatus tendon healing in rabbits. J. Shoulder Elb. Surg. 15 (3), 371–377. doi:10.1016/j.jse.2005.09.003
Korcari, A., Buckley, M. R., and Loiselle, A. E. (2022). Characterization of scar tissue biomechanics during adult murine flexor tendon healing. J. Mech. Behav. Biomed. Mater 130, 105192. doi:10.1016/j.jmbbm.2022.105192
Korcari, A., Przybelski, S. J., Gingery, A., and Loiselle, A. E. (2023). Impact of aging on tendon homeostasis, tendinopathy development, and impaired healing. Connect. Tissue Res. 64 (1), 1–13. doi:10.1080/03008207.2022.2102004
Kosiol, J., Keiler, A., Loizides, A., Gruber, H., Henninger, B., Bölderl, A., et al. (2023). Operative versus conservative treatment of acute Achilles tendon ruptures: preliminary results of clinical outcome, kinematic MRI and contrast-enhanced ultrasound. Arch. Orthop. Trauma Surg. 143 (5), 2455–2465. doi:10.1007/s00402-022-04457-7
Kurtz, C. A., Loebig, T. G., Anderson, D. D., DeMeo, P. J., and Campbell, P. G. (1999). Insulin-like growth factor I accelerates functional recovery from Achilles tendon injury in a rat model. Am. J. Sports Med. 27 (3), 363–369. doi:10.1177/03635465990270031701
Lai, F., Wang, J., Tang, H., Huang, P., Liu, J., He, G., et al. (2022). VEGF promotes tendon regeneration of aged rats by inhibiting adipogenic differentiation of tendon stem/progenitor cells and promoting vascularization. FASEB J. 36 (8), e22433. doi:10.1096/fj.202200213R
Leahy, T. P., Fung, A. K., Weiss, S. N., Dyment, N. A., and Soslowsky, L. J. (2023). Investigating the temporal roles of decorin and biglycan in tendon healing. J. Orthop. Res. 41 (10), 2238–2249. doi:10.1002/jor.25590
Leong, N. L., Kator, J. L., Clemens, T. L., James, A., Enamoto-Iwamoto, M., and Jiang, J. (2020). Tendon and ligament healing and current approaches to tendon and ligament regeneration. J. Orthop. Res. 38 (1), 7–12. doi:10.1002/jor.24475
Li, H., Li, Y., Luo, S., Zhang, Y., Feng, Z., and Li, S. (2024). The roles and mechanisms of the NF-κB signaling pathway in tendon disorders. Front. Vet. Sci. 11, 1382239. doi:10.3389/fvets.2024.1382239
Li, H., Luo, S., Wang, H., Chen, Y., Ding, M., Lu, J., et al. (2023b). The mechanisms and functions of TGF-β1 in tendon healing. Injury 54 (11), 111052. doi:10.1016/j.injury.2023.111052
Li, J., Weber, E., Guth-Gundel, S., Schuleit, M., Kuttler, A., Halleux, C., et al. (2018). Tough composite hydrogels with high loading and local release of biological drugs. Adv. Healthc. Mater 7 (9), e1701393. doi:10.1002/adhm.201701393
Li, S., Gong, F., Zhou, Z., and Gong, X. (2023a). Combined verapamil-polydopamine nanoformulation inhibits adhesion formation in achilles tendon injury using rat model. Int. J. Nanomedicine 18, 115–126. doi:10.2147/ijn.s377600
Liang, W., Zhou, C., Deng, Y., Fu, L., Zhao, J., Long, H., et al. (2024). The current status of various preclinical therapeutic approaches for tendon repair. Ann. Med. 56 (1), 2337871. doi:10.1080/07853890.2024.2337871
Liang, Y., Zhang, C., Wei, H., Du, H., Zhang, G., Yang, Y., et al. (2022). The pharmacokinetic and pharmacodynamic properties and short-term outcome of a novel once-weekly PEGylated recombinant human growth hormone for children with growth hormone deficiency. Front. Endocrinol. (Lausanne) 13, 922304. doi:10.3389/fendo.2022.922304
Ling, Q., Herstine, J. A., Bradbury, A., and Gray, S. J. (2023). AAV-based in vivo gene therapy for neurological disorders. Nat. Rev. Drug Discov. 22 (10), 789–806. doi:10.1038/s41573-023-00766-7
Liu, X., Zhu, B., Li, Y., Liu, X., Guo, S., Wang, C., et al. (2021). The role of vascular endothelial growth factor in tendon healing. Front. Physiol. 12, 766080. doi:10.3389/fphys.2021.766080
Lygren, T., Schjerling, P., Jacobsen, S., Berg, L. C., Nielsen, M. O., Langberg, H., et al. (2013). Validation of the IDS Octeia ELISA for the determination of insulin-like growth factor 1 in equine serum and tendon tissue extracts. Vet. Clin. Pathol. 42 (2), 184–189. doi:10.1111/vcp.12038
Lyras, D. N., Kazakos, K., Agrogiannis, G., Verettas, D., Kokka, A., Kiziridis, G., et al. (2010). Experimental study of tendon healing early phase: is IGF-1 expression influenced by platelet rich plasma gel? Orthop. Traumatol. Surg. Res. 96 (4), 381–387. doi:10.1016/j.otsr.2010.03.010
Macvanin, M., Gluvic, Z., Radovanovic, J., Essack, M., Gao, X., and Isenovic, E. R. (2023). New insights on the cardiovascular effects of IGF-1. Front. Endocrinol. (Lausanne) 14, 1142644. doi:10.3389/fendo.2023.1142644
Maeda, E., Kawamura, R., Suzuki, T., and Matsumoto, T. (2022). Rapid fabrication of tendon-like collagen gel via simultaneous fibre alignment and intermolecular cross-linking under mechanical loading. Biomed. Mater 17 (4), 045018. doi:10.1088/1748-605x/ac7305
Mao, D., Wang, K., Jiang, H., Mi, J., Pan, X., Zhao, G., et al. (2024). Suppression of overactive insulin-like growth factor 1 attenuates trauma-induced heterotopic ossification in mice. Am. J. Pathol. 194 (3), 430–446. doi:10.1016/j.ajpath.2023.11.012
Matsushita, M., Fujita, K., Hatano, K., De Velasco, M. A., Uemura, H., and Nonomura, N. (2022). Connecting the dots between the gut-IGF-1-prostate Axis: a role of IGF-1 in prostate carcinogenesis. Front. Endocrinol. (Lausanne) 13, 852382. doi:10.3389/fendo.2022.852382
Miescher, I., Rieber, J., Calcagni, M., and Buschmann, J. (2023). In vitro and in vivo effects of IGF-1 delivery strategies on tendon healing: a review. Int. J. Mol. Sci. 24 (3), 2370. doi:10.3390/ijms24032370
Muller, S. A., Todorov, A., Heisterbach, P. E., Martin, I., and Majewski, M. (2015). Tendon healing: an overview of physiology, biology, and pathology of tendon healing and systematic review of state of the art in tendon bioengineering. Knee Surg. Sports Traumatol. Arthrosc. 23 (7), 2097–2105. doi:10.1007/s00167-013-2680-z
Muraguchi, T., Nanba, D., Nishimura, E. K., and Tashiro, T. (2019). IGF-1R deficiency in human keratinocytes disrupts epidermal homeostasis and stem cell maintenance. J. Dermatol Sci. 94 (2), 298–305. doi:10.1016/j.jdermsci.2019.05.001
Musson, D. S., Tay, M. L., Chhana, A., Pool, B., Coleman, B., Naot, D., et al. (2017). Lactoferrin and parathyroid hormone are not harmful to primary tenocytes in vitro, but PDGF may be. Muscles Ligaments Tendons J. 7 (2), 215–222. doi:10.32098/mltj.02.2017.03
Nielsen, R. H., Holm, L., Jensen, J. K., Heinemeier, K. M., Remvig, L., and Kjaer, M. (2014). Tendon protein synthesis rate in classic Ehlers-Danlos patients can be stimulated with insulin-like growth factor-I. J. Appl. Physiol. 117 (7), 694–698. doi:10.1152/japplphysiol.00157.2014
Olesen, J. L., Hansen, M., Turtumoygard, I. F., Hoffner, R., Schjerling, P., Christensen, J., et al. (2021). No treatment benefits of local administration of insulin-like growth factor-1 in addition to heavy slow resistance training in tendinopathic human patellar tendons: a randomized, double-blind, placebo-controlled trial with 1-year follow-up. Am. J. Sports Med. 49 (9), 2361–2370. doi:10.1177/03635465211021056
Oxvig, C., and Conover, C. A. (2023). The stanniocalcin-PAPP-A-IGFBP-IGF Axis. J. Clin. Endocrinol. Metab. 108 (7), 1624–1633. doi:10.1210/clinem/dgad053
Perks, C. M. (2023). Role of the insulin-like growth factor (IGF) Axis in diseases. Int. J. Mol. Sci. 24 (23), 16969. doi:10.3390/ijms242316969
Prabhath, A., Vernekar, V. N., Esdaille, C. J., Eisenberg, E., Lebaschi, A., Badon, M., et al. (2022). Pegylated insulin-like growth factor-1 biotherapeutic delivery promotes rotator cuff regeneration in a rat model. J. Biomed. Mater Res. A 110 (7), 1356–1371. doi:10.1002/jbm.a.37378
Prabhath, A., Vernekar, V. N., Sanchez, E., and Laurencin, C. T. (2018). Growth factor delivery strategies for rotator cuff repair and regeneration. Int. J. Pharm. 544 (2), 358–371. doi:10.1016/j.ijpharm.2018.01.006
Raghavan, S. S., Woon, C. Y. L., Kraus, A., Megerle, K., Pham, H., and Chang, J. (2012). Optimization of human tendon tissue engineering: synergistic effects of growth factors for use in tendon scaffold repopulation. Plast. Reconstr. Surg. 129 (2), 479–489. doi:10.1097/prs.0b013e31823aeb94
Reda, Y., Farouk, A., Abdelmonem, I., and El Shazly, O. A. (2020). Surgical versus non-surgical treatment for acute Achilles' tendon rupture. A systematic review of literature and meta-analysis. Foot Ankle Surg. 26 (3), 280–288. doi:10.1016/j.fas.2019.03.010
Ribitsch, I., Bileck, A., Aldoshin, A. D., Kańduła, M. M., Mayer, R. L., Egerbacher, M., et al. (2021). Molecular mechanisms of fetal tendon regeneration versus adult fibrous repair. Int. J. Mol. Sci. 22 (11), 5619. doi:10.3390/ijms22115619
Rieber, J., Meier-Burgisser, G., Miescher, I., Weber, F. E., Wolint, P., Yao, Y., et al. (2023). Bioactive and elastic emulsion electrospun DegraPol tubes delivering IGF-1 for tendon rupture repair. Int. J. Mol. Sci. 24 (12), 10272. doi:10.3390/ijms241210272
Rieger, L., and O'Connor, R. (2020). Controlled signaling-insulin-like growth factor receptor endocytosis and presence at intracellular compartments. Front. Endocrinol. (Lausanne) 11, 620013. doi:10.3389/fendo.2020.620013
Roberts, R. E., Cavalcante-Silva, J., Del Rio-Moreno, M., Bilgen, O., Kineman, R. D., and Koh, T. J. (2023). Liver insulin-like growth factor-1 mediates effects of low-intensity vibration on wound healing in diabetic mice. J. Pathol. 260 (1), 97–107. doi:10.1002/path.6068
Scott, A., Cook, J. L., Hart, D. A., Walker, D. C., Duronio, V., and Khan, K. M. (2007). Tenocyte responses to mechanical loading in vivo: a role for local insulin-like growth factor 1 signaling in early tendinosis in rats. Arthritis Rheum. 56 (3), 871–881. doi:10.1002/art.22426
Scott, A., Khan, K. M., and Duronio, V. (2005). IGF-I activates PKB and prevents anoxic apoptosis in Achilles tendon cells. J. Orthop. Res. 23 (5), 1219–1225. doi:10.1016/j.orthres.2004.12.011
Seow, D., Islam, W., Randall, G. W., Azam, M. T., Duenes, M. L., Hui, J., et al. (2023). Lower re-rupture rates but higher complication rates following surgical versus conservative treatment of acute achilles tendon ruptures: a systematic review of overlapping meta-analyses. Knee Surg. Sports Traumatol. Arthrosc. 31 (8), 3528–3540. doi:10.1007/s00167-023-07411-1
Silbernagel, K. G., Hanlon, S., and Sprague, A. (2020). Current clinical concepts: conservative management of achilles tendinopathy. J. Athl. Train. 55 (5), 438–447. doi:10.4085/1062-6050-356-19
Tang, C., Wang, Z., Xie, Y., Fei, Y., Luo, J., Wang, C., et al. (2024). Classification of distinct tendinopathy subtypes for precision therapeutics. Nat. Commun. 15 (1), 9460. doi:10.1038/s41467-024-53826-w
Tang, X. M., Dai, J., and Sun, H. L. (2019). Thermal pretreatment promotes the protective effect of HSP70 against tendon adhesion in tendon healing by increasing HSP70 expression. Mol. Med. Rep. 20 (1), 205–215. doi:10.3892/mmr.2019.10240
Tang, Y., Leng, Q., Xiang, X., Zhang, L., Yang, Y., and Qiu, L. (2015). Use of ultrasound-targeted microbubble destruction to transfect IGF-1 cDNA to enhance the regeneration of rat wounded Achilles tendon in vivo. Gene Ther. 22 (8), 610–618. doi:10.1038/gt.2015.32
Thomopoulos, S., Das, R., Sakiyama-Elbert, S., Silva, M. J., Charlton, N., and Gelberman, R. H. (2010). bFGF and PDGF-BB for tendon repair: controlled release and biologic activity by tendon fibroblasts in vitro. Ann. Biomed. Eng. 38 (2), 225–234. doi:10.1007/s10439-009-9844-5
Tian, Y., Miao, Y., Guo, P., Wang, J., and Han, D. (2024). Insulin-like growth factor 2-tagged aptamer chimeras (ITACs) modular assembly for targeted and efficient degradation of two membrane proteins. Angew. Chem. Int. Ed. Engl. 63 (5), e202316089. doi:10.1002/anie.202316089
Tsubone, T., Moran, S. L., Amadio, P. C., Zhao, C., and An, K. N. (2004). Expression of growth factors in canine flexor tendon after laceration in vivo. Ann. Plast. Surg. 53 (4), 393–397. doi:10.1097/01.sap.0000125501.72773.01
Vestergaard, P., Jorgensen, J. O., Olesen, J. L., Bosnjak, E., Holm, L., Frystyk, J., et al. (2012). Local administration of growth hormone stimulates tendon collagen synthesis in elderly men. J. Appl. Physiol. 113 (9), 1432–1438. doi:10.1152/japplphysiol.00816.2012
Wang, H., Yu, R., Wang, M., Wang, S., Ouyang, X., Yan, Z., et al. (2023b). Insulin-like growth factor binding protein 4 loaded electrospun membrane ameliorating tendon injury by promoting retention of IGF-1. J. Control Release 356, 162–174. doi:10.1016/j.jconrel.2023.02.039
Wang, H. N., Rong, X., Yang, L. M., Hua, W. Z., and Ni, G. X. (2022). Advances in stem cell therapies for rotator cuff injuries. Front. Bioeng. Biotechnol. 10, 866195. doi:10.3389/fbioe.2022.866195
Wang, J., Zhu, Q., Cao, D., Peng, Q., Zhang, X., Li, C., et al. (2023a). Bone marrow-derived IGF-1 orchestrates maintenance and regeneration of the adult skeleton. Proc. Natl. Acad. Sci. U. S. A. 120 (1), e2203779120. doi:10.1073/pnas.2203779120
Wang, S., Chi, K., Wu, D., and Hong, Q. (2021). Insulin-like growth factor binding proteins in kidney disease. Front. Pharmacol. 12, 807119. doi:10.3389/fphar.2021.807119
Wang, Y., He, G., Tang, H., Shi, Y., Kang, X., Lyu, J., et al. (2019b). Aspirin inhibits inflammation and scar formation in the injury tendon healing through regulating JNK/STAT-3 signalling pathway. Cell Prolif. 52 (4), e12650. doi:10.1111/cpr.12650
Wang, Y., He, G., Wang, F., Zhang, C., Ge, Z., Zheng, X., et al. (2019a). Aspirin inhibits adipogenesis of tendon stem cells and lipids accumulation in rat injury tendon through regulating PTEN/PI3K/AKT signalling. J. Cell Mol. Med. 23 (11), 7535–7544. doi:10.1111/jcmm.14622
Wang, Y., Lu, X., Lu, J., Hernigou, P., and Jin, F. (2024). The role of macrophage polarization in tendon healing and therapeutic strategies: insights from animal models. Front. Bioeng. Biotechnol. 12, 1366398. doi:10.3389/fbioe.2024.1366398
Werner, H. (2023). The IGF1 signaling pathway: from basic concepts to therapeutic opportunities. Int. J. Mol. Sci. 24 (19), 14882. doi:10.3390/ijms241914882
Weroha, S. J., and Haluska, P. (2008). IGF-1 receptor inhibitors in clinical trials--early lessons. J. Mammary Gland. Biol. Neoplasia 13 (4), 471–483. doi:10.1007/s10911-008-9104-6
Wu, T., Qi, W., Shan, H., Tu, B., Jiang, S., Lu, Y., et al. (2022). Ginsenoside Rg1 enhances the healing of injured tendon in achilles tendinitis through the activation of IGF1R signaling mediated by oestrogen receptor. J. Ginseng Res. 46 (4), 526–535. doi:10.1016/j.jgr.2021.08.005
Xiang, X., Leng, Q., Tang, Y., Wang, L., Huang, J., Zhang, Y., et al. (2018). Ultrasound-targeted microbubble destruction delivery ofInsulin-like growth factor 1cDNA andTransforming growth factor BetaShort hairpin RNA enhances tendon regeneration and inhibits scar formationin vivo. Hum. Gene Ther. Clin. Dev. 29 (4), 198–213. doi:10.1089/humc.2018.121
Yang, Q. Q., Zhang, L., Ju, F., and Zhou, Y. L. (2021). Sustained-release hydrogel-based rhynchophylline delivery system improved injured tendon repair. Colloids Surf. B Biointerfaces 205, 111876. doi:10.1016/j.colsurfb.2021.111876
Yang, Q. Q., Zhang, L., Zhou, Y. L., and Tang, J. B. (2022). Morphological changes of macrophages and their potential contribution to tendon healing. Colloids Surf. B Biointerfaces 209 (Pt 1), 112145. doi:10.1016/j.colsurfb.2021.112145
Zerti, D., Molina, M. M., Dorgau, B., Mearns, S., Bauer, R., Al-Aama, J., et al. (2021). IGFBPs mediate IGF-1's functions in retinal lamination and photoreceptor development during pluripotent stem cell differentiation to retinal organoids. Stem Cells 39 (4), 458–466. doi:10.1002/stem.3331
Zhang, B., Brahma, R. K., Zhu, L., Feng, J., Hu, S., Qian, L., et al. (2023). Insulin-like growth factor 2 (IGF2)-Fused lysosomal targeting chimeras for degradation of extracellular and membrane proteins. J. Am. Chem. Soc. 145 (44), 24272–24283. doi:10.1021/jacs.3c08886
Zhang, C., Chen, S., Li, Q., Wu, J., Qiu, F., Chen, Z., et al. (2021). Ultrasound-targeted microbubble destruction mediates gene transfection for beta-cell regeneration and glucose regulation. Small 17 (31), e2008177. doi:10.1002/smll.202008177
Zhang, Y. J., Chen, X., Li, G., Chan, K. M., Heng, B. C., Yin, Z., et al. (2018). Concise review: stem cell fate guided by bioactive molecules for tendon regeneration. Stem Cells Transl. Med. 7 (5), 404–414. doi:10.1002/sctm.17-0206
Keywords: tendon injury, IGF-1, tendon regenerative healing, tendon repair, tenocyte, mechanisms
Citation: Wang M, Zhang J, Li H, Li Y and Li Z (2025) Insulin-like growth factor-1 (IGF-1) empowering tendon regenerative therapies. Front. Bioeng. Biotechnol. 13:1492811. doi: 10.3389/fbioe.2025.1492811
Received: 11 September 2024; Accepted: 14 March 2025;
Published: 27 March 2025.
Edited by:
Leo R. Quinlan, University of Galway, IrelandReviewed by:
Chen Yu Huang, National Cheng Kung University, TaiwanCopyright © 2025 Wang, Zhang, Li, Li and Li. This is an open-access article distributed under the terms of the Creative Commons Attribution License (CC BY). The use, distribution or reproduction in other forums is permitted, provided the original author(s) and the copyright owner(s) are credited and that the original publication in this journal is cited, in accordance with accepted academic practice. No use, distribution or reproduction is permitted which does not comply with these terms.
*Correspondence: Zhigang Li, TGl6Z0Bzd211LmVkdS5jbg==
†ORCID ID: Zhigang Li, orcid.org/0009-0001-0254-8155
‡These authors have contributed equally to this work
Disclaimer: All claims expressed in this article are solely those of the authors and do not necessarily represent those of their affiliated organizations, or those of the publisher, the editors and the reviewers. Any product that may be evaluated in this article or claim that may be made by its manufacturer is not guaranteed or endorsed by the publisher.
Research integrity at Frontiers
Learn more about the work of our research integrity team to safeguard the quality of each article we publish.